Open Access
ARTICLE
Growth, Yield, Energetics, CO2 Emissions and Production Economics of Zero-Tillage Wheat as Influenced by Different Rice Residue Loads and Nutrient Management Options
1 Department of Agronomy, Uttar Banga Krishi Viswavidyalaya, Coochbehar, 736165, West Bengal, India
2 Department of Biology, Faculty of Science, Taif University, Taif, 21944, Saudi Arabia
3 Division of Soil Science, Bangladesh Wheat and Maize Research Institute, Dinajpur, 5200, Bangladesh
* Corresponding Author: Akbar Hossain. Email:
(This article belongs to the Special Issue: Integrated Nutrient Management in Cereal Crops)
Phyton-International Journal of Experimental Botany 2024, 93(12), 3509-3530. https://doi.org/10.32604/phyton.2024.056789
Received 31 July 2024; Accepted 06 November 2024; Issue published 31 December 2024
Abstract
The rice‒wheat farming system is considered as the greatest energy-intensive agricultural practice in South‒East Asia. In light of declining system production and profitability, burning of residues, soil erosion, depletion of renewable resources, and environmental degradation, the sustainability of the rice‒wheat cropping system is being questioned. As a result, energy and money efficient conservation agricultural methods are becoming more and more necessary to accomplish sustainable output. A field experiment was carried out in this regard to evaluate the effects of varying degrees of rice residue retention and nutrient management options on zero-tillage grown wheat growth, yield, phenology, economics, energy budgeting, and CO2 emissions. In the experiment, there were five different nutrient management options (recommended dose and Nutrient Expert based dose with and without seed inoculating biofertilizer Azotobacter and Phosphate Solubilizing Bacteria, PSB in short along with a control) and four different rice residue load treatments (rice residues retained at 0, 15, 30, and 45 cm from the ground level). The treatments that managed nutrients using Nutrient Expert (NE) and kept residues at a height of 15 and 30 cm above ground, both with and without biofertilizer inoculation, showed better growth and yield in both years. Higher net energy, energy productivity and energy use efficiency were achieved with the treatment combination that kept residues at a height of 30 cm and suggested nutrients using NE software or recommended fertilizer doses (RDF), which included inoculating seeds with biofertilizers. Under the treatments where fertilizers were given more often, there was a larger release of CO2. The treatment that kept residues at a height of 30 cm and used NE software to recommend nutrients along with seed inoculation of biofertilizers (R2N5) resulted in higher gross returns (₹111,315 ha−1 and ₹109,342 ha−1), net returns (₹60,957 ha−1 and ₹61,797 ha−1), and B:C (2.21 and 2.30) during 2021–2022 and 2022–2023, respectively. Therefore, maintaining residues at their ideal level, managing nutrients through NE, and incorporating biofertilizers into the seeds could be advantageous for wheat production and result in an energy-efficient and lucrative cropping system.Keywords
Supplementary Material
Supplementary Material FileIn the present era of ecological degradation, depleted resources and inadequate food supplies, energy consumption and greenhouse gas (GHG) emissions are associated with the long-term viability of farming systems. Energy assessment in agricultural systems could develop and improve eco-friendly and energy efficient agro-technologies [1,2]. Agricultural mechanization, increased use of fertilizers, and increased use of irrigation have all contributed to a notable increase in energy consumption in Indian agriculture [3]. Approximately 18% of India’s total GHG emissions are attributed to agriculture, primarily to the burning of crop residues, the conventional puddled transplanted rice system, the increased use of nitrogen fertilizers, and the raising of animals [4]. In the context of increasing uncertainty over scarce resources as well as changing climates, ensuring food security to feed an increasing population is essential. The current agricultural production system has to prioritize energy-efficient technology with a lower carbon footprint [5].
The rice (Oryza sativa L.)–wheat (Triticum aestivum L.) cropping system is India’s most prevalent agricultural and nutritional production system [6,7]. In India, the majority of rice is planted via wet tillage, which involves repeatedly puddled fields, whereas the succeeding wheat crop is sown on conventionally ploughed soils [8]. The conventional methods of growing rice and wheat over time have led to decreased rice and wheat productivity with increasing cultivation costs, thus resulting in decreasing profit margins, decreasing factor productivity and overall soil health [9]. The current rice-wheat production system ultimately jeopardizes the overall sustainability of the soil [10].
A site-specific approach known as conservation agriculture (CA) seeks to address problems with conventional agricultural systems by optimizing resource use efficiency and conserving resources [11,12]. Although one of the fundamental tenets of CA is to retain at least 30% of residues, it is yet unknown what would happen to soil and crop yields if residues are retained to a greater or lesser extent. Furthermore, accurate nutritional management of wheat needs to be considered from energetic, economic, and environmental perspectives. The static fertilizer advice in wheat on the basis of the average response has led to both excessive and insufficient fertilizer application with greater N losses [13]. The 4Rs (right method, right time, right source, right rate) of nutrient stewardship are employed by decision support systems such as Nutrient Expert [14] and may be employed precisely for balanced fertilization in the eastern sub-Himalayan plains of India. In this location, wheat nutrient management under both conventional and zero tillage systems has already demonstrated the benefits of using such easy-to-use nutrient decision support tools [15,16]. In addition to the fertilizer recommendation through decision support systems, using biofertilizers such as phosphate-solubilizing bacteria and Azotobacter may further improve plant nutrient availability because of the rapid growth of bacteria [17].
Keeping these factors in mind, the present research was conducted in ZT wheat, which was grown after transplanted rice had puddled, using various loads of rice residue and nutrient management strategies. The main goal of this study was to evaluate how different residue loads and nutrient management strategies affect the overall growth and yield performance of wheat crops. This study also investigated how these factors might affect the energetics, CO2 emissions, and production economics of the ZT-wheat production system in the eastern subHimalayan plains.
The research study was carried out during two consecutive rabi (winter) seasons, 2021–2022 and 2022–2023, at the Research Field, Uttar Banga Krishi Viswavidyalaya, Coochbehar, West Bengal, India, which is located at 26°24′02.2″ N latitude and 89°23′21.7″ E longitude. The soil of the experimental field had a sandy loam texture, with a soil pH of 5.53. The fertilizer conditions of the experimental soil before the start of the first year of wheat cultivation were estimated as follows: an organic carbon content of 0.71%, an available nitrogen content of 134.58 kg ha−1, an available phosphorous content of 39.90 kg ha−1 and an available potassium content of 129.46 kg ha−1.
The average annual precipitation of the region is 3000 mm, 70%–90% (2100–2700 mm) of which is received from June to September (southwest monsoon). The winter months tend to remain dry, irrespective of occasional and minimal rainfall (Fig. 1).
Figure 1: Meteorological data prevailing during the experimental years (a) 2021–2022 and (b) 2022–2023; RH-relative humidity
The temperature began to decrease in November, whereas the lowest temperature occurred in January. Thereafter, the temperature showed an increasing trend from mid-February and reached its peak from April–May. Even in the winter, the relative humidity remained relatively high during the crop growing period. The prevailing weather conditions throughout the growing phases during both seasons reflected a favourable climate for wheat (Fig. 1).
A split-plot design comprising 4 different rice residue load treatments (main plot) and 5 different nutrient management strategies (subplot) was employed to establish the experiment. There were twenty (20) treatment combinations, each with three replicates. The specifics of the treatments are outlined in detail in Table 1.
The previous rice crop was harvested manually at the optimum heights (0, 15, 30 and 45 cm). After the rice crop was harvested at different heights, the residue load was determined on a dry weight basis. As the crop stand varied during both years, the residue load also varied considerably even under the same treatment. The rice variety used in this study was MTU 1153, a short maturing variety (115–120 days) with a potential yield of 6.5 t ha−1. Table 2 illustrates the residual load attained during the two experimental years. The preceding rice produced aerial biomasses of 10.7 and 13.6 t ha−1 from 2021–2022 and 2022–2023, respectively. Owing to the variation in biomass production in the two different experimental years, the residue load also varied widely over the two years. The herbicide glyphosate 41% SL was applied at 3.5 lit ha−1 one week prior to wheat sowing to suppress the preexisting weed flora in the experimental plot. To control broad-leaf weeds at later stages, the ready-mix postemergence herbicide metribuzin 42% + clodinofop propagyl 12% at 0.75 kg ha−1 was applied at 4 weeks after sowing. One day prior to seeding, the seeds were treated with biofertilizers (Azotobacter and PSB) as per the treatments. Considering the variable amount of retained rice residue load, the sowing operation was performed with the help of a happy seeder with seed rates of 135 and 120 kg ha−1 from 2021–2022 and 2022–2023, respectively, in 20 cm rows. The application of fertilizers was carried out in accordance with the treatments. The wheat variety used for this experiment was DBW 187, developed by the Indian Council of Agricultural Research (ICAR)-Indian Institute of Wheat & Barley Research (IIWBR), Karnal, Haryana. This variety is suitable for timely sowing under irrigated conditions and matures in 115–120 days, with a potential yield of 6.4 t ha−1. Given micronutrient deficiency, particularly B and Zn deficiencies, in this region, B was foliar applied twice in the form of Solubor (20% B) at 0.20% at 35 and 55 DAS. A foliar spray of 0.10% zinc-EDTA (Chelamin) was applied at 55 DAS. During both years, the crop received 3 irrigations at crown root initiation (CRI), active tillering and milking stages through the check basin method at a depth of 5 cm.
The soil bulk density was determined by using soil samples collected at depths of 0–15 cm via a core sampler with a known volume, as suggested by Blake et al. [18]. A Sorensen pH meter (1909) with a 2.5:1 ratio of water:soil was used to measure the soil pH via a potentiometric method. The wet digestion method was used to determine the organic carbon content in the soil samples [19]. The amount of mineralizable nitrogen was determined via the hot alkaline potassium permanganate method [20]. For available phosphorus (P), the soil was extracted with 0.5 M NaHCO3, pH 8.5 [21], and the P content was determined via a UV–VIS spectrophotometer. Using 1 N neutral normal ammonium acetate, the available potassium (K) in the soil was estimated, and a flame photometer was then used to estimate the final K content in the soil [22].
2.6 Measurements of Growth, Yield and Phenological Traits
The tiller number was recorded periodically at 15-day intervals from one running meter over five marked places in each plot and converted into square meters accordingly. The leaf surface area (cm2) was measured, and through an area–weight relationship, the leaf area index (LAI) was recorded periodically as the ratio of the total green leaf surface area (cm2) to the total ground area of the plant (cm2), as suggested by Watson [23], at 15-day intervals. For estimation of grain yield, a 34.4 m2 (18 m long and 1.8 m wide) net plot area was selected, excluding border rows. After the plants were threshed, the grains were sun dried and weighed separately, and the obtained value was converted to t ha−1. The total biological yield of wheat was calculated by summing the grain yield and straw yield of the corresponding treatments. Phenological parameters such as days to reach maximum tillering, 80% booting, heading, flowering and days to reach physiological maturity were recorded on the basis of visual observations.
2.7 Studies on Crop Energetics
Both direct (labour) and indirect (chemical fertilizers, biofertilizers, seeds, herbicides, herbicides) forms of energy were considered for calculating energy inputs. The energy input was then computed via the energy equivalent for each input. The output energy was estimated by multiplying the amount of produce (grain and straw yield) with the corresponding energy equivalent. The energy equivalents were taken into consideration by employing primary data [24–26]. The conversion units for the energy inputs and outputs are listed in Table 3.
Various energy indices (net energy, energy use efficiency, energy productivity and specific energy) were calculated via the following formulae [27].
Net Energy (MJ ha−1) = Energy output (MJ ha−1)− Energy input (MJ ha−1)
Energy Use Efficiency = Energy output/Energy input
Energy Productivity = Grain yield (kg ha−1)/Energy input (MJ ha−1)
Specific Energy (MJ kg−1) = Energy input (MJ ha−1)/Total energy output (grain + straw) (kg ha−1)
2.8 Studies on CO2 Emission (kg CO2 –e kg−1)
The CO2 emission was computed by multiplying various inputs (seed, labour, fuel, herbicide and fertilizers) with their respective conversion coefficients, as given in Table 3.
To determine the most profitable treatment, various economic analyses, including cultivation cost (₹ ha−1), gross return (₹ ha−1), net return (₹ ha−1) and the benefit–cost ratio (B:C), were computed for wheat under varying rice residue loads and different nutrient management options during both years. The cost of cultivation for wheat was estimated by taking into consideration the prevailing costs in local markets for various inputs, viz., fertilizers, seeds, labour, herbicides, biofertilizers and other expenses associated with crop growth. To calculate gross and net income, the minimum support price (MSP) of wheat for each year was considered.
Statistical evaluation of the recorded data for the split plot design (SPD) was performed via analysis of variance (ANOVA) for each year separately. Using MS Excel, the significance of different residues and nutrient and interaction effects was evaluated at the 5% probability level utilizing the mean square error (MSe). To compare F tables and calculate critical differences (CDs), the Fisher and Yates table was used [37]. In addition, the mean values were adjusted via Duncan’s multiple range test (DMRT) via SPSS version 20.0.3.
3.1 Tillers, LAIs, Yields and Phenologies under Varying Rice Residue Loads and Nutrient Management Options
When determining the effectiveness of a treatment, the quantity of tillers per unit area is a major consideration. The average number of tillers per square meter tended to increase linearly up to 60 DAS; after that, intraplant competition, a lack of resources, and self-thinning, which resulted in tiller mortality, caused the number of tillers to drastically decrease (Fig. 2a,b). Owing to variations in the amount of rice residue applied and fertilizer management strategies, the number of wheat tillers per m−2 changed during the course of the two-year trial. In general, the treatments in which residues were retained at an optimal level (R1 and R2) yielded more tillers per m−2 in comparison with treatments without residue retention (R0) and treatments with a relatively high residue load (R3) at different growth stages.
Figure 2: Tiller number of wheat at different growth stages as influenced by (a) various rice residue load treatments and (b) different nutrient management options. Note: R0: Harvested preceding rice crop to ground level; R1: Harvested preceding rice crop at 15 cm above ground level; R2: Harvested preceding rice crop at 30 cm above ground level; R3: Harvested preceding rice crop at 45 cm above ground level. N1: Control (no fertilizer application); N2: Recommended dose of fertilizer (RDF) (150, 60, 40 kg ha−1 of N, P2O5 and K2O, respectively); N3: Nutrient Expert (NE)-based fertilizer recommendation (155, 76, 85 and 144, 72, 72 kg ha−1 of N, P2O5 and K2O in year 1 and year 2, respectively); N4: RDF + Seed treated with biofertilizer (Azotobactor and Phosphate Solubilizing Bacteria) (RDF + Bio); N5: NE + Seed treated with biofertilizer (Azotobactor and Phosphate Solubilizing Bacteria) (NE + Bio)
Amongst different nutrient management options, treatment N3 produced noticeably more tillers (317, 359, 348, and 330 m−2 at 45, 60, 75, and 90 DAS, respectively) than the other nutrient management options during the first year (2021–2022). However, in the second year (2022–2023), excepting at 45 DAS, treatment N5 produced more tillers (336, 322, and 300 m–2 at 60,75 and 90 DAS, respectively). The control plot (N1) for both years presented the lowest number of tillers over the whole growth period. The mean separation test-based variation in tiller number in relation to residue load and nutrient management options is also presented in Tables S1 and S2 for better understanding.
The physiological measure known as the leaf area index (LAI) has a major impact on agricultural output because it affects crop canopy light absorption. The photosynthetic surface of a crop is represented by the leaf area index. Up to 75 DAS, the leaf area index increased linearly. After that, the index began to decrease at 90 DAS, irrespective of the treatment (Fig. 3a,b).
Figure 3: Leaf area indices of wheat at different growth stages as influenced by (a) various rice residue load treatments and (b) different nutrient management options. Note: R0: Harvested preceding rice crop to ground level; R1: Harvested preceding rice crop at 15 cm above ground level; R2: Harvested preceding rice crop at 30 cm above ground level; R3: Harvested preceding rice crop at 45 cm above ground level. N1: Control (no fertilizer application); N2: Recommended dose of fertilizer (RDF) (150, 60, 40 kg ha−1 of N, P2O5 and K2O, respectively); N3: Nutrient Expert (NE)-based fertilizer recommendation (155, 76, 85 and 144, 72, 72 kg ha−1 of N, P2O5 and K2O in year 1 and year 2, respectively); N4: RDF + Seed treated with biofertilizer (Azotobactor and Phosphate Solubilizing Bacteria) (RDF + Bio); N5: NE + Seed treated with biofertilizer (Azotobactor and Phosphate Solubilizing Bacteria) (NE + Bio)
The LAI is affected by nutrient management options as well as the amount of rice residue retained on land. During the early phases, residues kept at a height of 30 cm above ground level (R2) produced a noticeably greater leaf area index (1.12 and 0.93 during 2021–2022 and 2022–2023, respectively) (Fig. 3a,b). The LAI values were similar to various residue loads during the overall crop growth period. However, the NE-based approach plus biofertilizer seed inoculation (N5) outperformed the other nutrient management options proportionately and resulted in higher LAIs (1.01 and 0.94, 1.99 and 1.54, 4.36 and 4.25, 2.98 and 2.63 at 30, 45, 75, and 90 DAS, respectively, during 2021–2022 and 2022–2023) (Fig. 3a,b). Throughout both years, the control treatment (N1) had the lowest leaf area index. Only in the early stages (30 DAS) of the second year there was a significant relationship between rice residue and nutritional methods. The interaction effect between residue maintenance and fertilizer application on the basis of the NE combined with biofertilizer seed inoculation resulted in a greater leaf surface area; thereafter, the LAI remained unaffected by the interaction effect. The mean separation test-based variation in the LAI in relation to residue load and nutrient management options is also presented in Tables S3 and S4 for a better understanding.
In the second year (2022–2023), rice residue management strategies had a considerable effect on wheat grain yield (Table 4). Significantly higher grain yields (4.48 and 4.16 t ha−1 during 2021–2022 and 2022–2023, respectively) were obtained with residue loads of approximately 2.5–3.5 t ha−1 (R2) than those of the other residue load treatments (R0, R1, and R2) during both experimental years. Except for the N4 treatment, where the grain yields (5.12 and 4.45 t ha−1) produced were statistically similar to those of the N5 treatment, the grain yield under the N5 treatment (5.27 and 4.64 t ha−1) exceeded those under the other nutrient management options.
During the two research trial years, the N2 and N3 treatments also resulted in good yields. The biological yield in the first year (2021–2022) was not impacted by the load of rice residue. However, in the 2021–2022 and 2022–2023 seasons, greater biological yields (10.72 and 9.51 t ha–1, respectively) were attained in the treatment where rice residues were kept at 30 cm above the ground. In the first year of testing, the biological yield of the R0 treatment was the lowest (9.75 t ha−1). On the other hand, from 2022–2023, the treatment in which residues were kept at a height of 45 cm (R3) resulted in the lowest biological yield (8.83 t ha−1). Overall, over the two years of testing, the biological yield of wheat was impacted by various nutrient management strategies. Compared with the other nutrient management techniques, the N5 treatment produced noticeably more biomass (12.27 and 10.57 t ha−1 from 2021–2022 and 2022–2023, respectively). The control treatment (N1) yielded the lowest biological yield (4.58 and 4.73 t ha−1 from 2021–2022 and 2022–2023, respectively) during the two experimental years. The total biomass obtained via the N2, N3, and N4 nutrient management systems was statistically equivalent to that obtained via the other methods (Table 4).
During both years, the phenological stages (maximal tillering, 80% booting, 80% heading, 80% flowering, and physiological maturity) associated with different rice residue loads and nutrition management choices were recorded (Table 5). During the years 2021–2022 and 2022–2023, the variety employed in this experiment (DBW 187) took an average of 56–68, 70–79, 76–87, 84–97, and 114–127 days to reach maximum tillering, 80% booting, 80% heading, 80% flowering, and physiological maturity, respectively. The phenological characteristics of wheat are somewhat impacted by rice residue management. Each phenological stage was reached earlier in the treatments where residues were entirely eliminated above the ground (R0). However, the phenological dates were similar under the R1 and R2 treatments, where residues were held at 15 and 30 cm above ground level; in contrast, the crop took longer to reach different growth stages under the R1 and R2 treatments, where residues were retained at greater heights, i.e., 45 cm from ground level. When considering the various possibilities for managing nutrients, the N2, N3, N4 and N5 treatments demonstrated relatively consistent attainment of each phenological phase at dates that were comparable, with little variation. On the other hand, in both years, the N1 treatment and the control presented considerably earlier growth stages than did the alternative nutrient management strategies (Table 5).
3.2 Energetics and CO2 Emissions as Influenced by Rice Residue Loads and Nutrient Management Options
The energy input was computed and is shown in Table 6, which is based on the sources of inputs used during the two experimental years. When various inputs were considered for various operations, fertilizers (50.74% and 42.53%) made the greatest contribution, followed by fuels (19.07% and 26.36%) and seeds (16.55% and 12.20%). In comparison, the labor component’s energy use was much lower. The greatest contribution to energy inputs under the different nutrient management approaches was NE-based fertilizer management. Under different rice residue loads and nutrient management strategies, the energy production of wheat (grain and straw yield) followed a similar trend as the wheat grain yield in both years. The grain and straw yields varied greatly due to different rice residue loads and nutrient management methods, reflecting significant variations in energy output.
The R2N5 treatment combination, where residues were maintained at a height of 30 cm and nutrient application was based on NE software, including biofertilizer seed inoculation, produced the highest total energy outputs (208,986 and 183,987 MJ ha−1 from 2021–2022 and 2022–2023, respectively) among the various treatment combinations. The greatest net energy gain was obtained with the same nutrient management technique. The energy use efficiency from 2021–2022 and 2022–2023 varied from 10.00–13.33 and from 8.30–12.17, respectively. The energy usage efficiency attained under the N1 treatment (no external fertilizer application) was somewhat greater than that of the other treatments, regardless of the rice residue loading treatment (Table 3a,b). The treatment combinations R2N4 (residues retained at 30 cm in height and nutrients applied on the basis of the recommended dose of fertilizers along with inoculation of biofertilizers) and R2N5 (residues retained at 30 cm in height and nutrient application guided by NE software along with inoculation of biofertilizer) presented the highest energy productivity (0.304 kg MJ−1). Throughout both years, the specific energy changed in response to different rice residue loads and nutrient management strategies. The reduced specific energy was reflected in these two treatment combinations.
Fig. 4 shows the percentage contributions of the various inputs to CO2 emissions. From 2021–2022 and 2022–2023, CO2 emissions varied according to different rice residue and nutrient management methods, ranging from 343.37 to 1266.20 and 428.43 to 1278.76 kg CO2–eq ha−1, respectively. With respect to the rice residue treatments, there was no difference in CO2 emissions among the different treatments. Compared with the other nutrient management methods, the NE-based fertilizer treatments (N3 and N5) led to increased CO2 emissions in both years.
Figure 4: Percent contributions of various input sources to CO2 emissions from (a) 2021–2022 and (b) 2022–2023
3.3 Production Economics as Influenced by Rice Residue Loads and Nutrient Management Options
The different rice residue load treatments did not significantly affect the overall cost of farming. Among all the nutrient management alternatives, the treatments that included seed inoculation with biofertilizers and nutrient application guided by the NE software tool (N5) resulted in a relatively high cultivation cost. During the two test years, the control treatment, which involved no fertilizer application (N1), had the lowest possible cultivation costs. Different loads of rice residue and nutrient management practices during both years had substantial impacts on the gross and net returns achieved with crop cultivation (Fig. 5).
Figure 5: Production economics of wheat as influenced by various rice residue loads and nutrient management options
Under the R2N5 treatment, where residues were retained at a height of 30 cm and nutrient management was based on NE recommendations, including biofertilizers, significant maximum gross returns (₹111,315 ha−1) and ₹109,342 ha−1 from 2021–2022 and 2022–2023, respectively) and net returns (₹60,957 ha−1 and ₹61,797 ha−1 from 2021–2022 and 2022–2023, respectively) were obtained. The treatments with no residue retention and no fertilizer application throughout both years presented the lowest gross and net returns because of the large fluctuations in production. Similarly, the treatments with relatively high net and gross returns also markedly registered the highest B:C ratios. The treatment combinations of R2N4 (residues retained at 30 cm height with nutrient management through RDF + biofertilizers) and R2N5 (rice residues retained at 30 cm height and nutrient management was based on NE + biofertilizers) produced the highest B:C ratio (2.21) during the first year, which was statistically comparable to R1N4 (2.20) and R1N5 (2.18). Under the R2N5 condition (rice residues retained at a height of 30 cm and nutrient application based on NE + biofertilizers), the highest B:C ratio (2.30) was noted during the second year (Fig. 5).
4.1 Impact of Rice Residue Loads on the Growth Parameters and Yields of Wheat
The findings clearly revealed that for both years, there was little change in the number of tillers, with variation in the retention of residues at different heights. The tiller numbers were positively impacted by treatments with optimal residue retention and negatively impacted by treatments with no residue and greater loads of rice residue. Owing to improved soil moisture content near the root zone and nutrient availability through the residues upon decomposition, treatments R1 and R2 performed better than the other levels of residue retention did, according to the data. A greater number of tillers in wheat under ideal residue retention was previously reported [38–40]. The detrimental effects of increasing residue load on the number of wheat tillers were noted by Sirazuddin et al. [41]. The findings indicated that removing residues in excess was detrimental to the crop and that maintaining 2.5 to 3.5 t ha−1 residues remained the most beneficial to the wheat crop. Since the grain yield was much lower with both approaches, maintaining the optimal residue load was more advantageous than maintaining excessive (R3) or no residue (R0). The overabundance of residues complicates sowing procedures, which in turn results in incorrect crop stands and decreased crop productivity [41,42]. Optimal residue load also allowed the crop for efficient utilization of solar radiation reflected by an increased leaf area index under the appropriate residue loading treatment. The optimum residue-retained plots reflected a better crop growth and yield performances due to a number of positive effects such as soil temperature regulation, better nutrient and moisture availability vis-à-vis greater root proliferation [41]. The biological yield produced with the R2 treatment was superior to that of the other alternative residue management procedures, as demonstrated by the results of the present study. When the R2 treatment was compared with the R0, R1, and R3 treatments, the percentage increases in biomass yield were 9.95%, 3.08%, and 2.98% (2021–2022) and 7.34%, 6.49%, and 7.70% (2022–2023), respectively. The improved grain and straw yields under ideal residue retention may be the cause of increased biological productivity under the aforementioned treatment (R2).
4.2 Impact of Nutrient Management Options on the Growth Parameters and Yields of Wheat
Various nutrient management strategies significantly affect the number of wheat tillers throughout the entire crop growth cycle. The tiller number m−2 under NE-based fertilizer led to the generation of more tillers and attained higher LAI values, according to the results. The maximum number of tillers m−2 was observed when the microbial inoculants (Azotobacter and PSB) were seeded in conjunction with the application of nutrients as directed by Nutrient Expert. The maximum number of tillers under the aforementioned treatments may have resulted from providing the crop with the right amount of nutrients, with a focus on higher rates of potassium and phosphorus delivery. The number of tillers per square meter was also increased by the combined application of inorganic fertilizer and biofertilizer for seed inoculation, which also improved nutrient availability, healthier main culm, and overall crop stand establishment [43]. The combination of conventional and biofertilizers may have helped to improving nutrient absorption, nitrogen availability, and photosynthetic acid accumulation, all of which improved crop growth and increased leaf area index values under the appropriate treatments. These results are consistent with the findings of Mohanty et al. [44] and Kumar et al. [45], who reported that NE-based fertilizer recommendations substantially increased the tiller number and LAI. The results revealed that applying nutrients in accordance with the advice of nutrient experts in addition to biofertilizer seed inoculation increased the wheat grain yield (N5). The ability to better address the nutritional needs of the crop and improved photosynthate transfer to the sink may be the cause of increased grain yields under the previously described treatments. The greater wheat production was the outcome of balanced nutrient management with more phosphorus and potassium application carried out under the guidance of NE [15,16]. Furthermore, our findings demonstrated that biofertilizers, particularly PSB, contributed to the increase in wheat grain productivity. This was likely due to the improved activity of various beneficial microbes that solubilize insoluble phosphorous by producing various organic acids [46]. When the NE-based approach was used instead of the recommended fertilizer doses, the biological yield was considerably greater [45,47].
The phenological development of a crop is the most important biological indicator of changes in the climate and the consequences of rising temperatures. Since the crop was sown during the optimal sowing time for both the study years, the phenological stages in our experiment were quite comparable, notwithstanding the possibility of variations depending on meteorological circumstances. The crop required approximately 10 to 15 days to reach booting from the active tillering phase, 6 to 8 days to reach heading from the booting phase, 5 to 7 days to reach flowering from the heading phase, and 15 to 20 days to reach the physiological maturity stage, according to the study’s results. However, complete residue removal plots (R0) and plots without any fertilizer application (N1) reached each growth stage 7 to 10 days earlier. This could be due to nutritional pressure under residue removal and without nutrient application, which allows the crop to reduce its vegetative and reproductive phases to acquire each phenological phase more quickly and finish its life cycle. Furthermore, the crop reached phenological phases slightly later under greater loads of residue (R3), which may have been caused by increased residual moisture, which improved crop succulence and hence prolonged the vegetative phase. An additional explanation for the delayed phenological attainment observed with larger residue treatments would be the longer time it took for the seeds to surface, which resulted in a delayed start to each phenological phase. Previous studies [15,38,48] have indicated that while nutrient management strategies have a considerable effect on wheat phenological phases, residue management does not influence wheat phenology.
4.3 Crop Energetics and CO2 Emissions under Varying Rice Residue Loads and Nutrient Management Options
The fertilizers accounted for approximately 50.74% and 42.53% of the input energy during first and second year of experimentation, respectively. Among the three key nutritional elements, nitrogen was the major contributor, and phosphorous and potassium made up the next two largest proportions. As the fertilizer application was carried out at higher rates in accordance with the NE’s guidance in first year, the first-year trial’s fertilizer energy consumption was greater than that of the second-year trials. Fuel was the second largest input provider contributing 19.07% and 26.36% during first and second year, respectively. Using diesel oil for various tractor-drawn agricultural tasks has become the primary source of energy from fuel-based inputs. Our research clearly revealed that there was an increase in fuel energy use in the second year when the crop was harvested via a combine harvester. The seeds contributed approximately 16.55% and 12.20% of input energy; while the energy inputs of human labor for 2021–2022 and 2022–2023 were approximately 8.55% and 3.85%, respectively. A higher seeding rate and more labor requirement during the first year of harvest (since it was harvested by hand) contributed higher share in input energy. From 2021–2022 and 2022–2023, herbicides, biofertilizers, and micronutrients made up approximately 3.87% and 3.21%, 1.21% and 1.00% and 0.02% and 0.02%, respectively. Previous studies [49,50] have also pointed to fuel, fertilizers, and seeds as the main sources of energy input in wheat. When several fertilizer approaches were combined, the N5 treatment led to higher energy consumption in the first year because of a higher rate of potassium and phosphorus delivery through use of NE. Higher input energy with greater application of nutrient elements were reported previously [51,52].
Our research findings revealed that the output energy recorded in the first year was greater than the output energy attained in the second year. This could be due to attainment of higher yields in the first year and crop productivity and energy output are directly correlated [53–55]. The residue retention plots revealed better yields, which eventually led to the maximum total output energy [56]. The increased productivity of grains and straw may result in increased energy production with higher net energy gains. Compared with alternative nutrient management strategies, the greater energy use efficiency of the control (N1) treatment is related to its minimal input energy use; however, the proposal is meaningless due to attainment of very poor grain output. The R1N4 treatment combination was the most energy-efficient treatment when considering the other alternatives for managing residues and nutrients. Using less phosphorous and potassium fertilizers and generating significantly higher yields resulted in greater energy use efficiency for the stated treatment combination. According to previous findings [53,57], crop productivity and the fertilizer usage rate are directly related to energy use efficiency. Energy productivity indicates a lower energy consumption per unit of grain production. The results of our two-year study revealed that, with the R2N4 treatment combination, we were able to achieve better grain production with reduced energy input during the first year. Again, R2N5 treatment combination was registered as an efficient energy productivity therapy in the second year for the same reason. According to previous findings [2,58], residue retention under CA-based techniques may increase the output energy by lowering the input energy, which ultimately results in increased energy productivity and lower specific energy. Research [59] has also shown that, in a rice–wheat cropping system, site-specific nutrient management techniques led to increased energy production with decreased specific energy.
Data on CO2 emissions revealed that, compared with that in the first year (2021–2022), the CO2 equivalent during the second year of the experiment (2022–2023) was somewhat greater. This was mostly because greater rates of fuel energy usage during the second year. Among all the nutritional management options available, N3 and N5 presented the highest CO2 equivalent values from 2021–2022. The greater CO2 equivalent in both years may have been caused by higher rates of fertilizer application (phosphorus and potassium in particular) under the aforementioned treatments. When residues were retained and fertilizer application rates were relatively high, CO2 emissions were noticeably greater than when there was no residue and lower fertilizer application rates [35,60]. The maximum overall carbon input under the different nutrient management options came from fertilizers (N, P, and K), which contributed approximately 57% of the CO2 emissions. Fuel (18% and 26%), herbicides (11% and 10%), seeds (10% and 8%), and labour (4% and 3%) followed (Fig. 4b). Increasing rates of fuel and fertilizer application are supposed to be the two main causes of CO2 emissions [61].
4.4 Production Economics of Wheat under Varying Rice Residue Loads and Nutrient Management Options
Compared with residue removal and no fertilizer application, production economics data revealed that residue retention combined with balanced nutrient management, including biofertilizers, was economically advantageous. The main reason for the lower cultivation costs in the second year was crop harvest through the combine harvester, and fertilizers, especially phosphorous and potassium, were applied at lower rates. In the first year, on the other hand, more man-days were required for manual harvesting, and higher rates of phosphorous and potassium application as suggested by NE were used. The higher application rates of phosphorous and potassic fertilizers were the main cause of the increased cultivation costs experienced under NE-based advice [15,16].
The options available for managing nutrient load and rice residue load had considerable impacts on the profitability of the crops. Compared with the other treatment combinations, the R2N5 treatment produced significantly greater gross and net returns over both the years. This was achieved by maintaining residues at a height of 30 cm and managing nutrients via guidelines from the NE, which included the use of biofertilizers. The wide variation in returns and benefits among the different treatment combinations may be attributed to the beneficial effects of residue retention and balanced nutrient management using NE, including the use of biofertilizers as seed inoculants, leading to improved growth, yield attributes, and yield. Under residue retention and balanced nutrient management, higher returns and profits were observed [53,62,63]. Higher profitability with the NE-based fertilizer recommendation under the eastern sub-Himalayan plains of India was also reported in previous studies [15,16].
Based on two-year field trials results, it was determined that crop growth, productivity, and profitability were better when the residue load was between 2.5 and 3.5 t ha−1, and the NE-based suggestions were combined with Azotobacter and PSB seed inoculation. Under NE-based recommendations, lower specific energy, higher net energy, and energy use efficiency are noted. Our results also revealed that higher rates of fertilizer application were associated with increased CO2 emissions. These findings unequivocally demonstrated that the region’s zero-tillage wheat crop growth, productivity, and profitability were negatively impacted by removal as well as excessive residue burden.
Acknowledgement: The authors extend their appreciation to Taif University, Saudi Arabia, for supporting this work through project number TU-DSPP-2024-07.
Funding Statement: This study was funded by the Department of Agronomy, Uttar Banga Krishi Viswavidyalaya, West Bengal 736 165, India. This research was also funded by Taif University, Saudi Arabia, Project No. (TU-DSPP-2024-07).
Author Contributions: Conceptualization: Biplab Mitra; methodology: Biplab Mitra, Mallepu S Likhitha Reddy; software: Akbar Hossain, Ahmed Gaber; validation, Biplab Mitra, Mallepu S Likhitha Reddy; formal analysis: Biplab Mitra, Mallepu S Likhitha Reddy, Akbar Hossain, Ahmed Gaber; investigation and resources: Biplab Mitra, Mallepu S Likhitha Reddy, Akbar Hossain Ahmed Gaber; data curation: Akbar Hossain, Ahmed Gaber; writing—original draft preparation: Biplab Mitra, Mallepu S Likhitha Reddy; writing—review and editing: Biplab Mitra, Mallepu S Likhitha Reddy, Akbar Hossain, Ahmed Gaber; supervision: Biplab Mitra, Akbar Hossain; project administration: Biplab Mitra, Mallepu S Likhitha Reddy, Akbar Hossain, Ahmed Gaber; funding acquisition: Ahmed Gaber, Akbar Hossain. All authors reviewed the results and approved the final version of the manuscript.
Availability of Data and Materials: The data may be available upon request to the corresponding author.
Ethics Approval: Not applicable.
Conflicts of Interest: The authors declare no conflicts of interest to report regarding the present study.
Supplementary Materials: The supplementary material is available online at https://doi.org/10.32604/phyton.2024.056789.
References
1. Jat ML, Gathala MK, Saharawat YS, Ladha JK, Singh Y. Conservation agriculture in intensive rice–wheat rotation of western indo-gangetic plains: effect on crop physiology, yield, water productivity and economic profitability. J Environ Sci Nat Resour. 2019a;18(3):88–102. doi:10.19080/IJESNR.2019.18.555988. [Google Scholar] [CrossRef]
2. Chaudhary VP, Singh KK, Pratibha G, Bhattacharyya R, Shamim M, Srinivas I, et al. Energy conservation and greenhouse gas mitigation under different production systems in rice cultivation. Energy. 2017;130(4):307–17. doi:10.1016/j.energy.2017.04.131. [Google Scholar] [CrossRef]
3. Ghosh S, Das TK, Rana KS, Biswas DR, Das DK, Singh G, et al. Energy budgeting and carbon footprint of contrasting tillage and residue management scenarios in rice-wheat cropping system. Soil Tillage Res. 2022;223(2):105445. doi:10.1016/j.still.2022.105445. [Google Scholar] [CrossRef]
4. Sapkota TB, Vetter SH, Jat ML, Sirohi S, Shirsath PB, Singh R, et al. Cost-effective opportunities for climate change mitigation in Indian agriculture. Sci Total Environ. 2019;655:1342–54. doi:10.1016/j.scitotenv.2018.11.225. [Google Scholar] [PubMed] [CrossRef]
5. Talukder B, Gary W, Hipel KW. Energy efficiency of agricultural systems in the southwest coastal zone of Bangladesh. Ecol Indic. 2019;98(2):641–8. doi:10.1016/j.ecolind.2018.11.030. [Google Scholar] [CrossRef]
6. Das TK, Saharawat YS, Bhattacharyya R, Sudhishri S, Bandyopadhyay KK, Sharma AR, et al. Conservation agriculture effects on crop and water productivity, profitability and soil organic carbon accumulation under a maize-wheat cropping system in the north-western Indo-Gangetic Plains. Field Crops Res. 2018;215(1):222–31. doi:10.1016/j.fcr.2017.10.021. [Google Scholar] [CrossRef]
7. Jat RK, Singh RG, Kumar M, Jat ML, Parihar CM, Bijarniya D, et al. Ten years of conservation agriculture in a rice-maize rotation of eastern Gangetic plains of India: yield trends, water productivity and economic profitability. Field Crops Res. 2019b;232:1–10. doi:10.1016/j.fcr.2018.12.004. [Google Scholar] [CrossRef]
8. Gupta DK, Bhatia A, Kumar A, Das TK, Jain N, Tomer R, et al. Mitigation of greenhouse gas emission from rice-wheat system of the Indo-Gangetic plains: through tillage, irrigation and fertilizer management. Agric Ecosyst Environ. 2016;230(5):1–9. doi:10.1016/j.agee.2016.05.023. [Google Scholar] [CrossRef]
9. Mondal DD, Ghosh A, Sen S, Roy D, Bandopadhyay P, Ghosh R, et al. Effect of herbicides and their combinations on weeds and productivity of direct-seeded rice (Oryza sativa). Indian J Agron. 2019;64(4):464–70. doi:10.59797/ija.v64i4.5313. [Google Scholar] [CrossRef]
10. Biswakarma N, Pooniya V, Zhiipao RR, Kumar D, Shivay YS, Meena MC, et al. Designing resource efficient integrated crop management modules for direct seeded rice-zero till wheat rotation of north western India: impacts on system productivity, energy-nutrient-carbon dynamics. Arch Agron Soil Sci. 2022:1–15. doi:10.1080/03650340.2022.2079635. [Google Scholar] [CrossRef]
11. FAO. Conservation agriculture; 2019. Available from: http://www.fao.org/conservation-agriculture/overview/what-is-conservationagriculture/en/. [Accessed 2024]. [Google Scholar]
12. Das TK, Nath CP, Das S, Biswas S, Bhattacharyya R, Sudhishri S, et al. Conservation agriculture in rice– Indian mustard cropping system for five years: impacts on crop productivity, profitability, water-use efficiency, and soil properties. Field Crops Res. 2020;250(1):107781. doi:10.1016/j.fcr.2020.107781. [Google Scholar] [CrossRef]
13. Thierfelder C, Baudron F, Setimela P, Nyagumbo I, Mupangwa W. Complementary practices supporting conservation agriculture in southern Africa. A review. Agron Sustain Dev. 2018;38:16–24. doi:10.1007/s13593-018-0492-8. [Google Scholar] [CrossRef]
14. Pampolino M, Majumdar K, Jat ML, Satyanarayana T, Kumar A, Shahi VB, et al. Development and evaluation of nutrient expert for wheat in South Asia. Better Crops. 2012;96(3):29–31. [Google Scholar]
15. Mondal T, Mitra B, Das S. Precision nutrient management in wheat (Triticum aestivum) using Nutrient Expert®: growth phenology, yield, nitrogen-use efficiency and profitability under eastern sub-Himalayan plains. Indian J Agron. 2018;63(2):174–80. [Google Scholar]
16. Mitra B, Majumdar K, Dutta SKR, Mondal T, Das S, Banerjee H, et al. Nutrient management in wheat (Triticum aestivum) production system under conventional and zero tillage in eastern sub-Himalayan plains of India. Indian J Agric Sci. 2019;89(5):775–84. doi:10.56093/ijas.v89i5.89657. [Google Scholar] [CrossRef]
17. Yasin M. Review article: biofertilizers, substitution of synthetic fertilizers in cereals for leveraging agriculture. Crop Environ. 2012;3:62–6. [Google Scholar]
18. Blake GR. Methods of soil analysis: part 1 physical and mineralogical properties, including statistics of measurement and sampling. American Society of Agronomy, Inc.; 1965. p. 374–90. doi:10.2134/agronmonogr9.1 [Google Scholar] [CrossRef]
19. Walkley AJ, Black CA. Estimation of soil organic carbon by the chromic acid titration method. Soil Sci. 1934;37(1):29–38. doi:10.1097/00010694-193401000-00003. [Google Scholar] [CrossRef]
20. Subbaiah BV, Asija GL. A rapid procedure for estimation of available nitrogen in soil. Curr Sci. 1956;25:259–60. [Google Scholar]
21. Olsen SR, Cole CV, Watanabe FS, Dean LA. Estimation of available phosphorus in soils by extraction with NaHCO3. USDA Circular. 1954;939:1–19. [Google Scholar]
22. Warncke D, Brown JR. Potassium and other basic cations. Recommended chemical soil tests procedures for the North Central Region. North Central Regi Res Pub. 1988;221:31–3. [Google Scholar]
23. Watson DJ. Comparative physiological studies on the growth of field crops: I. Variation in net assimilation rate and leaf area between species and varieties, and within and between years. Ann Bot. 1947;11(41):41–76. [Google Scholar]
24. Lal R. Carbon emission from farm operations. Environ Int. 2004;30(7):981–90. doi:10.1016/j.envint.2004.03.005. [Google Scholar] [PubMed] [CrossRef]
25. Wang W, Lai DYF, Wang C, Pan T, Zeng C. Effects of rice straw incorporation on active soil organic carbon pools in a subtropical paddy field. Soil Till Res. 2015;152:8–16. doi:10.1016/j.still.2015.03.011. [Google Scholar] [CrossRef]
26. Parihar CM, Yadav MR, Jat SL, Singh AK, Kumar B, Pooniya V, et al. Long-term conservation agriculture and intensified cropping systems: effects on growth, yield, water and energy-use efficiency of maize in north-western India. Pedosphere. 2018;28(6):952–63. doi:10.1016/S1002-0160(17)60468-5. [Google Scholar] [CrossRef]
27. Canakci M, Topakci M, Akinci I, Ozmerzi A. Energy use pattern of some field crops and vegetable production: case study for Antalya Region. Turkey Energy Convers Manag. 2005;46(4):655–66. doi:10.1016/j.enconman.2004.04.008. [Google Scholar] [CrossRef]
28. Yadav SN, Chandra R, Khura TK, Chauhan NS. Energy input-output analysis and mechanization status for cultivation of rice and maize crops in Sikkim. Int Agric Eng J. 2013;15(3):108–16. [Google Scholar]
29. Kumar V, Saharawat YS, Gathala MK, Jat AS, Singh SK, Chaudhary N, et al. Effect of different tillage and seeding methods on energy use efficiency and productivity of wheat in the Indo-Gangetic Plains. Field Crops Res. 2013;142:1–8. doi:10.1016/j.fcr.2012.11.013. [Google Scholar] [CrossRef]
30. Shahin S, Jafari A, Mobli H, Rafiee S, Karini M. Effect of farm size on energy ratio on wheat production, a case study from Arbadil province of Iran. Am-Eurasian J Agric Environ Sci. 2008;3:604–8. [Google Scholar]
31. Nassiri SM, Singh S. Nonparametric energy use efficiency, energy ratio and specific energy for irrigated wheat crop production. Iran Agric Res. 2009;27:30–8. [Google Scholar]
32. Rahman S, Rahman MS. Energy productivity and efficiency of maize accounting for the choice of growing season and environmental factors: an empirical analysis from Bangladesh. Energy J. 2013;49(1):329–36. doi:10.1016/j.energy.2012.10.042. [Google Scholar] [CrossRef]
33. Rahman S, Hassan MK. Energy productivity and efficiency of wheat farming in Bangladesh. Energy J. 2014;66:107–14. doi:10.1016/j.energy.2013.12.070. [Google Scholar] [CrossRef]
34. Zhang D, Zhang WF. Low carbon agriculture and a review of calculation methods for crop production carbon footprint accounting. Res Sci Educ. 2016;38(7):1395–405. doi:10.18402/resci.2016.07.19. [Google Scholar] [CrossRef]
35. Lal B, Gautam P, Nayak AK, Panda BB, Bihari P, Tripathi R, et al. Energy and carbon budgeting of tillage for environmentally clean and resilient soil health of rice–maize cropping system. J Clean Prod. 2019;226(8):815–30. doi:10.1016/j.jclepro.2019.04.041. [Google Scholar] [CrossRef]
36. Adaya B, Ertekin C, Evrendilek F. Total CO2-equivalent GHG Emissions from agricultural human labour in Turkey. Chem Eng Trans. 2017;58:37–42. doi:10.3303/CET1758007. [Google Scholar] [CrossRef]
37. Panse VG, Sukhatme PV. Statistical method for agricultural workers. 4th editionNew Delhi: ICAR, 1985. [Google Scholar]
38. Netam AK, Sahu B, Netam CR, Markam SK. Performance of crop residue management practices on growth and yield of wheat (Triticum aestivum L.) under rice–wheat system in Bastar region of Chhattisgarh, India. Int J Curr Microbiol Appl Sci. 2019;8(6):150–7. doi:10.20546/ijcmas.2019.806.019. [Google Scholar] [CrossRef]
39. Timalsina HP, Marahatta S, Sah SK, Gautam AK. Effect of tillage method, crop residue and nutrient management on growth and yield of wheat in rice–wheat cropping system at Bhairahawa condition. Agron J Nepal. 2021;5(1):52–62. doi:10.3126/ajn.v5i01.44783. [Google Scholar] [CrossRef]
40. Arora K, Bana RS, Sepat S, Phogat B, Devatwal P, Anchra S. Effect of residue recycling on leaf area index and nutrient uptake pattern of wheat crop under different potassium management practices. Biol Forum Int J. 2023;15(2):414–9. [Google Scholar]
41. Sirazuddin V, Singh P, Chandra S, Singh SP, Guru SK, Pareek N, et al. Growth and productivity of wheat under tillage systems and residue loads in Tarai Region of Uttarakhand, India. Int J Curr Microbiol Appl Sci. 2019;8(8):11–9. doi:10.20546/ijcmas.2019.808.003. [Google Scholar] [CrossRef]
42. Reddy MSL, Mitra B. Differential (Triticum aestivum L.) in eastern sub-Himalayan plains. J Agric Technol. 2023;10(2):43–52. [Google Scholar]
43. Choudhary L, Singh KN, Gangwar K, Sachan R. Effect of FYM and Inorganic fertilizers on growth performance, yield components and yield of wheat (Triticum aestivum L.) under Indo-Gangetic plain of Uttar Pradesh. Pharm Innov J. 2022;11(4):1476–9. [Google Scholar]
44. Mohanty SK, Singh AK, Jat SL, Parihar CM, Pooniya V, Sharma S, et al. Precision nitrogen-management practices influences growth and yield of wheat (Triticum aestivum) under conservation agriculture. Indian J Agron. 2015;60(4):617–21. doi:10.59797/ija.v60i4.4505. [Google Scholar] [CrossRef]
45. Kumar M, Singh B. Precision nutrient management in wheat using nutrient expert. Int J Curr Microbiol Appl Sci. 2019;8(2):2265–9. doi:10.20546/ijcmas.2019.802.263. [Google Scholar] [CrossRef]
46. Gayatri DR, Singh AK, Verma SON, Sahu U. Effect of Azotobacter and phosphate solubilizing bacteria on the yield of different wheat (Triticum aestivum L.) Cultivar. J Pharm Innov. 2022;11(1):1629–33. doi:10.5958/2229-4473.2017.00130.6. [Google Scholar] [CrossRef]
47. Kumar M, Pannu RK, Singh B. Response of irrigation frequencies and nitrogen doses on yield and yield attributes of late sown wheat. Ann Biol. 2017;33(1):69–73. [Google Scholar]
48. Pandey BP, Kandel TP. Growth and yield response of wheat to tillage, rice residue and weed management under rice–wheat copping system. Global J Agric Allied Sci. 2019;1(1):43–8. doi:10.35251/gjaas.2019.005. [Google Scholar] [CrossRef]
49. Singh P, Singh G, Sodhi GPS. Applying DEA optimization approach for energy auditing in wheat cultivation under rice–wheat and cotton–wheat cropping systems in north-western India. Energy. 2019;181(1):18–28. doi:10.1016/j.energy.2019.05.147. [Google Scholar] [CrossRef]
50. Singh P, Singh G, Sodhi GPS, Sharma S. Energy optimization in wheat establishment following rice residue management with Happy Seeder technology for reduced carbon footprints in north-western India. Energy. 2021;230(6206):120680. doi:10.1016/j.energy.2021.120680. [Google Scholar] [CrossRef]
51. Choudhary RL, Behera UK, Singh HV, Meena MD, Dotaniya ML, Jat RS. Energetics and nitrogen-use efficiency of Kharif maize in conservation agriculture-based maize (Zea mays)–wheat (Triticum aestivum) sequence. Int J Chem Stud. 2020;8(2):1252–8. doi:10.22271/chemi.2020.v8.i2s.8937. [Google Scholar] [CrossRef]
52. Hulmani S, Salakinkop SR, Somangouda G. Productivity, nutrient use efficiency, energetic, and economics of winter maize in south India. PLoS One. 2022;17(7):e0266886. doi:10.1371/journal.pone.0266886. [Google Scholar] [PubMed] [CrossRef]
53. Gathala MK, Laing AM, Tiwari TP, Timsina J, Islam S, Bhattacharya PM, et al. Energy-efficient, sustainable crop production practices benefit smallholder farmers and the environment across three countries in the Eastern Gangetic Plains, South Asia. J Clean Prod. 2020;246(3):118982. doi:10.1016/j.jclepro.2019.118982. [Google Scholar] [CrossRef]
54. Yadav GS, Das A, Kandpal BK, Babu S, Lal R, Datta M, et al. The food-energy-water-carbon nexus in a maize-mustard cropping sequence of the Indian Himalayas: an impact of tillage-cum-live mulching. Renew Sustain Energy Rev. 2021;151(4):111602. doi:10.1016/j.rser.2021.111602. [Google Scholar] [CrossRef]
55. Singh VK, Dwivedi BS, Singh Y, Singh SK, Mishra RP, Shukla AK, et al. Effect of tillage and crop establishment, residue management and K fertilization on yield, K use efficiency and apparent K balance under rice–maize system in north-western India. Field Crops Res. 2018;224:1–12. doi:10.1016/j.fcr.2018.04.012. [Google Scholar] [CrossRef]
56. Jat L, Rana NS, Naresh RK, Dhyani BP, Purushottam, Dimple, et al. Effect of integrated nutrient management on yield of wheat (Triticum aestivum L.) under in Indo-Gangetic Plains. J Pharmacogn Phytochem. 2020;9(6):1378–83. [Google Scholar]
57. Timsina J, Dutta S, Devkota KS, Chakraborty S, Krishna R, Neupane, et al. Assessment of nutrient management in major cereals: yield prediction, energy-use efficiency and greenhouse gas emission. Curr Res Environ Sustain. 2022;4(1):100147. doi:10.1016/j.crsust.2022.100147. [Google Scholar] [CrossRef]
58. Kakraliya SK, Jat HS, Singh I, Gora MK, Kakraliya M, Bijarniya D, et al. Energy and economic efficiency of climate-smart agriculture practices in a rice–wheat cropping system of India. Sci Rep. 2022;12(1):8731. doi:10.1038/s41598-022-12686-4. [Google Scholar] [PubMed] [CrossRef]
59. Parihar CM, Jat SL, Singh AK, Majumdar K, Jat ML, Saharawat YS, et al. Bioenergy, biomass water-use efficiency and economics of maize-wheat-mungbean system under precision-conservation agriculture in semiarid agro-ecosystem. Energy. 2017;119(2–3):245–56. doi:10.1016/j.energy.2016.12.068. [Google Scholar] [CrossRef]
60. Kumar P, Dubey SD, Tiwari US, Pandey RK, Hussain K, Singh RK. Azotobacter and PSB on growth and yield attributes of maize and wheat. Int J Curr Microbiol Appl Sci. 2021;10(6):764–73. doi:10.20546/ijcmas.2021.1006.083. [Google Scholar] [CrossRef]
61. Sarauskis E, Masilionyte L, Juknevicius D, Buragiene S, Kriauciuniene Z. Energy use efficiency, GHG emissions, and cost−effectiveness of organic and sustainable fertilization. Energy. 2019;172(3):1151–60. doi:10.1016/j.energy.2019.02.067. [Google Scholar] [CrossRef]
62. Harish MN, Choudhary AK, Singh YV, Pooniya V, Das A, Babu S, et al. Nutrient management practices for improved crop and water productivity, grain quality and energy productivity of promising rice cultivars in Eastern Himalayas. J Environ Biol. 2021;42(2):309–18. doi:10.22438/jeb/42/2/MRN-1372. [Google Scholar] [CrossRef]
63. Puniya R, Bazaya BR, Kumar A, Sharma BC, Ahmed N, Bochalya RS, et al. Effect of residue and weed management practices on weed flora, yield, energetics, carbon footprint, economics and soil quality of zero tillage wheat. Sci Rep. 2023;13:19311. doi:10.1038/s41598-023-45488-3. [Google Scholar] [PubMed] [CrossRef]
Cite This Article
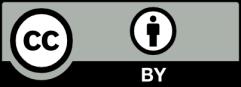
This work is licensed under a Creative Commons Attribution 4.0 International License , which permits unrestricted use, distribution, and reproduction in any medium, provided the original work is properly cited.