Open Access
ARTICLE
Seed Priming Improves Chilling Stress Tolerance in Rice (Oryza sativa L.) Seedlings
1 Department of Biochemistry and Molecular Biology, Bangladesh Agricultural University, Mymensingh, 2202, Bangladesh
2 Graduate School of Environmental, Life, Natural Science and Technology, Okayama University, Okayama, 700-8558, Japan
3 Atopy (Allergy) Research Center, Graduate School of Medicine, Juntendo University, Tokyo, 113-8421, Japan
4 Rice Farming Systems Division, Bangladesh Rice Research Institute, Gazipur, 1701, Bangladesh
5 Agricultural Research Station, Bangladesh Agricultural Research Institute, Satkhira, 9400, Bangladesh
6 Breeding Division, Bangladesh Sugarcrop Research Institute, Pabna, 6620, Bangladesh
7 Department of Horticulture, Faculty of Agriculture, South Valley University, Qena, 83523, Egypt
8 Botany and Microbiology Department, Faculty of Science, South Valley University, Qena, 83523, Egypt
9 Molecular Biology and Biotechnology Center, South Valley University, Qena, 83523, Egypt
* Corresponding Authors: Md. Tahjib-Ul-Arif. Email: ; Arafat Abdel Hamed Abdel Latef. Email:
(This article belongs to the Special Issue: Abiotic and Biotic Stress Tolerance in Crop)
Phyton-International Journal of Experimental Botany 2024, 93(11), 3013-3027. https://doi.org/10.32604/phyton.2024.058710
Received 19 September 2024; Accepted 24 October 2024; Issue published 30 November 2024
Abstract
Chilling is one of the major abiotic stresses for plants, especially for rice cultivation. Many essential metabolic processes for growth and development are temperature-dependent. In that case, reducing the negative effects of cold stress using exogenous chemicals is a possible option. Therefore, the current study examined the effects of pre-sowing seed treatment with different chemicals, viz. hydrogen peroxide (H2O2), salicylic acid (SA), calcium chloride (CaCl2), thiourea (TU), and citric acid (CA) on the germination of rice seeds (cv. BRRI dhan28) under chilling environments. Rice seeds were soaked in distilled water (control), 10 mM CA, 2 mM SA, 10 mM CaCl2, 10 mM H2O2, and 10 mM TU solutions for 24 h. After that, seeds were exposed to chilling stress by incubating at 4 ± 1°C for 8 h, followed by at 25 ± 2°C for 16 h for 7 days. Exposure to chilling stress significantly reduced the final germination percent (13.6%), germination rate index (36.0%), coefficient of the velocity (25.0%), shoot fresh weight (44.4%), and root fresh weight (60.5%). Moreover, chilling induced oxidative damage and reduced the activity of antioxidant enzymes (catalase and ascorbate peroxidase). In contrast, treatments with H2O2, SA, CaCl2, TU, and CA considerably enhanced germination indices and seedling growth compared to chilling stress conditions. The study showed that priming with H2O2, SA, CaCl2, TU, and CA significantly boosted antioxidant enzyme activities and reduced MDA and H2O2 contents in chilling-stressed rice plants, indicating less oxidative stress and improved tolerance. Principal component analysis showed that among these priming agents, H2O2, SA, and CA are most effective in chilling stress mitigation. Therefore, using seed-treating chemicals to combat the effect of chilling stress can help rice seedlings grow better in the winter season.Keywords
Rice (Oryza sativa L.) is the most vital cereal crop, serving as a staple food for over half of the world’s population [1,2]. There are more than 110 countries in the world occupying rice in almost 160 million hectares (mha) of land [3]. Bangladesh is the third-largest rice-producing country in the world, with a yearly production of 37.97 million tons in approximately 11 mha of land [4]. However, rice cultivation is constrained by various abiotic stresses, such as drought, salinity, high or low temperatures, flooding, heavy metals, etc. [5,6]. Chilling stress is ranked second among the abiotic stresses due to its severe effect on crops particularly in rice production in Bangladesh [7]. There are two major rice production seasons in Bangladesh, Aman (rainy) and Boro (winter or dry). The germination and seedling stages of Boro rice are exposed to cold waves. In particular, the establishment of rice seedlings is hindered in the northern part of Bangladesh in December and January due to low temperatures [7]. The low temperature causes poor germination, discoloration, chlorosis, and swallow seedlings, ultimately producing unhealthy seedlings [8–10]. The impaired seedlings eventually result in decreased crop production.
Plants experience oxidative stress when exposed to chilling stress [9,11]. Chilling stress induces an overproduction and accumulation of reactive oxygen species (ROS) within plant tissues, resulting in oxidative injury at both cellular and sub-cellular levels. Excessive ROS impairs cell membranes, disrupts ionic balance, and deactivates enzymes and proteins [11,12]. Plants typically possess a highly effective and complex antioxidant defense system to control the excessive accumulation of ROS [13]. This defense mechanism consists of various enzymatic components as well as non-enzymatic antioxidants [14,15]. The efficiency of plants in scavenging ROS has been associated with their ability to tolerate various environmental stresses like chilling [9,14,16]. To reduce abiotic stress-induced oxidative damage and improve the stress tolerance of crop plants, researchers are exploring various strategies and methodologies.
Seed priming is a widely utilized method in agriculture to enhance the germination rate, promote uniformity in seedling emergence, and facilitate optimal crop growth. This technique is favored for its simple execution and cost-effectiveness [17,18]. During the priming process, the activation of internal metabolic activities occurs concomitantly, preventing radicle protrusion. It has been observed that genes associated with stress tolerance, such as those involved in carbohydrate metabolism, protein synthesis, and signaling pathways, exhibited increased expression levels during the priming stage [19]. Furthermore, seed priming techniques have been employed to enhance the plant’s efficiency in adverse environmental circumstances. Following the process of post-priming germination, it has been observed that primed seeds exhibit better results compared to unprimed seeds when subjected to stressful environmental conditions. Recent studies have investigated the use of various compounds or factors as priming agents, including signaling molecules [9,20], temperature [21], ROS [22], osmolytes [22], hormones [23], salts [24], and antioxidants [24].
Studies showed that pre-treating Zoysia matrella plants with low concentrations of hydrogen peroxide (H2O2) may promote chilling tolerance [25]. Hydrogen peroxide can initiate the activation of antioxidant capacity within plants. This activation mitigates oxidative damage and enhances the physiological characteristics of plants experiencing stress [26–28]. By controlling the antioxidant enzyme activities in maize, wheat, and banana seedlings, exogenous salicylic acid (SA) also increases cold tolerance [29–31]. Calcium is an essential mineral that performs important structural and signaling roles and acts as a stress sensor and transducer for plants, modulating how they respond to abiotic stresses [32,33]. Priming with calcium chloride (CaCl2) significantly increased the harvest index, grain yield, and straw yield of late-sown wheat under chilling stress [34]. Thiourea (TU) has many beneficial effects, including the ability to improve plant growth and yield under stress conditions. TU applied as a foliar spray improves stress tolerance in plants [35,36]. Citric acid (CA) is an essential organic acid that is significantly associated with plant abiotic stress tolerance [37]. Therefore, it has been hypothesized that seed priming with SA, H2O2, CaCl2, CA, and TU might improve the chilling stress tolerance of rice seedlings.
This study aimed to investigate the comparative efficacy of H2O2, SA, Ca2+, TU, and CA priming in enhancing growth and activating stress-related defense mechanisms in rice seedlings under chilling stress. Our findings underscore the importance of these priming agents in improving seedling resilience and growth under cold conditions. This research contributes to the development of an effective strategy for managing chilling stress in rice, ultimately supporting agricultural productivity in the face of climate variability.
The experiment was performed using the BRRI dhan28 rice cultivar developed by the Bangladesh Rice Research Institute (BRRI). It is an Indica-type mega rice variety for the Boro season (winter rice) in Bangladesh. It is a cold-sensitive, high-yielding inbred rice cultivar. The seeds were first surface sterilized with 2.5% sodium hypochlorite (FUJIFILM Wako, Osaka, Japan) and 2.0% Tween-20 (FUJIFILM Wako, Osaka, Japan) for 5 min. Subsequently, the seeds were rinsed three times with distilled water. After that, different treatments were applied to the sterilized rice seeds for seed priming. All the seeds were soaked continuously for 24 h in each treatment, viz, in distilled water (control), 10 mM CA (TCI, Tokyo, Japan), 2 mM SA (Loba Chemie, India), 10 mM CaCl2 (TCI, Tokyo, Japan), 10 mM H2O2 (Millipore, Darmstadt, Germany), and 10 mM TU (TCI, Tokyo, Japan) solutions. Based on previous studies, we have fixed the concentration of H2O2 [38], SA [39], Ca2+ [40], TU [41], and CA [42] for seed priming. After soaking, seeds were rinsed thrice with distilled water before being put in 9 cm Petri dishes. Two layers of blotting paper were placed in the bottom of the petri dish and soaked with water daily. Each petri dish contained 100 rice seeds. These petri dishes were then incubated at 4 ± 1°C for 8 h, followed by at 25 ± 2°C for 16 h at 80 μmol m−2 s−1 light intensity, and this cycle was maintained for 7 days. For the control treatment, the petri dishes were kept at 25 ± 2°C for 7 days. The number of germinated seeds was counted starting on the second day after incubation (DAI). From the 2nd–8th DAI, various morphological features were observed, and samples were prepared for biochemical analysis using liquid N2. Each treatment was replicated three times. The overview of the experimental procedure is presented in Fig. 1.
Figure 1: Seed priming procedure with exogenous application of 10 mM hydrogen peroxide (H2O2), 2 mM salicylic acid, 10 mM calcium chloride (CaCl2), 10 mM thiourea, and 10 mM citric acid
2.2 Measurement of Germination Indices
The germination of seeds was considered to have occurred once the length of the radical had reached 2 mm. Starting from the second day after incubation (DAI), the number of germinated seeds was documented until the seventh DAI. These recorded germination counts were then utilized to calculate various germination indices, such as the final germination percentage (FGP), germination index (GI), mean germination time (MGT), germination rate index (GRI), and coefficient of the velocity of germination (CVG) (adopted from Afrin et al. [38] and Kader [43]).
(1)
(2)
(3)
(4)
(5)
where Sg represents the number of germinated seeds on the final day and ST represents the number of seeds tested for germination assay in Eq. (1). n1, n2 … n7 indicates the number of germinated seeds on the 1st, 2nd, and subsequent days until the 7th day and 7, 6 … and 1 are weights given to the number of germinated seeds on the 1st, 2nd, and subsequent days first, respectively in Eq. (2). Ni represents the germinated seed number on day i and Ti represents the number of days from sowing in Eqs. (3)–(5).
2.3 Growth Performance Measurement
During the 8th DAI, various growth factors were assessed. The growth factors were evaluated by quantifying shoot length (SL), root length (RL), and fresh weight (FW). The shoot length measurement was conducted by determining the distance between the shoot base and the leaf tip. Similarly, root length was determined by measuring the distance from the root base to the root tip. Twenty seedlings were selected from each treatment group, and their weights were measured to determine the shoot fresh weight (SFW) and root fresh weight (RFW).
2.4 Determination of Hydrogen Peroxide Content
The determination of hydrogen peroxide (H2O2) content was conducted using the methodology established by Velikova et al. [44]. Rice shoots weighing 0.1 g were subjected to grinding using 1.0 mL of a 0.10% trichloroacetic acid (TCA) (TCI, Tokyo, Japan) solution. The resulting mixture was then centrifuged at a force of 11,500× g for 12 min at a temperature of 4°C. A volume of 0.5 mL of the supernatant was transferred into a test tube. Then, 0.5 mL of a 10 mM potassium phosphate buffer (pH 7.0) and 1.0 mL of a 1.0 M potassium iodide (Millipore, Darmstadt, Germany) solution were added to the test tube. The resultant mixture was incubated in the absence of light for 60 min. The absorbance measurement was recorded at a wavelength of 390 nm using a Shimadzu UV-1201 spectrophotometer (Shimadzu, UV-1201, Kyoto, Japan).
2.5 Determination of Malondialdehyde Content
The level of lipid peroxidation in rice seedlings was assessed by measuring the amount of malondialdehyde (MDA), following the procedure explained by Heath et al. [45]. The rice shoots (0.1 g) were pulverized using 5.0% trichloroacetic acid (TCA) and subjected to centrifugation at 11,500× g for 10 min at 4°C. The supernatant was carefully transferred into a tube with a screw cap. Subsequently, 4 mL of a solution containing 20% trichloroacetic acid (TCA) and 0.5% thiobarbituric acid (TBA) (Loba Chemie, India) was added. Then, the tubes were kept in a water bath for 15 min at 90°C. After that, the tubes were promptly transferred into an ice bath and placed under centrifugation at a force of 11,500× g for 12 min. The absorbance of the chromophore was determined using a spectrophotometer (Shimadzu, UV-1201, Kyoto, Japan) operating at a wavelength of 532 nm. MDA content was quantified by employing the extinction coefficient of 155 mM−1 cm−1.
2.6 Antioxidant Enzymes Activity Assay
The shoots of rice that had germinated for 7 days were utilized to assess the activity of antioxidant enzymes, specifically catalase (CAT, EC: 1.11.1.6) and ascorbate peroxidase (APX, EC: 1.11.1.11). The determination of CAT activity was conducted using the methodology established by Fimognari et al. [46]. The CAT activity was determined by measuring the rate of absorbance decrease at a wavelength of 240 nm per minute. The extinction coefficient of H2O2 was found to be 39.4 M−1 cm−1. The measurement of APX activity was conducted by the methodology described by Tahjib-Ul-Arif et al. [40]. The activity of ascorbate peroxidase (APX) was determined by measuring the rate of change in absorbance at a wavelength of 290 nm over a one-minute time interval. The absorbance measurements were conducted using a UV-VIS spectrophotometer (Shimadzu, UV-1201, Tokyo, Japan). The extinction coefficient used for this calculation was 2.8 mM−1 cm−1.
Two-way analysis of variance and Tukey’s test were carried out to comprehend the statistically significant differences among treatments. The p-value less than 0.05 is considered a significant difference. The heatmap with hierarchical clustering was constructed using ‘pheatmap’ package. The principal component analysis (PCA) was constructed using ‘ggplot2’, ‘factoextra’, and ‘FactoMineR’ packages. All the analyses were performed in R 4.1.2 using RStudio.
3.1 Effects on Seed Germination
In comparison with normal temperature (25°C) control, the FGP, GRI, CVG, and GI were decreased (by 13.6%, 36.0%, 25.0%, and 34.9%, respectively) and MGT was increased (by 33.3%) significantly under chilling stress (Fig. 2). Priming with hydrogen peroxide (HP), SA, Ca, TU, and CA significantly increased FGP (by 15.4%, 12.1%, 8.5%, 9.7%, and 11.3%, respectively), GRI (by 36.9%, 18.7%, 12.3%, 12.8%, and 21.6%, respectively), and GI (by 39.8%, 19.9%, 11.1%, 12.1%, and 22.9%, respectively) vs. stressed alone plants (Fig. 2A,B,E). Priming of chilling-stressed rice seeds with HP, SA, and CA markedly increased the CVG (by 19.2%, 5.6%, and 8.6%, respectively) and decreased the MGT (by 16.2%, 5.2%, and 7.9%, respectively) compared to stressed alone plants (Fig. 2C,D). Among the treatments, priming with HP showed a greater effect on GRI, MGT, CVG, and GI, followed by priming with CA and SA (Fig. 2B–E).
Figure 2: Effects of different seed priming treatments on (A) final germination percent, FGP (%); (B) germination rate index, GRI (% day–1); (C) mean germination time, MGT (days); (D) coefficient of velocity of germination, CVG (% day–1); and (E) germination index, GI of rice seeds under chilling stress. The treatments included, Con, control; C, chilling + distilled water; C+CA, chilling + 10 mM citric acid; C+SA, chilling + 2 mM salicylic acid; C+Ca, chilling + 10 mM calcium chloride; C+HP, chilling + 10 mM hydrogen peroxide; and C+TU, chilling + 10 mM thiourea. The error bars represent standard error. Treatments with different letters are statistically different. Differences among the treatments were analyzed by Tukey’s test (p < 0.05)
3.2 Effects on Seedling Growth
Due to the exposure to chilling stress, all the phenotypic traits such as SL, RL, SFW, and RFW decreased significantly (by 73.7%, 46.8%, 44.4%, and 60.5%, respectively) compared to non-stress conditions (Fig. 3A–D). However, CA-, SA-, Ca-, HP-, and TU-primed seeds grown under chilling stress induced a significant improvement of SL (by 115.4%, 103.9%, 114.1%, 98.4%, and 78.9%, respectively), RL (by 58.1%, 66.9%, 42.5%, 61.9%, and 40.8%, respectively), and RFW (by 74.3%, 101.3%, 112.5%, 102.6%, and 75.0%, respectively) compared to that grown under only chilling stress conditions (Fig. 3A,B,D). Priming with CA, SA, Ca, and HP significantly boosted the SFW (by 38.6%, 42.9%, 43.3%, and 36.3%, respectively) of chilling-stressed rice seedlings than the non-primed seeds grown under chilling stress (Fig. 3C). The photograph demonstrated that priming treatments with CA, SA, Ca, HP, and TU have effectively enhanced the growth of rice seedlings under chilling stress (Fig. 3E).
Figure 3: Effects of different seed priming treatments on (A) shoot length (SL); (B) root length (RL); (C) shoot fresh weight (SFW); (D) root fresh weight (RFW), and (E) phenotypic appearances of rice seedlings under chilling stress. The treatments included, Con, control; C, chilling + distilled water; C+CA, chilling + 10 mM citric acid; C+SA, chilling + 2 mM salicylic acid; C+Ca, chilling + 10 mM calcium chloride; C+HP, chilling + 10 mM hydrogen peroxide; and C+TU, chilling + 10 mM thiourea. The error bar represents the standard error. Treatments with different letters are statistically different. Differences among the treatments were analyzed by Tukey’s test (p < 0.05)
3.3 Effect on ROS Scavenging Capacity, Hydrogen Peroxide and Malondialdehyde
A significant increase in H2O2 (by 98.5%) and MDA (by 49.4%) contents was observed in rice seedlings exposed to chilling treatment (C) compared to those grown under normal conditions. However, HP-, SA-, Ca-, TU-, and CA-primed rice seeds grown under chilling stress exhibited significantly lower H2O2 content (by 15.1%, 18.9%, 12.5%, 13.6%, and 16.5%, respectively) and MDA content (by 32.4%, 37.0%, 26.5%, 24.4%, and 33.0%, respectively) compared to non-primed seeds under the same stress conditions (Fig. 4A,B). Among these priming agents, SA was the most effective in reducing MDA content, followed by CA and HP (Fig. 4B).
Figure 4: Effects of different seed priming treatments on (A) hydrogen peroxide (H2O2) content (nmol g–1 FW), (B) malondialdehyde (MDA) content (nmol g–1 FW), (C) catalase (CAT) activity (mmol min–1 g–1 FW), and (D) ascorbate peroxidase (APX) activity (µmol min–1 g–1 FW) in leaves of rice (Oryza sativa L.) seedlings under chilling stress. The treatments included, Con, control; C, chilling + distilled water; C+CA, chilling + 10 mM citric acid; C+SA, chilling + 2 mM salicylic acid; C+Ca, chilling + 10 mM calcium chloride; C+HP, chilling + 10 mM hydrogen peroxide; and C+TU, chilling + 10 mM thiourea. The error bars represent standard error. Treatments with different letters are statistically different. Differences among the treatments were analyzed by Tukey’s test (p < 0.05)
Rice seedlings exposed to chilling stress exhibited significantly lower activities of CAT (by 48.0%) and APX (by 34.9%) compared to non-stressed seedlings (Fig. 4C,D). However, HP-, SA-, Ca-, TU-, and CA-primed rice seeds grown under chilling stress showed significantly elevated activities of CAT (by 250.8%, 249.2%, 173.1%, 153.8%, and 186.9, respectively) and APX (by 175.5%, 136.1%, 127.3%, 121.9%, and 158.5%, respectively) compared to non-primed rice seedlings (Fig. 4C,D). Among these treatments, HP, SA, and CA were most effective in improving antioxidant defense under chilling stress (Fig. 4C,D).
3.4 Hierarchal Clustering with Heatmap and PCA
The mean values of all the variables were normalized to perform the heatmap and PCA. The first two components of PCA, PC1 and PC2 altogether explain 93.3% of the total variability of the dataset. The heatmap categorized all variables into three major groups (I, II, and III). The first two components of PCA, PC1, and PC2, together explained 93.3% of the total variability in the dataset. The heat map classified all variables into three main groups (I, II, and III). The first cluster consisted of MGT, MDA, and H2O2, the second cluster consisted of antioxidant enzymes CAT and APX; the third cluster consisted of the remaining parameters, which mainly represented the phenotypic responses of rice seedlings (Fig. 5A). From the PCA, the variables MGT, MDA, and H2O2 were highly correlated with the C+TU and C+Ca treatments. The variables APX and CAT were closely correlated with the C+SA and C+CA treatments. However, the other variables FGP, RL, RFW, SFW, GI, GRI, SL, and CVG showed higher correlations with the C+HP treatment (Fig. 5B).
Figure 5: Treatment-variable interaction with hierarchal clustering (A), and principal component analysis (PCA) (B). The mean values of different variables were normalized and analyzed for constructing the hierarchal clustering and PCA. Three distinct clusters were formed (I, II, and III) at variable levels. The variables included MGT, mean germination time; H2O2, hydrogen peroxide; MDA, malondialdehyde content; APX, ascorbate peroxidase activity, CAT, catalase activity; FGP, final germination (%); RL, root length; RFW, root fresh weight; SFW, shoot fresh weight; GI, germination index; GRI, germination rate index; SL, shoot length; CVG, coefficient of velocity of germination. The treatments included, Con, control; C, chilling + distilled water; C+CA, chilling + 10 mM citric acid; C+SA, chilling + 2 mM salicylic acid; C+Ca, chilling + 10 mM calcium chloride; C+HP, chilling + 10 mM hydrogen peroxide; and C+TU, chilling + 10 mM thiourea
The seed germination stage and early seedling establishment are considered the most critical factors in determining the success or failure of crop establishment [47]. Rice seedlings are particularly vulnerable to chilling stress, especially during their early developmental stages [48]. Chilling stress severely impairs the growth and development of rice seedlings. Under chilling conditions, rice seedlings exhibited slowed germination, reduced root and shoot growth, and overall poor vigor [49]. Additionally, chilling stress affects key physiological processes such as photosynthesis, impairing the activity of crucial enzymes and nutrient uptake, further weakening the growth of seedlings [50]. However, seed priming with SA, HP, CaCl2, CA, and TU has shown promise in mitigating the hostile effects of chilling stress on rice seedlings. In this study, enhanced seed germination, healthier seedling growth, and improved antioxidant defense mechanism, in pre-treated seeds suggested that the exogenous seed priming agents amended the tolerance of rice seedlings against chilling stress.
In the present research, prolonged chilling stress significantly decreased FGP, GRI, GI, and CVG; while it increased the MGT in rice (Fig. 2). The result is in harmony with previous studies, which claim that cold stress restricts the kinetics of several physiological and metabolic processes in plants, which ultimately hinder seed germination and growth of rice [51], cotton [52], wheat [53], and maize [54]. The findings also showed that seeds under cold stress not only decrease seed germination rate but also decrease seed vigor. The RL, SL, SFW, and RFW decrease in chilling treatment in comparison to control and other chemical treatments (Fig. 2). A similar result is reported where plant growth parameters were negatively affected by cold stress in rice [10], chickpea [55], and maize [56]. The impact of chilling stress on root growth and development is characterized by a reduction in root length, biomass, and morphology, leading to a decrease in the overall volume of the root system [57]. This, in turn, hampers the root’s ability to efficiently absorb essential nutrients and water [57,58]. The results of the study indicate that the application of chemical treatments, including HP, SA, Ca2+, CA, and TU during chilling stress, positively influence the growth of rootlets and shootlets in seedlings of Oryza sativa L. (Fig. 3). According to previous studies, SA causes an increase in cell division within the apical meristem of seedling, which led to the enhancement of RL and SL [59–62]. In addition, Ca2+, HP, and CA activated different signaling mechanisms which ultimately modulated growth-promoting gene expression and antioxidant defense system that improved root and shoot growth of plants [63–65]. Furthermore, TU helps maintain membrane integrity that enables healthier root and shoot growth under cold conditions [66,67].
It was observed from the experiment that, exposure to chilling stress resulted in a significant increase in the activity of CAT and APX enzymes (Fig. 4). However, the observed level of increase in APX and CAT activity does not appear to be adequate for effectively safeguarding plants against chilling-induced stress. The present experiment exhibited a decline in growth and biomass (Fig. 3). Jan et al. [68] found that the application of an enhanced enzymatic antioxidant could potentially mitigate the harmful effects of ROS in rice plants. This, in turn, could protect cellular membranes and various cellular components, thereby enhancing the overall tolerance of the plants [63].
In the intricate cycle of crop growth, the germination stage is a crucial starting point for a successful harvest. For rice, a crop sensitive to temperature, chilling stress can severely disrupt this process, leading to weak seedlings and poor development. Our research findings highlight that chilling stress presents major challenges like slowing germination, reducing root growth, and limiting biomass. However, by priming rice seeds with substances like HP, SA, CaCl2, CA, and TU, we observed improved seedling growth and stronger antioxidant defenses. These treatments not only enhanced germination but also helped the plants better cope with oxidative stress by boosting key antioxidant enzymes (CAT and APX).
In this study, the application of different priming agents revealed distinct benefits at various stages of rice development under chilling stress. Specifically, HP was most effective in enhancing germination indices (Fig. 2). Conversely, other agents, such as CA, SA, and Ca, demonstrated superior effectiveness in promoting seedling growth under the same stress conditions. These results indicate that the use of a combination of priming agents could offer a more comprehensive strategy for improving chilling stress tolerance in rice. By targeting different physiological processes, germination with HP, and growth with CA, SA, or CaCl2, priming can lead to improved crop establishment and resilience. The use of multiple agents could ultimately enhance overall crop performance, which is crucial for maintaining productivity in regions prone to low-temperature stress during critical growth stages. However, further studies should be conducted in this direction in the future.
In conclusion, the study demonstrates that seed priming is an effective and promising tool to mitigate chilling stress and unlock the plant’s full potential for improving seedling growth. Overall, the heatmap and PCA analysis suggest that priming with HP, SA, and CA has shown better performance in mitigating the adverse effects of chilling stress. However, future research should focus on exploring the molecular mechanisms underlying the protective effects and validating its use in agricultural practices through field trials.
Acknowledgement: None.
Funding Statement: The authors received no specific funding for this study.
Author Contributions: Study conception and design: Md. Tahjib-Ul-Arif; data collection: Md. Tahjib-Ul-Arif; analysis and interpretation of results: Md. Tahjib-Ul-Arif; draft manuscript preparation: Md. Asaduzzaman, Bir Jahangir Shirazy, Md. Shihab Uddine Khan, A. M. Sajedur Rahman; manuscript review and editing: Yoshiyuki Murata, Sozan Abdel Hamed, Arafat Abdel Hamed Abdel Latef. All authors reviewed the results and approved the final version of the manuscript.
Availability of Data and Materials: Not applicable.
Ethics Approval: Not applicable.
Conflicts of Interest: The authors declare no conflicts of interest to report regarding the present study.
References
1. Sen S, Chakraborty R, Kalita P. Rice-not just a staple food: a comprehensive review on its phytochemicals and therapeutic potential. Trends Food Sci Technol. 2020;97:265–85. doi:10.1016/j.tifs.2020.01.022. [Google Scholar] [CrossRef]
2. Shirazy B, Mostafizur A, Khatun L, Khatun A, Rashid M, Akter N. Evaluation of salt tolerant winter rice variety for coastal region of Bangladesh. Int J Appl Res. 2019;5:63–6. [Google Scholar]
3. Freed S, Kura Y, Sean V, Mith S, Cohen P, Kim M, et al. Rice field fisheries: wild aquatic species diversity, food provision services and contribution to inland fisheries. Fish Res. 2020;229:105615. doi:10.1016/j.fishres.2020.105615. [Google Scholar] [CrossRef]
4. Chowhan S, Rahman MM, Sultana R, Rouf MA, Islam M, Jannat SA. Agriculture policy and major areas for research and development in Bangladesh. Sarhad J Agric. 2024;40:819–31. [Google Scholar]
5. Amin MR, Zhang J, Yang M. Effects of climate change on the yield and cropping area of major food crops: a case of Bangladesh. Sustainability. 2014;7:898–915. [Google Scholar]
6. Osmani SR, Ahmed AU, Ahmed T, Hossain N, Huq S, Shahan A. Strategic review of food security and nutrition in Bangladesh. Dhaka, Bangladesh: World Food Programme (WFP); 2016. Available from:https://www.wfp.org/content/food-and-nutrition-security-bangladesh. [Accessed 2024]. [Google Scholar]
7. Rashid M, Yasmeen R. Cold injury and flash flood damage in Boro rice cultivation in Bangladesh: a review. Bangladesh Rice J. 2017;21:13–25. [Google Scholar]
8. Lukatkin AS, Brazaitytė A, Bobinas Č, Duchovskis P. Chilling injury in chilling-sensitive plants: a review. Zemdirbyste-Agric. 2012;99:111–24. [Google Scholar]
9. Sohag AAM, Tahjib-Ul-Arif M, Afrin S, Khan MK, Hannan MA, Skalicky M, et al. Insights into nitric oxide-mediated water balance, antioxidant defence and mineral homeostasis in rice (Oryza sativa L.) under chilling stress. Nitric Oxide. 2020;100:7–16 [Google Scholar] [PubMed]
10. Shi Y, Guo E, Cheng X, Wang L, Jiang S, Yang X, et al. Effects of chilling at different growth stages on rice photosynthesis, plant growth, and yield. Environ Exp Bot. 2022;203:105045. doi:10.1016/j.envexpbot.2022.105045. [Google Scholar] [CrossRef]
11. Hussain HA, Hussain S, Khaliq A, Ashraf U, Anjum SA, Men S, et al. Chilling and drought stresses in crop plants: implications, cross talk, and potential management opportunities. Front Plant Sci. 2018;9:393. doi:10.3389/fpls.2018.00393 [Google Scholar] [PubMed] [CrossRef]
12. Tarchoune I, Sgherri C, Izzo R, Lachaal M, Ouerghi Z, Navari-Izzo F. Antioxidative responses of Ocimum basilicum to sodium chloride or sodium sulphate salinization. Plant Physiol Biochem. 2010;48:772–7. doi:10.1016/j.plaphy.2010.05.006 [Google Scholar] [PubMed] [CrossRef]
13. Arif N, Yadav V, Shweta S, Kushwaha BK, Singh S, Tripathi DK, et al. Assessment of antioxidant potential of plants in response to heavy metals. In: Singh A, Prasad S, Singh R, editors. Plant responses to xenobiotics. Singapore: Springer; 2006. p. 97–125. [Google Scholar]
14. Gill SS, Tuteja N. Reactive oxygen species and antioxidant machinery in abiotic stress tolerance in crop plants. Plant Physiol Biochem. 2010;48:909–30. doi:10.1016/j.plaphy.2010.08.016 [Google Scholar] [PubMed] [CrossRef]
15. Dawood MFA, Tahjib-Ul-Arif M, Sohag AAM, Abdel Latef AAH. Role of acetic acid and nitric oxide against salinity and lithium stress in Canola (Brassica napus L.). Plants. 2024;13:51. [Google Scholar]
16. Abdel Latef AAH, Chaoxing H. Arbuscular mycorrhizal influence on growth, photosynthetic pigments, osmotic adjustment and oxidative stress in tomato plants subjected to low temperature stress. Acta Physiol Plant. 2011;33:1217–25. doi:10.1007/s11738-010-0650-3. [Google Scholar] [CrossRef]
17. Abdel Latef AAH, Tran L-SP. Impacts of priming with silicon on the growth and tolerance of maize plants to alkaline stress. Front Plant Sci. 2016;7:243 [Google Scholar] [PubMed]
18. Marthandan V, Geetha R, Kumutha K, Renganathan VG, Karthikeyan A, Ramalingam J. Seed priming: a feasible strategy to enhance drought tolerance in crop plants. Int J Mol Sci. 2020;21:8258. doi:10.3390/ijms21218258 [Google Scholar] [PubMed] [CrossRef]
19. Soeda Y, Konings MC, Vorst O, van Houwelingen AM, Stoopen GM, Maliepaard CA, et al. Gene expression programs during Brassica oleracea seed maturation, osmopriming, and germination are indicators of progression of the germination process and the stress tolerance level. Plant Physiol. 2005;137:354–68. doi:10.1104/pp.104.051664 [Google Scholar] [PubMed] [CrossRef]
20. Dawood MF, Sohag AAM, Tahjib-Ul-Arif M, Abdel Latef AAH. Hydrogen sulfide priming can enhance the tolerance of artichoke seedlings to individual and combined saline-alkaline and aniline stresses. Plant Physiol Biochem. 2021;159:347–62. doi:10.1016/j.plaphy.2020.12.034 [Google Scholar] [PubMed] [CrossRef]
21. Paparella S, Araújo S, Rossi G, Wijayasinghe M, Carbonera D, Balestrazzi A. Seed priming: state of the art and new perspectives. Plant Cell Rep. 2015;34:1281–93. doi:10.1007/s00299-015-1784-y [Google Scholar] [PubMed] [CrossRef]
22. Gelaw TA, Sanan-Mishra N. Molecular priming with H2O2 and proline triggers antioxidant enzyme signals in maize seedlings during drought stress. BBA-Gen Subjects. 2024;1868(7):130633. doi:10.1016/j.bbagen.2024.130633 [Google Scholar] [PubMed] [CrossRef]
23. Savvides A, Ali S, Tester M, Fotopoulos V. Chemical priming of plants against multiple abiotic stresses: mission possible? Trends Plant Sci. 2016;21:329–40. doi:10.1016/j.tplants.2015.11.003 [Google Scholar] [PubMed] [CrossRef]
24. Tahjib-Ul-Arif M, Afrin S, Polash MAS, Akter T, Ray SR, Hossain MT, et al. Role of exogenous signaling molecules in alleviating salt-induced oxidative stress in rice (Oryza sativa L.a comparative study. Acta Physiol Plant. 2019;41:1–14. [Google Scholar]
25. Wang Y, Li J, Wang J, Li Z. Exogenous H2O2 improves the chilling tolerance of manilagrass and mascarenegrass by activating the antioxidative system. Plant Growth Regul. 2010;61:195–204. doi:10.1007/s10725-010-9470-0. [Google Scholar] [CrossRef]
26. Goud P, Kachole M. Effect of exogenous hydrogen peroxide on peroxidase and polyphenol oxidase activities in Cajanuscajan (L.) Millsp. detached leaves. Inter J Curr Res. 2011;3:61–5. [Google Scholar]
27. He L, Gao Z. Pretreatment of seed with H2O2 enhances drought tolerance of wheat (Triticum aestivum L.) seedlings. Afr J Biotechnol. 2009;8(22):6151–7. doi:10.5897/AJB. [Google Scholar] [CrossRef]
28. Abdel Latef AAH, Kordrostam M, Zakir A, Zaki H, Saleh OM. Eustress with H2O2 facilitates plant growth by improving tolerance to salt stress in two wheat cultivars. Plants. 2019;8(9):303. doi:10.3390/plants8090303 [Google Scholar] [PubMed] [CrossRef]
29. Janda T, Szalai G, Tari I, Paldi E. Hydroponic treatment with salicylic acid decreases the effects of chilling injury in maize (Zea mays L.) plants. Planta. 1999;208:175–80. doi:10.1007/s004250050547. [Google Scholar] [CrossRef]
30. Kang G, Wang C, Sun G, Wang Z. Salicylic acid changes activities of H2O2-metabolizing enzymes and increases the chilling tolerance of banana seedlings. Environ Exp Bot. 2003;50:9–15. doi:10.1016/S0098-8472(02)00109-0. [Google Scholar] [CrossRef]
31. Taşgın E, Atıcı Ö, Nalbantoğlu B, Popova LP. Effects of salicylic acid and cold treatments on protein levels and on the activities of antioxidant enzymes in the apoplast of winter wheat leaves. Phytochemistry. 2006;67:710–5. doi:10.1016/j.phytochem.2006.01.022 [Google Scholar] [PubMed] [CrossRef]
32. Dodd AN, Kudla J, Sanders D. The language of calcium signaling. Annu Rev Plant Biol. 2010;61:593–620. doi:10.1146/arplant.2010.61.issue-1. [Google Scholar] [CrossRef]
33. Abdel Latef AAH. Ameliorative effect of calcium chloride on growth, antioxidant enzymes, protein patterns and some metabolic activities of canola (Brassica napus L.) under seawater stress. J Plant Nutr. 2011;34:1303–20. doi:10.1080/01904167.2011.580817. [Google Scholar] [CrossRef]
34. Farooq M, Aziz T, Basra S, Wahid A, Khaliq A, Cheema M. Exploring the role of calcium to improve chilling tolerance in hybrid maize. J Agron Crop Sci. 2008;194:350–9. doi:10.1111/jac.2008.194.issue-5. [Google Scholar] [CrossRef]
35. Baqer RA, Al-Kaaby HK, Adul-Qadir LH. Antioxidant responses in wheat plants (Triticum aestivum L.) treated with thiourea. Plant Arch. 2020;20:717–22. [Google Scholar]
36. Yadav T, Kumar A, Yadav R, Yadav G, Kumar R, Kushwaha M. Salicylic acid and thiourea mitigate the salinity and drought stress on physiological traits governing yield in pearl millet-wheat. Saudi J Biol Sci. 2020;27:2010–7. doi:10.1016/j.sjbs.2020.06.030 [Google Scholar] [PubMed] [CrossRef]
37. Tahjib-Ul-Arif M, Zahan MI, Karim MM, Imran S, Hunter CT, Islam MS, et al. Citric acid-mediated abiotic stress tolerance in plants. Int J Mol Sci. 2021;22(13):7235. doi:10.3390/ijms22137235 [Google Scholar] [PubMed] [CrossRef]
38. Afrin S, Tahjib-Ul-Arif M, Sakil MA, Sohag AAM, Polash MAS, Hossain MA. Hydrogen peroxide priming alleviates chilling stress in rice (Oryza sativa L.) by enhancing oxidant scavenging capacity. Fundam Appl Agric. 2019;4(1):713–22. [Google Scholar]
39. Apon TA, Ahmed SF, Bony ZF, Chowdhury MR, Asha JF, Biswas A. Sett priming with salicylic acid improves salinity tolerance of sugarcane (Saccharum officinarum L.) during early stages of crop development. Heliyon. 2023;9:e16030. doi:10.1016/j.heliyon.2023.e16030 [Google Scholar] [PubMed] [CrossRef]
40. Tahjib-Ul-Arif M, Roy PR, Sohag AAM, Afrin S, Rady MM, Hossain MA. Exogenous calcium supplementation improves salinity tolerance in BRRI dhan28; a salt-susceptible high-yielding Oryza sativa cultivar. J Crop Sci Biotechnol. 2018;21:383–94. doi:10.1007/s12892-018-0098-0. [Google Scholar] [CrossRef]
41. Anjum F, Wahid A, Farooq M, Javed F. Potential of foliar applied thiourea in improving salt and high temperature tolerance of bread wheat (Triticum aestivum). Int J Agri Biol. 2011;13(2):251–6. [Google Scholar]
42. Lu LL, Tian SK, Yang XE, Peng HY, Li TQ. Improved cadmium uptake and accumulation in the hyperaccumulator Sedum alfredii: the impact of citric acid and tartaric acid. J Zhejiang Univ Sci B. 2013;14:106–14. doi:10.1631/jzus.B1200211 [Google Scholar] [PubMed] [CrossRef]
43. Kader MA. A comparison of seed germination calculation formulae and the associated interpretation of resulting data. J Proc R Soc NSW. 2005;138:65–75. [Google Scholar]
44. Velikova V, Yordanov I, Edreva A. Oxidative stress and some antioxidant systems in acid rain-treated bean plants: protective role of exogenous polyamines. Plant Sci. 2000;151:59–66. doi:10.1016/S0168-9452(99)00197-1. [Google Scholar] [CrossRef]
45. Heath RL, Packer L. Photoperoxidation in isolated chloroplasts: I. Kinetics and stoichiometry of fatty acid peroxidation. Arch Biochem Biophys. 1968;125:189–98. doi:10.1016/0003-9861(68)90654-1 [Google Scholar] [PubMed] [CrossRef]
46. Fimognari L, Dölker R, Kaselyte G, Jensen CN, Akhtar SS, Großkinsky DK, et al. Simple semi-high throughput determination of activity signatures of key antioxidant enzymes for physiological phenotyping. Plant Methods. 2020;16:1–9. [Google Scholar]
47. Finch-Savage WE, Bassel GW. Seed vigour and crop establishment: extending performance beyond adaptation. J Exp Bot. 2016;67(3):567–91. doi:10.1093/jxb/erv490 [Google Scholar] [PubMed] [CrossRef]
48. da Cruz RP, Sperotto RA, Cargnelutti D, Adamski JM, de FreitasTerra T, Fett JP. Avoiding damage and achieving cold tolerance in rice plants. Food Energy Secur. 2013;2:96–119. doi:10.1002/fes3.2013.2.issue-2. [Google Scholar] [CrossRef]
49. Wang W, Chen Q, Hussain S, Mei J, Dong H, Peng S, et al. Pre-sowing seed treatments in direct-seeded early rice: consequences for emergence, seedling growth and associated metabolic events under chilling stress. Sci Rep. 2016;6:19637. doi:10.1038/srep19637 [Google Scholar] [PubMed] [CrossRef]
50. Kondamudi R, Swamy KN, Chakravarthy DVN, Vishnuprasanth V, Rao YV, Rao PR, et al. Heat stress in rice—physiological mechanisms and adaptation strategies. In: Venkateswarlu B, Shanker A, Shanker C, Maheswari M, editors. Crop stress and its management: perspectives and strategies. Dordrecht: Springer; 2012. p. 193–224. [Google Scholar]
51. Cong Dien D, Yamakawa T. Phenotypic variation and selection for cold-tolerant rice (Oryza sativa L.) at germination and seedling stages. Agriculture. 2019;9:162. doi:10.3390/agriculture9080162. [Google Scholar] [CrossRef]
52. Shen Q, Zhang S, Liu S, Chen J, Ma H, Cui Z, et al. Comparative transcriptome analysis provides insights into the seed germination in cotton in response to chilling stress. Int J Mol Sci. 2020;21:2067. doi:10.3390/ijms21062067 [Google Scholar] [PubMed] [CrossRef]
53. Calderon Flores P, Yoon JS, Kim DY, Seo YW. Effect of chilling acclimation on germination and seedlings response to cold in different seed coat colored wheat (Triticum aestivum L.). BMC Plant Biol. 2021;21:252. doi:10.1186/s12870-021-03036-z [Google Scholar] [PubMed] [CrossRef]
54. Sun Y, He Y, Irfan AR, Liu X, Yu Q, Zhang Q, et al. Exogenous brassinolide enhances the growth and cold resistance of maize (Zea mays L.) seedlings under chilling stress. Agronomy. 2020;10:488. doi:10.3390/agronomy10040488. [Google Scholar] [CrossRef]
55. Nayyar H, Bains T, Kumar S. Chilling stressed chickpea seedlings: effect of cold acclimation, calcium and abscisic acid on cryoprotective solutes and oxidative damage. Environ Exp Bot. 2005;54:275–85. doi:10.1016/j.envexpbot.2004.09.007. [Google Scholar] [CrossRef]
56. Waqas MA, Khan I, Akhter MJ, Noor MA, Ashraf U. Exogenous application of plant growth regulators (PGRs) induces chilling tolerance in short-duration hybrid maize. Environ Sci Pollut Res. 2017;24:11459–71. doi:10.1007/s11356-017-8768-0 [Google Scholar] [PubMed] [CrossRef]
57. Shahzad N, Nabi HG, Qiao L, Li W. The molecular mechanism of cold-stress tolerance: cold responsive genes and their mechanisms in rice (Oryza sativa L.). Biology. 2024;13:442. doi:10.3390/biology13060442 [Google Scholar] [PubMed] [CrossRef]
58. Tachibana S. Effect of root temperature on the rate of water and nutrient absorption in cucumber cultivars and figleaf gourd. J Jpn Soc Hortic Sci. 1987;55:461–7. doi:10.2503/jjshs.55.461. [Google Scholar] [CrossRef]
59. Cutt J, Klessing D. Salicylic acid in plants: a changing perspective. Pharm Technol. 1992;16:26–34. [Google Scholar]
60. Hmmam I, Ali AE, Saleh SM, Khedr N, Abdellatif A. The role of salicylic acid in mitigating the adverse effects of chilling stress on “Seddik” mango transplants. Agronomy. 2022;12:1369. doi:10.3390/agronomy12061369. [Google Scholar] [CrossRef]
61. Sakhabutdinova A, Fatkhutdinova D, Bezrukova M, Shakirova F. Salicylic acid prevents the damaging action of stress factors on wheat plants. Bulg J Plant Physiol. 2003;21:314–9. [Google Scholar]
62. Sher A, Wang X, Sattar A, Ijaz M, Ul-Allah S, Nasrullah M, et al. Exogenous application of thiourea for improving the productivity and nutritional quality of bread wheat (Triticum aestivum L.). Agronomy. 2021;11:1432. doi:10.3390/agronomy11071432. [Google Scholar] [CrossRef]
63. Hossain MA, Bhattacharjee S, Armin S-M, Qian P, Xin W, Li H-Y, et al. Hydrogen peroxide priming modulates abiotic oxidative stress tolerance: insights from ROS detoxification and scavenging. Front Plant Sci. 2015;6:420 [Google Scholar] [PubMed]
64. Prasad TK, Anderson MD, Martin BA, Stewart CR. Evidence for chilling-induced oxidative stress in maize seedlings and a regulatory role for hydrogen peroxide. Plant Cell. 1994;6:65–74. doi:10.2307/3869675. [Google Scholar] [CrossRef]
65. Wang Y, Shen C, Jiang Q, Wang Z, Gao C, Wang W. Seed priming with calcium chloride enhances stress tolerance in rice seedlings. Plant Sci. 2022;323:111381. doi:10.1016/j.plantsci.2022.111381 [Google Scholar] [PubMed] [CrossRef]
66. Ahmed N, Rahman K, Rahman M, Sathi KS, Alam MM, Nahar K, et al. Insight into the thiourea-induced drought tolerance in two chickpea varieties: regulation of osmoprotection, reactive oxygen species metabolism and glyoxalase system. Plant Physiol Biochem. 2021;167:449–58. doi:10.1016/j.plaphy.2021.08.020 [Google Scholar] [PubMed] [CrossRef]
67. Fiaz K, Maqsood MF, Shahbaz M, Zulfiqar U, Naz N, Gaafar A-RZ, et al. Application of thiourea ameliorates drought induced oxidative injury in Linum usitatissimum L. by regulating antioxidant defense machinery and nutrients absorption. Heliyon. 2024;10(4):e25510. doi:10.1016/j.heliyon.2024.e25510 [Google Scholar] [PubMed] [CrossRef]
68. Jan R, Asif S, Asaf S, Du XX, Park JR, Nari K, et al. Melatonin alleviates arsenic (As) toxicity in rice plants via modulating antioxidant defense system and secondary metabolites and reducing oxidative stress. Environ Pollut. 2023;318(3):120868. doi:10.1016/j.envpol.2022.120868 [Google Scholar] [PubMed] [CrossRef]
Cite This Article
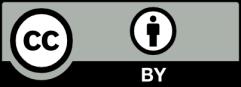
This work is licensed under a Creative Commons Attribution 4.0 International License , which permits unrestricted use, distribution, and reproduction in any medium, provided the original work is properly cited.