Open Access
ARTICLE
The Developmental and Physiological Traits of Rare and Threatened Moss Physcomitrium eurystomum Sendtn. (Funariaceae) Valuable for Its Conservation
1 Institute of Botany and Botanical Garden Jevremovac, Faculty of Biology, University of Belgrade, Belgrade, 11000, Serbia
2 Biodiversity, Ecology and Environment Area, Research and Innovation Centre of Fondazione Edmund Mach, San Michele all’Adige (TN), 38098, Italy
3 Division of Botany, Department of Biology, Faculty of Science, University of Zagreb, Zagreb, 10000, Croatia
4 Center of Plant Biotechnology and Conservation (CPBC), Belgrade, 11000, Serbia
5 Institute of Biology and Ecology, Department of Plant Biology, Faculty of Science, Pavol Jozef Šafárik University in Košice, Košice, 040 01, Slovakia
6 NBFC, National Biodiversity Future Center, Palermo, 90133, Italy
* Corresponding Author: Marko S. Sabovljević. Email:
Phyton-International Journal of Experimental Botany 2024, 93(11), 2949-2961. https://doi.org/10.32604/phyton.2024.057995
Received 02 September 2024; Accepted 10 October 2024; Issue published 30 November 2024
Abstract
Physcomitrium eurystomum Sendtn. is a very rare European ephemeral funaroid moss. The entire European population of this species is considered threatened and it is red-listed in many regions and countries. In addition to being recognized as threatened and included in nature conservation legislation, it also requires active protection measures. This study aims to contribute to effective conservation practices for P. eurystomum. Different conservation physiology tests were carried out to propagate this species to achieve a reliable procedure for biomass production and the potential reintroduction of germplasm. Ex situ tests, both in vitro and ex vitro, were carried out to determine the optimal method for spore production under laboratory conditions, considering that the spores are the best propagules for the reintroduction of the species. An important outcome of this research is a deeper understanding of the role of the spore bank of this species for its survival in a variable environment. Some additional characterizations of the biology of this ephemeral moss species are also discussed, such as the functioning of the photosynthetic apparatus of the vegetative vs. sexual phases to clarify the transition to the reproductive phase. It is proposed that both types of sporophytes be used in conservation practices due to viable spore bank self-sustainability in rapidly changing environmental conditions.Keywords
Bryophytes, like other organisms, are vulnerable to rapid environmental changes, and as they are adapted to specific microhabitats, their populations can easily decline. While red lists highlight such entities, the biological features of rare and threatened species often remain obscure and unknown. Recently, the emerging scientific field of conservation physiology has led to a new approach to conservation science and practice. It involves conducting studies and tests in both field and laboratory conditions to develop effective recovery measures for target species, thus increasing their survival potential. Such a concept has emerged over the last two decades [1] and is now widely accepted. More recently, this concept has driven ex situ conservation, also in bryophytes [2–4]. This approach has documented the important features of some rare and threatened species. For example, in Sabovljević et al. [5], the authors found spore dormancy and strategies for its disruption in the brackish water liverwort Riella helicophylla (Bory et Mont.) Mont. In addition, it was also discovered that the salty grassland species Entosthodon hungaricus (Boros) Loeske is indifferent to salt, thriving in such sites due to its low competition with other mosses from such habitats [6]. Laboratory experiments have facilitated methodological improvements and provided new insights into the biology of these species and also growth optimization for some rare and threatened bryophyte species (e.g., Entosthodon pulchellus (H. Philib.) Brugues [7], Pterygoneurum sibiricum Otnyukova [8] and Hennediella heimii (Hedw.) R. H. Zander [9]).
Physcomitrium eurystomum Sendtn. is a riparian funaroid moss species with a temperate distribution, mainly in Europe and Asia, but it is also recorded out of its main range. It is an ephemeral species that occurs on muddy soils along streams and the margins of lakes with fluctuating water levels [10,11]. This acrocarpous moss with an annual shuttle life strategy, rapid life span, and frequent formation of sporophytes often appears twice a year in favorable sites during the wet seasons (i.e., spring and autumn) when the muddy banks of water bodies are affected by flooding and general water mirror fluctuations. Interestingly, the spores of the species can be found in sediments more than 100 years old, which remain dormant until optimal conditions occur in the habitat and serve as a spore bank [12].
It has been confirmed that P. eurystomum has an allopolyploid origin [13–16], resulting from a hybridization event between the two early divergent lineages of the Physcomitrium-Physcomitrella complex, with the ancestral population sizes of these lineages being significantly smaller than their current population sizes [16].
The overall European population of this species is assessed as threatened with extinction and is considered vulnerable (VU). Also, within the European Union, the species is red-listed as endangered (EN) [17]. In Austria, Great Britain and Hungary, the species is also listed nationally as EN and in the Czech Republic, Estonia, Germany, Slovakia and Switzerland as VU [18]. The species is also localized in Serbia and is listed as EN [19]. According to Hodgetts & Lockhart [18], the species is considered to be at some risk of extinction in Belarus, Belgium and the Netherlands, while it has not been observed in Slovenia since 1910 [20]. There are also some more recent, but rather rare, reports in Europe [21].
This study aim to assess whether the spores originating from sporophyte capsules obtained under axenic conditions can serve as viable material for reintroduction and population reinforcement procedures in nature. Specifically, we investigated the germination percentage of spores at two different developmental stages prior to the natural opening and release of the capsules, which are characterized by pale and dark coloration despite there being no morphological differences between them. We address three main questions: (1) Can spores at different maturity stages achieve successful germination under suboptimal in vitro conditions? (2) Do plants in the reproductive phase exhibit different photosynthesis and CO2 assimilation rates to maintain the higher energy costs of sexual reproduction compared to non-reproductive plants? (3) Can leafy gametophores be used for the successful acclimation of the species ex vitro in an ex situ environment?
The plant material of P. eurystomum originated from a population located on the shores of the Bilje lake, situated in the Baranja Region of Croatia (18.736°E, 45.5901°N, altitude 84 m a.s.l.). The collection took place on 23 September 2017, leg. A. Rimac, N. Koletić, N. Vuković, det. A. Rimac (voucher ZA49325). Lake Bilje is a lowland area between the Drava and Danube rivers. It is a eutrophic oxbow lake, an old meander of the Drava River, with gently sloping muddy and sandy margins. The total area of the lake is 0.56 km2.
P. eurystomum was found growing in habitats typical for this species, where it was associated with other bryophytes such as Riccia rhenana Lorb. ex Müll., Physcomitrium patens (Hedw.) Mitt., and Leptobryum pyriforme (Hedw.) Wilson. It was found in the vegetation of the alliances Nanocyperion W. Koch ex Libbert 1932 and Phragmition australis W. Koch 1926.
This genotype of P. eurystomum was cultivated in vitro to obtain sufficient biomass for testing in this study and for ex situ conservation purposes.
2.2 In Vitro Culture Establishment, Growth Conditions and Growth Optimization
Mature sporophyte capsules, separated from the collected specimens and cleaned of residual debris, were used to establish the axenic in vitro culture of P. eurystomum. The capsules were rinsed in distilled water and immersed in different concentrations (1%, 3%, 5%, 7%, 10%, 13% and 15%) of sodium hypochlorite (NaOCl, as commercial bleach Lavax Varikinal, 3.5% of active chlorine) and NaDCC (sodium dichloroisocyuronate, Sigma Aldrich) solutions at the same concentrations (1%, 3%, 5%, 7%, 10%, 13% and 15%) to determine the optimal concentration of sterilizing agent that would eliminate all contaminating cohabitants and ensure the avoidance of spore damage. Furthermore, the exposure time of the sporophyte capsules to each solution was varied (30, 60, 90, 120, 180 and 300 s) in order to achieve cohabitant-free material prior to spreading them on KNOP basal medium [22] in sterile conditions under the laminar hood.
Petri dishes containing the spores were maintained under sterile conditions for two months at a constant temperature of 18 ± 2°C, humidity of 60%–70%, and a long day light regime (16 h light/8 h dark). Illumination was provided by cool white fluorescent tubes (Tesla Pančevo) with a photon flux density of 40 µmol m−2 s−1. After the spores had germinated and the plantlets developed a primary protonema, axenic, cohabitant-free plant material was obtained. Growth optimization and micro-propagation of the plant material followed previously elaborated methods [8,9].
2.3 In Vitro Micro-Propagation and Induction of the Sporophyte Formation
Once the cohabitant-free gametophores (leafy plantlets) of the species were obtained and the most suitable growth conditions were found, the micro-propagation of P. eurystomum was carried out with the aim of obtaining sufficient biomass for the experiments. Plant explants were subcultured and propagated bimonthly on fresh KNOP minimal medium [22], with the pH adjusted to 5.8, prior to autoclaving at 121°C for 45 min. It is important to note that the plant material was derived from multiple micro-propagated gametophores (clones) that emerged from different spores in order to maintain some genetic diversity within the tested population. Once sufficient plant biomass was obtained, the plantlets were placed under a long-day light regime (16 h light/8 h dark) with low light intensity (10 µmol m−2 s−1) to induce sporophyte formation.
2.4 Characterization of Sporogenesis
Once the sex organs and sporophytes had formed, the number of capsules emerging from a single moss patch, the number of spores per capsule and the spore diameter were determined. To determine the spore parameters, aliquots of spore suspensions from randomly selected capsules were prepared by crushing urns and dispersing the spores in Eppendorf tubes containing sterile distilled water.
Aliquots for the assessment of the number of spores per capsule were prepared by crushing one capsule in an Eppendorf tube containing 100 µL of sterile distilled water. The number of spores per capsule was determined by counting the spores in a 0.1 µL portion of the suspension using conventional light microscopy (Leica DMLS, Santa Clara, CA, USA) and extrapolating the count to the entire sample. Seven capsules (aliquots) with seven subsamples each (49 observations in total) were used to determine the average number of spores per capsule.
The spore diameter was determined by mixing the remaining aliquots (increased randomization) and measuring the diameter of individual spores using a Nikon ECLIPSE Si stereomicroscope (Nikon, Tokyo, Japan) and Bresser MikroCamLab II software for microscopic imaging at 10× magnification with a sample size of 30 spores.
In the axenically-grown moss patches, the fully developed, mature capsules were either pale or dark. Both sporophytes looked mature, i.e., they appeared to be full of spores and their calyptra had detached from the capsule, when spore germination of both types was evaluated. The spore germination assays consisted of two experimental groups with sporophytes of different colors in order to test which one was more suitable for axenic/xenic propagation of the species and whether the spores were germinable and mature enough for dispersal at any level of sporophyte maturity. In conservation practice, it is of great importance whether the spores can adapt, germinate and survive to an acceptable extent when dispersed under more variable natural conditions.
In order to test the hypothesis that spores at different stages of maturity achieve successful germination under suboptimal in vitro conditions, aliquots of spores were prepared by crushing two sporophyte capsules per experimental group in 1600 µL of sterile distilled water each. Spore sowing was performed in six petri dishes per experimental group, and 50 µL of the aliquot were dispersed in a thin film on the KNOP minimal growth medium. This ensured the optimum spore density and number of spores per petri dish so that they could be counted upon germination over time. The total number of spores observed per experimental group was 1010 and 1029 for the experimental groups with pale and dark capsules, respectively. The spores were grown under the same controlled conditions as previously described (see plant material section). Spore germination was assessed every 4 days through nine time intervals by counting the germinated spores using a Leica MZ stereomicroscope (Leica MZ 7.5 Bi-Optic Inc. Santa Clara, CA, USA). It is important to note that the first measurements (first time interval) were taken three days after the start of spore germination (when the first germinated spore was detected). A spore was considered to be germinated once the initial deformation of the spore exine by the emerging protonemal filament was observed, and the germination assays were conducted until the emergence of the first gametophore buds of the protonemata.
2.6 Measurements of Chlorophyll Fluorescence
The experiment consisted of three experimental groups, the control group (gametophytes without developed sex organs or sporophytes) and the moss patches with predominantly pale and dark sporophyte capsules (explained in the section on spore germination assays). In order to test the hypothesis that in the reproductive phase plants exhibit different rates of photosynthesis and CO2 assimilation to maintain the higher energy cost of sexual reproduction compared to non-reproductive plants, various parameters describing the functioning of the photosynthetic apparatus of the moss were assessed.
Chlorophyll a fluorescence was measured using a FluorCam 800 MF instrument (Photon Systems Instruments Ltd., Brno, Czech Republic). The measurements were performed on well-hydrated, dark-adapted samples (for 10 min) using a saturating flash of light (2000 μmol m−2 s−1) for 1 s. Several parameters were measured, including the maximum quantum yield of photosystem II (QY max), which was determined using the Fv/Fm ratio calculated as (Fm–F0)/Fm, where F0 represents the ground fluorescence in the dark-adapted state and Fm represents the maximum fluorescence during a saturating radiation pulse in the dark-adapted state. Photochemical quenching (Qp), non-photochemical quenching (NPQ), peak fluorescence (Fp) and the fluorescence decrease ratio (Rfd) were also measured. Two areas (one per phylloid) were measured on four gametophytes (one per petri dish), resulting in a total of 8 measurements per experimental group.
2.7 Acclimation of P. Eurystomum
When a sufficient biomass of the in vitro propagated gametophytes was obtained, their acclimation followed. The mosses propagated in axenic cultures were placed on a substrate material collected in the vicinity of Lake Dajićko on Golija Mountain, W. Serbia (May 2021), closely resembling the species’ natural substrate. They were kept shaded and saturated with water every fourth day. The acclimation test for the leafy gametophores for xenic ex situ propagation took place in the open environment of the Institute of Botany and Botanical Garden Jevremovac, Belgrade, Serbia.
The entire statistical analysis was performed using the R programming language (v. 4.3.1) [23]. Different types of statistical analysis were used depending on the experiment and data obtained. In the case of the spore germination assays, binomial logistic regression [24] was used to predict the probability of germination using two categorical predictors and their interaction (sporophyte capsule appearance and time expressed as a time category). This method was used because the germination data follow a binomial distribution, since spores, just like seeds in angiosperms, can either germinate or not [25]. After the logistic regression was implemented, estimated marginal means (EMMs) were calculated using the emmeans function from the emmeans R package (version 1.8.9; http://cran.r-project.org/package=emmeans, accessed on 09 May 2024). Pairwise comparisons were then performed using contrast tests with the Benjamini-Hochberg p-value adjustment method, employing the contrast function from the same R package.
Different types of analyses were used for the data obtained from the chlorophyll a fluorescence measurements compared to the germination data. Firstly, a preliminary data exploration was conducted in order to determine the appropriate analysis method using the Levene test to assess the homogeneity of variance and the Shapiro–Wilk normality test to determine whether the data followed a normal distribution. The results showed that not all experimental groups followed a normal distribution and that the homogeneity of variance was violated across the groups. Consequently, a non-parametric Kruskal-Wallis test was used for the comparisons of the experimental groups, followed by a Dunn test for multiple comparisons with the Benjamini-Hochberg p-value adjustment method. The significance level (α) was set at 0.05.
3.1 In Vitro Sporogenesis Characterization
After subcultivation on KNOP minimal media (Fig. 1A) under low light intensity (10 µmol m−2 s−1), sporophytes of varying morphological appearance emerged. Two months after subculturing, pale capsules appeared, while it took four months for the dark capsules to emerge (Fig. 1B), both containing viable spores with no morphological distinctions (Fig. 1C). We consider pale capsules of yellowish to light brown coloration as almost mature sporophytes, although morphologically they are fully developed. These spores participate in the spore-bank and retain their viability. These sporophytes did not continue to develop further but changed coloration to dark brown over time, possibly due to the accumulation of secondary metabolites and loss of photosynthetic ability. The number of sporophytes per moss patch originating from a single starting explant varied between 1 and 14 after 60 days. The spores of this species, derived from in vitro cultivation, displayed a brown-yellowish color with spinose-papillose ornamentation (Fig. 1B). The measured spore diameter varied between 27.41 and 36.2 µm (min-max values) with an average spore size of 32.13 ± 0.35 µm (mean ± standard error). The average number of spores per capsule was also determined, ranging between 10,286 and 13,857 spores (min-max values), with an average of 12,245 ± 498 spores per capsule (mean ± standard error).
Figure 1: The appearance of Physcomitrium eurystomum explants in vitro (A), with fully developed sporophytes of different morphological appearance (B) and spores of the species originating from in vitro propagated plants (C). The bars correspond to a size of 2 mm (A and B) and 50 µm (C)
3.2 Effects of Sporophyte Developmental Stage on Spore Germinability
Both individual factors (time frame and developmental stage of the sporophyte) had a significant effect on the predicted spore germination (i.e., germinability) of P. eurystomum (p < 0.001) grown under axenic laboratory conditions. However, no significant interactions between time (expressed as a time category) and developmental stage were statistically supported (Table 1), suggesting that developmental stage does not modify the effect of time on predicted spore germination (germinability). This absence of any interaction suggests that time and developmental stage influence spore germination independently, with no multiplicative or combined effects.
The spores originating from the sporophytes of different morphological appearance (pale/dark) began to germinate at different times. The first germinated spores were observed after 29 and 7 days in the spores from the pale and dark sporophyte capsules, respectively. In general, the percentage of germination increased over time in both experimental groups and reached saturation towards the end of the experiment (Fig. 2), with the spores from the light and dark sporophyte capsules reaching a peak of 8.42% and 40.43%, respectively.
Figure 2: The predicted probability of the germination of P. euristomum spores originating from the pale (light green) and dark (dark green) sporophyte capsules. The time frame is shown on the x-axis, with the numbers indicating the number of days elapsed since the sowing of the spores from the pale and dark sporophyte capsules (the numbers on the left and right, respectively). The data are presented as mean values, with the error bars indicating the 95% CI. The letters above the bars indicate the statistically significant differences (p < 0.05) between the experimental groups
Further observation of germination showed that the spores from the pale-colored sporophyte capsules exhibited significantly lower germination percentages throughout the experimental timeframe, i.e., for each time category (p < 0.05) (Fig. 2). Notably, after the eighth time category (i.e., 60 days after sowing) the spores from the pale capsules exhibited a similar germination percentage to those from the dark-colored capsules in the second time category (14 days after sowing). It was also evident that the spores originating from the dark capsules reached the plateau phase of germination earlier than those from the pale capsules, with the last four or two time categories showing no statistically significant differences (Fig. 2). The spore germination assays were conducted for nine time categories until the occurrence of gametophore buds on the protonemata, which was documented for the spores from the pale and dark sporophyte capsules after 64 and 42 days, respectively.
3.3 Functioning of the Photosynthetic Apparatus in Different Developmental Phases of P. Eurystomum
The maximum quantum yield of photosystem II in the dark-adapted state (QY max) showed a significant increase (p < 0.05), indicating that the maximum photosynthetic output was higher in the mosses developing sporophytes (both the pale and dark capsules bearing moss patches) compared with the control group, i.e., those moss patches without developed sporophytes or sexual organs (Fig. 3A). The NPQ values (Fig. 3B) decreased in the mosses with dark sporophyte capsules compared to the control group (p < 0.05). In contrast, the NPQ measurements showed no statistically significant differences between the mosses with pale-colored capsules and the control group, nor between the two groups of mosses with sporophytes. Furthermore, there were no statistically significant differences among the experimental groups in the data obtained for qP (Fig. 3C). The stable Rfd values (Fig. 3D) indicated that the vitality of the mosses was maintained in all the experimental groups. However, a slight but non-significant decrease in Rfd values was documented in the mosses bearing dark capsules.
Figure 3: The chlorophyll a fluorescence parameters (dark-adapted samples) of P. eurystomum plants without (control) and with sporophytes of different morphological appearance (pale and dark capsules). The maximum quantum yield of photosystem II (A); non-photochemical quenching (B); photochemical quenching (C); and the fluorescence decline ratio (D). The values are presented as mean ± standard error (SE). The letters above the bars indicate the statistically significant differences (p < 0.05) between the experimental groups
3.4 Acclimation of P. Eurystomum Gametophytes
Mature gametophytes were successfully acclimated outside the axenic culture on a suitable substrate (lakeside sediments), away from direct sunlight, during spring in the ex situ conditions of the Belgrade Botanical Garden. Media-free moss patches were placed above the substrate, and gametophore multiplication was achieved. Newly formed shoots were observed (Fig. 4A,B) during the three-month period (late February–late May 2024) allowing for the accumulation of sufficient biomass for population strengthening and potential reintroductions. However, with the increase of daily temperatures and the decrease in air humidity, the visual vitality of the moss seemed to decline until they fully disappeared. Under these conditions, we were unable to detect any sex organs or sporophytes.
Figure 4: The ex situ growth and propagation of ex vitro vegetative material of Physcomitrium eurystomum placed on lake sediment substrate and successfully multiplied for a short period at the Institute of Botany and Botanical Garden Jevremovac, Belgrade, Serbia. Initial moss patch (A) and well spreading moss patch in ex situ conditions (B)
The in vitro sporogenesis in P. eurystomum seems to have some peculiarities compared to the sporogenesis documented in nature [10], even though the morphology of the plants seems to be the same. In general, in vitro sporogenesis is a much rarer event compared to that in nature because the culture conditions present a stressful environment for the plant, hence sexual organs rarely appear and the reproduction conditions are difficult to meet [2,3,4]. Furthermore, fertilization success in monocultures is more likely to occur in monoicous species [26,27]. The spore sizes appear to vary somewhat, which is not surprising given that they are generally highly variable in the Funariaceae family [15]. In specimens of P. eurystomum collected from nature they are reported to be 30–40 µm [28], 33–40 µm [29], and 32–46 µm [15]. The observed spore diameter developed in axenic cultures was 32.13 ± 1.93 µm (mean ± standard deviation) and appears to be at the lower end of the previously reported size ranges, which could possibly be explained by the stress conditions during axenic cultivation.
Obtaining viable spores of the species grown in axenic cultures has strong conservation implications. It provides a germplasm of reproductive material that enables rapid propagation of the species, which is of great importance for obtaining sufficient biomass prior to population strengthening and possible reintroduction [6]. Although the plants reproduce sexually, there is a high rate of intragametangial self-fertilization, resulting in completely homozygous sporophytes [27,30,31], and because there is no crossing-over due to the clonal gametophytes, genetically uniform spores are formed. The spores originating from in vitro cultures did not express any dormancy or recalcitrance problems, but this cannot be ruled out for long-term spore storage.
There are no previous data on the germination rates of P. eurystomum in vitro or in nature. However, a similar methodology of germination assays with aliquoting and spore sowing on plant growth media has been applied to Physcomitrium patens (Hedw.) Mitt., another phylogenetically related species [32–34]. In the case of P. patens, a higher germination percentage was generally observed, albeit on different growth media, while a similar timing of germination onset and a similar trend of cumulative germination percentage were reported. The saturation of the germination curve in P. patens [32] occurs at a similar time as documented in this study for the spores of P. eurystomum released from the dark sporophyte capsules.
We assume that the change in coloration with maturation was a response to the accumulation of secondary metabolites as the spores appeared to be morphologically equally developed and capable of germination. The differences observed in this study are in accordance with the survival strategy of this species in nature, where not all spores should germinate at the same time, thus avoiding suboptimal stochastic climate oscillations and where a certain number of un-germinated spores must be maintained in the spore bank for further suitable growth environmental conditions. It seems that both types of mature sporophytes (i.e., pale and dark) contribute to the spore bank, as shown by the empirical observations in this study. The spores originating from the pale capsules seem to have programmed delayed germination (survival adaptation), but no distinction in their ability to yield fully developed moss gametophores, as confirmed by laboratory tests. Therefore, both types of capsules should be included in the ex situ conservation of this ephemeral funaroid species.
There are no previous data on photosynthetic measurements of any kind available for P. eurystomum. However, studies have included measurements of the maximum quantum yield [35] of dark-adapted plants in P. patens grown in vitro [36], and the values of light grown samples are similar to the results obtained in this study.
The reduced heat dissipation assessed by non-photochemical quenching and the increased values of maximum photosynthetic output in the plants with dark sporophyte capsules, together with the constant values of photochemical quenching indicate that increased assimilation is required in plants that have entered the reproductive phase. This is in accordance with the general assumption that reproductive effort has high energy costs for the organism. A similar phenomenon was also observed in an endangered cycad species (Zamia portoricensis Urb.), where a higher photosynthetic rate was documented in fertile plants compared to sterile plants at the canopy level [37]. Numerous other studies have shown that tracheophytes can also increase photosynthesis during the reproduction phase [38–40]. In P. eurystomum, the decrease in NPQ suggests that this photo-protective mechanism is less activated in plants with a dark sporophyte capsule, probably due to a higher maximum photosynthetic output, at constant light intensity.
The observed (albeit non-significant) decrease in the fluorescence decline ratio, a parameter proposed to quantify plant fitness, in the mosses with dark sporophytes may indicate a possible decrease in fitness that can be explained by a significant allocation of resources to reproductive tissues at the expense of vegetative development, widely known as reproductive effort.
Gametophore multiplication under ex situ conditions is highly dependent on the microclimate, but is also affected by the weather. Spring time was chosen to imitate the natural growth conditions of P. eurystomum. However, parameters such as frequently changing humidity and light quality seem to play an important role in the transition to sex organ development and spore production. The preliminary open space test suggests that cool, humid and shady conditions are favorable for vegetative propagation, but further tests are needed to discover the triggers for the transition to sexual reproduction under ex situ and ex vitro conditions.
Given the numerous parameters involved in the germination, establishment and survival of this species in nature, we can assume from these experiments that both types of sporophytes are welcome in conservation practice. In addition, gametophores can also be used, but only in short seasons when the optimal ecological conditions (humid and cool periods) are present for an appropriate period of time. However, in accordance with climatic and ecological variations, genetic diversity may also play a pivotal role in the survival rate of this species in its native habitats. Biotic factors and possible hybridization with other funaroid species should also be considered.
The axenic culture of the rare and widely threatened species, P. eurystomum, has been established and the optimal conditions for its vegetative growth and biomass production have been achieved. Additionally, light intensity under laboratory conditions seems to be the main trigger for the transition to sexual reproduction in P. eurystomum. Laboratory produced viable spores can be obtained by sporophyte induction in vitro, and newly developed spores from both the pale and dark capsules can be used to form a natural self-sustaining spore bank, as inferred by the laboratory test due to the presence of delayed germination in some of them. This approach is highly valuable when harvesting sporophytes from the laboratory, enabling the collection of both pale and dark sporophytes for the transfer of spores to natural sites. The measurements of the photosynthetic parameters suggest a higher energy cost during the transition from vegetative to sexual phases as well as higher reproductive effort in those mosses bearing dark capsules. Although the propagation of P. eurystomum in ex situ conditions by vegetative moss patches originating from axenic cultures is feasible, further tests are needed to assess both spore and gametophore dispersal in natural conditions. Certain constraints in conservation practices still remain for effective species survival action plans: the lack or limited knowledge of the biology of the species, including hybridization with other funaroid species, the genetic structure of native local and global populations, the regulation of spore dormancy (induction and elimination), competition with other co-inhabitants for resources, and other biotic interactions.
Acknowledgement: The authors wish to thank the anonymous reviewers who provided constructive suggestions for improving the manuscript.
Funding Statement: The project is supported by the Serbian Ministry of Science, Technological Development and Innovations, Contract Nos. 451-03-65/2024-03/200178 and 451-03-66/2024-03/200178. Djordje Božović was supported through the framework of the PhD program of the Edmund Mach Foundation. This work was further supported by the Autonomous Province of Trento through core funding of the Ecogenomics Unit.
Author Contributions: The authors confirm their contribution to the paper as follows: study conception and design: Marko S. Sabovljević, Aneta D. Sabovljević, Mingai Li, and Claudio Varotto; data collection: Djordje P. Božović, Anja Rimac, Michal Goga, Pragya Singh, and Milorad M. Vujičić; analysis and interpretation of the results: Djordje P. Božović, Marko S. Sabovljević, and Mingai Li; draft manuscript preparation: Djordje P. Božović, and Marko S. Sabovljević. All authors reviewed the results and approved the final version of the manuscript.
Availability of Data and Materials: The data that support the findings of this study are available from the corresponding authors upon reasonable request.
Ethics Approval: Not applicable.
Conflicts of Interest: The authors declare that they have no conflicts of interest to report regarding the present study.
References
1. Tracy CR, Nussear KE, Esque TC, Dean-Bradley K, Tracy CR, DeFalco LA, et al. The importance of physiological ecology in conservation biology. Integr Comp Biol. 2006;46(6):1191–205. doi:10.1093/icb/icl054 [Google Scholar] [PubMed] [CrossRef]
2. Rowntree JK, Pressel S, Ramsay MM, Sabovljević A, Sabovljević M. In vitro conservation of European bryophytes. In Vitro Cell Dev Biol Plant. 2011;47(1):55–64. [Google Scholar]
3. Sabovljević M, Vujičić M, Pantovic J, Sabovljević A. Bryophyte conservation biology: in vitro approach to the ex situ conservation of bryophytes from Europe. Plant Biosyst. 2014;148(4):857–68. [Google Scholar]
4. Sabovljević MS, Ćosić MV, Jadranin BZ, Pantović JP, Giba ZS, Vujičić MM, et al. The conservation physiology of bryophytes. Plants. 2022;11(10):1282. doi:10.3390/plants11101282 [Google Scholar] [PubMed] [CrossRef]
5. Sabovljević MS, Segarra-Moragues JG, Puche F, Vujičić M, Cogoni A, Sabovljević A. Eco-physiological and biotechnological approach to conservation of the world-wide rare and endangered aquatic liverwort Riella helicophylla (Bory et Mont.) Mont. Acta Bot Croat. 2016;75(2):194–8. doi:10.1515/botcro-2016-0030. [Google Scholar] [CrossRef]
6. Sabovljević M, Nikolić N, Vujičić M, Šinžar-Sekulić J, Pantović J, Papp B, et al. Ecology, distribution, in vitro propagation, ex situ conservation and native population strengthening of the rare and threatened halophyte moss Entosthodon hungaricus in Serbia. Wulfenia. 2018;25:117–30. [Google Scholar]
7. Ćosić MV, Božović DP, Ignatov MS, Ignatova EA, Vujičić MM, Sabovljević AD, et al. Micropropagation and optimization of in vitro production of the rare and threatened moss Entosthodon pulchellus (Funariaceae). Acta Bot Croat. 2025;84(2). doi:10.37427/botcro-2025-012. [Google Scholar] [CrossRef]
8. Jadranin BZ, Ćosić MV, Božović DP, Vujičić MM, Ignatov MS, Ignatova EA, et al. An insight into the biology of the rare and peculiar moss Pterygoneurum sibiricum (Pottiaceaea conservation physiology approach. Plants. 2023;12(6):1359. doi:10.3390/plants12061359 [Google Scholar] [PubMed] [CrossRef]
9. Ćosić MV, Sabovljević MS, Papp B, Giba ZS, Šinžar-Sekulić JB, Sabovljević AD, et al. Micropropagation of rare bryo-halophyte Hennediella heimii. Bot Serb. 2022;46(2):187–95. doi:10.2298/BOTSERB2202187C. [Google Scholar] [CrossRef]
10. Bansal P, Srivastava A. Sporophyte characterization and sporogenesis in Physcomitrium eurystomum Sendtn. (Bryophyta: funariaceae). Caryologia. 2017;70(2):120–7. [Google Scholar]
11. Chetia T, Roy H. Physcomitrium eurystomum Sendtn. (Funariaceaea rare species recorded for Assam. India J Threat Taxa. 2024;16(6):25474–7. doi:10.11609/jott.8923.16.6.25474-25477. [Google Scholar] [CrossRef]
12. Callaghan DA, During H, Medina R, Yang H. Long-term survival of bryophytes underground: an investigation of the diaspore bank of Physcomitrium eurystomum Sendtn. J Bryol. 2022;44(2):1–9. [Google Scholar]
13. Patel N, Medina R, Williams LD, Lemieux O, Goffinet B, Johnson MG. Frequent allopolyploidy with distant progenitors in the moss genera Physcomitrium and Entosthodon (Funariaceae) identified via subgenome phasing of targeted nuclear genes. Evolution. 2023;77(12):2561–75. doi:10.1093/evolut/qpad171 [Google Scholar] [PubMed] [CrossRef]
14. Beike AK, von Stackelberg M, Schallenberg-Rüdinger M, Hanke ST, Follo M, Quandt D, et al. Molecular evidence for convergent evolution and allopolyploid speciation within the Physcomitrium-Physcomitrella species complex. BMC Evol Biol. 2014;14(1):158. doi:10.1186/1471-2148-14-158 [Google Scholar] [PubMed] [CrossRef]
15. Ostendorf AK, van Gessel N, Malkowsky Y, Sabovljevic MS, Rensing SA, Roth-Nebelsick A, et al. Poliploidization within the Funariaceae—A key principle behind speciation, sporophyte reduction and the high variance of spore diameters? Bryophyte Diversit Evoluti. 2021;43(1):164–79. doi:10.11646/bde.43.1.13. [Google Scholar] [CrossRef]
16. McDaniel SF, Von Stackelberg M, Richardt S, Quatrano RS, Reski R, Rensing SA. The speciation history of the Physcomitrium-Physcomitrella species complex. Evolution. 2010;64(1):217–31. doi:10.1111/j.1558-5646.2009.00797.x [Google Scholar] [PubMed] [CrossRef]
17. Hodgetts N, Cálix M, Englefield E, Fettes N, García Criado M, Patin L, et al. A miniature world in decline: European Red List of Mosses, Liverworts and Hornworts. Brussels, Belgium: IUCN; 2019. [Google Scholar]
18. Hodgetts NG, Lockhart N. Checklist and country status of European bryophytes—Update 2020. Irish Wildlife Manuals, No. 123. Dublin, Ireland: National Parks and Wildlife Service, Department of Culture, Heritage and the Gaeltacht; 2020. [Google Scholar]
19. Sabovljević MS, Pantović JP, Širka P, Vujičić MM, Sabovljević AD, Papp B. Red-list of moss species of Serbia: 2024 assessment. Bot Serb. 2024;48(2):207–22. doi:10.2298/BOTSERB2402207S. [Google Scholar] [CrossRef]
20. Martinčič A. New checklist and the red list of the mosses (Bryophyta) of Slovenia. Hacquetia. 2024;23(1):69–118. doi:10.2478/hacq-2023-0006. [Google Scholar] [CrossRef]
21. Stešević D, Andjić B, Stanišić-Vujačić M. Physcomitrium eurystomum Sendt., a new moss species in the bryophyte flora of Montenegro. Acta Bot Croat. 2020;79(1):95–7. doi:10.37427/botcro-2020-004. [Google Scholar] [CrossRef]
22. Reski R, Abel WO. Induction of budding on chloronemata and caulonemata of the moss Physcomitrella patens, using isopentenyladenine. Planta. 1985;165(3):354–8. doi:10.1007/BF00392232 [Google Scholar] [PubMed] [CrossRef]
23. R Core Team. A language and environment for statistical computing. Vienna, Austria: R Foundation for Statistical Computing; 2024. Available from: https://www.R-project.org/. [Accessed 2024]. [Google Scholar]
24. Agresti A. An introduction to categorical data analysis. 2nd edHoboken, NJ, USA: Wiley; 2007. [Google Scholar]
25. Carvalho F, Santana D, Araujo L. Why analyze germination experiments using generalized linear models? J Seed Sci. 2018;40(3):281–7. doi:10.1590/2317-1545v40n3185259. [Google Scholar] [CrossRef]
26. Longton RE. Reproduction and rarity in British mosses. Biol Conserv. 1992;59:89–98. [Google Scholar]
27. Eppley SM, Taylor PJ, Jesson LK. Self-fertilization in mosses: a comparison of heterozygote deficiency between species with combined versus separate sexes. Heredity. 2007;98:38–44 [Google Scholar] [PubMed]
28. Van Zuijlen K, Nobis MP, Hedenäs L, Hodgetts N, Calleja Alarcón JA, Albertos B, et al. Bryophytes of Europe Traits (BET) data set: a fundamental tool for ecological studies. J Veg Sci. 2023;34(2):e13179. [Google Scholar]
29. Ducker BFT, Warburg EF. Physcomitrium eurystomum Sendtn. Britain J Bryol. 1961;4(1):95–7. [Google Scholar]
30. Jesson LK, Cavanagh AP, Perley DS. Polyploidy influences sexual system and mating patterns in the moss Atrichum undulatum sensu lato. Ann Bot. 2011;107:135–43 [Google Scholar] [PubMed]
31. Perroud PF, Cove DJ, Quatrano RS, McDaniel SF. An experimental method to facilitate the identification of hybrid sporophytes in the moss Physcomitrella patens using fluorescent tagged lines. New Phytol. 2011;191:301–6. doi:10.1111/j.1469-8137.2011.03668.x [Google Scholar] [PubMed] [CrossRef]
32. Vesty EF, Saidi Y, Moody LA, Holloway D, Whitbread A, Needs S, et al. The decision to germinate is regulated by divergent molecular networks in spores and seeds. New Phytol. 2016;211(3):952–66. doi:10.1111/nph.14018 [Google Scholar] [PubMed] [CrossRef]
33. Vesty EF, Whitbread AL, Needs S, Tanko W, Jones K, Halliday N, et al. Cross-kingdom signalling regulates spore germination in the moss Physcomitrella patens. Sci Rep. 2020;10:2614 [Google Scholar] [PubMed]
34. Phokas A, Meyberg R, Briones-Moreno A, Hernandez-Garcia J, Wadsworth PT, Vesty EF, et al. DELLA proteins regulate spore germination and reproductive development in Physcomitrium patens. New Phytol. 2023;238:654–72. doi:10.1111/nph.18756a. [Google Scholar] [CrossRef]
35. Genty B, Briantais J, Baker NR. The relationship between the quantum yield of photosynthetic electron transport and quenching of chlorophyll fluorescence. BBA-General Subjects. 1989;990(1):87–92. doi:10.1016/S0304-4165(89)80016-9. [Google Scholar] [CrossRef]
36. Pettinari G, Finello J, Plaza Rojas M, Liberatore F, Robert G, Otaiza-González S, et al. Autophagy modulates growth and development in the moss Physcomitrium patens. Front Plant Sci. 2022;13:1052358. doi:10.3389/fpls.2022.1052358 [Google Scholar] [PubMed] [CrossRef]
37. Krieg CP, Gosetti S, Watkins JEJr, Griffith MP, McCulloh KA. Reproductive phases coincide with changes in morphology and photosynthetic physiology in an endangered cycad species. Conserv Physiol. 2023;11(1). doi:10.1093/conphys/coad020 [Google Scholar] [PubMed] [CrossRef]
38. Raven JA, Griffiths H. Photosynthesis in reproductive structures: costs and benefits. J Exp Bot. 2015;66(7):1699–705. doi:10.1093/jxb/erv009 [Google Scholar] [PubMed] [CrossRef]
39. Obeso JR. The costs of reproduction in plants. New Phytol. 2002;155:321–48. doi:10.1046/j.1469-8137.2002.00477.x [Google Scholar] [PubMed] [CrossRef]
40. Laporte MM, Delph LF. Sex-specific physiology and source-sink relations in the dioecious plant Silene latifolia. Oecologia. 1996;106(1):63–72. doi:10.1007/BF00334408 [Google Scholar] [PubMed] [CrossRef]
Cite This Article
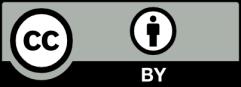
This work is licensed under a Creative Commons Attribution 4.0 International License , which permits unrestricted use, distribution, and reproduction in any medium, provided the original work is properly cited.