Open Access
ARTICLE
EMS-Mediated Mutagenesis in Marigold Seeds and Its Effects on Seedling Growth and Physiology
1 Institute of Flower, Yunnan Academy of Agricultural Sciences, Yunnan Key Laboratory for Flower Breeding, National Engineering Research Center for Ornamental Horticulture, Yunnan Provincial Key Laboratory of Flower Genetic Improvement, Kunming, 650205, China
2 College of Horticulture, Northwest A&F University, Yangling, 712100, China
3 West Yunnan University, Lincang, 67700, China
4 School of Biotechnology, Centurion University of Technology and Management, Bhubaneswar, 752050, India
5 Plant Production Department, College of Food and Agriculture Sciences, King Saud University, Riyadh, 11451, Saudi Arabia
* Corresponding Authors: Yalian Jiang. Email: ; Wangqi Huang. Email:
# These authors contributed equally to this work
Phyton-International Journal of Experimental Botany 2024, 93(11), 3029-3038. https://doi.org/10.32604/phyton.2024.057857
Received 29 August 2024; Accepted 23 October 2024; Issue published 30 November 2024
Abstract
Marigolds (Tagetes spp.) are popular horticultural plants worldwide. The current study aimed to investigate the optimal mutagenic conditions for marigold seeds using EMS (ethyl methanesulfonate) mutagenesis. Different concentrations and treatment times of EMS were applied to investigate their effects on the marigold seed germination rate, growth traits, antioxidant enzyme activities (i.e., SOD and POD), and malondialdehyde (MDA) contents. Results indicated that with increasing the EMS treatment duration and concentration, the seed germination rate and growth treatments were reduced, accompanied by elevated MDA content. In addition, SOD and POD activities initially correlated positively with the growth tratis at the lowest concentrations and shortest durations of EMS, but such relationship diminished beyond certain thresholds. The comprehensive analysis identified the optimal mutagenic conditions as 1% EMS treatment for 12 h, achieving a semi-lethal dose and enhancing stress-resistant components in seedlings. These findings are pivotal for advancing genetic enhancement and germplasm innovation in marigolds.Keywords
Mutagenesis is a breeding method that uses physical and chemical factors under artificial conditions to alter the genetic material of a species, enabling the selection and cultivation of new varieties [1]. Chemical mutagenesis is considered advantageous due to its low cost, simplicity, and stable effects. Compared to other breeding methods, it can be quickly and efficiently to address the problem of limited germplasm resources and enhance germplasm diversity [2]. In addition, the point mutations caused by these mutagens make them suitable enough to result in functional mutations [3]. Ethyl methanesulfonate (EMS) is an alkylating chemical mutagen that has diverse effects on host DNA [4]. The mutation produced by EMS is mainly due to the alkylation of the guanine bases, resulting in the transitions of G/C to A/T and transversions of G/C to T/A [5,6]. However, the efficiency of EMS in causing mutagenesis varies across different plant species [7]. For instance, the mutagenesis effects caused by EMS treatment in Arabidopsis, maize, sugarcane, and wheat varied from one another [5,8,9]. EMS mutagenesis technology has been employed successfully in constructing mutant libraries and selecting various plants, including agave, wheat and birch [10]. EMS mutagenesis is frequently studied to determine its lethal dose (LD50), indirectly identifying the optimal dose for inducing plant mutations. This method leverages the toxic effects of EMS on plants, with lethality varying based on concentration and treatment duration [10]. Under stressful conditions, including mutagenesis, plants can exhibit increased activities of antioxidant enzymes such as superoxide dismutase (SOD) and peroxidase (POD) [11,12]. Thus, the extent of these antioxidant enzymes provides essential insights into the plant responses against a particular stimulus.
Marigold, an annual herbaceous plant from the Asteraceae family, possesses medicinal properties such as clearing heat and detoxifying, resolving phlegm, and relieving cough [13]. Its petals are rich in lutein, making it an ideal source for lutein extraction [14,15]. Lutein content is a crucial evaluation index for marigolds. EMS mutagenesis can significantly broaden the gene pool of marigolds, enhancing genetic diversity and providing a more extensive range of germplasm resources for long-term varietal improvement and innovation. In Yunnan, China, the primary cultivation area for marigolds often faces drought conditions. EMS mutagenesis could induce varieties with improved drought adaptability, enhancing planting adaptability and yield stability.
This study used EMS as a mutagen to treat mature marigold seeds. We investigated the effects of different EMS concentrations and treatment durations on seed germination, seedling growth, and development. Additionally, we aimed to determine the optimal EMS treatment time and concentration for mutagenesis in marigolds. The outcomes of this study lay the foundation for creating marigold germplasm resources and selecting superior varieties.
The experiment was conducted at the Institute of Flowers, Yunnan Academy of Agricultural Sciences, Yunnan, China. Healthy and high-quality seeds of marigolds, sourced from Yunnan Bohao Biotechnology Co., Kunming, China, were used for the mutagenic treatments. EMS (ethyl methanesulfonate), the mutagen employed in this study, was procured from Sigma-Aldrich, Saint Louis, MO, USA.
Whole marigold seeds were moistened with distilled water at 28°C for 12 h. Subsequently, the seeds were treated with EMS mutagen according to the method of He et al. [16]. To prepare the EMS solutions, concentrations of 0%, 0.5%, 1%, and 1.5% were made using 0.1 mol/L phosphate buffer at pH 7. The treatment durations were set at 6, 12, 18, and 24 h. The control treatment involved using the phosphate buffer without EMS. Pre-treated seeds, 100 per replication, were transferred to the different concentrations of EMS mutagenic solution and subjected to agitation on a shaker at 140 rpm while maintaining a temperature of 25 ± 1°C. Following the treatment, the seeds were enclosed in gauze, rinsed under tap water for 2 h, drained, and sown in seedling trays. The seedling trays were placed under 14 h of light per day at a temperature of 28 ± 1°C. The soil in the trays was kept moist throughout this period.
2.3 Indicator Measurement Determination of Seed Germination
We monitored seed germination by assessing bud break as the standard indicator. Seed germination counts were conducted at fixed intervals each day. Germination was considered complete when no further changes were observed in the number of germinated seeds for three consecutive days. The seed germination rate (%) was calculated as the number of seeds germinating within seven days after treatment divided by the total number of seeds used. The relative germination rate (%) was determined by dividing the number of seeds germinated in the experimental group by the number of seeds in the control group.
2.4 Determination of Morphological Indexes
The mutagenized seeds were cultivated until the ‘four leaves and one heart stage’. Measurements were then taken for plant height, root length, fresh weight, and dry weight. Five plants were randomly selected from each treatment group for these measurements.
2.5 Determination of Physiological Indexes
The mutagenized seeds were cultivated until they reached the ‘four leaves and one heart’ stage. At this stage, measurements were taken for malondialdehyde (MDA) content, chlorophyll content, peroxidase (POD) activity, superoxide dismutase (SOD) activity, and root vigor [17]. Five plants per treatment group were randomly selected for these determinations.
The data obtained were subjected to statistical analysis using STATA statistical software version 14 (StataCorp, 2015). Excel was employed to compute the germination percentage, speed, and number of seeds per plant. Graphical plots were generated using Origin software.
3.1 Effect of Different Treatments on Germination of Marigolds
The different treatment times and EMS concentrations significantly influenced the germination rate and relative germination rate of marigolds, as shown in (Fig. 1). Our findings revealed that, under non-blank control conditions, an increase in treatment time and EMS concentration led to a gradual decrease in germination and relative germination rates. The blank control condition exhibited the highest germination rate, with no significant differences between treatment times. At the same treatment time, the germination rate varied significantly across different EMS concentrations, consistently decreasing as EMS concentration increased. The highest relative germination rate of approximately 90% was observed when seeds were treated with 0.5% EMS for 6 h, indicating minimal impact on germination. Conversely, the lowest relative germination rate was observed when seeds were treated with 1.5% EMS for 12 h. When treated with 1% EMS for 12 h or 0.5% EMS for 4 h, the relative germination rate was approximately 50%. This rate was comparable to that of other treatments at 4 h. Therefore, a relative germination rate of about 50%, achieved by treating marigold seeds with 1% EMS for 12 h or 0.5% EMS for 4 h, can be considered the semi-lethal dose.
Figure 1: Effects of different EMS treatments on the germination of marigolds
3.2 Effects of Different Treatments on the Growth of Marigolds
3.2.1 Effect of EMS Treatment on Plant Height
Based on Fig. 2, it is clear that the plant height of marigold seeds treated with EMS gradually decreased as the treatment time increased, with the same EMS concentration. The plant height decreased further with higher EMS concentrations, indicating a significant inhibition of plant height in marigold plants by EMS. Moreover, the plant height also reduced as the treatment time increased in the blank control without EMS. Even in the blank control, the plant height decreased over time. In all treatments, the plant height differed significantly from that of the blank control, and there were also significant differences between the same EMS treatment concentrations. Therefore, the inhibition of plant height in marigolds increased with longer treatment times and higher concentrations, reaching the maximum inhibition rate after a 24-h treatment with 1.5% EMS (Fig. 2).
Figure 2: Effect of different EMS treatments on plant height and root length of marigold seedlings
3.2.2 Effect of EMS Treatment on Root Length
The root length of marigold seeds treated with EMS gradually decreased as the treatment time increased at the same EMS concentration. Similarly, at the same treatment time, higher EMS concentrations resulted in shorter root lengths, clearly reflecting the inhibitory effect of EMS on the root length of marigolds. In the blank control without EMS, the differences in root length between treatments were small and insignificant. The treatments evaluated with EMS, only the 0.5% and 1% treatments for 6 h were not significantly different from the blank control, while the rest were significantly different. This demonstrates that the inhibition of root growth in marigolds increased with the prolongation of treatment time and the increase in treatment concentration, reaching its maximum inhibition rate at 12 h of 1.5% EMS treatment (Fig. 3).
Figure 3: Effect of different EMS treatments on marigold seedlings
3.2.3 Effect of EMS Treatment on Fresh Weight
The overall performance of marigold seeds treated with EMS at the same concentration tends to decrease gradually with increased treatment time. While the differences between some treatments may not be significant, the data reflect a general inhibitory effect of EMS on the fresh weight of marigolds. In the blank control without EMS, the fresh weight was significantly lower than at other treatment times once the duration reached 24 h. Across all experimental treatments, there was a significant difference compared to the blank control. This indicates that the inhibition of fresh weight in marigolds increases significantly with longer treatment times and higher EMS concentrations, reaching a maximum inhibition rate at 12 h of 1.5% EMS treatment (Fig. 4).
Figure 4: Effects of different EMS treatments on fresh weight and chlorophyll content of marigold seedlings
3.2.4 Effect of EMS on Chlorophyll Content
Fig. 4 shows that the chlorophyll content of marigold seeds remained stable across different EMS concentrations and treatment times, with no significant differences observed between treatments. These results indicate that EMS treatment did not significantly inhibit the chlorophyll content of marigold seedlings.
3.3 Effects of Different Treatments on Antioxidant Enzyme Activities of Marigolds
As shown in Fig. 5, at an EMS concentration of 0% and treatment times below 12 h, POD activity increased as treatment duration increased. Upon reaching 12 h, POD activity stabilized. At 0.5% EMS concentration, there was minimal overall change in POD activity with increasing treatment time. For the 1% EMS concentration, POD activity was initially increased with treatment duration, reaching its peak at 12 h, after which it gradually declined. Conversely, at 1.5% EMS concentration, POD activity decreased steadily with increasing treatment time, reaching its lowest level after 24 h.
Figure 5: Effect of different EMS treatments on POD and SOD activities of marigold seedlings
At an EMS concentration of 0% (i.e., blank), the SOD activity of marigold seedlings showed a slight increase with longer treatment times, although this change was minimal and not statistically significant. When the EMS concentration was 0.5%, SOD activity increased steadily with treatment time, peaking at 18 h, with no significant difference between 12 and 18 h, followed by a decline after 18 h. At 1% EMS concentration, SOD activity increased up to 12 h of treatment and subsequently started to decrease. Conversely, at an EMS concentration of 1.5%, SOD activity decreased progressively with longer treatment times, reaching its lowest point at 24 h (Fig. 5).
The MDA content remained low at an EMS concentration of 0% but increased with longer treatment times. When the EMS concentration was 0.5%, the MDA content increased with treatment time, showing significant differences from the control. At EMS concentrations of 1% and 1.5%, the MDA content of the seedlings increased over time; however, the changes were similar and not significantly different. These results indicate that % EMS concentration of 1% effectively inhibits marigold growth (Fig. 6).
Figure 6: Effects of different EMS treatments on MDA content and root vigor of marigold seedlings
3.4 Effect of EMS on Root Vigor
At an EMS concentration of 0%, root vigor in marigold seedlings started high but decreased throughout the treatment period. At 0.5% EMS concentration, root vigor decreased with longer treatment durations, showing significant differences from the blank control. For 1% EMS concentration, root vigor decreased up to 12 h of treatment, after which no significant differences were observed. Similarly, at 1.5% EMS concentration, root vigor remained consistently lower and stable across different treatment times (Fig. 6).
EMS mutagenesis is a well-established plant breeding method for inducing point mutations that generate genetic variation. This study focuses on the effects of EMS on seed germination indices and overall physiology. EMS treatment significantly impacts seed germination rates, germination potential, root length, and shoot length. Ntuli et al. found that high EMS concentrations significantly reduced the germination rate of drunken grass seeds, while lower concentrations could promote germination [18]. They identified that a 3% EMS treatment for 48 h was closest to the semi-lethal condition. Similarly, Feng’s study on surimi seeds showed that increasing EMS concentration and treatment duration inhibited seed germination and growth, with 0.9% EMS for 10 h or 0.6% EMS for 14 h optimal for creating a mutant library [19]. Our findings align with these studies, showing a decrease in germination rate with increased treatment time and concentration, similar to results observed by Cheng et al. in bitter melon [20]. This indicates that EMS mutagenesis effects on seed germination are dose-and time-dependent, with different combinations yielding varying biological effects.
Reyes-Zambrano et al. [21] reported that the use of ethyl methanesulfonate induced variations in the morphometric and morphological parameters of the seedlings obtained from Agave americana L., where 60% of the seedlings presented dwarfism and different foliar forms, with a lack of spines. Also, an increase in the fructan content of 30% with respect to the seedlings in the control treatment could be observed, along with an increase in PAL activity.
Plants subjected to external stress typically show reduced growth indices, such as plant height and fresh weight, while enzymes like superoxide dismutase (SOD) and peroxidase (POD) increase under stress. Our study found that longer treatment times and higher EMS concentrations decreased growth indices. SOD and POD activities were positively correlated with lower EMS concentrations and shorter treatment times but decreased at higher concentrations and longer durations. Malondialdehyde (MDA) content increased with longer treatment times and higher concentrations but decreased when stress exceeded the plant’s tolerance limit. These results are consistent with Shang et al.’s findings in tomatoes [22]. EMS as a mutagen has been extensively explored to develop desirable plant traits across various species. For instance, Wani et al. found that mung bean seeds treated with low and medium EMS concentrations significantly increased pod length, number of seeds per pod, and grain weight in the M2 generation [23]. Shahwar et al. demonstrated that treating lentil seeds with EMS resulted in a new genotype with increased pod number and size and stable genetic traits after self-crossing [24].
Currently, mutagenesis represents an effective biotechnological tool to be used in genetic improvement programs, this technique becomes more relevant when it is applied to species of agronomic importance such as those of the Agave genus, which are characterized by having little genetic variation and slow natural regenerative capacity.
These studies indicate that EMS mutagenesis and environmental stress can effectively be used as a screen for mutants with desirable traits. The construction of mutant libraries can support the beneficial genetic variations and provide the materials for functional genomics studies. For example, Cao et al. reported that EMS mutagenesis significantly increased mutant production in wheat plants, offering valuable resources for functional genomics research. However, EMS mutagenesis can have adverse effects if used in high concentrations or applied at unsuitable durations. For instance, Cao et al. reported that high EMS concentrations can significantly inhibit the growth of embryonic roots and axes in tomato seedlings [25]. Therefore, it is crucial to be careful and consider the mutagenesis conditions for balancing the generation of variation with the potential adverse effects on plant growth and its development.
With increasing EMS concentrations and durations, seed germination rates and growth traits of marigolds were reduced accompanied by elevated MDA content. SOD and POD activities initially correlated positively with growth indicators at lower concentrations and shorter durations, but this relationship diminished beyond certain thresholds in comparison to blank treatment. The application of EMS at 1% EMS for 12 h emerged as the semi-lethal dose, balancing mutagenesis with seedling viability and enhancing resistance component levels. This half-lethal dose serves as a reliable measure of mutagen impact, minimizing excessive damage and supporting future research and breeding efforts. The physiological indices examined further validate the LC50 findings, guiding selection processes.
Acknowledgement: We are very grateful to Yunnan Provincial Department of Science and Technology and Yunnan Provincial Key Laboratory of Flower Breeding for supporting this study. The authors also extend their appreciation to the Researchers Supporting Project number (RSPD2025R751), King Saud University, Riyadh, Saudi Arabia.
Funding Statement: This work was supported by Yunnan Provincial Department of Science and Technology Key R&D Plan (202303AM140018, 202303AK140029, 202303AK140028), Yunnan Flower Breeding Key Experiment Open Foundation (FKL-202203), Yunnan Provincial Department of Science and Technology Science and Technology Project Agriculture Joint Foundation (202301BD070001-208), Yunnan Provincial Expert Basic Research Workstation Foundation. We also acknowledge the financial support from the Researchers Supporting Project (RSPD2025R751), King Saud University, Riyadh, Saudi Arabia.
Author Contributions: Study conception and design: Wangqi Huang, Ikram Ullah and Chao Meng; data collection: Chao Meng, Wenjin Wu, Ruixue Shi and Shaodan Luo; analysis and interpretation of results: Chao Meng, Ikram Ullah, Cuixia Luo, Yalian Jiang and Yiping Zhang; draft manuscript preparation: Ikram Ullah, Satyabrata Nanda, Wangqi Huang and Mahmoud F. Seleiman; supervision: Wangqi Huang and Yalian Jiang; project administration: Wangqi Huang. All authors reviewed the results and approved the final version of the manuscript.
Availability of Data and Materials: The datasets generated and analyzed during the current study are available from the corresponding author upon reasonable request.
Ethics Approval: Not applicable.
Conflicts of Interest: The authors declare that they have no conflicts of interest to report regarding the present study.
References
1. Ali S, Suryakant TN. Mutation breeding and its importance in modern plant breeding: a review. J Exper Agric Inter. 2024;46(7):264–75. doi:10.9734/jeai/2024/v46i72581. [Google Scholar] [CrossRef]
2. Animasaun DA, Oguntoye EO. Mutagenesis in crop improvement: methods and applications. J Crop Improv. 2024;38(3):156–78. doi:10.1080/15427528.2024.2336257. [Google Scholar] [CrossRef]
3. Shelar A, Singh AV, Maharjan RS, Laux P, Luch A, Gemmati D, et al. Sustainable agriculture through multidisciplinary seed nanopriming: prospects of opportunities and challenges. Cells. 2021;10(9):2428. doi:10.3390/cells10092428 [Google Scholar] [PubMed] [CrossRef]
4. Ramesh M, Vanniarajan C, Ravikesavan, Eraivan-Arutkani AK, Mahendran PP. Determination of lethal dose and effect of EMS and gamma ray on germination percentage and seedling parameters in barnyard millet variety Co(Kv)2. Elect J Plant Breed. 2019;10(2):957–62. doi:10.5958/0975-928X.2019.00123.6. [Google Scholar] [CrossRef]
5. Talebi AB, Talebi AB, Shahrokhifar B. Ethyl methane sulphonate (EMS) induced mutagenesis in Malaysian rice (cv. MR219) for lethal dose determination. Amer J Plant Sci. 2012;3(12):1661–5. doi:10.4236/ajps.2012.312202. [Google Scholar] [CrossRef]
6. Serrat X, Esteban R, Guibourt N, Moysset L, Nogués S, Lalanne E. EMS mutagenesis in mature seed-derived rice calli as a new method for rapidly obtaining TILLING mutant populations. Plant Meth. 2014;10(1):5. doi:10.1186/1746-4811-10-5 [Google Scholar] [PubMed] [CrossRef]
7. Teh SL, Fresnedo-Ramírez J, Clark MD, Gadoury DM, Sun Q, Cadle-Davidson L, et al. Genetic dissection of powdery mildew resistance in interspecific half-sib grapevine families using SNP-based maps. Molec Breed. 2017;37:1. doi:10.1007/s11032-016-0586-4 [Google Scholar] [PubMed] [CrossRef]
8. Li J, Jiang Y, Zhang J, Ni Y, Jiao Z, Li H, et al. Key auxin response factor (ARF) genes constraining wheat tillering of mutant dmc. PeerJ. 2021;9:e12221. doi:10.7717/peerj.12221 [Google Scholar] [PubMed] [CrossRef]
9. Gadakh SS, Patel DU, Narwade A, Singh D. Screening of EMS induced drought tolerant sugarcane (Saccharum spp. Complex) mutants employing physiological, molecular and biochemical approaches. Ind J Gen Plant Breed. 2021;81(4):575–81. doi:10.31742/IJGPB.81.4.10. [Google Scholar] [CrossRef]
10. Maluszynski MSM. Induced mutations and biotechnology in improving crops. In: In vitro application in crop improvement. Boca Raton, FL, USA: CRC Press; 2004. p. 187–220. [Google Scholar]
11. Ahmad R, Hussain S, Anjum MA, Khalid MF, Saqib M, Zakir I, et al. Oxidative stress and antioxidant defense mechanisms in plants under salt stress. In: Plant abiotic stress tolerance. Cham: Springer; 2019. p. 191–205. doi:10.1007/978-3-030-06118-0_8. [Google Scholar] [CrossRef]
12. Ahmad P, Jaleel CA, Salem MA, Nabi G, Sharma S. Roles of enzymatic and nonenzymatic antioxidants in plants during abiotic stress. Crit Rev Biotech. 2010;30(3):161–75. doi:10.3109/07388550903524243 [Google Scholar] [PubMed] [CrossRef]
13. McIntyre A. Herbal treatment of children: Western and Ayurvedic perspectives. Elsev Health Sci. 2005. doi:10.1016/B978-0-7506-5174-5.X5001-4. [Google Scholar] [CrossRef]
14. Manzoor S, Rashid R, Panda BP, Sharma V, Azhar M. Green extraction of lutein from marigold flower petals, process optimization and its potential to improve the oxidative stability of sunflower oil. Ultr Sonochem. 2022;85(6):105994. doi:10.1016/j.ultsonch.2022.105994 [Google Scholar] [PubMed] [CrossRef]
15. Muhammad G, Butler TO, Chen B, Lv Y, Xiong W, Zhao X, et al. Sustainable production of lutein—an underexplored commercially relevant pigment from microalgae. Bio Conv Bioref. 2024;14(6):7255–76. doi:10.1007/s13399-022-03349-5. [Google Scholar] [CrossRef]
16. He J, Wen H, Yin H, Li R, Wu X, Liu H. EMS mutagenesis in vitro and mutant screening of Echeveria agavoides ‘Corderoyi’. J Yunnan Univ (Nat Sci Ed). 2020;42(1):187–93 (In Chinese). [Google Scholar]
17. Jia P. Varietal features of development and performance of Brassica Juncea L. according to growth regulators in terms of the Forest-Steppe of Ukraine (Ph.D. Thesis). Sumy National Agrarian University: Ukraine; 2022. Available from: https://repo.snau.edu.ua:8080/xmlui/handle/123456789/10192. [Accessed 2024]. [Google Scholar]
18. Ntuli NR. Genetic improvement of selected indigenous Cucurbitaceae species important for food and medicinal purposes in KwaZulu-Natal. University of Zululand: South Africa; 2007. Available from: https://hdl.handle.net/10530/869. [Accessed 2024]. [Google Scholar]
19. Zhang Z, Zhang J, Lu P, Wu B, Liu M, Gao J, et al. Six underutilized grain crops for food and nutrition in China. Plants. 2022;11(19):2451. doi:10.3390/plants11192451 [Google Scholar] [PubMed] [CrossRef]
20. Cheng JW, Zhong J, Cheng YR, Hu F, Cui JJ, Hu KL. Analysis of EMS mutagenesis condition for bitter gourd (Momordica charantia L.) seed. China Veg. 2019;1(1):26–31 (In Chinese). [Google Scholar]
21. Reyes-Zambrano SJ, Ramírez-Merchant ML, Arias-Castro C, Rodríguez-Mendiola MA, Lecona-Guzmán CA, Ruíz-Valdiviezo VM, et al. Morphometric and biochemical changes in Agave americana L. plantlets induced by ethyl methanesulfonate. Phyton-Int J Exp Bot. 2019;88(3):277–84. doi:10.32604/phyton.2019.06504. [Google Scholar] [CrossRef]
22. Shang J, Zhang H, Wang S, Wang X, Jiang S, Zha D, et al. Effects of EMS mutagenesis on seed germination, seedling growth and antioxidant system of eggplant (Solanum melongena). Acta Agric Jiangxi. 2020;32(2):33–7 (In Chinese). doi:10.19386/j.cnki.jxnyxb.2020.02.06. [Google Scholar] [CrossRef]
23. Wani M, Dar A, Tak A, Amin I, Shah N, Rehman R, et al. Chemo-induced pod and seed mutants in mungbean (Vigna Radiata L. Wilczek). SAARC J Agric. 2018;15(2):57–67. doi:10.3329/sja.v15i2.35161. [Google Scholar] [CrossRef]
24. Shahwar D, Ansari M, Khatoon B, Park Y. Phenotypic characterization of ethyl methanesulfonate (EMS)-induced bigger pod (bp) with multiple seed mutants in lentil (Lens culinaris). Biocat Agricult Biotech. 2024;59(2):103259. doi:10.1016/j.bcab.2024.103259. [Google Scholar] [CrossRef]
25. Cao Y, Wu Y, Liu B, Ji J, Yuan C, Shiping L, et al. Creation and ldentification of Jimai 22 mutants with drought-resistant and water-saving features induced by EMS. J Wheat Crops. 2024;44(3):315–22. [Google Scholar]
Cite This Article
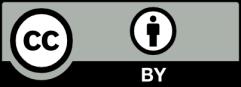
This work is licensed under a Creative Commons Attribution 4.0 International License , which permits unrestricted use, distribution, and reproduction in any medium, provided the original work is properly cited.