Open Access
ARTICLE
The Integration of Water and Fertilizer Regulated Soil Nutrients and Enzyme Activities of Greenhouse Tomato (Solanum lycopersicum) by Moistube Irrigation
1 Faculty of Engineering, Huanghe Science and Technology University, Zhengzhou, 450063, China
2 Northwest Land and Resources Research Center, Shaanxi Normal University, Xi’an, 710119, China
* Corresponding Author: Mingzhi Zhang. Email:
Phyton-International Journal of Experimental Botany 2024, 93(11), 2813-2836. https://doi.org/10.32604/phyton.2024.057795
Received 27 August 2024; Accepted 14 October 2024; Issue published 30 November 2024
Abstract
Moistube irrigation was driven by the gradient of water potential inside and outside the pipe wall, which has the advantages of continuous irrigation during the crop growth period. However, the response mechanism of soil nutrients and enzyme activities of greenhouse tomatoes under the integration of water and fertilizer by moistube irrigation is still unclear. In order to explore the changes in soil nutrients and enzyme activities of greenhouse tomatoes regulated by irrigation and fertilization in moistube irrigation greenhouse, a completely randomized experimental design was conducted to explore the effects of different irrigation amounts (I, controlling the working pressure head of moistube to achieve different irrigation amount gradients, capillary working pressure was 1 (I1), 2 (I2), 3 (I3) m) and fertilization amounts (F, single fertilization amount was 100 (F1), 200 (F2), 300 (F3) kg/hm2) on soil nutrients, enzyme activities and yield of greenhouse tomato. It was found that with the increase of I, the total organic carbon content (TOC), soil β-glucosidase (BG), leucine amino peptidase (LAP), N-acetylglucosaminidase (NAG), and alkaline phosphatase (AP) activities enzyme of greenhouse tomato soil increased first and then decreased. When the I increased from I1 to I3, the soil total nitrogen (TN) decreased by 5.07% and 4.91%, respectively, and the soil total phosphorus (TP) by 4.37% and 4.22%, respectively. With the increase of fertilizer amounts, the TOC of tomato soil increased first and then decreased, the activities of BG, LAP, NAG and AP in soil increased first and then decreased, and the contents of TN and TP in soil decreased by 4.79% and 4.68%, 12.32% and 10.47%, respectively. The yield of tomatoes treated with I2 was significantly higher than that of I1 and I3 by about 13.99% and 1.29%, respectively. The tomato yield of F2 treatment was significantly higher than that of F1 and F3 treatments by about 22.57% and 1.72%, respectively. Based on the analysis of stoichiometry, it was found that soil carbon was more scarcity than nitrogen in greenhouse tomatoes under the integration of water and fertilizer by moistube irrigation. Soil carbon, nitrogen, phosphorus, enzyme activity and their respective stoichiometry showed a quadratic curve relationship with yield. Therefore, I2F2 treatment of greenhouse tomatoes can not only reduce soil carbon and nitrogen limitations but also improve soil enzyme activity and achieve multiple goals of increasing greenhouse tomato yield, and saving water and fertilizer. This conclusion contributes to a more reasonable irrigation and fertilization of tomatoes in moistube irrigation greenhouse and soil nutrient management of facility agriculture through empirical proofs.Keywords
Glossary/Nomenclature/Abbreviations
MI | Moistube Irrigation |
I | Irrigation Amount |
F | Fertilization Amount |
TOC | Total Organic Carbon Content |
TN | Total Nitrogen |
TP | Total Phosphorus |
C | Total Organic Carbon Content |
N | Total Nitrogen |
P | Total Phosphorus |
BG | Soil β-Glucosidase |
LAP | Leucine Amino Peptidase |
NAG | N-Acetylglucosaminidase |
AP | Alkaline Phosphatase |
Y | Yield |
As one of the important vegetable crops in China, facility tomato, of which industry’s development is significant for ensuring national food security and promoting farmers’ income. Meanwhile, tomato has become a very popular crop in facility agriculture because of its high nutritional value [1]. The irrigation water of facility agricultural tomatoes in Huang-Huai-Hai Plain mainly depends on groundwater, which aggravates the shortage of water resources in this area [2,3]. At the same time, most of the nutrients required for the growth of tomatoes in facility agriculture are provided by fertilization, and unscientific fertilization methods will bring negative effects such as soil degradation, salinization and greenhouse gas emissions [4]. It has become the focus of current research to explore more advanced irrigation methods to formulate reasonable irrigation and fertilization schemes, and thus to achieve the increase of tomato yield and quality while reducing the impact on the environment and financial burden of farmers. Therefore, the study of water and fertilizer integration technology is significant for the sustainable development of the tomato industry.
Water and fertilizer integration technology plays an important role in achieving precise irrigation and fertilization of crops. It determines how water and fertilizer effectively enter the soil of the crop root zone, which is the key to regulating the optimal equilibrium of irrigation water and fertilizer amount [5,6]. The best balance between irrigation and fertilization in the integrated technology of water and fertilizer in facility agriculture is important in saving water, increasing yield and improving fruit quality [7]. Water and fertilizer integration technology can easily affect the nutrient turnover between crop roots and soil, and directly or indirectly affect soil carbon, nitrogen and phosphorus, soil enzyme activity and their respective stoichiometric characteristics [8,9]. Studies have shown that different irrigation and fertilization treatments can change the soil enzyme activity, nutrient distribution and the growth status of various organs of crops in facility agriculture and then affect the nutrient cycle process and balance characteristics of facility agriculture micro-system [10–12]. In the practice of production, farmers choose different water and fertilizer integration models for different crops based on long-term planting experience. At present, drip irrigation technology has been widely used in facility agriculture. For example, different combinations of drip irrigation and fertilization can change the soil enzyme activity in the root zone of crops [13,14]; in the Mediterranean region of Morocco, drip irrigation water and fertilizer integration increases crop yields and improves soil quality [15]; appropriate water and fertilizer coupling under mulched drip irrigation can increase the yield of kiwifruit, cottonseed and panax notoginseng in agricultural areas of China [16–19].
Moistube irrigation represents an innovative polymer semi-membrane technology characterized by the presence of 100,000 nanopore micropores, each measuring between 10 to 900 nanometers, distributed across every square centimeter of its wall. This system utilizes the water potential gradient between the interior and exterior of the membrane as the driving force for its operation, classifying it as a sustainable, line-source infiltration irrigation technique aimed at water conservation. During the crop growth period, Moistube irrigation facilitates a continuous and timely water supply throughout the entire growth cycle, thereby enhancing the soil’s water and gas environments. This method effectively minimizes surface evaporation and optimizes the utilization of both soil water and fertilizers [20,21]. It was discovered that the yield and water use efficiency of tomatoes and winter wheat under moistube irrigation outperformed those under drip irrigation within the Loess Plateau [22,23]. Currently, moistube irrigation has seen widespread implementation in various fields, including greening, food crops, and vegetable cultivation [23–25]. The predominant focus of research has been on assessing the impact of alterations in parameters such as the embedded depth of capillaries, spacing, and operational pressure in moistube irrigation on crop growth and soil moisture distribution [22,26,27]. Nevertheless, there is a scarcity of studies examining the integrated technology of moistube irrigation combined with fertilization. Investigating this integrated approach is crucial for enhancing the comprehensive technological framework of water and fertilizer management, as well as for expanding the application scope of moistube irrigation technology.
Currently, the response mechanism of soil nutrients and enzyme activities within the crop root zone, as influenced by the integration of moistube irrigation and fertilization, remains unclear. The stoichiometric relationships involving energy, material cycling, and ecological chemical elements—alongside the quality of environmental elements—within facility agriculture utilizing moistube irrigation and fertilizer integration have rarely been reported. Additionally, there is a lack of both qualitative and quantitative descriptions regarding the correlations among soil carbon, nitrogen, phosphorus, enzyme activity, their respective stoichiometries, and the yield of greenhouse tomatoes under the combined effects of moistube irrigation and fertilization. In this study, greenhouse tomatoes in facility agriculture were selected as the focal point, with the goal of investigating how varying irrigation amounts and fertilization rates impact soil nutrients, enzyme activities, and tomato yield. To achieve this, regression analysis was employed to provide both qualitative and quantitative descriptions of the correlations among soil carbon, nitrogen, phosphorus, enzyme activities, stoichiometry, and greenhouse tomato yield in the context of moistube irrigation and fertilization coupling. Furthermore, leveraging range analysis, an optimal combination of irrigation amounts and fertilization rates suited for tomatoes in moistube irrigation greenhouses was determined. The overarching aim of this study is to furnish a theoretical foundation to support the sustainable development of the facility agriculture tomato industry.
2.1 Experimental Site and Management
The experiment was carried out in a greenhouse located in Chencao Township, Xuchang City, Henan Province, China (N 34°08′, E 113°59′). This greenhouse is situated within the northern warm temperate monsoon climate zone, characterized by an annual precipitation of approximately 700 mm. The structure of the greenhouse measures 86 m in length and 10 m in width, oriented in a north-south direction. The soil type within the greenhouse is classified as alluvial soil. In the 0.80-m soil layer, the average bulk density is recorded at 1.39 g/cm3, with a field capacity water holding capacity of 25.60%. Additionally, the soil organic matter content is measured at 20.1 mg/kg, while the total nitrogen, phosphorus, and potassium contents are 1.15 g/kg, 1.71 g/kg, and 72.34 mg/kg, respectively. The tomato variety employed for this study is ‘Nongbofenba 1316’ (Jingyan Yinong Seed Sci-Tech Co. Ltd., Beijing, China), cultivated using a one-tube, one-row system on the ridges. The depth of the moisture tube is set at 20 cm, with a row spacing of 50 cm and a plant spacing of 40 cm, while each plot is separated by a distance of 2.0 m. Field management practices, including fertilization, irrigation, and pesticide application, were standardized across all plots to ensure uniformity. The irrigation water is sourced from the local groundwater supply. To guarantee the healthy establishment of seedlings on the day of planting, the irrigation water was applied based on established local practices for tomato cultivation. Moistube irrigation (MI) fertilization system is shown in Fig. 1. The irrigation management practices throughout the growth period of the tomatoes in the greenhouse are detailed in Table 1. The relevant meteorological data of the whole growth period of greenhouse tomato are shown in Fig. 2.
Figure 1: Schematic diagram of moistube irrigation system, where 1 represents the headwaters; 2 represents the valve; 3 represents the cistern; 4 represents the water pump; 5 represents the valve; 6 represents the tomato; 7 represents the moistube; 8 represents the filter; 9 represents the capillary valve; 10 represents the pressure gauge; 11 represents the fertilizer applicator. (A–C) represent three independent water supply systems, respectively. Adapted with permission from Reference [28]. Copyright ©2022, Mingzhi Zhang
Figure 2: Greenhouse meteorological data
The MI system consists of micro-embellishment pipes, water pumps, feed-water tanks, water meters, filters, and pressure gauges [28]. In this system, the working pressure of each treatment area is regulated through the principle of diversion, enabling the establishment of distinct working pressures across different plots. We used the third generation of Moistube, developed by Shenzhen Moistube Irrigation Technology Co., Ltd., Shenzhen, China. The inner layer of this tubing consists of a polymer semi-permeable membrane, with a thickness of 0.06 mm and a pore diameter ranging between 10 to 900 nm. By adjusting the fertilizer applicator, a mixture of water and fertilizer was prepared in a dilution ratio of 1:600, which served as the irrigation solution for the tomatoes. The test fertilizer employed in this experiment was a high-strength, balanced water-soluble fertilizer (N-P-K: 20%-20%-20%) produced by Shenzhen Dugao Biological New Technology Co., Ltd., Shenzhen, China.
In this experiment, two factors of irrigation amount and fertilization amount were set up, with three levels for each. A total of 9 treatments (Table 2) were used in a completely randomized experimental design, and each treatment was repeated three times. Among them: irrigation amount (I) by controlling the micro-irrigation capillary working pressure to achieve different irrigation gradient, set three levels: working pressure head were 1 (I1), 2 (I2), 3 (I3) m. The fertilization amount (F) was set at three levels: 100 (F1), 200 (F2) and 300 (F3) kg/hm2, and fertilization was applied once every 10 days. A total of 11 fertilizations were applied during the tomato growth period.
2.3 Test Equipment and Observation Item
(1) Collection and determinationof soil samples
The soil samples analyzed in this study were exclusively rhizosphere soil, sourced using the shaking soil method. Specifically, tomato roots measuring 5 to 25 cm were excavated as intact units, during which the loosely associated soil was dislodged from the roots. For the soil that adhered closely to the tomato roots in the greenhouse, a gentle brushing technique was employed with a soft brush to collect the rhizosphere soil. From each plot, three soil samples were randomly selected at 40, 70, and 110 days post-planting of tomatoes, for the years 2021 and 2022, respectively. Upon collection, the samples were transported back to the laboratory, where fresh plant residues were meticulously removed. The soil samples were subsequently divided into two portions; the majority were preserved in a refrigerator at −20 degrees Celsius for subsequent analysis of soil nutrients and enzyme activities, while a smaller fraction was subjected to air drying. The moisture content of the soil was determined using a drying method, which was then converted into soil volume moisture content. All measurements and determinations related to soil indicators were conducted within a span of 10 days.
The soil samples collected during various periods were analyzed to ascertain the nutrient content, and the methodologies for determining soil nutrient indices were as follows. Total organic carbon (TOC) was measured using a total organic carbon analyzer (Vario El, Elementar Analysen Systeme GmbH, Germany). Total nitrogen (TN) was assessed through the degradation of concentrated sulfuric acid accompanied by potassium sulfate-copper sulfate catalysis, employing the semi-micro Kjeldahl method for nitrogen determination. Total phosphorus (TP) was obtained by digesting samples with hydrochloric and sulfuric acids (HClO4-H2SO4) and subsequently analyzed using the molybdenum-antimony (Mo-Sb) colorimetric method.
Additionally, soil samples collected at different times were utilized to evaluate enzyme activity. The methods for determining the indices of soil enzyme activity were as follows: Soil β-glucosidase activity (BG), soil leucine aminopeptidase activity (LAP), and soil N-acetylglucosaminidase activity (NAG) were extracted via enzyme-linked immunosorbent assay and analyzed using a microplate reader (RT-6100, Shanghai Precision Instrument Co., Ltd., Shanghai, China). Alkaline phosphatase activity (AP) in the soil was determined by the disodium phenyl phosphate colorimetric method.
(2) Tomato yield
The electronic scale measured the tomato yield in each plot and converted the results into yields per hectare.
The significant differences were analyzed using the F test in SPSS version 22.0 (IBM Corp., Armonk, New York, NY, USA), with a significance level set at p < 0.05. The graphs were created using OriginPro 2019 (Origin Lab Corporation, Northampton, MA, USA). Additionally, regression analysis was conducted to evaluate the correlations between the stoichiometric ratios of soil carbon, nitrogen, and phosphorus, enzyme stoichiometric ratios, and tomato yield utilizing Excel 2016 (Microsoft Corporation, Redmond, WA, USA).
3.1 Effects of Integration of Water and Fertilizer by Moisture Irrigation on Soil Moisture of Greenhouse Tomato
Fig. 3 indicates that the average moisture content of rhizosphere soil in F2I2 tomato growth period was significantly higher than that in I1F1 by about 9.19% and 15.07% (2021 and 2022, the same below). However, the average moisture content of rhizosphere soil during tomato growth period was lower than that of I3F3 by about 6.38% and 3.89%. When the irrigation amount increased from I1 to I3, the rhizosphere soil moisture of tomato increased by 9.50% and 13.03%, respectively. With the increase of fertilizer amount, the soil moisture of tomatoes in the integration of moistube irrigation and fertilizer greenhouse also showed an increasing trend. The soil moisture of tomato rhizosphere in F2 treatment was about 2.81% and 3.70% higher than that in F1 treatment, but lower than that in F3 treatment about 2.24% and 2.03%.
Figure 3: Effects of different treatments on soil moisture of tomato. I represents irrigation amount; F represents fertilization amount; the data are shown as average ± standard deviation in the figure, different letters in the same color column meant significant difference at 0.05 level, the same as below
3.2 Effect of Moistube Irrigation and Fertilizer Integration on Tomato Soil Nutrients
Table 3 demonstrates that under the integration of moistube irrigation and fertilizer, with the advancement of tomato growth period, the total organic carbon (TOC) of tomato rhizosphere soil increased. Soil total nitrogen (TN) and total phosphorus (TP) showed a decreasing trend. The two factors of irrigation and fertilization had significant effects on TOC, TN and TP in rhizosphere soil of greenhouse tomato in the integration of moistube irrigation and fertilizer (p ≤ 0.05).
With the increase of I, the TOC of tomato soil increased first and then decreased. When the I increased from I1 to I3, the soil TN decreased by 5.07% and 4.91%, and the soil TP decreased by 4.37% and 4.22%. With the increase of F, the TOC of tomato soil increased first and then decreased. The TOC of tomato soil under F2 treatment was higher than that of F1 by about 7.56% and 11.29%, and higher than that of F3 by about 0.17% and 1.58%. When the F increased from F1 to F3, the soil TN decreased by 4.79% and 4.68%, and the soil TP decreased by 12.32% and 10.47%.
3.3 Effects of Moistube Irrigation and Fertilizer Integration on Tomato Soil Enzyme Activity
It can be seen from Table 4 that with the advancement of tomato growth period, the activities of BG, LAP, NAG and AP in tomato soil of moistube irrigation and fertilizer integration greenhouse increased first and then decreased. Irrigation and fertilization had significant effects on the activities of BG, LAP, NAG and AP in tomato soil (p ≤ 0.05). With the increase of irrigation amount, the activities of BG, LAP, NAG and AP in tomato soil increased first and then decreased. The activity of BG under I2 treatment was about 26.14% and 18.80% higher than that of I1, and about 11.09% and 6.28% higher than that of I3. LAP activity under I2 treatment was about 14.28% and 17.02% higher than that of I1, and about 6.68% and 8.37% higher than that of I3. The activity of NAG in I2 was higher than that in I1 by about 41.10% and 29.74%, and higher than that in I3 by about 18.44% and 12.43%. AP activity under I2 treatment was about 24.39% and 38.49% higher than that of I1, and about 10.04% and 19.02% higher than that of I3. With the increase of fertilizer amount, the activities of BG, LAP, NAG and AP in tomato soil increased first and then decreased. The activity of BG was about 12.11% and 12.68% higher than that of I1 under I2 treatment, and about −0.51% and 1.40% higher than that of I3. LAP activity under I2 treatment was 16.46% and 14.53% higher than that of I1, and about -0.82% and 1.50% higher than that of I3. The activity of NAG under I2 treatment was about 17.71% and 15.12% higher than that of I1, and about −1.22% and 1.53% higher than that of I3. The activity of AP under I2 treatment was about 11.56% and 14.36% higher than that of I1, and about 0.94% and 0.30% higher than that of I3.
3.4 Effect of the Integration of Water and Fertilizer by Moisture Irrigation on Tomato Soil Stoichiometry
It can be seen from Fig. 4 that with the advancement of tomato growth period, the C/N, C/P and N/P of tomato soil in greenhouse with the integration of moistube irrigation and fertilizer showed an increasing trend. Under the condition of the integration of moistube irrigation and fertilizer, with the increase of irrigation amount, soil C/N, C/P and N/P increased first and then decreased. Among them, soil C/N under I2 treatment was higher than I1 by about 11.82% and 14.70%, higher than I3 by about 0.51% and 3.46%. The soil C/P under I2 treatment was about 41.10% and 22.86% higher than that of I1, and about 14.70% and 11.35% higher than that of I3. The soil N/P under I2 treatment was about 14.36% and 6.91% higher than that of I1, and about 14.41% and 7.21% higher than that of I3. With the increase of fertilizer amount, soil C/N, C/P and N/P showed an increasing trend. When the fertilizer amount increased from F1 to F3, the soil C/N increased by about 12.13% and 14.88%. The soil C/P increased by about 21.36% and 21.68%; the soil N/P increased by about 5.19% and 5.95%.
Figure 4: Effects of different treatments on stoichiometry of soil nutrients and enzyme activity. C represents total organic carbon, N represents total nitrogen, P represents total phosphorus. BG represents β-Glucosidase active enzyme, LAP represents Leucine aminopeptidase activity, NAG represents N-acetyl-d-glucosaminidasealk, AP represents aline phosphatase activity. (a) represents the change of C/N with tomato growth period, (b) represents the change of C/P with tomato growth period, (c) represents the change of N/P with tomato growth period, (d) represents the change of BG/LAP+NAG with tomato growth period, (e) represents the change of BG/AP with tomato growth period, (f) represents the change of LAP+NAG/AP with tomato growth period. Different letters in the same tomato growth meant significant difference at 0.05 level
Stoichiometry of Soil Enzyme Activity
From Fig. 4, it can be seen that with the advancement of tomato growth period, the BG/(LAP+NAG), BG/AP, (LAP+NAG)/AP of tomato soil in the integrated greenhouse of moistube irrigation and fertilization showed a trend of increasing first and then decreasing. The two factors of irrigation and fertilization had significant effects on soil BG/(LAP+NAG), BG/AP, (LAP+NAG)/AP (p ≤ 0.05). Under the condition of moistube irrigation and fertilizer integration, the soil BG/(LAP+NAG) and BG/AP decreased first and then increased with the increase of irrigation amount. Soil (LAP+NAG)/AP showed a decreasing trend. When the amount of fertilizer increased from F1 to F3, the soil BG/(LAP+NAG) and BG/AP decreased by about 4.94% and 2.96%, 1.29% and 2.63%, respectively. Soil (LAP+NAG)/AP increased by about 5.75% and 0.29%.
3.5 Effects of the Integration of Water and Fertilizer by Moisture Irrigation on Tomato Yield in Greenhouse
It can be seen from Fig. 5 that the amount of irrigation and fertilizer had a significant effect on the yield of tomato in the integration of water and fertilizer by moisture irrigation (p ≤ 0.05). One-way analysis of variance showed that the yield of tomato treated with I2F2 was significantly higher than that of I1F1, I1F2, I1F3, I2F1, I2F3, I3F3, I3F1 and I3F2 by about 38.84% and 45.50%, 18.02% and 19.83%, 18.99% and 23.71%, 28.37% and 29.66%, 3.53% and 1.07%, 26.16% and 29.47%, 3.96% and 1.99%, 4.68% and 7.73%.
Figure 5: Effects of different treatments on tomato yield. I represents irrigation amount; F represents fertilization amount; the data are shown as average ± standard deviation in the figure, different letters in the same color column meant significant difference at 0.05 level, the same as below
With the increase of irrigation amount, the yield of tomato reached the peak value in I2 treatment, and the tomato in I2 treatment was about 13.99% and 18.48% higher than that in I1 treatment, and about 1.29% and 2.97% higher than that in I3 treatment. With the increase of fertilizer amount, the tomato yield increased first and then decreased, in which F2 treatment tomato yield was higher than than F1 about 22.57% and 26.18%, higher than F3 about 1.72% and 3.26%.
3.6 Correlation Analysis of Rhizosphere Soil Nutrients, Enzyme Activity and Yield of Greenhouse Tomato under the Integration of Water and Fertilizer by Moisture Irrigation
3.6.1 Quantitative Description of the Correlation between Soil Nutrients, Enzyme Activity and Yield
It can be seen from Fig. 6 that the relationship between soil carbon, nitrogen, phosphorus, soil enzyme activity and yield of tomato in the integration of moistube irrigation and fertilizer showed a quadratic parabolic curve, and the coefficient of determination R2 > 0.6819, indicating that soil carbon, nitrogen, phosphorus and soil enzyme activity in the regression model can explain yield to 68.19%. Soil carbon, nitrogen, phosphorus and soil enzyme activity can be used to estimate yield. In the regression model of soil carbon, nitrogen and phosphorus and yield, the fitting degree of the model of tomato soil TN and yield was better, and the determination coefficient R2 > 0.8451. When the TOC of tomato soil was 18.00–19.00, the yield of tomato could reach 127.71–122.23 t/ha. In the regression model of soil enzyme activity and yield, the fitting degree of the model of soil BG and yield was better, and the determination coefficient R2 > 0.7531. When the soil BG activity was 6.40–7.40, the tomato yield could reach 130.36–136.20 t/ha.
Figure 6: Regression analysis of soil carbon, nitrogen, phosphorus, enzyme activity and yield. TOC represents total organic carbon, TN represents total nitrogen, TP represents total phosphorus. BG represents β-Glucosidase active enzyme, LAP represents Leucine aminopeptidase activity, NAG represents N-acetyl-d-glucosaminidasealk, AP represents aline phosphatase activity. (a) represents the correlation between yield and TOC, (b) represents the correlation between yield and TN, (c) represents the correlation between yield and TP, (d) represents the correlation between yield and BG, (e) represents the correlation between yield and LAP+NAG, (f) represents the correlation between yield and AP
3.6.2 Quantitative Description of the Correlation between Soil Nutrients, Enzyme Stoichiometry and Yield
A quadratic parabolic relationship can be observed between the stoichiometry of soil carbon, nitrogen, and phosphorus, soil enzyme activity, and tomato yield under the combined effects of moistube irrigation and fertilizer, as shown in Fig. 7. The coefficient of determination (R2 > 0.7042) indicates that the stoichiometry of soil carbon, nitrogen, phosphorus, and soil enzyme activity in the regression model explains over 70.42% of the variation in yield. The stoichiometry of soil carbon, nitrogen and phosphorus, and soil enzyme activity can be used to estimate Yield. In the regression model of soil carbon, nitrogen and phosphorus stoichiometry and yield, the fitting degree of the model between tomato soil C/P and yield is better, and the determination coefficient R2 > 0.8451. When the tomato soil C/P is 17.50–18.50, the tomato yield can reach 126.63–137.76 t/ha. In the regression model of soil enzyme activity stoichiometry and yield, the fitting degree of the model of soil BG/AP and yield was better, and the determination coefficient R2 > 0.8015. When the soil BG/AP was 0.60–0.65, the tomato yield could reach 118.998.750–118.86 t/ha.
Figure 7: Regression analysis of soil nutrient, enzyme activity stoichiometry and yield. C represents total organic carbon, N represents total nitrogen, P represents total phosphorus. BG represents β-Glucosidase active enzyme, LAP represents Leucine aminopeptidase activity, NAG represents N-acetyl-d-glucosaminidasealk, AP represents aline phosphatase activity. (a) represents the correlation between yield and C/N, (b) represents the correlation between yield and C/P, (c) represents the correlation between yield and N/P, (d) represents the correlation between yield and BG/LAP+NAG, (e) represents the correlation between yield and BG/AP, (f) represents the correlation between yield and LAP+NAG/AP
The appropriate regulation of water and fertilizer application can significantly reduce soil greenhouse gas emissions while promoting plant growth and development. This strategy enhances both crop quality and yield [29,30]. Compared to conventional drip irrigation methods, moistube irrigation technology presents distinct advantages, including lower operational pressure, higher irrigation uniformity, and a consistent, stable supply of water and fertilizers [20,31].
4.1 Effects of Different Treatments on Soil Nutrients and Their Stoichiometry of Greenhouse Tomato
Irrigation and fertilization alter the distribution of soil water and nutrients, with increased levels promoting the leaching of surface litter. This process facilitates the transfer of clay, organic matter, carbonate, and other substances from the surface litter and humus into the soil, thereby increasing the total organic carbon content of the soil [32,33]. This study found that with the increase of irrigation amount, the total organic carbon of tomato soil in the integration of moistube irrigation and fertilizer increased first and then decreased. The total nitrogen and total phosphorus contents of tomato soil in the integration of moistube irrigation and fertilizer decreased first and then increased with the increase of irrigation amount. The observed changes in soil total organic carbon in this study may be attributed to the uniform distribution of soil moisture in the I2 treatment. This uniformity prevents hypoxic stress or water stress, which could otherwise result from excessively high or low soil volumetric moisture content across large areas of the wetted soil zone. The root morphological development of I2 treatment is better than that of I1 and I3, and the better root morphological development is beneficial to improve the abundance of root microorganism. The remnant body of plants and soil microorganisms provide sufficient carbon for crop rhizosphere soil [34,35] The changes in soil total nitrogen and phosphorus may be attributed to the alternating dry-wet cycles under the I1 treatment, which led to the disintegration of soil aggregates. This process facilitated the release of additional nitrogen and phosphorus, thereby increasing their availability in the soil. The soil microbial biomass in arid environment was small, which reduced the amount of nitrogen fixed by microorganisms, and the mineralization nutrients were more released into the soil. At the same time, the lower irrigation amount can reduce the leaching of nitrogen in shallow soil [36,37], which promotes the relatively high nitrogen and phosphorus content in I1 tomato soil. The water holding capacity of I2 treatment soil is enhanced, which may lead to the re-fixation of nutrients, resulting in a reduction in total nitrogen and phosphorus content. This process also accelerates the growth and metabolic activities of tomato plants. The absorption of nutrients also increased accordingly; the soil water content of I3 treatment was significantly higher than that of I2. Higher soil moisture reduced soil aeration and limited microbial activity and mineralization. Simultaneously, plant growth was inhibited, leading to a reduction in soil nutrient absorption rates and a subsequent increase in soil total nitrogen and phosphorus content [38]. Yuan et al. [39] found that with the increase of irrigation amount of winter wheat, the total nitrogen of soil increased first and then decreased, which contrasts with the findings of this study, where total nitrogen in tomato soil under the integrated water and fertilizer moistube irrigation exhibited a decreasing trend. This discrepancy may be attributed to differences in crop types and variations in the water quality of the sources used for crop growth. The water requirement of winter wheat not only comes from irrigation, but also from rainfall. In this study, the water requirement of greenhouse tomato only comes from irrigation.
This study found that the total organic carbon of tomato soil in the integration of moistube irrigation and fertilizer increased first and then decreased with the increase of fertilizer application, probabably owing to the fact that the application of nitrogen fertilizer in F2 treatment can promote crop growth and increase crop biomass. After the crop residues are returned to the soil, the accumulation of soil organic carbon will also increase. When the fertilization amount reached F3, excessive soil ion concentration led to soil acidification, inhibited soil microbial activity and tomato root morphological development, and reduced the source of soil carbon from microorganisms and plant growth and development residues [40,41], which was consistent with Tuo et al. [14]. The change of soil microbial carbon with the increase of fertilizer amount was consistent. The total nitrogen and total phosphorus content of tomato soil in the greenhouse of moistube irrigation and fertilizer integration decreased with the increase of fertilizer amount, which may be due to the fact that appropriate fertilization can promote microbial activity and accelerate the decomposition of organic matter, thus releasing nitrogen and phosphorus in the soil and promoting a large amount of nitrogen and phosphorus to be converted into plants. However, excessive fertilization may inhibit the activity of some beneficial microorganisms, resulting in the fixation and transformation of nitrogen and phosphorus in soil, thus limiting the increase of total nitrogen and total phosphorus content in soil [42,43].
Soil C/N reflects a balance between soil carbon and nitrogen. It is an important index to measure the balance of soil nutrient elements and can reflect the ability of microorganisms to meet their nutrient needs with plants [44]. In this study, it was found that the soil C/N (peak value of 8.385) of tomato soil in the integration of moistube irrigation and fertilizer greenhouse was lower than the average soil value of 12.30 in China [34], because the soil C/N was inversely proportional to the decomposition rate and mineralization rate of organic matter [45], so the soil C in this study area was more deficient than N. The C/N of tomato soil in the integration of water and fertilizer by moisture irrigation increased with the increase of irrigation amount, which may be due to the influence of soil total organic carbon and total nitrogen content with the change of irrigation amount. Compared with I2, the decrease of total organic carbon in spring tomato and autumn tomato soil under I3 treatment was lower than that of total nitrogen. The change of soil C/N with irrigation amount further indicated that increasing irrigation amount could reduce the degree of soil carbon deficiency. With the increase of fertilizer amount, the soil C/N of tomato in the integration of moistube irrigation and fertilizer showed an increasing trend, which may be due to the promotion of crop growth by F2 treatment, the increase of crop biomass, and the increase of plant residues (such as leaves, dead branches, root metabolites, etc.). The decomposition of residues in the soil further increases the input of soil organic carbon, which can lead to the accumulation of organic carbon in the soil. The rate of accumulation exceeds the accumulation of total nitrogen, which promotes the increase of C/N; when the amount of fertilizer increased to F3, excessive nitrogen fertilizer may inhibit the growth of soil microorganisms, especially nitrogen-sensitive microorganisms, resulting in a decrease in the decomposition rate of organic carbon and a relative increase in the accumulation rate of soil organic carbon [46,47].
Soil C/P is a measure of the ability of organic matter to release or absorb phosphate mineralization [48]. The C/P (13.932) of tomato soil in this study area was much lower than the average level in China (52.700), indicating that the net mineralization rate of P in greenhouse tomato soil in this area was high and the potential of P release was large [49]. This study found that soil C/P increased first and then decreased with the increase of irrigation amount, which may be due to the increase of soil organic matter content in I2 treatment, which promoted the release of soil phosphorus and increased soil phosphorus availability. At the same time, various metabolites (such as acidic substances) released during microbial degradation can change soil pH, promote phosphorus mineralization and increase phosphorus availability, and aggravate the absorption of phosphorus in soil root zone by crops, resulting in a decrease in TP in this area, and an increase in C and a higher C/P [50]. It is consistent with the conclusion of studying the variation of soil C/P with irrigation amount in winter wheat, indicating that the change of soil C/P due to the change of irrigation amount has nothing to do with crop species. Fertilization promotes soil acidification, increases the absorption of phosphorus by plants, and reduces its bioavailability, which can offset the increase of phosphorus content caused by fertilization to a certain extent, which may be one of the reasons why the C/P of tomato soil increases with the increase of fertilizer amount [51].
Soil N/P can be used to measure the threshold of nutrient limitation of nitrogen and phosphorus, and to determine the lack of nutrient supply during plant growth [52]. The average value of N/P in this study area (1.607) was lower than that of China’s land soil (3.900) [53], indicating that the nitrogen element was deficient in this study area, and the soil phosphorus changed little. This study found that with the increase of irrigation amount, the soil N/P showed a trend of increasing first and then decreasing. It may be due to the fact that the P element in I2 treatment is conducive to the absorption and utilization of mineralization by plants, resulting in the rhizosphere soil phosphorus content lower than I1 and I3. The total nitrogen content also decreased with the increase of irrigation amount, resulting in a larger N/P ratio under I2 treatment, indicating that irrigation amount I2 will promote the absorption of soil nitrogen [54]. With the increase of fertilizer amount, the N/P of tomato soil showed an increasing trend, which may be due to the increase of fertilizer amount will stimulate soil microbial activity. These microorganisms will release nitrogen and absorb phosphorus in the process of decomposing organic matter. The demand for nitrogen is also greater than that of phosphorus, which may lead to the mineralization rate of nitrogen in soil higher than that of phosphorus, and the N/P ratio is increased [55,56].
4.2 Effects of Different Treatments on Soil Enzyme Activities and Their Stoichiometry of Greenhouse Tomato
Soil BG, LAP, NAG, and AP are four common extracellular hydrolases involved in microbial decomposition processes. These enzymes serve as key indicators of soil carbon, nitrogen, and phosphorus turnover rates and are positively correlated with soil organic matter concentration [57]. Previous studies have shown that irrigation and fertilization can increase soil water availability to promote plant growth and microbial-driven biological processes, thus promoting enzyme secretion and substrate diffusion, which is conducive to improving soil hydrolase activity [58]. This study found that with the increase of irrigation amount, the activity of soil hydrolase in greenhouse tomato soil increased first and then decreased, which may be due to the fact that soil moisture can directly participate in soil biochemical reactions and affect the life activities of microorganisms and plants. Lower moisture is not conducive to the diffusion of soil nutrients and the infiltration of effective elements into microorganisms. At the same time, the decrease of soil moisture can limit the leaching of litter and inhibit the increase of soil hydrolase activity [59]. When the irrigation amount is too high, the soil aeration is poor, and the low oxygen soil micro-environment will inhibit the increase of aerobic microorganisms (Bacillus, etc.), resulting in the decrease of soil microbial community structure diversity and population abundance, the decrease of root absorption and utilization of soil nutrients, and the restriction of crop root metabolism cycle. The lower root cycle metabolites root exudates and soil microbial biomass directly limit the increase of soil hydrolase activity [60,61]. This conclusion is consistent with the variation of soil β-glucosidase activity of Wang [60] and soil alkaline phosphatase activity of Zhou et al. [62] with the change of irrigation amount. This study also found that with the increase of fertilizer amount, the activity of soil hydrolase in tomato greenhouse increased first and then decreased, which may be due to the fact that F2 treatment provided a direct nutrient source for soil microorganisms, especially nitrogen, phosphorus, potassium and other elements. The available substrates (such as organic substances) in the soil were also high, which would promote the growth and reproduction of microorganisms, thus increasing the activity of hydrolase in the soil. The binding sites of soil enzymes and substrates in F3 treatment may be saturated. At this time, when the pH value deviates from the optimal growth range of microorganisms or the soil salinity is too high, the microbial activity will be inhibited, which in turn reduces the production and activity of hydrolases [63].
Globally, the ratio of BG to LAP+NAG to AP enzyme activities typically follows a 1:1:1 scaling pattern, indicating a coupled relationship between carbon, nitrogen, and phosphorus cycles. However, this study found that the ratio of BG/LAP+NAG/AP enzyme activity (1:0.12:0.77) in tomato rhizosphere soil under different treatments of facility agriculture moistube irrigation and fertilizer integration in this area deviated greatly from 1:1:1. It showed that the activity of C-degrading enzyme was less than that of N or P-degrading enzyme and the activity of N-degrading enzyme was the largest among the three, which indicated that the input of microorganisms to the enzymes involved in soil C, N and P cycles was significantly different under different irrigation and fertilization amounts, and highlighted the regionality of the test area [64]. The stoichiometry of soil ecological enzymes can also be used to evaluate the demand of microbial carbon, nitrogen, phosphorus and nutrients [65]. Previous studies have found that soil enzyme activity ratios BG/AP and LAP+NAG/AP are higher than the global scale soil enzyme activity ratios BG/AP (0.62) and (LAP+NAG)/AP (0.44) indicate that soil microorganisms in the region are limited by carbon and nitrogen [66]. This study found that the BG/AP of tomato soil in facility agriculture is lower than the global scale, and the LAP+NAG/AP is higher than the global scale, further indicating that the rhizosphere soil microorganisms in the region are limited by carbon and phosphorus.
4.3 Effects of Different Treatments on Tomato Yield in Greenhouse
Drought stress on tomato roots adversely affects both the number of fruits and the weight of individual fruits [67,68]. The findings of this study indicate that tomato yield initially increases with rising irrigation amounts before ultimately declining. This phenomenon may be attributed to a reduction in soil volumetric water content under low irrigation treatment (I1), which subjects tomato roots to drought stress. Zhang et al. [69] observed that flowering and fruit enlargement in tomatoes are particularly sensitive to water stress. Consequently, as irrigation amounts decrease, the weight of individual fruits also trends downward, and this reduction in fruit weight constrains overall tomato yield. In the case of high irrigation treatment (I3), the soil’s water-filled porosity in the tomato root zone was approximately 6.64% greater than that observed in treatment I2. Such elevated soil water-filled porosity can impede the diffusion rate of oxygen in the soil [70]. This hinders the respiration of both roots and microorganisms, intensifying the activity of anaerobic respiration enzymes, decreasing the population of soil microorganisms [71], and disrupting the respiration, growth, and development of crops, which ultimately limits the potential increase in tomato yield [72]. Studies conducted by Agbna et al. [73], Yang et al. [74], and Zhu et al. [75] demonstrated that drip irrigation enhances tomato yield in accordance with increased irrigation amounts, which aligns with the effects noted in this study regarding moisture tube irrigation on tomato yield. Moreover, tomato absorption efficiency for nitrogen, phosphorus, and potassium was significantly influenced by the amount of fertilizer applied. An increase in fertilizer application tends to decrease nitrogen utilization efficiency while promoting the accumulation of nitrate nitrogen in the soil, consequently diminishing soil fertility [76,77]. Excessive fertilizer applications can reduce the fruit setting rate in tomatoes, impair stress resistance, and predispose plants to pests and diseases, thereby restricting the enhancement of tomato yield [78]. This study corroborates the earlier findings that tomato yield follows an initial increase followed by a decrease in response to rising fertilizer amounts, mirroring the trends reported in the research by Zhao et al. [79], Wu et al. [30], and Wang et al. [80].
The response mechanism of soil nutrients and enzyme activity in greenhouse tomatoes to varying irrigation and fertilizer amounts under moistube irrigation was investigated through a randomized experimental design. This study aims to identify an optimal combination of irrigation and fertilizer amounts conducive to the growth of greenhouse tomatoes. The conclusions of this study are as follows:
With the increase of I, the TOC content, BG, LAP, NAG, and AP activity of greenhouse tomato soil increased first and then decreased. The contents of TN and TP in soil decreased first and then increased. With the increase of fertilizer amount, the TOC of tomato soil increased first and then decreased. The activities of BG, LAP, NAG and AP in soil increased first and then decreased. The contents of TN and TP in soil showed a decreasing trend.
The combination of micro-irrigation capillary working pressure working pressure head of 2 m and fertilization amount of 200 kg/hm2 under the integration of water and fertilizer by moistube irrigation not only alleviates soil carbon and nitrogen limitations but also enhances soil enzyme activity, thereby simultaneously achieving multiple objectives, such as increasing tomato yields while conserving water and fertilizer resources.
Through the correlation analysis of tomato soil carbon, nitrogen and phosphorus, enzyme activity and their respective stoichiometry and yield at different tomato planting periods, it was found that the tomato soil carbon, nitrogen and phosphorus, enzyme activity and their respective stoichiometric ratio and yield showed a quadratic curve relationship at 72 days after planting, and the correlation was better (R2 > 0.681), which can realize the prediction of tomato yield, and can also be used as an important index to measure the nutrient cycle of facility agricultural soil in this area.
Acknowledgement: We thank Xuchang Irrigation Experimental Station for providing experimental sites and equipment.
Funding Statement: The research is funded by the Natural Science Foundation of China (No. 41807041), the Science and Technology Research Project of Henan Province (No. 242102111101), Social Science Foundation of Shaanxi Province (2023SJ03). We are grateful for the helpful comments of the anonymous reviewers. We are grateful for the helpful comments of the anonymous reviewers.
Author Contributions: The authors confirm contribution to the paper as follows: study conception and design: Mingzhi Zhang, Yuan Li; data collection: Haijian Yang; analysis and interpretation of results: Na Xiao; draft manuscript preparation: Mingzhi Zhang. All authors reviewed the results and approved the final version of the manuscript.
Availability of Data and Materials: The data that support the findings of this study are available from the corresponding author, upon reasonable request.
Ethics Approval: Not applicable.
Conflicts of Interest: The authors declare that they have no conflicts of interest to report regarding the present study.
References
1. Han Y, Lu L, Wang L, Liu Z, Huang P, Chen S, et al. In-situ straw return, combined with plastic film use, influences soil properties and tomato quality and yield in greenhouse conditions. Agric Commun. 2024;2(1):100028. doi:10.1016/j.agrcom.2024.100028. [Google Scholar] [CrossRef]
2. Wu P, Wang Y, Li Y, Yu H, Shao J, Zhao Z, et al. Evaluating optimized irrigation strategies on crop productivity and field water utilization under micro sprinkling irrigation in typical cropping systems of the Huang-Huai-Hai Plain. Eur J Agron. 2024;154:127093. doi:10.1016/j.eja.2024.127093. [Google Scholar] [CrossRef]
3. Thiloka Edirisooriya EMN, Wang H, Banerjee S, Longley K, Wright W, Mizuno W, et al. Economic feasibility of developing alternative water supplies for agricultural irrigation. Curr Opin Chem Eng. 2024;43:100987. doi:10.1016/j.coche.2023.100987. [Google Scholar] [CrossRef]
4. Liu J, Li H, Yuan Z, Feng J, Chen S, Sun G, et al. Effects of microbial fertilizer and irrigation amount on growth, physiology and water use efficiency of tomato in greenhouse. Sci Hortic. 2024;323:112553. doi:10.1016/j.scienta.2023.112553. [Google Scholar] [CrossRef]
5. Volpi I, Bosco S, Ragaglini G, Laville P, Bonari E. Tomato productivity and soil greenhouse gas emissions under reduced water and N fertilizers in a Mediterranean environment. Agric Ecosyst Environ. 2022;326:107819. doi:10.1016/j.agee.2021.107819. [Google Scholar] [CrossRef]
6. Hua K, He J, Liao B, He T, Yang P, Zhang L. Multi-objective decision-making for efficient utilization of water and fertilizer in paddy fields: a case study in Southern China. Agr Water Manage. 2023;289:108507. doi:10.1016/j.agwat.2023.108507. [Google Scholar] [CrossRef]
7. Li J, Zhu Y, Liu M, Liu Z, Zhou T, Liu Y, et al. Network interpenetrating slow-release nitrogen fertilizer based on carrageenan and urea: a new low-cost water and fertilizer regulation carrier. Int J Biol Macromol. 2023;242:124858. doi:10.1016/j.ijbiomac.2023.124858. [Google Scholar] [PubMed] [CrossRef]
8. Liu X, Peñuelas J, Sardans J, Fang Y, Wiesmeier M, Wu L, et al. Response of soil nutrient concentrations and stoichiometry, and greenhouse gas carbon emissions linked to change in land-use of paddy fields in China. Catena. 2021;203:105326. doi:10.1016/j.catena.2021.105326. [Google Scholar] [CrossRef]
9. Li J, Wan X, Liu X, Chen Y, Slaughter LC, Weindorf DC, et al. Changes in soil physical and chemical characteristics in intensively cultivated greenhouse vegetable fields in North China. Soil Tillage Res. 2019;195:104366. doi:10.1016/j.still.2019.104366. [Google Scholar] [CrossRef]
10. Zeyada AM, Al-Gaadi KA, Tola E, Amrir AS, Madugundu R, Marey SA, et al. Feasibility of variable rate application of diammonium phosphate fertilizer to wheat crop under center pivot irrigation system. J King Saud Univ-Sci. 2022;34:101904. doi:10.1016/j.jksus.2022.101904. [Google Scholar] [CrossRef]
11. Islam SMM, Gaihre YK, Islam MR, Ahmed MN, Akter M, Singh U, et al. Mitigating greenhouse gas emissions from irrigated rice cultivation through improved fertilizer and water management. J Environ Manage. 2022;307:114520. doi:10.1016/j.jenvman.2022.114520. [Google Scholar] [PubMed] [CrossRef]
12. Zapata-Sierra AJ, Moreno-Pérez MF, Reyes-Requena R, Manzano-Agugliaro F. Root distribution with the use of drip irrigation on layered soils at greenhouses crops. Sci Total Environ. 2021;768:144944. doi:10.1016/j.scitotenv.2021.144944. [Google Scholar] [PubMed] [CrossRef]
13. Chen S, Zhang S, Hu T, Li H, Sun J, Sun G, et al. Responses of soil reactive nitrogen pools and enzyme activities to water and nitrogen levels and their relationship with apple yield and quality under drip fertigation. Sci Hortic. 2024;324:112632. doi:10.1016/j.scienta.2023.112632. [Google Scholar] [CrossRef]
14. Tuo Y, Tan H, Liang J, Li J, Xiang P, Yang Q, et al. Optimization of water and fertilizer management of Panax pseudoginseng based on changes in soil microbial biomass carbon and nitrogen and enzyme activities. Appl Soil Ecol. 2024;196:105282. doi:10.1016/j.apsoil.2024.105282. [Google Scholar] [CrossRef]
15. Chtouki M, Naciri R, Oukarroum A. A review on phosphorus drip fertigation in the Mediterranean region: fundamentals, current situation, challenges, and perspectives. Heliyon. 2024;10:e25543. doi:10.1016/j.heliyon.2024.e25543. [Google Scholar] [PubMed] [CrossRef]
16. Hu M, Qf M, Hb S, Yu Q. Assessing the influence of water fertilizer, and climate factors on seed cotton yield under mulched drip irrigation in Xinjiang agricultural regions. Water Saving Irrig. 2018;35:25–31. [Google Scholar]
17. Wang H, Wu L, Wang X, Zhang S, Cheng M, Feng H, et al. Optimization of water and fertilizer management improves yield, water, nitrogen, phosphorus and potassium uptake and use efficiency of cotton under drip fertigation. Agr Water Manage. 2021;245:106662. doi:10.1016/j.agwat.2020.106662. [Google Scholar] [CrossRef]
18. Shen F, Fei L, Tuo Y, Peng Y, Yang Q, Zhao Z, et al. Effects of water and fertilizer regulation on soil physicochemical properties, bacterial diversity and community structure of Panax notoginseng. Cientia Hortic. 2024;326:112777. doi:10.1016/j.scienta.2023.112777. [Google Scholar] [CrossRef]
19. Zha Y, Chen F, Wang Z, Jiang S, Cui N. Effects of water and fertilizer deficit regulation with drip irrigation at different growth stages on fruit quality improvement of kiwifruit in seasonal arid areas of Southwest China. J Integr Agr. 2023;22:3042–58. doi:10.1016/j.jia.2023.08.015. [Google Scholar] [CrossRef]
20. Qi W, Zhang Z, Wang C, Huang M. Prediction of infiltration behaviors and evaluation of irrigation efficiency in clay loam soil under Moistube® irrigation. Agr Water Manage. 2021;248:106756. doi:10.1016/j.agwat.2021.106756. [Google Scholar] [CrossRef]
21. Fan Y, Ren C, Yang Z, Zhang C, Yin W. Prediction of wetting pattern dimensions under moistube irrigation with a multivariate nonlinear model. Water Sci Eng. 2024 Sep;17(3):217–25. doi:10.1016/j.wse.2023.12.006. [Google Scholar] [CrossRef]
22. Lv W, Niu W, Gu J. Effects of moistube depth and density on tomato yield and quality in solar greenhouse. Chin J Eco Agric. 2016;24(12):1663–73 (In Chinese). [Google Scholar]
23. Zhang MZ, Niu WQ, Lu ZG , Wang JW, Qiu XQ, Li Y. Effects of Moistube irrigation on winter wheat’s yield and irrigation water use efficiency. J Irrig Drainage. 2018;37:8–15. [Google Scholar]
24. Ou Y, Xu P. Comparative study on water-saving irrigation techniques for urban greening. Water Saving Irrig. 2021:16–23. [Google Scholar]
25. Zhu YM. Effect of pressure head on soil water and nitrogen migration and greenhouse vegetable growth in moistube-irrigation and fertilization. Taiyuan University of Technology: China; 2021. doi:10.27352/d.cnki.gylgu.2021.000853. [Google Scholar] [CrossRef]
26. Kanda EK, Senzanje A, Mabhaudhi T. Soil water dynamics under Moistube irrigation. Phys Chem Earth, Parts A/B/C. 2020;115:102836. doi:10.1016/j.pce.2020.102836. [Google Scholar] [CrossRef]
27. Niu WQ, Zhang MZ, Xu J, Li Y. Prediction methods and characteristics of flow for Moistube. Trans Chin Soc Agric Mach. 2017;48(6):217–24 (In Chinese). [Google Scholar]
28. Zhang MZ, Xiao N, Li Y, Li Y, Zhang D, Xu Z, et al. Growth and fruit yields of greenhouse tomato under the integrated water and fertilizer by moistube irrigation. Agronomy. 2022;12:1630. doi:10.3390/agronomy12071630. [Google Scholar] [CrossRef]
29. Xiao C, Zou H, Fan J, Zhang F, Li Y, Sun S, et al. Optimizing irrigation amount and fertilization rate of drip-fertigated spring maize in northwest China based on multi-level fuzzy comprehensive evaluation model. Agr Water Manage. 2021;257:107157. doi:10.1016/j.agwat.2021.107157. [Google Scholar] [CrossRef]
30. Wu Y, Yan S, Fan J, Zhang F, Zhao W, Zheng J, et al. Combined effects of irrigation level and fertilization practice on yield, economic benefit and water-nitrogen use efficiency of drip-irrigated greenhouse tomato. Agr Water Manage. 2022;262:107401. doi:10.1016/j.agwat.2021.107401. [Google Scholar] [CrossRef]
31. Niu W, Lü W, Gu J, Liang B, Guo L, Guan Y. Effects of moistube depth and spacing on soil water and salt transports of tomato in solar greenhouse. Trans Chin Soc Agric Eng. 2017;33(19):131–40 (In Chinese). [Google Scholar]
32. Yan Z, Zhang W, Wang Q, Liu E, Sun D, Liu B, et al. Changes in soil organic carbon stocks from reducing irrigation can be offset by applying organic fertilizer in the North China Plain. Agr Water Manage. 2022;266:107539. doi:10.1016/j.agwat.2022.107539. [Google Scholar] [CrossRef]
33. Henry HAL, Juarez JD, Field CB, Vitousek PM. Interactive effects of elevated CO2, N deposition and climate change on extracellular enzyme activity and soil density fractionation in a California annual grassland. Global Change Biol. 2005;11:1808–15. doi:10.1111/j.1365-2486.2005.001007.x. [Google Scholar] [CrossRef]
34. Yao X, Xiao B, Kidron GJ, Hu K. Respiration rate of moss-dominated biocrusts and their relationships with temperature and moisture in a semiarid ecosystem. Catena. 2019;183:104195. doi:10.1016/j.catena.2019.104195. [Google Scholar] [CrossRef]
35. Buckeridge KM, Mason KE, McNamara NP, Ostle N, Puissant J, Goodall T, et al. Environmental and microbial controls on microbial necromass recycling, an important precursor for soil carbon stabilization. Commun Earth Environ. 2020;1:49. doi:10.1038/s43247-020-00031-4. [Google Scholar] [CrossRef]
36. Hou X, Fan J, Hu W, Zhang F, Yan F, Xiao C, et al. Optimal irrigation amount and nitrogen rate improved seed cotton yield while maintaining fiber quality of drip-fertigated cotton in northwest China. Ind Crop Prod. 2021;170:113710. doi:10.1016/j.indcrop.2021.113710. [Google Scholar] [CrossRef]
37. Lu Y, Wang E, Zhao Z, Liu X, Tian A, Zhang X. Optimizing irrigation to reduce N leaching and maintain high crop productivity through the manipulation of soil water storage under summer monsoon climate. Field Crop Res. 2021;265:108110. doi:10.1016/j.fcr.2021.108110. [Google Scholar] [CrossRef]
38. Arifin I, Hanafi MM, Roslan I, Ubaydah MU, Karim YA, Tui LC, et al. Responses of irrigated oil palm to nitrogen, phosphorus and potassium fertilizers on clayey soil. Agr Water Manage. 2022;274:107922. doi:10.1016/j.agwat.2022.107922. [Google Scholar] [CrossRef]
39. Yuan JY, Li G, Yan LJ, Chen GP, Zhang SW, Teng R. Soil and plant carbon, nitrogen, and phosphorus content and their stoichiometry in spring wheat under different irrigation treatments in the Loess Plateau. Pratacultural Sci. 2020;37(9):1803–12. [Google Scholar]
40. Pan Y, Ren L, Huo J, Xiang X, Meng D, Wang Y, et al. Soil geochemistry prevails over root functional traits in controlling soil organic carbon fractions of the alpine meadow on the Qinghai-Tibet Plateau, China. Catena. 2024;237:107814. doi:10.1016/j.catena.2024.107814. [Google Scholar] [CrossRef]
41. Sarkis LF, Dutra MP, Dos Santos CA, Rodrigues Alves BJ, Urquiaga S, Guelfi D. Nitrogen fertilizers technologies as a smart strategy to mitigate nitrous oxide emissions and preserve carbon and nitrogen soil stocks in a coffee crop system. Atmos Environ: X. 2023;20:100224. doi:10.1016/j.aeaoa.2023.100224. [Google Scholar] [CrossRef]
42. Xie J, Evgenia B, Zhang Y, Wan Y, Hu Q, Zhang C, et al. Substituting nitrogen and phosphorus fertilizer with optimal amount of crop straw improves rice grain yield, nutrient use efficiency and soil carbon sequestration. J Integr Agr. 2022;21:3345–55. doi:10.1016/j.jia.2022.08.059. [Google Scholar] [CrossRef]
43. Guo T, Yao X, Wu K, Guo A, Yao Y. Response of the rhizosphere soil microbial diversity to different nitrogen and phosphorus application rates in a hulless barley and pea mixed-cropping system. Appl Soil Ecol. 2024;195:105262. doi:10.1016/j.apsoil.2023.105262. [Google Scholar] [CrossRef]
44. Xia H, Riaz M, Zhang M, Liu B, Li Y, El-Desouki Z, et al. Biochar-N fertilizer interaction increases N utilization efficiency by modifying soil C/N component under N fertilizer deep placement modes. Chemosphere. 2022;286:131594. doi:10.1016/j.chemosphere.2021.131594. [Google Scholar] [PubMed] [CrossRef]
45. Liu H. Effects of nitrogen deposition on soil carbon and nitrogen transformation and microbial characteristics in Stipa baicalensis Steppe. Chinese Academy of Agricultural Sciences: China; 2019. [Google Scholar]
46. Ren T, Fan P, Zuo W, Liao Z, Wang F, Wei Y, et al. Biochar-based fertilizer under drip irrigation: more conducive to improving soil carbon pool and promoting nitrogen utilization. Ecol Indic. 2023;154:110583. doi:10.1016/j.ecolind.2023.110583. [Google Scholar] [CrossRef]
47. Johns MM, Beggs RA, Crites RW. Effects of tomato cannery water forage irrigation on nitrogen and carbon in a clay loam soil. Geoderma. 2011;167–8:310–8. [Google Scholar]
48. Chen X, Feng J, Ding Z, Tang M, Zhu B. Changes in soil total, microbial and enzymatic C-N-P contents and stoichiometry with depth and latitude in forest ecosystems. Sci Total Environ. 2022;816:151583. doi:10.1016/j.scitotenv.2021.151583. [Google Scholar] [PubMed] [CrossRef]
49. Xie M, Li G, Yan L, Yuan J, Liu S, Qi X, Chang H. Stoichiometry and steady-state characteristics of plant-soil carbon, nitrogenand phosphorus under water regulation of spring wheat in loess hilly region. Agric Res Arid Areas. 2022;40:184–92. [Google Scholar]
50. Cui Y, Fang L, Deng L, Guo X, Han F, Ju W, et al. Patterns of soil microbial nutrient limitations and their roles in the variation of soil organic carbon across a precipitation gradient in an arid and semi-arid region. Sci Total Environ. 2019;658:1440–51. doi:10.1016/j.scitotenv.2018.12.289. [Google Scholar] [PubMed] [CrossRef]
51. Bouras H, Mamassi A, Devkota KP, Choukr-Allah R, Bouazzama B. Integrated effect of saline water irrigation and phosphorus fertilization practices on wheat (Triticum aestivum) growth, productivity, nutrient content and soil proprieties under dryland farming. Plant Stress. 2023;10:100295. doi:10.1016/j.stress.2023.100295. [Google Scholar] [CrossRef]
52. Wang L, Zhang G, Zhu P, Xing S, Wang C. Soil C, N and P contents and their stoichiometry as affected by typical plant communities on steep gully slopes of the Loess Plateau, China. Catena. 2022;208:105740. doi:10.1016/j.catena.2021.105740. [Google Scholar] [CrossRef]
53. Ågren GI. Stoichiometry and nutrition of plant growth in natural communities. Annu Rev Ecol Evol Syst. 2008;39:153–70. doi:10.1146/ecolsys.2008.39.issue-1. [Google Scholar] [CrossRef]
54. Wang Y, Zhai D, Cheng X. Soil nitrogen and phosphorus dynamics respond divergently to afforestation. Forest Ecol Manage. 2024;555:121705. doi:10.1016/j.foreco.2024.121705. [Google Scholar] [CrossRef]
55. Das AC, Debnath A. Effect of systemic herbicides on N2-fixing and phosphate solubilizing microorganisms in relation to availability of nitrogen and phosphorus in paddy soils of West Bengal. Chemosphere. 2006;65:1082–6. doi:10.1016/j.chemosphere.2006.02.063. [Google Scholar] [PubMed] [CrossRef]
56. Zhang Y, Wu H, Xu R, Wang Y, Chen L, Wei C. Machine learning modeling for the prediction of phosphorus and nitrogen removal efficiency and screening of crucial microorganisms in wastewater treatment plants. Sci Total Environ. 2024;907:167730. doi:10.1016/j.scitotenv.2023.167730. [Google Scholar] [PubMed] [CrossRef]
57. Sinsabaugh RL, Lauber CL, Weintraub MN, Ahmed B, Allison SD, Crenshaw C, et al. Stoichiometry of soil enzyme activity at global scale. Ecol Lett. 2008;11:1252–64. doi:10.1111/j.1461-0248.2008.01245.x. [Google Scholar] [PubMed] [CrossRef]
58. Vargas R, Collins SL, Thomey ML, Johnson JE, Brown RF, Natvig DO, et al. Precipitation variability and fire influence the temporal dynamics of soil CO2 efflux in an arid grassland. Global Change Biol. 2012;18:1401–11. doi:10.1111/j.1365-2486.2011.02628.x. [Google Scholar] [CrossRef]
59. Baldrian P, Merhautová V, Petránková M, Cajthaml T, Šnajdr J. Distribution of microbial biomass and activity of extracellular enzymes in a hardwood forest soil reflect soil moisture content. Appl Soil Ecol. 2010;46:177–82. doi:10.1016/j.apsoil.2010.08.013. [Google Scholar] [CrossRef]
60. Wang J. Effect of mulched drip irrigation on crop root-zone soil microenvironment and crop growth in plastic greenhouse. Northwest A&F University: China; 2017. [Google Scholar]
61. Zhang X, Dippold MA, Kuzyakov Y, Razavi BS. Spatial pattern of enzyme activities depends on root exudate composition. Soil Biol Biochem. 2019;133:83–93. doi:10.1016/j.soilbio.2019.02.010. [Google Scholar] [CrossRef]
62. Zhou S, Zhang M, Zhang K, Yang X, He D, Yin J, et al. Effects of reduced nitrogen and suitable soil moisture on wheat (Triticum aestivum L.) rhizosphere soil microbiological, biochemical properties and yield in the Huanghuai Plain, China. J Integr Agr. 2020;19:234–50. doi:10.1016/S2095-3119(19)62697-3. [Google Scholar] [CrossRef]
63. De Mastro F, Brunetti G, Traversa A, Blagodatskaya E. Fertilization promotes microbial growth and minimum tillage increases nutrient-acquiring enzyme activities in a semiarid agro-ecosystem. Appl Soil Ecol. 2022;177:104529. doi:10.1016/j.apsoil.2022.104529. [Google Scholar] [CrossRef]
64. Yang Y, Liang C, Wang Y, Cheng H, An S, Chang SX. Soil extracellular enzyme stoichiometry reflects the shift from P-to N-limitation of microorganisms with grassland restoration. Soil Biol Biochem. 2020;149:107928. doi:10.1016/j.soilbio.2020.107928. [Google Scholar] [CrossRef]
65. Sinsabaugh RL, Hill BH, Follstad SJ. Ecoenzymatic stoichiometry of microbial organic nutrient acquisition in soil and sediment. Nature. 2009;462:795–8. doi:10.1038/nature08632. [Google Scholar] [PubMed] [CrossRef]
66. Bell C, Carrillo Y, Boot CM, Rocca JD, Pendall E, Wallenstein MD. Rhizosphere stoichiometry: are C : N : P ratios of plants, soils, and enzymes conserved at the plant species-level? New Phytol. 2014;201:505–17. doi:10.1111/nph.2013.201.issue-2. [Google Scholar] [CrossRef]
67. Liu H, Duan A, Li F, Sun J, Wang Y, Sun C. Drip irrigation scheduling for tomato grown in solar greenhouse based on pan evaporation in north china plain. J Integr Agr. 2013;12:520–31. doi:10.1016/S2095-3119(13)60253-1. [Google Scholar] [CrossRef]
68. Patanè C, Tringali S, Sortino O. Effects of deficit irrigation on biomass, yield, water productivity and fruit quality of processing tomato under semi-arid Mediterranean climate conditions. Sci Hortic. 2011;129:590–6. doi:10.1016/j.scienta.2011.04.030. [Google Scholar] [CrossRef]
69. Zhang MZ, Wang L, Wang H, Xiao N, Liu JF, Liu H, et al. Exploration of water-saving and high-yield irrigation model for tomato under microsprinkler irrigation with plastic film in a greenhouse based on spatial analysis. J Sensors. 2022;2022:1–12. doi:10.1155/2022/3452727. [Google Scholar] [CrossRef]
70. Yalin D, Schwartz A, Tarchitzky J, Shenker M. Soil oxygen and water dynamics underlying hypoxic conditions in the root-zone of avocado irrigated with treated wastewater in clay soil. Soil Tillage Res. 2021;212:105039. doi:10.1016/j.still.2021.105039. [Google Scholar] [CrossRef]
71. Ouyang Z, Tian J, Yan X, Shen H. Effects of different concentrations of dissolved oxygen on the growth, photosynthesis, yield and quality of greenhouse tomatoes and changes in soil microorganisms. Agr Water Manage. 2021;245:1–9. [Google Scholar]
72. Shang Z, Cai H, Chen H, Sun Y, Li L, Zhu Y, et al. Effect of water-fertilizer-gas coupling on soil N2O emission and yield in greenhouse tomato. Environ Sci. 2020;6(41):1–18. doi:10.13227/j.hjkx.201910056. [Google Scholar] [PubMed] [CrossRef]
73. Agbna GHD, Dongli S, Zhipeng L, Elshaikh NA, Guangcheng S, Timm LC. Effects of deficit irrigation and biochar addition on the growth, yield, and quality of tomato. Sci Hortic. 2017;222:90–101. doi:10.1016/j.scienta.2017.05.004. [Google Scholar] [CrossRef]
74. Yang F, Wu P, Zhang L, Wei Y, Tong X, Wang Z. Effects of subsurface irrigation types on root distribution, leaf photosynthetic characteristics, and yield of greenhouse tomato. Sci Hortic. 2024;328:112883. doi:10.1016/j.scienta.2024.112883. [Google Scholar] [CrossRef]
75. Zhu Y, Cai H, Song L, Wang X, Shang Z, Sun Y. Aerated irrigation of different irrigation levels and subsurface dripper depths affects fruit yield, quality and water use efficiency of greenhouse tomato. Sustainability. 2020;12:2703. doi:10.3390/su12072703. [Google Scholar] [CrossRef]
76. Hebbar SS, Ramachandrappa BK, Nanjappa HV, Prabhakar M. Studies on NPK drip fertigation in field grown tomato (Lycopersicon esculentum Mill.). Eur J Agron. 2004;21:117–27. doi:10.1016/S1161-0301(03)00091-1. [Google Scholar] [CrossRef]
77. Wu W, Lin Z, Zhu X, Li G, Zhang W, Chen Y, et al. Improved tomato yield and quality by altering soil physicochemical properties and nitrification processes in the combined use of organic-inorganic fertilizers. Eur J Soil Biol. 2022;109:103384. doi:10.1016/j.ejsobi.2022.103384. [Google Scholar] [CrossRef]
78. Liu J, Hu T, Feng P, Yao D, Gao F, Hong X. Effect of potassium fertilization during fruit development on tomato quality, potassium uptake, water and potassium use efficiency under deficit irrigation regime. Agr Water Manage. 2021;250:106831. doi:10.1016/j.agwat.2021.106831. [Google Scholar] [CrossRef]
79. Zhao W, Ma F, Cao W, Ma FF, Han L. Effects of water and fertilizer coupling on the yield and quality of tomatoes. Trans Chin Soc Agric Eng. 2022;38:95–101 (In Chinese). [Google Scholar]
80. Wang H, Qu Y, Wen Z, Cheng M, Zhang F, Fan J, et al. Interactive effects of irrigation and N fertilization management on fruit yield, quality and water-N productivity of greenhouse cherry tomato. Sci Hortic. 2024;328:112895. doi:10.1016/j.scienta.2024.112895. [Google Scholar] [CrossRef]
Cite This Article
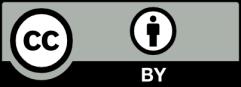
This work is licensed under a Creative Commons Attribution 4.0 International License , which permits unrestricted use, distribution, and reproduction in any medium, provided the original work is properly cited.