Open Access
ARTICLE
Phytochemical and Pharmacological Study on the Dry Extract of Matricaria discoidea DC. herb and Its Amino Acids Preparations
1 Institute of Pharmacy, Faculty of Medicine, University of Tartu, Tartu, 50411, Estonia
2 Department of Pharmacognosy and Nutritionology, National University of Pharmacy, Kharkiv, 61002, Ukraine
3 Institute of Pharmaceutical Technologies, Lithuanian University of Health Sciences, Kaunas, 44307, Lithuania
4 Department of Clinical Pharmacology, Institute for Continuing Education of Pharmacy Professionals, National University of Pharmacy, Kharkiv, 61002, Ukraine
* Corresponding Authors: Oleh Koshovyi. Email: ; Ain Raal. Email:
Phyton-International Journal of Experimental Botany 2024, 93(11), 2909-2925. https://doi.org/10.32604/phyton.2024.056536
Received 24 July 2024; Accepted 17 October 2024; Issue published 30 November 2024
Abstract
Pineappleweed (Matricaria discoidea DC., Asteraceae) herb is an essential oil containing raw material with spasmolytic and anti-inflammatory activity. It is also rich in phenolics, which may be used in pharmaceutical practice. This study aimed to investigate the phenolic and amino acid composition and the hyporific and analgesic effects of the M. discoidea aqueous-ethanolic extract and its amino acid modifications. In addition, we developed a polyethylene oxide gel formulation with M. discoidea extracts for the 3D-printed oral solid dosage preparations. In M. discoidea extracts, 16 phenolic substances and 14 amino acids were established. The extract and its amino acid preparations revealed both analgesic and soporific activity in the studies with rodents. Amino acids enhanced these effects. Arginine, β-alanine, glycine, valine, and lysine potentiated the analgesic activity by 27%–64%, while β-alanine, glycine, and valine increased the soporific activity by 136%, 176%, and 129%, respectively. The composition of the polyethylene oxide (PEO) printing gel with the M. discoidea extract was developed, and its printability was confirmed. The proposed 3D-printed medicines could be implemented for sleep disorders and painful conditions.Keywords
Chamomiles have been widely used in folk and traditional medicine. German chamomile (Matricaria chamomilla L.) is very common in the European pharmaceutical practice since it is easily cultivated [1,2]. However, there is also an increasing interest in the other species of the genus Chamomilla, such as pineappleweed (Matricaria discoidea DC.) [3,4].
Previously, we found a total of 44 terpenoids in M. discoidea essential oil, and the following three terpenoids were the predominant ones: farnesene, myrcene, and geranyl isovalerate [4,5]. Among phenolic compounds chlorogenic and ferulic acids, quercetin, apigenin, and luteolin glycosides were found [4,6]. Unlike M. chamomilla, M. discoidea has been studied less from both a phytochemical and a pharmacological point of view. To date, there are only a limited number of scientific papers on M. discoidea published in the state-of-the-art literature [4,7–11].
Recently, we found 16 phenolics in the aqueous-ethanolic extract of M. discoidea herb, and the present dry extract showed a promising analgesic and soporific activity [4]. However, both analgesic and soporific activities were lower than those reported with the corresponding extract of M. chamomilla [12]. It is believed that the chamomile effect on sleep results from its binding to gamma-aminobutyric acid (GABA) and benzodiazepine receptors [13]. The antioxidant properties of chamomile can also impact its improvement in sleep since chronic oxidative stress reportedly disrupts key mechanisms in regulating circadian rhythms and sleep homeostasis [14]. Such pharmacological activities can be improved by pharmaceutical formulation development approaches. The well-known strategy for improving biologically active substance (BAS) pharmacokinetics is the conjugation with amino acids [15,16]. In our previous study, we showed that Leonurus cardiaca L. tincture, in combination with amino acids, additionally showed a promising anxiolytic activity [17]. The combination of perindopril with arginine improves its absorption rate and stability and reduces its side effects [15]. Conjugation of acyclovir and valine resulted in the creation of valacyclovir, which has higher oral bioavailability and increases systemic plasma levels of acyclovir [16]. This mechanism of enhancement is not fully understood and studied, but improvements in pharmacokinetics are obvious. Considering these, it would be a promising approach to modify the M. discoidea dry extract [4] with amino acids to enhance its analgesic and soporific activity.
To further practical use of plant extracts in medical practice, it’s very important to develop a dosage form with them. Even though they usually have a positive safety profile. Only a limited number of oral solid dosage forms have been developed for such preparations. The oral dosage form of choice for the M. discoidea dry extract combined with amino acids would be the preparation that is convenient to use (administered orally), easy to prepare, and stable under established storage conditions. Currently, the modern trend is to use 3D printing technologies for preparing such dosage forms. Thus, It is topical to develop 3D-printed dosage forms with the most promising M. discoidea dry extract, which is suitable for oral administration.
The aim of this research was to carry out a phytochemical and pharmacological study (soporific and analgesic effects) of the M. discoidea extract and its amino acid modifications and to develop 3D-printed oral dosage forms with the present dry extract with potential uses in the pharmaceutical practice.
The M. discoidea herb (1.0 kg) was harvested from wild nature during the flowering time in July 2020 by the Veskijärv lake (58°16′30″N, 26°31′32″E), Nõo municipality, Tartumaa county, Estonia. The flowering tops were used, thick lignified stems were thrown away. The herb was dried in a well-ventilated room at 22 ± 2°C (relative humidity, RH 21%), and then packed in paper packages. The plant was identified by Prof. Ain Raal [18]. The herbarium #Ast/Mat/D13 of M. discoidea is kept in the Institute of Pharmacy, University of Tartu, Tartu, Estonia.
A total of 500.0 g of chopped M. discoidea dried flowering tops of the herb was macerated overnight with 70% aqueous ethanol (2500 mL) at 22 ± 2°C; after that, it was repeated twice with 1000.0 mL of the same solvent. The combined extract was carefully filtrated. To obtain amino acids preparations of the extract, extract’s portions of 300 mL were mixed with amino acids: arginine (Arg, 2.32 g), phenylalanine (Phe, 2.19 g), β-alanine (β-Ala, 1.17 g), glycine (Gly, 1.00 g), valine (Val, 1.18 g) and lysine (Lys, 1.92 g), and infused overnight at a room temperature. The M. discoidea herb extract and its preparations were evaporated in Buchi Rotavapor R-300 (Buchi Labortechnik AG, Flawil, Switzerland) using the following process conditions: rotation 80 rpm, vacuum 150 mbar and the temperature in a heating bath 80°C). Then, the soft extract was dried in a lyophilizer SCANVAC COOLSAFE 55-4 Pro (LaboGene ApS, Lillerød, Denmark). The dry M. discoidea herb extract (Pw) and its modifications with glycine (Pw-Gly), lysine (Pw-Lys), β-alanine (Pw-β-Ala), phenylalanine (Pw-Phe), valine (Pw-Val) and arginine (Pw-Arg) were obtained as described above.
The content of hydroxycinnamic acids, flavonoids, and total phenolic compounds in the M. discoidea herb extracts was determined with Shimadzu UV-1800 spectrophotometer (Shimadzu Corporation, Kyoto, Japan). Flavonoids were measured after forming a complex with aluminium chloride at 417 nm in the calculation to rutin [4,19]. The assay of hydroxycinnamic acids was carried out after reaction with sodium molybdate and sodium nitrite at the wave-length of 525 nm in the calculation to chlorogenic acid [19]. The total phenolic compounds were established by using the calculation of gallic acid at the wavelength of 270 nm [12]. The measurement was performed three times.
The phenolic compounds of the pineappleweed herb extracts have been determined with a UPLC-MS/MS (Acquity H-class UPLC system (Waters, Milford, MA, USA)), using a YMC Triart C18 (100 mm × 2.0 mm, A 1.9 µm) column at 40°C for compound separation. The flow rate of a mobile phase was 0.5 mL/min, and the mobile phase was eluent A (formic acid 0.1%) and eluent B (MS-grade acetonitrile). From 0 to 1 min, 95% of eluent A was constantly flowing; between 1 and 5 min, there was a linear increase of eluent B to 30%; from 5 to 7 min, increase of up to 50%; and from 7.5 to 8 min, the column was washed with 100% eluent B; after 8.1 min, it was returned to initial conditions. The analysis time was 10 min. Mass spectrometer (Xevo, Waters, Milford, MA, USA) was used to get mass spectrometric data. Such parameters were used: the capillary voltage 2 kV, cone voltage at 30 V, nitrogen as a desolvation gas at 400°C and the flow rate 700 L/h, the nitrogen flow at 20 L/h. The ion source temperature was 150°C. Analytical grade standards (MS/MS data, retention times) were used for identification of phenolics. Standard dilution methods and linear regression fit and were utilized to quantify phenolics [20,21].
2.5 UPLC-MS/MS Method for Amino Acids
The content of amino acids in the M. discoidea herb extracts was determined on Acquity H-class UPLC system (Waters, Milford, MA, USA), using a BEH Amide (150 mm × 2.1 mm, 1.7 µm) column (Waters, Milford, MA, USA) at 25°C. A total of 1 µL was injected. The mobile phase was eluent A (10 mmol aqueous ammonium formate with 0.125% formic acid) and eluent B (acetonitrile). The flow rate of the mobile phase was 0.6 mL/min. The gradient elution method was used as follows: 0 min to 1 min., 95% eluent B; 1–3.9 min, 70% eluent B; 3.9–5.1 min, 30% eluent B; 5.1–6.4 min, the column was flushed with 70% of eluent A; at 6.5 min it was returned to initial conditions. The period of analysis was 10 min. Mass spectrometric data was obtained with Xevo TQD mass spectrometer (Waters, Milford, MA, USA) under the following conditions: positive electrospray ionization was +3.5 kV, cone voltage 30 V, ion source temperature–120°C, gas flow at 800 L/h at 400°C. For identifying amino acids in the pineappleweed herb extracts, the corresponding amino acids were utilized to compare their retention times and MS/MS data with the standards. The standard dilution method was used to assay amino acids [22,23].
The soporific and analgesic effects of M. discoidea herb extracts were studied in vivo using rats and mice models according to the international rules [24] and other common laws [25–27]. The present study was approved by the protocol No. 4 of Bioethics Commission (03 October 2023) of the National University of Pharmacy, Kharkiv, Ukraine.
The analgesic effect of M. discoidea extracts and its amino acids preparations was studied in vivo. Non-linear white mice of 20–40 g, which were born and bred at the vivarium of the National University of Pharmacy (Kharkiv, Ukraine), were used in the experiment. Acetaminophen (paracetamol-Zdorovye, 500 mg capsules, pharmaceutical company “Zdorovye”, Kharkiv, Ukraine) was used as a reference medicine [28].
The mice were not fed for 2 h prior to the study. Totally 23 groups of 6 mice were randomly selected: Group 1—the intact group of animals, which were given 1 mL NaCl 0.9% per 100 g of animal weight; Group 2–4—the mice, which were given the extract Pw (100, 50 and 25 mg/kg accordingly); Group 5–7—the animals, which were given the extract Pw-Arg (100, 50 and 25 mg/kg accordingly); Group 8–10—the animals, which were given the extract Pw-Phe (100, 50 and 25 mg/kg accordingly); Group 11–13—the animals, which were given the extract Pw-β-Ala (100, 50 and 25 mg/kg accordingly); Group 14–16—the animals, which were given the extract Pw-Gly (100, 50 and 25 mg/kg accordingly); Group 17–19—the animals, which were given the extract Pw-Val (100, 50 and 25 mg/kg accordingly); Group 20–22—the animals, which were given the extract Pw-Lys (100, 50 and 25 mg/kg accordingly); and Group 23—control group, which were given acetaminophen (50 mg/kg).
The extracts were taken intragastrically, the mice were placed on a hot plate (55°C). The stay of the individual animal did not exceed 60 s on the hot plate. An indicator of pain sensitivity was the time, when the protective reflexes started. The test was repeated regularly at 0.5, 1, 2, 3, and 4 h. The analgesic activity was detected by increasing the latent period of response in comparison to the intact animals, the control group and the group of animals, which were given the extract Pw [29,30].
The pineappleweed extracts soporific activity research was conducted with white rats (190–280 g). Sodium thiopental lyophilizate (solution for injection, PLC “Kiivmedpreparat”, Kyiv, Ukraine) and “Valerian syrup AN NATU-REL” syrup (LLC Beauty and Health, Kharkiv, Ukraine) were used as reference medicines [28].
Totally 24 groups of 6 mice were randomly selected: Group 1—intact control, included animals, which were given 1 mL of NaCl 0.9% per 100 g of rat weight; Group 2–4—the animals, which were given the extract Pw (100, 50 and 25 mg/kg accordingly); Group 5–7—the animals, which were given the extract Pw-Arg (100, 50 and 25 mg/kg accordingly); Group 8–10—the animals, which were given the extract Pw-Phe (100, 50 and 25 mg/kg accordingly); Group 11–13—the animals, which were given the extract Pw-β-Ala (100, 50 and 25 mg/kg accordingly); Group 14–16—the animals, which were given the extract Pw-Gly (100, 50 and 25 mg/kg accordingly); Group 17–19—the animals, which were given the extract Pw-Val (100, 50 and 25 mg/kg accordingly); Group 20–22—the animals, which were given the extract Pw-Lys (100, 50 and 25 mg/kg accordingly); Group 23—control group, the animals which were given sodium thiopental (40 mg/kg); and Group 24—control group, the rats which were given valerian syrup (2.14 mg/kg). The extracts, reference medicines and sodium thiopental were administered via oral gavage. Thiopental was administered 45 min after administration of the extracts’ solutions or reference medicines. Sleep period was measured after sleep induction, as the time of rats perform a righting reflex after waking [31,32].
2.7 3D Printing of M. discoidea Herb Extracts
For the semi-solid extrusion (SSE) 3D printing of M. discoidea herb extract, a 12% aqueous polyethylene oxide (PEO) (MW approx. 900,000, Sigma-Aldrich, St. Louis, MO, USA; Laborat GmbH, Hamburg, Germany) gel [33] was utilized as a carrier polymer printing gel. The herb extract (0.5, 1.0, and 1.5 g) and Tween 80 (Laborat GmbH, Hamburg, Germany) (0.5 g) were mixed with 12% PEO gel. The surface-active agent (Tween 80) was added to enhance the homogeneity of a printing gel and to enhance the extract’s release from the 3D-printed preparations.
The gel viscosity was determined at 22 ± 2°C with a rheometer (Physica MCR 101, Anton Paar, Austria).
A bench-top 3D printing system (Hyrel 3D, Norcross, GA, USA) equipped with the Repetrel, Rev3.083_K control software was used for SSE 3D printing the aqueous PEO gels loaded with the pineappleweed herb extract. The printing head speed with a blunt needle (Gauge, 21G) was 0.5 mm/s at 22 ± 2°C. The syringe content and a printed plate were not heated. Grid lattices (30 mm × 30 mm × 0.5 mm, total 6 layers) and round scaffolds (20 mm, total 5 layers) were used to verify printability of the gels. These printed herb extract preparations were made with FreeCAD software (vers. 0.19/release date 2021) [34]. The weight and practical area of the lattices were determined to assess the 3D printability of the gel formulations studied. The theoretical surface area of the 3D-printed lattice model was 324 mm2. The ratio between the practical and theoretical areas was calculated, and this value was used to indicate the quality of the 3D-printing process [33]. The photos were examined with ImageJ software (National Institute of Health, Bethesda, MD, USA, version 1.51k). The weight of the scaffolds was determined with Scaltec SBC 33 (Scaltec, Goettingen, Germany).
The pharmacological data are shown as the average of 6 measurements ± standard deviation (SD) (a Student t-test). The level of statistically significant difference was p < 0.05. A total of three parallel measurements were carried out for the phytochemical assay. The data are shown as the mean values ± SD [17,35].
The pineappleweed herb extract and its modifications are brown-greenish powders with a characteristic smell. When stored, the extracts turn into a viscous soft mass. Their loss of drying [17] ranged 4.4%–4.8%.
The identified phenolics of the pineappleweed herb extract and its preparations are resulted in Table 1. 7 hydroxycinnamic acids, 2 phenolic acids and 7 flavonoids were established by a UPLC-MS/MS method. Hydrocinnamic acids, flavonoids and total phenolics were assayed by spectrophotometry and these results s are presented in Table 1.
The amino acids in the pineappleweed herb extracts were studied by a UPLC-MS/MS method (Table 2).
The results on the extracts’ analgesic activity in the hot-plate test of mice, are summarized in Table 3. The herb extracts studied changed the stimulus-response of mice, and some amino acids potentiated this activity.
The results on the soporific activity of the dry M. discoidea herb extracts in rats are summarized in Table 4. The pineappleweed extracts changed the period of sleeping by typically prolonging it. The results of an average sleep duration were compared with the corresponding results of the control groups and the animal groups having the extract Pw. As shown in Table 4, some amino acids had a positive influence on the soporific effect in rats.
3.3 Novel 3D-Printed Oral Dosage Forms for Dry M. discoidea Herb Extract
For developing oral dosage forms for dry M. discoidea herb extract SSE 3D printing has been used. This shows the prospects for further implementing the developed extracts in the medical and pharmaceutical practice.
The gels loaded with a dry M. discoidea herb extract were yellow-brown-greenish viscous masses with a specific odor. The gels’ viscosity was measured at 0.01 RPM speed and 0.021/s shear rate at 22 ± 2°C, and the results are shown in Table 5.
The standard-size square- and round-shaped 3D-printed scaffolds were printed to verify the probability of the PEO gels loaded with the pineappleweed extract. (Fig. 1). The surface area, average Spractical/S theoretical ratio and their average mass were determined (Table 5).
Figure 1: Photographs of the 3D-printed scaffolds with the M. discoidea extract (from left to right 0.5, 1.0 and 1.5 g, accordingly)
In the dry pineappleweed extracts 16 phenolic substances were found and established. Pineapple weed and German chamomile extracts contain quite the same individual phenolic compounds but their concentrations differ [4,12]. The dominant flavonoids are luteolin and its glycoside luteolin-7-O-glucoside. Hydrocinnamic acids, such as chlorogenic, 4,5-dicaffeoylquinic, 3,5-dicaffeoylquinic and 3,4-dicaffeoylquinic acids are predominated as well. Luteolin and luteo`lin-7-O-glucoside have been revealed an anti-inflammatory effects. They are capable to blocking the interaction of pathogen-associated molecular patterns ligands, with pattern recognition receptors and to suppressing the downstream activation signals [36,37]. Luteolin-7-O-glucoside affects energy production, glucose homeostasis and lipid metabolism [38]. Luteolin shows a particular antioxidant activity and anti-ageing properties [39]. It can absorb a large amount of solar UV radiation, thus preventing oxidative damage [40]. It was also reported that luteolin shows anti-cancer activity [41]. The caffeoylquinic acids present anti-oxidation, anti-inflammation, enzyme inhibition, hepatoprotective and anti-platelet-activating factor effects [42]. Thus, the phenolics of the dry M. discoidea herb extract demonstrate prospects for implementing the extract in pharmaceutical practice.
14 amino acids (6 among them—essential ones) were established in the pineappleweed extracts. Proline is an agonist of the glycine receptor and predominated in the extracts; both non-N-methyl-D-aspartate (NMDA) (the α-amino-3-hydroxy-5-methyl-4-isoxazolepropionic acid receptor (AMPA/kainate)) and NMDA ionotropic glutamate receptors, which are glutamate receptors and predominantly Ca2+ ion channel found in neurons [43,44]. So, it is evident that proline can affect soporific activity. Alanine, isoleucine, phenylalanine serine, threonine and valine are also the predominant amino acids. The essential amino acid threonine is needed for the biosynthesis of glycine in the brain and to regulate neuro-transmission, which plays a key role in fighting against depression [45,46]. Deficit of threonine leads to fast fatigue [47].
It should be noted that the concentration of all the established phenolics is lower in the amino acid modifications of herb extract, which is logical due to the addition of an amino acid, but at the same time, the pharmacological activity of these preparations increases in some cases (Tables 4 and 5). This increase in pharmacological activity can be clarified by the potentiation of the effects of phenolics with amino acids. Amino acids certainly do have pharmacological activity, but it depends on a dose. Therapeutic doses of amino acids are generally known and available [47]. As the amino acids were used for the M. discoidea extract modification in much smaller amounts than their therapeutic doses, so they could not have caused these effects on their own. Here it is obvious that there is a synergism and increasing activity of the extract BAS, particularly phenolics. In our opinion, this is related to the formation of their conjugates with amino acids on phenolic hydroxyls. Previously, we proved that M. discoidea herb extracts have analgesic activity [4]. The main phenolics of M. discoidea herb showed COX-1,2 inhibition in molecular docking research [4]. The preparations with amino acids potentiated the M. discoidea herb extracts analgesic activity (Table 3). The results of the present study showed that preparations Pw-Arg with arginine at a dose of 25 and 50 mg/kg, Pw-β-Ala with alanine at a dose of 50 mg/kg, Pw-Gly with glycine at a dose of 50 mg/kg, Pw-Val with valine at a dose of 25 and 50 mg/kg and Pw-Lys with lysine at a dose of 25 and 50 mg/kg significantly enhanced (by 27%–64%) the Pw analgesic activity after 30 min of their administration. We also found that at 60, 120 and 180 min after the administration, the amino acid preparations increased analgesic activity, but the difference in the results between the corresponding animal groups and the Pw group was not statistically significant. At 240 min, the analgesic activity of the amino acid preparations was found to be still remaining in the mice studied. At 240 min, however, a significant increase in the analgesic effect was found only with the preparation Pw-Val with valine.
In our opinion and based on the results of the other authors [15,16], the changes in the pharmacokinetics of active phytochemical compounds of the herb extract amino acids preparations may result in their facilitated transport across tissue barriers. Karpov [15] showed that arginine, for example, improves the absorption rate and stability of perindopril, at the same time reducing side effects. The authors compared the incidence of adverse effects after the administration of perindopril tertbutylamine and perindopril arginine, and showed a decrease in the frequency of drug-related adverse events from 16.7% to 5.6%, respectively. In addition, a significant increase in the stability of actives (remaining substance in open containers) from 1% to 100%, and an increase in shelf-life from 24 to 36 months were proved. Therefore, using arginine salt of perindopril has positive effects on the pharmacokinetic properties, stability (in various climatic zones) and tolerability of the drug substance, which increases its compliance and improves the target indicators of pharmacotherapy [15]. Another example could be related to the use of acyclovir and valacyclovir. The low and inconsistent oral bioavailability of acyclovir significantly reduces its activity against Herpesviridae. Modifying acyclovir by esterification with valine results in the formation of valacyclovir. Oral administration of this API leads to significantly higher level of acyclovir in systemic plasma [16]. The mechanism of the increased oral absorption of valacyclovir is not fully understood, but most likely, it involves intestinal dipeptide transporters to speed up ester hydrolysis in the liver and small intestine. Improvements in valacyclovir pharmacokinetics resulted in improved patient convenience and clinical efficacy [16].
According to our results (Table 4), the inclusion of β-alanine in the Pw extract had a positive dose-dependent effect on sedation in rats. This indicates the high effectiveness of Pw-β-Ala extract compared to the reference drugs, thiopental (103.23%) and valerian syrups (109.99%), and to the original extract Pw (135.96%). The increase in sedative effect with the addition of alanine may be due to several reasons. Firstly, alanine is a neurotransmitter involved in the inhibitory processes in the central nervous system (CNS). Secondly, alanine may enhance the activity of gamma-aminobutyric acid (GABA), which contributes to increased sedation. Thirdly, alanine may improve the bioavailability and metabolic stability of the extract, thus resulting in a longer-lasting and more pronounced sedative effect [48,49]. The significant increase in sleep duration with the administration of Pw-β-Ala can also be explained by the fact that the combination of alanine and the extract components can have a synergistic effect. Then, the total effect of the combination exceeds the separate effect of each individual component.
The maximum pharmacological effect of Pw-Gly extract was observed in rats at 100 mg/kg. The duration of sleep was 141.1 ± 11.6 min, which corresponds to 136.8% compared to the animal group that received thiopental, and 175.7% compared to the group that received Pw extract. Compared to the animal group given Valerian syrup, the difference was 145.7%. The pronounced sedative effect of the Pw-Glyextract may be caused by conjugation of phenolics and glycine, which is an inhibitory neurotransmitter in the CNS. It acts on the glycine receptors found in the spinal cord and brain stem, and also on NMDA receptors in the brain by enhancing inhibitory processes and reducing neuronal excitability. This leads to a calming and sedative effect [50,51]. Like alanine, glycine can enhance the effects of other extract components. It is evident that glycine acts synergistically by improving the metabolism and bioavailability of the extract, thus increasing the effectiveness of its components.
The Pw-Val extract significantly increases the duration of sleep in rats, especially at a dose of 100 mg/kg (by 128.7% in comparison with the extract Pw). The hypnotic effect of Pw-Val extract was comparable to that obtained with thiopental and surpassed the effect of the Pw extract. Valine plays an important role in the body as a building block for proteins and is involved in various metabolic processes. Valine itself does not have a direct hypnotic effect. Theoretically, its hypnotic effect may be due to an indirect effect on overall metabolic balance and due to the support of neurotransmitter synthesis through its participation in protein metabolism [52,53]. However, we believe that the most obvious reason for the increase in sleep duration in rats administered with Pw-Val is that valine (added in the M. discoidea extract) promoted a synergistic effect. This means that the combination of active ingredients acts together to enhance the overall sedative effect, which in turn is associated with increased bioavailability of active compounds or interaction with various receptors in the brain. Previously the molecular docking analysis of phenolics of the M. discoidea extract showed their NMDA receptor antagonism [4]. In the same way, phenolics of M. chamomilla L. flowers extract, which are quite similar with M. discoidea ones, demonstrated N-methyl-D-aspartate (NMDA) and γ-aminobutyric acid type A (GABAA) receptor antagonism [54]. It was also reported that apigenin and its glycosides, components of M. chamomile, are likely to reduce sleep latency via benzodiazepine receptors [55].
Therefore, the M. discoidea extracts showed different levels of effectiveness in rats. The addition of beta-alanine, glycine, and valine in the herb extract leads to a significant increase in sleep duration, while the inclusion of arginine, phenylalanine and lysine resulted in an opposite effect. The present research results suggest a dose-dependent effect of amino acids: the hypnotic effect increased with increasing the dose of amino acids, especially with the Pw-Gly and Pw-β-Ala ex-tracts. The M. discoidea extracts studied here could be found to be used as drug-therapy supporting components in soporific medicines. However, further research is needed to implement these novel agents with promising anti-inflammatory and hypnotic activity.
The PEO gels loaded with 0.5 to 1.5 g of the M. discoidea herb extract per 10 mL were feasible for SSE 3D-printing. The 3D-printed dosage forms were equal in shape and size (Fig. 1). Moreover, the 3D-printed herb extract preparations dissolved rapidly in water at 22 ± 2°C. Thus, the proposed dosage forms could be implemented as an immediate-release dosage form for oral administration. The developed gel composition with the M. discoidea herb extract, the proposed 3D printing mode and novel 3D-printed forms show further perspectives and the possibility of their use in practical pharmacy and medicine. After minor modifications, this technology can be implemented for preparing dietary supplements or pharmaceutical products with the present herb extracts.
The dry pineappleweed (M. discoidea) herb extract and its amino acid modifications were proven to have analgesic and soporific activity in an animal model (rodents). A total of 16 phenolics and 14 amino acids were determined in the pineappleweed extracts. The extract phenolics in conjugation with the amino acids enhance their efficacy. Arginine, β-alanine, glycine, valine and lysine potentiate the analgesic activity of the M. discoidea extract in mice, while beta-alanine, glycine and valine evidently improve the soporific activity in rats. The proposed novel 3D-printed forms are promising for preparing dietary supplements or pharmaceutical products with the present herb extracts.
Acknowledgement: The authors sincerely appreciate all the partners’ support for standing with Ukraine and thank all Ukrainian defenders and the armed forces of Ukraine.
Funding Statement: This research was supported by the Estonian Research Council (PRG1903) and the MSCA4Ukraine “Design and Development of 3D-Printed Medicines for Bioactive Materials of Ukrainian and Estonian Medicinal Plants Origin” (ID number 1232466).
Author Contributions: Study conception and design: Oleh Koshovyi, Valdas Jakštas, Igor Kireyev, Jyrki Heinämäki and Ain Raal; data collection: Janne Sepp, Karina Tolmacheva, Vaidotas Žvikas and Oleh Koshovyi; analysis and interpretation of results: Janne Sepp, Igor Kireyev, Vaidotas Žvikas, Oleh Koshovyi and Ain Raal; draft manuscript preparation: Oleh Koshovyi, Janne Sepp, Igor Kireyev, Jyrki Heinämäki and Ain Raal. All authors reviewed the results and approved the final version of the manuscript.
Availability of Data and Materials: The data supporting the results of this study can be obtained from the corresponding authors upon reasonable request.
Ethics Approval: The pharmacological research is approved by the Bioethics Commission of the National University of Pharmacy, Ukraine (protocol No. 4 from 03 October 2023).
Conflicts of Interest: The authors declare that they have no conflicts of interest to report regarding the present study.
References
1. Srivastava JK, Shankar E, Gupta S. Chamomile: a herbal medicine of the past with a bright future (review). Mol Med Rep. 2010;3(6):895–901. [Google Scholar] [PubMed]
2. Sah A, Naseef PP, Kuruniyan MS, Jain GK, Zakir F, Aggarwal G. A comprehensive study of therapeutic applications of chamomile. Pharmaceuticals. 2022;15:1284. doi:10.3390/ph15101284. [Google Scholar] [PubMed] [CrossRef]
3. Arak E, Raal A, Tammeorg J. Aerial parts of Matricaria matricarioides: a substitute for Matricaria recutita flowers. Farmatsiya. 1986;35:19–22. [Google Scholar]
4. Sepp J, Koshovyi O, Jakštas V, Žvikas V, Botsula I, Kireyev I, et al. Phytochemical, pharmacological, and molecular docking study of dry extracts of Matricaria discoidea DC. with analgesic and soporific activities. Biomolecules. 2024;14:361. doi:10.3390/biom14030361. [Google Scholar] [PubMed] [CrossRef]
5. Orav A, Sepp J, Kailas T, Müürisepp M, Arak E, Raal A. Composition of essential oil of aerial parts of Chamomilla suaveolens from Estonia. Nat Prod Commun. 2010;5:133–6. [Google Scholar] [PubMed]
6. Raal A, Püssa T, Sepp J, Malmiste B, Arak E. Content of phenolic compounds in aerial parts of Chamomilla suaveolens from Estonia. Nat Prod Commun. 2011;6:1107–10. [Google Scholar] [PubMed]
7. Mailänder LK, Lorenz P, Bitterling H, Stintzing FC, Daniels R, Kammerer DR. Phytochemical characterization of chamomile (Matricaria recutita L.) roots and evaluation of their antioxidant and antibacterial potential. Molecules. 2022;27:8508. doi:10.3390/molecules27238508. [Google Scholar] [PubMed] [CrossRef]
8. Géron C, Lembrechts JJ, Hamdi R, Berckmans J, Nijs I, Monty A. Phenotypic variation along urban-to-rural gradients: an attempt to disentangle the mechanisms at play using the alien species Matricaria discoidea (Asteraceae). Plant Ecol. 2022;223:1219–31. doi:10.1007/s11258-022-01269-y. [Google Scholar] [CrossRef]
9. Lopes D, Kolodziejczyk PP. Essential oil composition of pineapple-weed (Matricaria Discoidea DC.) grown in Canada. J. Essent. Oil-Bear. Plants. 2005;8:178–82. doi:10.1080/0972060X.2005.10643440. [Google Scholar] [CrossRef]
10. Prosovskyi MA, Rybalko KS, Sheichenko VI, Shchablinskii AN, Oleshko AN. Chemical composition of Matricaria matricariodes. Khimiko-Farmatsevticheskii Zhurnal. 1985;19:981–84. [Google Scholar]
11. Prosovskii MA, Oleshko GI. Phenolic compounds of Matricaria discoidea. Chem Nat Compd. 1985;21:671. doi:10.1007/BF00579084. [Google Scholar] [CrossRef]
12. Sepp J, Koshovyi O, Jakstas V, Žvikas V, Botsula I, Kireyev I, et al. Phytochemical, technological, and pharmacological study on the galenic dry extracts prepared from German chamomile (Matricaria chamomilla L.) flowers. Plants. 2024;13:350. doi:10.3390/plants13030350. [Google Scholar] [PubMed] [CrossRef]
13. Kazemi A, Shojaei-Zarghani S, Eskandarzadeh P, Hashem Hashempur M. Effects of chamomile (Matricaria chamomilla L.) on sleep: a systematic review and meta-analysis of clinical trials. Complement Ther Med. 2024;84:103071. doi:10.1016/j.ctim.2024.103071. [Google Scholar] [PubMed] [CrossRef]
14. Morris G, Stubbs B, Köhler CA, Walder K, Slyepchenko A, Berk M, et al. The putative role of oxidative stress and inflammation in the pathophysiology of sleep dysfunction across neuropsychiatric disorders: focus on chronic fatigue syndrome, bipolar disorder and multiple sclerosis. Sleep Med Rev. 2018;41:255–65. doi:10.1016/j.smrv.2018.03.007. [Google Scholar] [PubMed] [CrossRef]
15. Karpov YA. Perindopril arginine: a new ACE inhibitor salt increases therapeutic potential. Cardiovasc Ther Prev. 2008;7:64–72. [Google Scholar]
16. MacDougall C. Pharmacokinetics of valaciclovir. J Antimicrob Chemother. 2004;53:899–901. doi:10.1093/jac/dkh244. [Google Scholar] [PubMed] [CrossRef]
17. Koshovyi O, Raal A, Kireyev I, Tryshchuk N, Ilina T, Romanenko Ye, et al. Phytochemical and psychotropic research of motherwort (Leonurus cardiaca L.) modified dry extracts. Plants. 2021;10:230. doi:10.3390/plants10020230. [Google Scholar] [PubMed] [CrossRef]
18. Dobrochaeva DN, Kotov MI, Prokudin YN, Barbarich AI. Key to higher plants of Ukraine. Kyiv, Ukraine: Naukova Dumka; 1999. [Google Scholar]
19. European pharmacopoeia. 11th edStrasbourg: Council of Europe; 2022. [Google Scholar]
20. Vilkickyte G, Raudone L, Petrikaite V. Phenolic fractions from Vaccinium vitis-Idaea L. and their antioxidant and anticancer activities assessment. Antioxidants. 2020;9:1261. doi:10.3390/antiox9121261. [Google Scholar] [PubMed] [CrossRef]
21. Marzullo L, Ochkur O, Orlandini S, Renai L, Gotti R, Furlanetto S, et al. Quality by design in optimizing the extraction of (poly)phenolic compounds from Vaccinium myrtillus berries. J Chromatogr A. 2022;1677:463329. doi:10.1016/j.chroma.2022.463329. [Google Scholar] [PubMed] [CrossRef]
22. Uminska K, Gudžinskas Z, Ivanauskas L, Georgiyants V, Kozurak A, Skibytska M, et al. Amino acid profiling in wild Chamaenerion angustifolium populations applying chemometric analysis. J App Pharm Sc. 2023;13(5):171–80. [Google Scholar]
23. Demeshko OV, Kovalev SV, Komisarenko SM. Study of the amino acid composition of the leaves of Robinia pseudoacacia L. Pharmacom. 2004;4:61–4. [Google Scholar]
24. European convention for the protection of vertebrate animals used for experimental and other scientific purposes. Off J. 1986;222:31–7. [Google Scholar]
25. Council directive 2010/63/EU of 22 September 2010 on the protection of animals used for scientific purposes. Off J Eur Union. 2010;276:33–79. Available from: https://www.fao.org/faolex/results/details/en/c/LEX-FAOC098296/. [Accessed 2024]. [Google Scholar]
26. On approval of the procedure for preclinical study of medicinal products and examination of materials of preclinical study of medicinal products. Ministry of Health of Ukraine; 2009. [Google Scholar]
27. The law of Ukraine “On the protection of animals from cruel treatment” dated 12/15/2009. Available from: https://zakon.rada.gov.ua/laws/show/3447-15#Text. [Accessed 2021]. [Google Scholar]
28. Stefanov OV. Preclinical studies of drugs. Kyiv, Ukraine: Avicenna; 2001. [Google Scholar]
29. Masocha W, Kombian SB, Edafiogho IO. Evaluation of the antinociceptive activities of enaminone compounds on the formalin and hot plate tests in mice. Sci Rep. 2016;6:21582. doi:10.1038/srep21582. [Google Scholar] [PubMed] [CrossRef]
30. Inaltekin A, Kivrak Y. Evaluation of the effect of Vortioxetine on pain threshold by hot-plate test in mice. Arch Neuropsychiat. 2021;58(4):274–7. [Google Scholar]
31. Hossain MF, Talukder B, Rana MN, Tasnim R, Nipun TS, Uddin SMN, et al. In vivo sedative activity of methanolic extract of Stericulia villosa Roxb. leaves. BMC Complement Altern Med. 2016;16:398. doi:10.1186/s12906-016-1374-8. [Google Scholar] [PubMed] [CrossRef]
32. Forouzanfar F, Ghorbani A, Hosseini M, Rakhshandeh H. Hydroalcoholic extract of needles of Pinus eldarica enhances pentobarbital-induced sleep: possible involvement of GABAergic system. Avicenna J Phytomed. 2016;6:449–57. [Google Scholar] [PubMed]
33. Viidik L, Seera D, Antikainen O, Kogermann K, Heinämäki J, Laidmäe I. 3D-printability of aqueous poly(ethylene oxide) gels. Eur Polym J. 2019;120:109206. doi:10.1016/j.eurpolymj.2019.08.033. [Google Scholar] [CrossRef]
34. Juergen R, Werner M, Yorik van H. FreeCAD (Version 0.19.24291); 2001–2021. Available from http://www.freecad.org. [Accessed 2024]. [Google Scholar]
35. Lapach SN, Chubenko AV, Babich PN. Statistical methods in biomedical research using Excel. Kyiv: MORION; 2000. [Google Scholar]
36. Shen R, Ma L, Zheng Y. Anti-inflammatory effects of luteolin on acute gouty arthritis rats via TLR/MyD88/NF-κB pathway. Zhong Nan Da Xue Xue Bao Yi Xue Ban. 2020;45:115–22. [Google Scholar] [PubMed]
37. Lee JK, Kim SY, Kim YS, Lee WH, Hwang DH, Lee JY. Suppression of the TRIF-dependent signaling pathway of Toll-like receptors by luteolin. Biochem Pharmacol. 2009;77:1391–400. doi:10.1016/j.bcp.2009.01.009. [Google Scholar] [PubMed] [CrossRef]
38. Zang Y, Igarashi K, Li Y. Anti-diabetic effects of luteolin and luteolin-7-o-glucoside on KK-A y mice. Biosci Biotechnol Biochem. 2016;80:1580–86. doi:10.1080/09168451.2015.1116928. [Google Scholar] [PubMed] [CrossRef]
39. Mu J, Ma H, Chen H, Zhang X, Ye M. Luteolin prevents UVB-induced skin photoaging damage by modulating SIRT3/ROS/MAPK signaling: an in vitro and in vivo studies. Front Pharmacol. 2021;12:728261. doi:10.3389/fphar.2021.728261. [Google Scholar] [PubMed] [CrossRef]
40. Gendrisch F, Esser PR, Schempp CM, Wölfle U. Luteolin as a modulator of skin aging and inflammation. Biofactors. 2021;47:170–80. doi:10.1002/biof.v47.2. [Google Scholar] [CrossRef]
41. Caporali S, De Stefano A, Calabrese C, Giovannelli A, Pieri M, Savini I, et al. Anti-inflammatory and active biological properties of the plant-derived bioactive compounds luteolin and luteolin-7-glucoside. Nutrients. 2022;14:1155. doi:10.3390/nu14061155. [Google Scholar] [PubMed] [CrossRef]
42. Zhao Y, Zhao J, Li X, Zhou C, Sun H, Hao X, et al. Advances in caffeoylquinic acid research. Zhongguo Zhong Yao Za Zhi. 2006;31:869–74 (In Chinese). [Google Scholar] [PubMed]
43. Henzi V, Reichling DB, Helm SW, MacDermott AB. L-Proline activates glutamate and glycine receptors in cultured rat dorsal horn neurons. Mol Pharmacol. 1992;41:793–801. [Google Scholar] [PubMed]
44. Abdelnour SA, Khalil WA, Khalifa NE, Khalil FMA, Hassan MAE. L-Proline: a promising tool for boosting cryotolerance and fertilizing ability of cryopreserved sperm in animals. Anim Reprod Sci. 2024;263:107429. doi:10.1016/j.anireprosci.2024.107429. [Google Scholar] [PubMed] [CrossRef]
45. Adeva-Andany M, Souto-Adeva G, Ameneiros-Rodríguez E, Fernández-Fernández C, Donapetry-García C, Domínguez-Montero A. Insulin resistance and glycine metabolism in humans. Amino Acids. 2018;50:11–27. doi:10.1007/s00726-017-2508-0. [Google Scholar] [PubMed] [CrossRef]
46. Zhu WL, Wang SJ, Liu MM, Shi HS, Zhang RX, Liu JF, et al. Glycine site N-methyl-D-aspartate receptor antagonist 7-CTKA produces rapid antidepressant-like effects in male rats. J Psychiatry Neurosci. 2013;38(5):306–16. doi:10.1503/jpn. [Google Scholar] [CrossRef]
47. Kozlov VA. Proteinogenic acids. Cheboksary: Chuvash State Pedagogical University; 2012. [Google Scholar]
48. Duttagupta S, Krishna Roy N, Dey G. Efficacy of amino acids in sports nutrition–review of clinical evidences. Food Res Int. 2024;187:114311. doi:10.1016/j.foodres.2024.114311. [Google Scholar] [PubMed] [CrossRef]
49. Meftahi GH, Jahromi GP. Biochemical mechanisms of beneficial effects of beta-alanine supplements on cognition. Biochem Moscow. 2023;88(8):1181–90. doi:10.1134/S0006297923080114. [Google Scholar] [PubMed] [CrossRef]
50. Soh J, Raventhiran S, Lee JH, Lim ZX, Goh J, Kennedy BK, et al. The effect of glycine administration on the characteristics of physiological systems in human adults: a systematic review. GeroScience. 2023;46(1):219–39. doi:10.1007/s11357-023-00970-8. [Google Scholar] [PubMed] [CrossRef]
51. Wu X, Zhang H, Shi M, Fang S. Clinical features in antiglycine receptor antibody-related disease: a case eport and update literature review. Front Immunol. 2024;15:1387591. doi:10.3389/fimmu.2024.1387591. [Google Scholar] [PubMed] [CrossRef]
52. Sperringer JE, Addington A, Hutson SM. Branched-chain amino acids and brain metabolism. Neurochem Res. 2017;42(6):1697–709. doi:10.1007/s11064-017-2261-5. [Google Scholar] [PubMed] [CrossRef]
53. Wang C, Peng Y, Zhang Y, Xu J, Jiang S, Wang L, et al. The Biological functions and metabolic pathways of valine in swine. J Animal Sci Biotechnol. 2023;14(1):135. doi:10.1186/s40104-023-00927-z. [Google Scholar] [PubMed] [CrossRef]
54. Koshovyi O, Sepp J, Jakštas V, Žvikas V, Kireyev I, Karpun Y, et al. German chamomile (Matricaria chamomilla L.) flower extract, its amino acid preparations and 3D-printed dosage forms: phytochemical, pharmacological, technological, and molecular docking study. Int J Mol Sci. 2024;25:8292. doi:10.3390/ijms25158292. [Google Scholar] [PubMed] [CrossRef]
55. Shinomiya K, Inoue T, Utsu Y, Tokunaga S, Masuoka T, Ohmori A, et al. Hypnotic activities of chamomile and passiflora extracts in sleep-disturbed rats. Biol Pharm Bull. 2005;28(5):808–10. doi:10.1248/bpb.28.808. [Google Scholar] [PubMed] [CrossRef]
Cite This Article
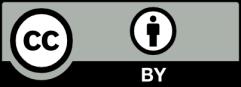
This work is licensed under a Creative Commons Attribution 4.0 International License , which permits unrestricted use, distribution, and reproduction in any medium, provided the original work is properly cited.