Open Access
ARTICLE
Genome-Wide Identification of the GST Gene Family in Loquat (Eriobotrya japonica Lindl.) and Their Expression under Cold Stress with ALA Pretreatment
1 College of Life Sciences, Fujian Agriculture and Forestry University, Fuzhou, 350002, China
2 College of Environmental and Biological Engineering, Putian University, Putian, 351100, China
3 College of JunCao Science and Ecology (College of Carbon Neutrality), Fujian Agriculture and Forestry University, Fuzhou, 350002, China
* Corresponding Authors: Shoukai Lin. Email: ; Jincheng Wu. Email:
# These authors contributed equally to this work
(This article belongs to the Special Issue: Influence of Biotic and Abiotic Stresses Signals on Plants and their Performance at Different Environments)
Phyton-International Journal of Experimental Botany 2024, 93(11), 2715-2735. https://doi.org/10.32604/phyton.2024.056484
Received 24 July 2024; Accepted 27 September 2024; Issue published 30 November 2024
Abstract
Loquat (Eriobotrya japonica Lindl.), a rare fruit native to China, has a long history of cultivation in China. Low temperature is the key factor restricting loquat growth and severely affects yield. Low temperature induces the regeneration and metabolism of reduced glutathione (GSH) to alleviate stress damage via the participation of glutathione S-transferases (GSTs) in plants. In this study, 16 GSTs were identified from the loquat genome according to their protein sequence similarity with Arabidopsis GSTs. On the basis of domain characteristics and phylogenetic analysis of AtGSTs, these EjGSTs can be divided into 4 subclasses: Phi, Theta, Tau and Zeta. The basic properties, subcellular localization, structures, motifs, chromosomal distribution and collinearity of the EjGST proteins or genes were further analyzed. Tandem and segmental gene duplications play pivotal roles in EjGST expansion. Cis-elements that respond to various hormones and stresses, especially those associated with low-temperature responsiveness, were predicted to be present in the promoters of EjGSTs. Moreover, analysis of gene expression profiles revealed that 9 of 16 EjGSTs may be involved in the low-temperature responsiveness of loquat leaves. In agriculture, 5-aminolevulinic acid (ALA), a potential multifunctional plant growth regulator, can improve the stress response of plants. Among the 9 low-temperature-responsive EjGSTs, the expression of EjGSTU1 and EjGSTF1 significantly differed under cold stress in response to exogenous 5-aminolevulinic acid (ALA) pretreatment. The remarkable increase in GST activity and GSH/GSSG ratio reflected the increase in the cold response ability of loquat plants caused by exogenous ALA, thereby alleviating H2O2 accumulation and membrane lipid preoxidation. Overall, this study provides an initial exploration of the cold tolerance function of GSTs in loquat, offering a theoretical foundation for the development of cold-resistant loquat cultivars and new antifreeze agents.Keywords
Plant glutathione S-transferases (GSTs), which are homo or heterodimer superfamily enzymes with two subunits, play crucial roles in the regeneration and metabolism of reduced glutathione (GSH). The structure of plant GSTs is highly conserved, with a GSH binding site (G-site) at the N-terminus of its subunit, which has high substrate specificity, and some GSTs have a GSH secondary binding site near the G-site [1,2]. There is an electrophilic hydrophobic complex binding site (H-site) at the C-terminus of its subunit [3]. Therefore, GSTs can catalyze the covalent binding of GSH with electrophilic and hydrophobic complexes to form conjugated hydrophilic substances, which can be further isolated in vacuoles or transferred to apoplasts to degrade harmful endo-and exogenous substances [4]. GSTs also possess GSH-Px activity to catalyze the reduction of H2O2 and organic hydroperoxide [5,6], thereby reducing cell damage. Plant GSTs have biological functions such as detoxification, antioxidation and metabolic regulation [7] and play many indispensable roles in the stress response, signal transduction and primary/secondary metabolism [8,9]. Notably, GSTs play a positive role in the plant response to abiotic stress. In particular, cold stress can induce the expression of certain GSTs in plants and reduce the level of reactive oxygen species (ROS), thus alleviating the damage caused by stress [10–13]. Relevant studies have shown that OsGSTL2 enhances cold tolerance by enhancing the antioxidant system in Arabidopsis thaliana [14]. Yang et al. [15] heterologously expressed JrGSTTau1 in tobacco and reported that, under cold stress conditions, the fresh weight, initial root length, total chlorophyll content, and GST and SOD activities of transgenic plants were significantly greater than those wild-type plants. Carmona et al. [16] reported that CsGSTs regulate the cold tolerance of blood orange (Citrus sinensis) by participating in anthocyanin transport.
Different plant species and varieties have different cold tolerances, mainly depending on their different genetic backgrounds, but plant cold tolerance could be improved in certain ways. 5-Aminolevulinic acid (ALA), also known as δ-aminolevulinic acid, is a naturally occurring, functional non-protein amino acid in plants. It serves as a vital precursor for the biosynthesis of tetrapyrrole compounds, including vitamin B12, chlorophyll, and heme, which are ubiquitous in living organisms [17,18]. ALA has a regulatory effect similar to that of plant hormones such as melatonin, abscisic acid and salicylic acid [19–21]. In agriculture, ALA acts as a natural, non-toxic, and environmentally friendly multifunctional plant growth regulator. It can increase plant growth and crop yields while improving plant tolerance to various abiotic and biotic stresses, such as weak or strong light, low or high temperatures, drought, waterlogging, salinity, alkalinity, heavy metal pollution, and chemical pesticide toxicity [22,23]. This highlights the application potential of ALA in agricultural production. Many studies have shown that exogenous ALA treatment can improve various physiological and biochemical characteristics of plants under low-temperature stress, including increasing their photosynthetic capacity, improving nutrient absorption, regulating defense systems and inducing endogenous hormone accumulation, thereby increasing crop yield and enhancing resistance [24–26]. Moreover, 5-ALA, as one of the most prominent gene fertilizers, can increase the expression of various genes, improving crop resistance, yield growth and quality. Studies have demonstrated that exogenous 5-ALA can alter the expression of related genes in plants under cold stress, helping to maintain metabolic homeostasis and ensure an adequate energy supply [25]. Chen et al. [27] reported that exogenous ALA participated in mediating the stomatal opening of plant leaves under drought stress by influencing the PTPA-PP2AC-SnRK2.6 regulatory module.
As a subtropical fruit tree, loquat can grow at 20–35° north latitude and 20–35° south latitude. To date, Eriobotrya plants have been widely cultivated in more than 30 regions, including China, the United States, India, Australia and Turkey. Eriobotrya plants are abundant, and approximately 20 of these species are native to China. However, only common loquat (E. japonica Lindl.) is widely cultivated in practice. As a typical subtropical evergreen fruit tree in China, loquat is rich in germplasm resources, covering more than 350 cultivars, strains and excellent strains. In China, the main producing areas of loquats include the southeast coast, the South China coast, central China and the southwest plateau, and loquats originating in China have spread overseas in different ways, forming foreign producing areas [28–31]. The distribution of loquat-producing areas is affected by many factors, among which low temperature is the main abiotic stress factor. Most of the loquat-producing areas in Southeast China and South China coastal areas are located in subtropical and tropical fringe areas, and the climate is warm and humid, so the cultivated loquat varieties are mostly tropical varieties with relatively poor cold tolerance [29,32]. According to previous research, the cold tolerance limits of the tree body, flower organ and young fruit are −12°C, −6°C and −3°C, respectively. In the case of low temperatures in winter, E. japonica Lindl. cv. ‘Zaozhong No. 6’ cultivated in high-elevation mountainous areas may suffer severe frost damage and may even have no harvest [33,34]. Therefore, improving the cold tolerance of loquat plants is highly important for promoting the development of loquat production.
The plant GST gene family has been identified in cucumber [35], Arabidopsis [36], tomato [37], grape [38], apple [39] and other crop species. However, the detailed identification of the GST gene family in the loquat genome has not been completed, the expression profiles of EjGSTs involved in alleviating frost damage in leaves are still unclear, and the regulatory mechanism of exogenous ALA on EjGST gene expression is still unknown. Therefore, we applied bioinformatics technology to identify and analyze EjGSTs and clarify the expression profiles of EjGSTs under cold stress in leaves. Moreover, the differences in the expression of EjGSTs in leaves in response to exogenous ALA under cold stress were compared and analyzed. This study provides a theoretical basis for further exploration of the cold resistance function of GSTs in loquat and important scientific evidence for the development of new antifreezes for loquat.
2.1 Plant Materials and Treatments
Two-year-old grafted ‘Zaozhong No. 6’ plants (Putian University Loquat Germplasm Resources Nursery, Fujian, China) with normal growth were used as the experimental material, and the leaves were cleaned with water before treatment. To study the effects of ALA on loquat leaves under cold stress, control seedling leaves (CK) were sprayed with pure water at room temperature (25°C); the seedling leaves of the low-temperature treatment group (T1) were subjected to low-temperature stress after being sprayed with pure water; and the seedling leaves of the ALA+low-temperature treatment group (T2) were sprayed with 175 mg/L ALA before being subjected to low-temperature stress. In strict reference to Wu et al. [31], ALA pretreatment and low-temperature stress treatment were carried out on loquat seedlings. T1 and T2 seedlings were cultured in an artificial climate chamber at low temperature for 6 h. The culture conditions were set as follows: 70% relative humidity, 12 h light, 2000 lx light intensity and −3°C temperature. The seedlings were then acclimated at room temperature for 10 h. Afterward, the seedling leaves were rapidly excised, frozen in liquid nitrogen, and stored at −70°C in a low-temperature refrigerator for subsequent experiments. The CK seedlings were cultivated in an artificial climate chamber at room temperature, and the other conditions were consistent with those of the treatment group. Each treatment included 10 loquat plants, and three replicates were performed. A total of 20 young leaves were taken from each replicate to form a mixed sample.
2.2 Identification and Characterization of GSTs in Loquat
We downloaded forty-seven complete Arabidopsis thaliana (A. thaliana) GST protein sequences from the TAIR database (https://www.arabidopsis.org/) (accessed on 26 September 2024) [36]. Additionally, we obtained the latest reference genome and annotations for ‘Jiefangzhong’ loquat from CNGB (https://db.cngb.org/cnsa/) (accessed on 26 September 2024) via accession number CNP0001531. These sequences were used to identify EjGSTs through the BLAST search function in TBtools, with an E value threshold set at ≤10−10. Moreover, the putative loquat GST protein sequences were submitted to the UniProt and HMMER 3.4 databases to confirm the presence of the typical domain, after which redundant proteins without conserved domains were removed. TBtools-II was applied to visualize the conserved domain of the Arabidopsis and loquat GSTs [40]. Furthermore, basic protein properties (molecular weight, amino acid number and theoretical isoelectric point) were determined via the ProtParam online tool (https://web.ExPASy.org/protparam/) (accessed on 26 September 2024). The subcellular location of the EjGSTs was predicted via the WoLF PSORT tool (https://wolfpsort.hgc.jp/) (accessed on 26 September 2024).
2.3 Bioinformatic Analysis of EjGSTs
To compare the evolutionary relationships and identities among the subfamilies, the GSTs from Arabidopsis and loquat were aligned via ClustalW. MEGA 11 software was used to construct the phylogenetic tree via the neighbor–joining method with 1000 bootstrap replicates. iTOL online software (https://itol.embl.de/) (accessed on 26 September 2024) was used to construct the evolutionary trees.
The gene structures of EjGST, showing the exon–intron organization on the basis of the loquat genome annotation, were visualized with GSDS 2.0 (http://gsds.gao-lab.org/) (accessed on 26 September 2024). After the conserved motifs of the EjGSTs were predicted via MEME (https://meme-suite.org/meme/) (accessed on 26 September 2024), the conserved domains were retrieved via NCBI-CDD online software. The promoter region (2000 bp upstream) of the EjGSTs was extracted on the basis of the loquat annotation and analyzed via PlantCARE (http://bioinformatics.psb.ugent.be/webtools/plantcare/html/) (accessed on 26 September 2024) online tools to predict the cis-acting elements. TBtools-II was used to visualize gene structure and cis-acting elements [40].
On the basis of the loquat annotation data, the chromosomal locations of the EjGSTs were determined and plotted via MapGene2Chro (https://mg2c.iask.in/mg2c_v2.0/) (accessed on 26 September 2024). MCScanX [41] was used to analyze the intra-and interspecific collinearity of GSTs on the basis of loquat, Arabidopsis and apple genome sequences, and the results were visualized via TBtools-II [40].
2.4 Quantitative Real-Time PCR
Total RNA was isolated via a Plant RNA Kit (Omega, Norcross, Georgia, USA). A reverse transcription kit (TaKaRa, Shiga, Japan) was used to synthesize cDNA. The quantitative primers used for the EjGSTs and internal reference genes (eIF2B and UBCE [42]) are listed in Table 1. Real-time quantitative PCR (RT–qPCR) was conducted via TB Green Premix Ex TaqTM (TaKaRa, Shiga, Japan) according to the manufacturer’s instructions. The PCR was run for 40 cycles, each consisting of 5 s at 95°C and 30 s at 60°C, on a real-time PCR system. RT–qPCR was performed in a CFX Connect™ Thermal Cycler (Bio-Rad, Singapore). The results were quantitatively analyzed by the 2−ΔΔCt method.
2.5 Determination of GSH, GSSG, H2O2, and MDA Contents and GST Activity
The contents of H2O2 and MDA in loquat leaves were determined via the methods of Tian et al. [43] with slight modifications. Loquat leaves (1 g) were crushed into powder in liquid nitrogen, added to phosphate buffered saline (PBS), vortexed and centrifuged. The absorbance value of supernatant was measured via the titanium reagent method with 510 nm, and the H2O2 content was calculated. Similarly, 0.5 g of loquat leaves was ground into powder in liquid nitrogen, added to an appropriate amount of thiobarbituric acid (TBA), vortexed and heated for 30 min. After cooling and centrifugation, the absorbance value of supernatant was measured at 532 nm, and the MDA content was calculated.
The contents of GSH and GSSG in loquat leaves were determined according to the methods of Fan et al. [44]. After grinding in liquid nitrogen, buffer containing 5% trichloroacetic acid (TCA) was added to 1 g loquat leaf sample, which was subsequently vortexed and centrifuged. The supernatant was collected, and 5,5′-dithiobis (2-nitrobenzoic acid) (DTNB) chromogenic agent was added. After standing in the dark at room temperature for a period of time, the absorbance was measured at a wavelength of 412 nm, and the GSH content was calculated on the basis of these readings. For the determination of GSSG, an appropriate amount of NADPH is needed to reduce GSSG to GSH. The GSH content, which was determined via the same method as above, was the GSSG content.
The method of Huang et al. [45] was used to detect the enzyme activity of GST in loquat leaves. Loquat leaves (1 g) were ground in liquid nitrogen. After adding PBS buffer, the mixture was vortexed and centrifuged. The supernatant was collected, and a reaction mixture containing 1-chloro-2,4-dinitrobenzene (CDNB) and GSH was added. The change in absorbance was continuously monitored at a wavelength of 340 nm, after which the GST activity was calculated.
The data were statistically analyzed via SPSS 26 software. One-way analysis of variance (ANOVA) and Duncan’s new multiple range test were performed to determine significant differences between groups (*, p < 0.05; **, p < 0.01). The data are presented as the mean ± standard error of the mean (n = 3). Bar graphs were ploted via GraphPad Prism 9 software.
3.1 Identification and Bioinformatic Analysis of EjGSTs
We used the Arabidopsis thaliana GST protein sequence and, through the BLAST Genome Utility (GUL) Wrapper function in TBtools, searched the Loquat protein database, identifying 16 loquat GST protein sequences that possess a typical GST domain (Fig. 1). The EjGSTs were categorized and named in accordance with the phylogenetic relationship of the GST family between Arabidopsis and loquat (Table 2 and Fig. 2). Based on the loquat genome annotation, the EjGSTs encoded 178 (EjGSTU6) to 464 (EjGSTF2) aa proteins with molecular weights between 20.61 (EjGSTU6) and 53.5 (EjGSTF2) kD, and the theoretical isoelectric points varied from 5.31 (EjGSTZ1) to 9.56 (EjGSTU6), with only 3 alkaline members (Table 2). Moreover, the subcellular localization prediction results revealed that 8 and 6 EjGSTs were located in the cytoplasm and chloroplasts, respectively, whereas only 1 EjGST was located in the nucleus (EjGSTU6) or peroxisome (EjGSTT1) (Table 2).
Figure 1: Conserved domains of EjGSTs. A total of 6 conserved GST domains are represented by different colored boxes. The Pfam IDs of these conserved GST domains are PF02798.23 (GST_N), PF13409.9 (GST_N_2), PF13417.9 (GST_N_3), PF00043.28 (GST_C), PF13410.9 (GST_C_2) and PF14497.9 (GST_C_3)
Figure 2: Phylogenetic analysis of the GSTs of Arabidopsis and loquat. The purple background represents the Tau subfamily, the yellow background represents the Phi subfamily, the blue background represents the Zeta subfamily, and the green background represents the Theta subfamily
To investigate the phylogenetic relationships of the EjGSTs, we constructed a phylogenetic tree of 16 loquat and 47 Arabidopsis GST protein sequences via the neighbor-joining method (bootstrap = 1000) (Fig. 2). EjGSTs presented high homology with Arabidopsis GST proteins. Therefore, all 16 EjGSTs were divided into four classes (Tau, Phi, Zeta and Theta) and named according to their protein homology with those of Arabidopsis [36]. Moreover, the proportions of subfamilies in the EjGSTs were similar to those in the AtGSTs. The Tau subfamily was the largest, comprising eight members, which represent 50% of all EjGSTs. This was followed by the Phi subfamily with five members, the Zeta subfamily with two members, and the Theta subfamily with just one member.
Intron gain or loss affects gene structure, which plays an essential role in the evolution of gene families [46]. To investigate the structural diversity of the loquat GST family, the introns and exons of the 16 EjGSTs were analyzed (Fig. 3). The results indicated that EjGSTs from the same subfamily presented similar exon–intron structures and that the EjGSTs contained two or more exons. In the Tau subfamily, most loquat GST genes contained two exons and one intron with the exception of EjGSTU1 and EjGSTU4; all EjGSTFs contained three exons, except for EjGSTF2 and EjGSTF4, which had seven and five exons, respectively. There were many exons in the Zeta and Theta subfamilies, of which EjGSTZ1 contained the largest number (12) of exons. Furthermore, 10 EjGST proteins (62.5%) had both 5′ and 3′ untranslated regions (UTRs).
Figure 3: Exon–intron structure analysis of EjGSTs. Color description: CDS—yellow color, intron—black thread, upstream and downstream UTR—green
To better understand the functions of the EjGST proteins, MEME online software was used to determine their conserved motifs, and 10 conserved motifs were found (Fig. 4). The longest conserved motif contained 50 amino acids, and the shortest conserved motif contained 11 amino acids (Table 3). EjGSTs in the same subfamily or branch of the phylogenetic tree contained similar motifs, suggesting that they have similar biological functions. In addition to EjGSTT1 (without motif 5) and EjGSTU6 (without motifs 3 and 5), motifs 1, 2, 3 and 5 were found in the other members, indicating that these four motifs are strongly conserved in loquat GST proteins. However, motifs 4, 7 and 10 were unique to the Tau subfamily; motif 9 was unique to the Zeta subfamily; Tau and Zeta were the only subfamilies that contained motif 8; and motif 6 occurred only in the Theta and Phi subfamilies. In particular, the motifs of EjGSTF2 and EjGSTF4 exhibited tandem repetition. Differences in conserved motifs between different subfamilies made functional diversity of EjGSTs possible. Moreover, the Tau subfamily included the most motif types among the EjGSTs.
Figure 4: Conserved motif distribution of EjGSTs. The 10 conserved motifs of the EjGSTs are represented by different colored boxes
3.2 Chromosomal Locations and Collinearity Analysis
The locations of the EjGSTs on loquat chromosomes were analyzed by examining their distribution within the loquat genome annotation via MapGene2Chro (Fig. 5). The 16 EjGSTs were randomly spread across the 10 chromosomes of loquat. Chromosome 17 contained 3 GSTs, followed by chromosomes 6, 10, 13 and 15 with two GSTs. Chromosomes 4, 7, 8, 9 and 16 each contained only one GST.
Figure 5: Chromosomal locations of the GSTs in the loquat genome. The orange vertical bar represents the loquat chromosome. The ‘Chr’ above the chromosome indicates the chromosome number. The different color markers on each chromosome represent the different subclasses of EjGSTs
Segmental and tandem duplications are crucial driving forces for the gene family expansion. A total of three tandemly duplicated gene pairs were dispersed on chromosomes 10, 13 and 15 (Fig. 5). One tandem duplication in the Zeta subfamily and two tandem duplications in the Tau subfamily suggested that tandem duplication events made significant contributions to the expansion of the Zeta and Tau subfamilies in loquat. Intraspecific collinearity analysis was performed to examine the segmental duplication of EjGSTs, which revealed 3 gene pairs in the Tau, Phi and Zeta subfamilies on 6 chromosomes (Fig. 6). Segmental duplication dramatically promoted the amplification of the EjGST gene family. In addition, the interspecific collinearity relationships of the GSTs among loquat, apple and Arabidopsis were determined via MCScanX (Fig. 7). The results revealed that 10 EjGSTs exhibited a collinear relationship across the three species, indicating that the EjGSTs were relatively highly conserved on the corresponding chromosomes during evolution. In total, we identified 16 orthologous gene pairs of EjGSTs in Arabidopsis involving 13 AtGSTs and 8 EjGSTs and 15 orthologous gene pairs of EjGSTs in apple involving 12 MdGSTs and 10 EjGSTs.
Figure 6: Interchromosomal relationships of EjGSTs. The red curve indicates collinear gene pairs of loquat GSTs on different chromosomes. The genomic locations of all the gene pairs in loquat are shown in a yellow font
Figure 7: Collinearity analysis of GSTs among loquat, apple and Arabidopsis chromosomes. The red line represents the top-down collinearity of the GST genes. Purple, orange and blue represent Arabidopsis thaliana, Eriobotrya japonica and Malus domestica, respectively
3.3 Analysis of the Cis-Regulatory Elements of EjGSTs and Expression Profiles of EjGSTs in Loquat Leaves under Cold Stress
The 2000 bp upstream sequences of the transcription start site in 16 EjGST promoters were integrated via the PlantCARE website to predict the main cis-acting elements related to stress responses, phytohormone signals, etc. (Fig. 8). Almost all EjGST promoters contained light-responsive, anaerobic induction, abscisic acid-responsive and jasmonic acid-responsive elements and MYB binding sites. In particular, the promoters of 10 EjGSTs, EjGSTF2/3/4/5, EjGSTU3/4/5/6/7 and EjGSTZ1, are low-temperature response elements.
Figure 8: The main cis-acting elements in the EjGST promoters are represented by different color modules, where each color corresponds to a specific type of cis-element
Many studies have shown that GSTs are involved in plant cold tolerance [14,15]. The cis-acting element prediction results indicated a certain responsiveness to low temperature in the EjGSTs. Hence, we measured the gene expression of 16 EjGSTs in loquat leaves under cold stress. The relative expression levels of the 9 EjGSTs were significantly different under cold stress (Fig. 9). Under cold treatment, the expression of EjGSTU7, EjGSTU8, EjGSTF2, EjGSTT1 and EjGSTZ1 strongly decreased, whereas the expression of EjGSTU1, EjGSTU3, EjGSTU4 and EjGSTF1 markedly increased. These 9 EjGSTs might play a vital role in the response of loquat leaves to cold stress, among which 5 genes belong to the Tau subfamily.
Figure 9: Gene expression patterns of nine EjGSTs in response to low temperature under ALA pretreatment in loquat leaves. T1 indicates that only low-temperature treatment is applied. T2 indicates that ALA treatment was applied while low-temperature stress was applied. One-way analysis of variance (ANOVA) and Duncan’s new multiple range test were performed to determine significant differences between groups (*, p < 0.05; **, p < 0.01; n, p > 0.05). The data are presented as the mean ± standard error of the mean (n = 3)
3.4 Effects of Exogenous ALA on the Expression Profiles of EjGSTs and Relevant Physiological and Biochemical Indices in Loquat Leaves under Cold Stress
Studies have shown that ALA is involved in improving cold tolerance by regulating GST expression in crops [47]. Therefore, we explored EjGST expression in response to low temperature under ALA pretreatment (Fig. 9). Overall, ALA significantly suppressed the expression of EjGSTU1 and EjGSTF1 in loquat leaves. Compared with those under the normal-temperature treatment, the expression of EjGSTF2, EjGSTT1, EjGSTZ1, EjGSTU3 and EjGSTU4 could be induced appropriately by ALA pretreatment under cold stress. However, ALA did not alter EjGSTU7 or EjGSTU8 expression in loquat leaves subjected to cold stress.
Furthermore, we examined the effects of exogenous ALA on relevant physiological and biochemical indices in loquat leaves under cold stress. The ability of GSTs to scavenge ROS depends on the participation of GSH and promotes the oxidation of GSH to GSSG, affecting the level of intracellular GSH [48]. As shown in Fig. 10, the GST activity in T1 was slightly lower than that in CK, but the difference was not significant (p > 0.05), indicating that low-temperature stress had little effect on the GST activity in loquat leaves. The GST enzyme activity in T2 was 1.81 times greater than that in T1, representing an increase of 81.30% (p < 0.01), suggesting that ALA pretreatment could activate GST enzyme activity in loquat leaves under cold stress. The intracellular GSH content of T1 was 49.33% lower than that of CK, indicating that cold stress inhibited intracellular GSH production. The intracellular GSH content in T2 was significantly greater than that in T1 (p < 0.01); thus, exogenous ALA promoted GSH accumulation in loquat leaves under low-temperature stress. Moreover, the intracellular GSSG content in T2 was lower than that in T1, suggesting that exogenous ALA promoted an increase in the GSH content in loquat leaves and reduced GSSG accumulation. Therefore, the GSH/GSSG ratio of T2 was the highest among all the treatment groups, indicating that ALA pretreatment obviously enhanced the ability of loquat leaves to scavenge ROS in a GSH-dependent manner under cold stress.
Figure 10: Effects of ALA on relevant physiological and biochemical indices in loquat leaves. CK indicates the loquat seedling group without any pretreatment or stress. T1 indicates the loquat seedling group subjected to only low-temperature treatment. T2 indicates the loquat seedling group pretreated with ALA before the low-temperature treatment. One-way analysis of variance (ANOVA) and Duncan’s new multiple range test were performed to determine significant differences between groups (*, p < 0.05; **, p < 0.01; n, p > 0.05). The data are presented as the mean ± standard error of the mean (n = 3)
Low temperature induces extreme accumulation of H2O2 in plant cells and leads to membrane lipid peroxidation damage, resulting in increased MDA content [49]. As shown in Fig. 10, the H2O2 content of low-temperature-treated T1 plants was dramatically greater than that of CK plants (p < 0.01), indicating that low temperature induced H2O2 accumulation in loquat leaf cells. Under cold stress, the H2O2 content of T2 plants treated with exogenous ALA was markedly lower than that of T1 plants treated with low temperature alone (p < 0.01), indicating that exogenous ALA reduced H2O2 accumulation in loquat leaf cells subjected to low-temperature stress. Moreover, the MDA content of T1 was 2.15 times greater than that of CK (p < 0.01), while the MDA content of T2 with exogenous ALA was reduced by 12.16% compared with that of T1 (p < 0.05) under cold conditions. Low temperature caused peroxidation damage in loquat leaves, but exogenous ALA effectively relieved membrane lipid peroxidation damage.
Plant GSTs are a major family of enzymes involved in GSH regeneration and metabolism and play vital roles in plant resistance to abiotic and biotic stresses by alleviating oxidative damage [13]. The GST gene family has been identified and analyzed in various plants because of its plentiful functions [12,50,51]. However, no genome-wide identification of the loquat GST family has been reported until now. In our study, a total of 16 full-length GSTs were identified and evaluated in loquat (Fig. 1), which were divided into 4 subfamilies and widely distributed on 10 of the 17 loquat chromosomes (Fig. 5). Compared with those of other species, the loquat GST family has shrunk [36,39]. Many studies have shown that plant GSTs play vital roles in cold resistance, so it was speculated that the much lower number of EjGST family members might reflect the relative cold intolerance of loquat to a certain extent. The function of a protein is related to its subcellular localization [52]. Subcellular location analysis revealed that 8 of the 16 (50%) proteins were located in the cytoplasm (Table 2), revealing the solubility of these GSTs [53]. In addition to EjGSTU6 (nucleus) and EjGSTT1 (peroxisome), the remaining 6 of the 16 EjGSTs were located in the chloroplast (Table 2). Studies have shown that GSTs located in different subcellular structures have different modes of action. For example, GSTT1, GSTT2 and GSTT3 in Arabidopsis are localized only to peroxisomes, which may be related to the protection of peroxisome membrane integrity [54]. Therefore, the localization of loquat EjGSTT1 in peroxisomes may also involve a similar mode of action. Similarly, GSTF in Arabidopsis is located in the cytoplasm and chloroplasts, which may be related to flavonoid transport [1,55]. Chromosome and subcellular localization analyses revealed the widespread distribution of EjGSTs in loquat, which may be among the key factors contributing to the diversity and complexity of the function of this gene family. In addition, EjGSTs have a wide range of amino acid lengths and molecular weights, and most of them (81.25%) are acidic (Table 2).
Phylogenetic analysis revealed that the EjGSTs were divided into the Tau, Phi, Zeta and Theta classes with high levels of similarity to GSTs in Arabidopsis (Fig. 2), which indicated high evolutionary conservation between EjGSTs and AtGSTs. Gene tandem and segmental duplications have vital effects on the genetic evolution of plant gene families [56], and many new functions are preserved through subfunctionalization or neofunctionalization of replicators [57]. For example, the MruGST gene of Medicago ruthenica is expanded by segmental and tandem replication [58]. Among the TaGST genes of Triticum aestivum L., 43 pairs and 171 pairs were confirmed as tandem and segmental repeat genes, respectively [59]. Like other crops [10,39,50], the Tau subfamily was the most gene-rich, followed by the Phi subfamily (Fig. 2), possibly because of tandem gene duplication on chromosomes 10 and 13. Conversely, the Zeta and Theta subfamilies contained fewer members (Fig. 2), possibly because of their scattering distributions across different chromosomes, preventing tandem duplication. In total, we identified 2 pairs of tandem Tau genes and 1 pair of segmentally duplicated Tau genes, representing 62.5% of the EjGSTU genes, whereas only 1 and 2 duplicated gene pairs were found in the Phi and Zeta classes, respectively (Figs. 2 and 6). These finding suggests that tandem and segmental gene duplication events have played a significant role in the evolution of the EjGST family. These findings indicate that the expansion of the EjGST family was largely due to the duplication of the Tau and Phi subfamilies, which is consistent with findings in tobacco [50]. Moreover, studies have shown that GSTs of the Tau and Phi classes are induced in plants subjected to stress [60]. Therefore, the large-scale expansion of the Tau and Phi subfamilies of EjGSTs may promote the tolerance of loquat to various stresses. Furthermore, interspecies collinearity analysis indicated relatively high conservation of EjGSTs on the corresponding chromosomes during evolution (Fig. 7), which illustrated functional conservation during the evolution of EjGSTs.
Exon–intron structure, whose alteration is one of the key factors causing gene duplication in organismal evolution [46], has been reported to play a crucial role in gene evolution. In Nicotiana tabacum L., NtGST, located on the same branch of the phylogenetic tree, has a similar exon-intron structure and motif composition [50]. In our study, we found that the GSTs within the same subfamilies shared similar numbers and positions of introns and exons in loquat (Fig. 3), suggesting the possibility of extensive functional redundancy within the EjGST family. It has been reported that compact gene structures with fewer introns can respond to stress in time [53]. Therefore, we suggest that loquat Tau and Phi members with fewer introns might respond quickly to stress. Conserved motif analysis revealed that genes in the same EjGST subfamily presented similar motif constitutions (Fig. 4) and may perform similar potential functions in loquat. This conclusion is consistent with the finding that similar motifs in the same subfamily of MdGSTs result in a wide range of similar functions [61]. However, we also detected some differences in motif construction within the same EjGST subfamily, revealing clues to gene function diversity. Moreover, cis-acting elements are involved in the regulation of target gene expression, thereby modulating plant adaptation to stress [62]. For example, the promoters of apple GSTs contain multiple hormone-related cis-regulatory elements and stress response elements related to cold and drought, which play vital regulatory roles in the gene expression of MdGSTs involved in anthocyanin accumulation [61]. Various environmental stress response elements are predicted to be present in the promoters of EjGSTs (Fig. 8), suggesting that the expression of EjGSTs may be regulated by phytohormones and environmental signals and certain transcription factors. In particular, 10 of 16 EjGSTs had low-temperature responsive elements upstream (Fig. 8), suggesting that genes in this family are highly likely to be regulated at low temperatures. Previous studies have shown that the promoters of plant GSTs are regulated by stress signals. For example, CsWRKY48 activates the expression of CsGSTU8 under drought conditions by binding directly to the W-box on its promoter [63]. The promoter of SbGSTU has dramatically high GUS expression activity in tobacco under salt and osmotic stress [64].
To explore gene responsiveness to cold stress in cold-susceptible loquat plants accurately, the expression profiles of EjGSTs under cold conditions were analyzed (Fig. 9). The expression of four genes (EjGSTU1, EjGSTU3, EjGSTU4 and EjGSTF1) was obviously upregulated in ‘Zaozhong No. 6’ leaves after treatment with cold temperature, and low temperature significantly inhibited the expression of 5 EjGSTs (EjGSTU7, EjGSTU8, EjGSTF2, EjGSTZ1 and EjGSTT1). Five of these 9 differentially expressed EjGSTs involved highly low-temperature responsive cis-acting elements in response to cold (Fig. 8). However, none of the remaining 7 EjGSTs, involving nearly half of the EjGST family members, responded to cold stress. These unresponsive or inhibited EjGSTs might contribute to the relatively low cold tolerance of the ‘Zaozhong No. 6’ loquat. Therefore, these genes might be used as putative candidates for breeding cold-tolerant loquat cultivars through molecular genetics technologies. Similarly, among the 5 most highly expressed BoGSTUs in cold-tolerant cabbage varieties under chilling, the expression of BoGSTU3, BoGSTU6 and BoGSTU19 did not significantly change during cold treatment [11]. Additionally, nearly half of the 9 EjGSTs whose expression differed under cold conditions were up-or downregulated in loquat leaves. This differential regulation might explain the lack of a significant difference in GST enzyme activity between the low-temperature treatment group and the control group (Fig. 10). Therefore, the GSH and GSSG levels in loquat leaves noticeably decreased and then increased (a remarkable decrease in the GSH/GSSG ratio), followed by markedly increased H2O2 and MDA contents (Fig. 2), resulting in weak cold tolerance in ‘Zaozhong No. 6’ loquat plants.
Clearly, cold stress has significant physiological effects on ‘Zaozhong No. 6’ loquat plants, which exhibit weak cold resistance and severely affect the normal growth and development of loquat plants [65]. Fortunately, recent studies have shown that exogenous ALA can increase the stress response ability of plants and improve crop yield and quality [66]. ALA improved the AsA-GSH cycle, an antioxidant mechanism composed of enzyme-and non-enzyme-promoted antioxidants, to remove H2O2 and MDA produced in large quantities in plants under stress, which is an important aspect of enhancing plant cold tolerance [67,68]. When subjected to cold stress, GST in the enzyme-promoted defense system can catalyze GSH with H2O2 to generate GSSG and H2O, and catalyze the reaction of GSH to react with membrane lipid peroxides resulting in the reducion of excessive ROS in plants, thus alleviating the damage caused by low temperature to membrane structural integrity and function [69,70]. Exogenous application of 175 mg·L−1 ALA under cold stress improved the cold tolerance of ‘Zaozhong No. 6’ loquat [71]. However, whether EjGSTs are involved in the mechanism by which ALA improves loquat cold tolerance remains unclear. Therefore, this study analyzed the expression patterns of EjGSTs in response to cold stress under ALA pretreatment (Fig. 9), providing an experimental basis for further investigations of the cold resistance function of EjGSTs. Exogenous ALA promoted the expression of EjGSTU3, EjGSTU4, EjGSTF2, EjGSTZ1 and EjGSTT1 under cold stress but significantly suppressed the expression of EjGSTU1 and EjGSTF1 under cold stress. The comprehensive promotion effect of exogenous ALA on EjGST expression was also reflected in the extremely significant increase in GST activity in loquat leaves at low temperature, which promoted intracellular GSH production and reduced intracellular GSSG accumulation with an increase in the GSH/GSSG ratio (Fig. 10). ALA pretreatment might dramatically increase the GSH-dependent ROS scavenging ability of loquat leaves under low temperature stress by affecting EjGST expression, which was reflected in the alleviation of H2O2 accumulation and membrane lipid peroxidation damage (Fig. 10). After ALA pretreatment, EjGSTF1 and EjGSTU1 were significantly down-regulated under cold stress, which seemed to have the opposite effect with ALA enhancing GST activity. This may be due to the compensatory effect of slight up-regulation and accumulation of the remaining 7 EjGST genes, which offset the significant down-regulation of EjGSTF1 and EjGSTU1. A similar phenomenon was observed in the ADH gene family of yeast [72]. Another possibility is that gene expression patterns and protein accumulation may be inconsistent due to the long stability and half-life of certain proteins or post-transcriptional regulation of genes [73–75]. Therefore, the significant down-regulation of the expression of these two EjGSTs did not prevent ALA from increasing GST activity and even increased the cold resistance of ‘Zaozhong No. 6’ loquat. Furthermore, exogenous ALA can induce plant GST expression through transcription factors such as ERFs and MYBs to protect plants from abiotic stress [47,76]. This gene was also predicted to be a cis-acting element of EjGSTs, which needs to be verified experimentally, and the specific regulatory mechanisms involved need to be further studied.
In summary, this was the first systematic genome-wide identification and characterization of GSTs in loquat and provided insight into their role in the response to cold stress. A total of 16 EjGSTs were identified and divided into four subfamilies, and information on the physicochemical properties, subcellular localization and chromosomal distribution of these genes was analyzed. EjGSTs in the same branch of the evolutionary tree presented similar gene structures and protein characteristics. Three pairs of segmental duplications and three pairs of tandem duplications were distributed on chromosomes, which suggested the importance of gene duplication events in the expansion of the loquat GST gene family. The collinearity analysis of these genes with the GSTs in other plants provided a crucial reference for exploring the evolutionary characteristics of the EjGSTs. Promoter analysis and expression profiling were performed, revealing the obvious cold responsiveness of the EjGSTs and the potential reason for the cold sensitivity of the ‘Zaozhong No. 6’ loquat. Among the 16 GSTs, four (EjGSTU1, EjGSTU3, EjGSTU4 and EjGSTF1) were highly upregulated in the cold-sensitive ‘Zaozhong No. 6’ loquat variety under cold stress and thus could be used to develop cold-tolerant cultivars of loquat through transgenic or molecular breeding. Furthermore, the expression of the most differentially expressed EjGSTs (EjGSTF2, EjGSTT1, EjGSTZ1, EjGSTU3 and EjGSTU4) during cold stress was promoted to a certain extent by ALA pretreatment, indicating that EjGSTs might be involved in ALA-induced cold tolerance. This comprehensive promotion effect of exogenous ALA on EjGST expression under cold conditions was also demonstrated by changes in AsA–GSH cycle-related physiological and biochemical indicators. This study revealed that EjGSTs are involved in the regulation of the cold stress responsiveness in loquat and contributes to the preliminary understanding of the mechanism by which ALA improves loquat cold resistance. Moreover, this study provides a theoretical basis for the molecular breeding of cold-resistant loquat varieties and the development of new antifreeze agents.
Acknowledgement: None.
Funding Statement: This research was funded by grants from the Fujian Provincial Science and Technology Project (2021N5014, 2022N5006) and the Science and Technology Plan Project of Putian (2023GJGZ001).
Author Contributions: Shoukai Lin and Jincheng Wu supervised this study and revised the manuscript. Guanpeng Huang and Ti Wu carried out the bioinformatics analysis and qRT–PCR experiments and completed the manuscript. Yinjie Zheng performed the biochemical experiments and revised the manuscript. Qiyun Gu and Qiaobin Chen participated in the bioinformatics analysis and qRT–PCR experiments. All authors reviewed the results and approved the final version of the manuscript.
Availability of Data and Materials: All of the data developed or examined during this investigation are available in this published article and its supplementary materials, or they can be provided at reasonable request.
Ethics Approval: Not applicable.
Conflicts of Interest: The authors declare that they have no conflicts of interest to report regarding the present study.
References
1. Dixon DP, Davis BG, Edwards R. Functional divergence in the glutathione transferase superfamily in plants. J Biolog Chem. 2002;277(34):30859–69. doi:10.1074/jbc.M202919200. [Google Scholar] [PubMed] [CrossRef]
2. Sheehan D, Meade G, Foley VM, Dowd CA. Structure, function and evolution of glutathione transferases: implications for classification of non-mammalian members of an ancient enzyme superfamily. Biochem J. 2001;360:1–16. doi:10.1042/bj3600001. [Google Scholar] [CrossRef]
3. Polidoros AN, Scandalios JG. Role of hydrogen peroxide and different classes of antioxidants in the regulation of catalase and glutathione S-transferase gene expression in maize (Zea mays L.). Physiol Plant. 1999;106(1):112–20. doi:10.1034/j.1399-3054.1999.106116.x. [Google Scholar] [CrossRef]
4. Mittler R, Vanderauwera S, Gollery M, Van Breusegem F. Reactive oxygen gene network of plants. Trends Plant Sci. 2004;9(10):490–8. doi:10.1016/j.tplants.2004.08.009. [Google Scholar] [PubMed] [CrossRef]
5. Qiao X, Zhang J. Research progress on GPX in plants. Biotechnol Bull. 2016;32(9):7–13. [Google Scholar]
6. Edwards R, Dixon DP. Plant glutathione transferases. Methods Enzymol. 2005;401:169–86. doi:10.1016/S0076-6879(05)01011-6. [Google Scholar] [PubMed] [CrossRef]
7. Cai Q, Wu Q. Progress in glutathione transferase. J Hainan Med Univ. 2011;17(12):1735–8 (In Chinese). [Google Scholar]
8. Shi QQ, Zhou L, Wang Y. Isolation and expression analysis of GST gene encoding glutathione S-transferase of Paeonia delavayi var. Lutea wild population in Yunnan. Scientia Silvae Sinicae. 2014;50(12):63–72. [Google Scholar]
9. Guo YL, Tao B, Zheng TJ, Li BY, Zhai XH, Pan YQ. Induction of herbicides and antidotes on plant glutathione S-transferase (GSTs). J Northeast Agricult Univ. 2008;39(7):136–9 (In Chinese). [Google Scholar]
10. Abdul Kayum M, Nath UK, Park JI, Biswas MK, Choi EK, Song JY, et al. Genome-wide identification, characterization, and expression profiling of glutathione S-transferase (GST) family in pumpkin reveals likely role in cold-stress tolerance. Genes. 2018;9(2):84. doi:10.3390/genes9020084. [Google Scholar] [PubMed] [CrossRef]
11. Vijayakumar H, Thamilarasan SK, Shanmugam A, Natarajan S, Jung H-J, Park J-I, et al. Glutathione transferases superfamily: cold-inducible expression of distinct GST genes in brassica oleracea. Int J Mol Sci. 2016;17(8):1211. doi:10.3390/ijms17081211. [Google Scholar] [PubMed] [CrossRef]
12. Islam S, Sajib SD, Jui ZS, Arabia S, Islam T, Ghosh A. Genome-wide identification of glutathione S-transferase gene family in pepper, its classification, and expression profiling under different anatomical and environmental conditions. Sci Rep. 2019;9(1):9101. doi:10.1038/s41598-019-45320-x. [Google Scholar] [PubMed] [CrossRef]
13. Pei DL. The progress of GSTs in plant defense. Chin Agricult Sci Bull. 2012;28(18):185–8 (In Chinese). [Google Scholar]
14. Kumar S, Asif MH, Chakrabarty D, Tripathi RD, Dubey RS, Trivedi PK. Expression of a rice Lambda class of glutathione S-transferase, OsGSTL2, in Arabidopsis provides tolerance to heavy metal and other abiotic stresses. J Hazard Mater. 2013;248–249:228–37. doi:10.1016/j.jhazmat.2013.01.004. [Google Scholar] [PubMed] [CrossRef]
15. Yang G, Xu Z, Peng S, Sun Y, Jia C, Zhai M. In planta characterization of a tau class glutathione S-transferase gene from Juglans regia (JrGSTTau1) involved in chilling tolerance. Plant Cell Rep. 2016;35(3):681–92. doi:10.1007/s00299-015-1912-8. [Google Scholar] [PubMed] [CrossRef]
16. Carmona L, Alquézar B, Tárraga S, Peña L. Protein analysis of moro blood orange pulp during storage at low temperatures. Food Chem. 2019;277(4):75–83. doi:10.1016/j.foodchem.2018.10.108. [Google Scholar] [PubMed] [CrossRef]
17. Wang LJ, Jiang WB, Zhang Z, Yao QH, Matsui H, Ohara H. Biosynthesis and physiological activities of 5-aminolevulinic acid (ALA) and its potential application in agriculture. Plant Physiol Commun. 2003;3:185–192. [Google Scholar]
18. Kim JG, Back K, Lee HY, Lee HJ, Phung TH, Grimm B, et al. Increased expression of Fe-chelatase leads to increased metabolic flux into heme and confers protection against photodynamically induced oxidative stress. Plant Mol Biol. 2014;86(3):271–87. doi:10.1007/s11103-014-0228-3. [Google Scholar] [PubMed] [CrossRef]
19. Qiu D, Hu W, Zhou Y, Xiao J, Hu R, Wei Q, et al. TaASR1-D confers abiotic stress resistance by affecting ROS accumulation and ABA signalling in transgenic wheat. Plant Biotechnol J. 2021;19(8):1588–601. doi:10.1111/pbi.13572. [Google Scholar] [PubMed] [CrossRef]
20. Tang M, Xu L, Wang Y, Dong J, Zhang X, Wang K, et al. Melatonin-induced DNA demethylation of metal transporters and antioxidant genes alleviates lead stress in radish plants. Hortic Res. 2021;8(1):124. doi:10.1038/s41438-021-00561-8. [Google Scholar] [PubMed] [CrossRef]
21. Bindu RC, Vivekanandan M. Hormonal activities of 5-aminolevulinic acid in callus induction and micropropagation. Plant Growth Regulat. 1998;26(1):15–8. doi:10.1023/A:1006098005335. [Google Scholar] [CrossRef]
22. Akram NA, Ashraf M. Regulation in plant stress tolerance by a potential plant growth regulator, 5-aminolevulinic acid. J Plant Growth Regulat. 2013;32(3):663–79. doi:10.1007/s00344-013-9325-9. [Google Scholar] [CrossRef]
23. Wang L, Zhang J, Zhong Y, Zhang L, Yang H, Liu L, et al. Regulation of 5-aminolevunilic acid and its application in agroforestry. Forests. 2023;14(9):1857. doi:10.3390/f14091857. [Google Scholar] [CrossRef]
24. Anwar A, Yan Y, Liu Y, Li Y, Yu X. 5-Aminolevulinic acid improves nutrient uptake and endogenous hormone accumulation, enhancing low-temperature stress tolerance in cucumbers. Int J Mol Sci. 2018;19(11):3379. doi:10.3390/ijms19113379. [Google Scholar] [PubMed] [CrossRef]
25. Xue X, Xie M, Zhu L, Wang D, Xu Z, Liang L, et al. 5-ALA improves the low temperature tolerance of common bean seedlings through a combination of hormone transduction pathways and chlorophyll metabolism. Int J Mol Sci. 2023;24(17):13189. doi:10.3390/ijms241713189. [Google Scholar] [PubMed] [CrossRef]
26. Balestrasse K, Tomaro ML, Batlle A, Noriega GO. The role of 5-aminolevulinic acid in the response to cold stress in soybean plants. Phytochemistry. 2010;71(17–18):2038–45. [Google Scholar] [PubMed]
27. Chen Z, Zhang J, Wang L. ALA induces stomatal opening through regulation among PTPA, PP2AC, and SnRK2.6. Front Plant Sci. 2023;14:1206728. doi:10.3389/fpls.2023.1206728. [Google Scholar] [PubMed] [CrossRef]
28. Badenes ML, Lin S, Yang X, Liu C, Huang X. Loquat (Eriobotrya Lindl.). In: Folta KM, Gardiner SE, editors. Genetics and genomics of rosaceae. New York, NY: Springer New York; 2009. p. 525–38. [Google Scholar]
29. Qiu WL. In the past 50 years of Fujian fruits. Fuzhou, China: Fujian Education Press; 2000. p. 372–9. [Google Scholar]
30. Huang JS. New technology of loquat culture. Fuzhou, China: Fujian Science and Technology Press; 2000. p. 25–7. [Google Scholar]
31. Wu JC, Chen Y, Wu BS, Huang LL, Zhang WF, Zheng FM. Effects of calcium on Ca2+-ATPase activity and lipid peroxidation level of loquat seedling under low temperature stress. J Northwest A&F Univ (Nat Sci Ed). 2016;44(2):121–8 (In Chinese). [Google Scholar]
32. Su XY, Liu ZT, Ji MX, Ren JP, Ji CZ, Mao NN. Research progress on effective components and abiotic stress tolerance of loquat. Jiangsu Agricult Sci. 2021;49(22):50–4 (In Chinese). [Google Scholar]
33. Xie ZC, Li J. Freezing injury temperature limit and division of optimum cultivation area of ‘Zaozhong No. 6’ loquat young fruits. Fujian Fruits. 2006;1:7–11+63 (In Chinese). [Google Scholar]
34. Zhang XP, Xu WD, Zheng CL. The research progress of freezing injury and prevention of loquat. Fujian Fruits. 2007;3:28–31 (In Chinese). [Google Scholar]
35. Duan X, Yu X, Wang Y, Fu W, Cao R, Yang L, et al. Genome-wide identification and expression analysis of glutathione S-transferase gene family to reveal their role in cold stress response in cucumber. Front Genet. 2022;13:1664–8021. [Google Scholar]
36. Wagner U, Edwards R, Dixon DP, Mauch F. Probing the diversity of the Arabidopsis glutathione S-transferase gene family. Plant Mol Biol. 2002;49(5):515–32. doi:10.1023/A:1015557300450. [Google Scholar] [PubMed] [CrossRef]
37. Islam S, Rahman IA, Islam T, Ghosh A. Genome-wide identification and expression analysis of glutathione S-transferase gene family in tomato: gaining an insight to their physiological and stress-specific roles. PLoS One. 2017;12(11):e0187504. doi:10.1371/journal.pone.0187504. [Google Scholar] [PubMed] [CrossRef]
38. Li H, Yang Y, Li H, Wang W, Zheng H, Tao J. Genome-wide identification of glutathione S-transferase and expression analysis in response to anthocyanin transport in the flesh of the new teinturier grape germplasm ‘Zhongshan-HongYu’. Int J Mol Sci. 2022;23(14):7717. doi:10.3390/ijms23147717. [Google Scholar] [PubMed] [CrossRef]
39. Fang X, An Y, Zheng J, Shangguan L, Wang L. Genome-wide identification and comparative analysis of GST gene family in apple (Malus domestica) and their expressions under ALA treatment. 3 Biotech. 2020;10(7):307. doi:10.1007/s13205-020-02299-x. [Google Scholar] [PubMed] [CrossRef]
40. Chen C, Wu Y, Li J, Wang X, Zeng Z, Xu J, et al. TBtools-II: a “one for all, all for one” bioinformatics platform for biological big-data mining. Mol Plant. 2023;16(11):1733–42. doi:10.1016/j.molp.2023.09.010. [Google Scholar] [PubMed] [CrossRef]
41. Wang Y, Tang H, Debarry JD, Tan X, Li J, Wang X, et al. MCScanX: a toolkit for detection and evolutionary analysis of gene synteny and collinearity. Nucleic Acids Res. 2012;40(7):e49. doi:10.1093/nar/gkr1293. [Google Scholar] [PubMed] [CrossRef]
42. Fu H, Huang T, Yin C, Xu Z, Li C, Liu C, et al. Selection and validation of reference genes for RT-qPCR normalization in Bradysia odoriphaga (Diptera: sciaridae) under insecticides stress. Front Physiol. 2021;12:818210. [Google Scholar] [PubMed]
43. Tian YQ, Nie GW, Li K, Zhang XP, Dai LR. Ameliorating effects of 5-aminolevulinic acid on damage to sweet cherry floral organ under low temperature stress. Acta Agric Boreali-Occidentalis Sin. 2020;29(4):595–602. [Google Scholar]
44. Fan HF, Li J, Guo SR, Du CX. Effects of exogenous nitric oxide on growth and glutathione dependent antioxidative system in cucumber seedling roots under NaCl stress. Acta Agric Boreali-Occidentalis Sin. 2007;8:1611–8. [Google Scholar]
45. Huang ZM, Wu JC, Chen WJ, Cai LQ, Xie CP, Lin LL, et al. Effects of SA on enzymes of ascorbate-glutathione cycle in young loquat fruits after low temperature stress. Scientia Silvae Sinicae. 2011;47(9):36–42. [Google Scholar]
46. Xu G, Guo C, Shan H, Kong H. Divergence of duplicate genes in exon-intron structure. Proc Natl Acad Sci U S A. 2012;109(4):1187–92. doi:10.1073/pnas.1109047109. [Google Scholar] [PubMed] [CrossRef]
47. Zhang Z, Yuan L, Dang J, Zhang Y, Wen Y, Du Y, et al. 5-Aminolevulinic acid improves cold resistance through regulation of SlMYB4/SlMYB88-SlGSTU43 module to scavenge reactive oxygen species in tomato. Hortic Res. 2024;11(3):uhae026. doi:10.1093/hr/uhae026. [Google Scholar] [PubMed] [CrossRef]
48. Qi YC, Zhang SM, Wang LP, Wang MD, Zhang H. Overexpression of GST gene accelerates the growth of transgenic Arabidopsis under salt stress. J Plant Physioldgy Molecul. 2004;30(5):517–22. [Google Scholar]
49. Guo P, Qi YP, Cai YT, Yang TY, Yang LT, Huang ZR, et al. Aluminum effects on photosynthesis, reactive oxygen species and methylglyoxal detoxification in two Citrus species differing in aluminum tolerance. Tree Physiol. 2018;38(10):1548–65. doi:10.1093/treephys/tpy035. [Google Scholar] [PubMed] [CrossRef]
50. Mo Z, Huang Y, Pu T, Duan L, Pi K, Luo J, et al. Genome-wide identification and characterization of Glutathione S-Transferases (GSTs) and their expression profile under abiotic stresses in tobacco (Nicotiana tabacum L.). BMC Genom. 2023;24(1):341. doi:10.1186/s12864-023-09450-x. [Google Scholar] [PubMed] [CrossRef]
51. Sabir IA, Manzoor MA, Shah IH, Liu X, Jiu S, Wang J, et al. Identification and comprehensive genome-wide analysis of glutathione S-transferase gene family in sweet cherry (Prunus avium) and their expression profiling reveals a likely role in anthocyanin accumulation. Front Plant Sci. 2022;13:1664–462X. [Google Scholar]
52. Dönnes P, Höglund A. Predicting protein subcellular localization: past, present, and future. Genom Proteom Bioinformat. 2004;2(4):209–15. doi:10.1016/S1672-0229(04)02027-3. [Google Scholar] [PubMed] [CrossRef]
53. Jeffares DC, Penkett CJ, Bähler J. Rapidly regulated genes are intron poor. Trends Genet. 2008;24(8):375–8. doi:10.1016/j.tig.2008.05.006. [Google Scholar] [PubMed] [CrossRef]
54. Dixon DP, Hawkins T, Hussey PJ, Edwards R. Enzyme activities and subcellular localization of members of the Arabidopsis glutathione transferase superfamily. J Exp Bot. 2009;60(4):1207–18. doi:10.1093/jxb/ern365. [Google Scholar] [PubMed] [CrossRef]
55. Zhang YZ, Zhang F, Wang LY, Wei K, Cheng H. Plant glutathione S-transferases: roles in flavonoid accumulation. Plant Physiol J. 2015;51(11):1815–20. [Google Scholar]
56. Cannon SB, Mitra A, Baumgarten A, Young ND, May G. The roles of segmental and tandem gene duplication in the evolution of large gene families in Arabidopsis thaliana. BMC Plant Biol. 2004;4:10. doi:10.1186/1471-2229-4-10. [Google Scholar] [PubMed] [CrossRef]
57. He X, Zhang J. Rapid subfunctionalization accompanied by prolonged and substantial neofunctionalization in duplicate gene evolution. Genetics. 2005;169(2):1157–64. doi:10.1534/genetics.104.037051. [Google Scholar] [PubMed] [CrossRef]
58. Wang T, Zhang D, Chen L, Wang J, Zhang WH. Genome-wide analysis of the Glutathione S-Transferase family in wild Medicago ruthenica and drought-tolerant breeding application of MruGSTU39 gene in cultivated alfalfa. Theor Appl Genet. 2022;135(3):853–64. doi:10.1007/s00122-021-04002-x. [Google Scholar] [PubMed] [CrossRef]
59. Wang R, Ma J, Zhang Q, Wu C, Zhao H, Wu Y, et al. Genome-wide identification and expression profiling of glutathione transferase gene family under multiple stresses and hormone treatments in wheat (Triticum aestivum L.). BMC Genom. 2019;20(1):986. doi:10.1186/s12864-019-6374-x. [Google Scholar] [PubMed] [CrossRef]
60. Nutricati E, Miceli A, Blando F, De Bellis L. Characterization of two Arabidopsis thaliana glutathione S-transferases. Plant Cell Reports. 2006;25(9):997–1005. doi:10.1007/s00299-006-0146-1. [Google Scholar] [PubMed] [CrossRef]
61. Zhao YW, Wang CK, Huang XY, Hu DG. Genome-wide analysis of the glutathione S-transferase (GST) genes and functional identification of MdGSTU12 reveals the involvement in the regulation of anthocyanin accumulation in apple. Genes. 2021;12(11):1733. doi:10.3390/genes12111733. [Google Scholar] [PubMed] [CrossRef]
62. Narusaka Y, Nakashima K, Shinwari ZK, Sakuma Y, Furihata T, Abe H, et al. Interaction between two cis-acting elements, ABRE and DRE, in ABA-dependent expression of Arabidopsis rd29A gene in response to dehydration and high-salinity stresses. Plant J. 2003;34(2):137–48. doi:10.1046/j.1365-313X.2003.01708.x. [Google Scholar] [PubMed] [CrossRef]
63. Zhang Y, He J, Xiao Y, Zhang Y, Liu Y, Wan S, et al. CsGSTU8, a glutathione S-transferase from camellia sinensis, is regulated by CsWRKY48 and plays a positive role in drought tolerance. Front Plant Sci. 2021;12:795919. doi:10.3389/fpls.2021.795919. [Google Scholar] [PubMed] [CrossRef]
64. Tiwari V, Patel MK, Chaturvedi AK, Mishra A, Jha B. Functional characterization of the Tau Class Glutathione-S-Transferases Gene (SbGSTU) promoter of Salicornia brachiata under salinity and osmotic stress. PLoS One. 2016;11(2):e0148494. doi:10.1371/journal.pone.0148494. [Google Scholar] [PubMed] [CrossRef]
65. Zheng GH, Zhang HY. Changes in ultrastructure of loquat young fruits under different lower temperature stress. J Fujian Agricult Forest Univ. 2008;37(5):473–6 (In Chinese). [Google Scholar]
66. Wu LL, Zhu ZY, Lei YH, Zhu ZH. Research progress on physiological responses of plants to low temperature and drought stress and 5-aminolevulinic acid response to stress mitigation. Anhui Agricult Sci Bull. 2021;27(17):26–9 (In Chinese). [Google Scholar]
67. Zhang JT, Wei X, Zhang HW, Liang RL, An YY, Wang LJ. Mitigative effect of 5-aminolevulinic acid pretreatment on chilling injury of young leaves in peach. J Fruit Sci. 2022;39(8):1407–21. [Google Scholar]
68. Wu Y, Liao W, Dawuda MM, Hu L, Yu J. 5-Aminolevulinic acid (ALA) biosynthetic and metabolic pathways and its role in higher plants: a review. Plant Growth Regulat. 2019;87(2):357–74. doi:10.1007/s10725-018-0463-8. [Google Scholar] [CrossRef]
69. Song W, Shan CH, Ning M, Zhang Q, Zhou FK, Tang FX. Advances in research of glutathione-S-transferase in response to cold stress in plants. The Food Indus. 2020;41(7):239–44. [Google Scholar]
70. Zhang X, Tao L, Qiao S, Du BH, Guo CH. Roles of glutathione S-transferase in plant tolerance to abiotic stresses. China Biotechnol. 2017;37(3):92–8 (In Chinese). [Google Scholar]
71. Cao BZ. Effects of exogenous 5-aminolevulinic acid (ALA) on the AsA-GSH circulation in loquat leaves under the stress of low temperature. Fujian Agricult Sci Technol. 2021;52(11):7–13 (In Chinese). [Google Scholar]
72. de Smidt O, du Preez JC, Albertyn J. The alcohol dehydrogenases of Saccharomyces cerevisiae: a comprehensive review. FEMS Yeast Res. 2008;8(7):967–78. doi:10.1111/j.1567-1364.2008.00387.x. [Google Scholar] [PubMed] [CrossRef]
73. Liu L, Chen J, Gu C, Wang S, Xue Y, Wang Z, et al. The exocyst subunit CsExo70B promotes both fruit length and disease resistance via regulating receptor kinase abundance at plasma membrane in cucumber. Plant Biotechnol J. 2024;22(2):347–62. doi:10.1111/pbi.14189. [Google Scholar] [PubMed] [CrossRef]
74. Liu J-X, Feng K, Duan A-Q, Li H, Yang Q-Q, Xu Z-S, et al. Isolation, purification and characterization of an ascorbate peroxidase from celery and overexpression of the AgAPX1 gene enhanced ascorbate content and drought tolerance in Arabidopsis. BMC Plant Biol. 2019;19(1):488. doi:10.1186/s12870-019-2095-1. [Google Scholar] [PubMed] [CrossRef]
75. Li ZQ, Li JT, Bing J, Zhang GF. The role analysis of APX gene family in the growth and developmental processes and in response to abiotic stresses in Arabidopsis thaliana. Hereditas. 2019;41(6):534–47. [Google Scholar]
76. Nianiou-Obeidat I, Madesis P, Kissoudis C, Voulgari G, Chronopoulou E, Tsaftaris A, et al. Plant glutathione transferase-mediated stress tolerance: functions and biotechnological applications. Plant Cell Rep. 2017;36(6):791–805. doi:10.1007/s00299-017-2139-7. [Google Scholar] [PubMed] [CrossRef]
Cite This Article
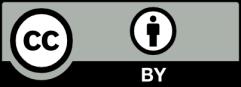
This work is licensed under a Creative Commons Attribution 4.0 International License , which permits unrestricted use, distribution, and reproduction in any medium, provided the original work is properly cited.