Open Access
ARTICLE
Effects of Water-Fertilizer Coupling on Growth Characteristics and Water Use Efficiency of Camellia petelotii Seedlings
1 Guangxi Key Laboratory of Forest Ecology and Conservation, Key Laboratory of National Forestry and Grassland Administration on Cultivation of Fast-Growing Timber in Central South China, College of Forestry, Guangxi University, Nanning, 530004, China
2 School of Architecture Engineering, Guangxi University of Nationalities, Nanning, 530006, China
* Corresponding Authors: Yijin Wang. Email: " />; Linghui Wang. Email:
" />
# These authors contributed equally to this work
(This article belongs to the Special Issue: Plant Secondary Metabolism and Functional Biology)
Phyton-International Journal of Experimental Botany 2024, 93(11), 2927-2947. https://doi.org/10.32604/phyton.2024.056429
Received 23 July 2024; Accepted 21 October 2024; Issue published 30 November 2024
Abstract
Camellia petelotii (Merr.) Sealy is an endangered Chinese native species that originates from Guangxi Zhuang Autonomous Region, China. Previous research demonstrated that proper water and fertilizer treatments could improve the growth and quality of Camellia species. This study uses a three-factor, five-level quadratic rotational combination experimental design to investigate the impact of water-fertilizer coupling on plant growth characteristics and the most suitable treatment for 24-month-old grafted C. petelotii seedlings. The experimental design includes irrigation levels [30%, 40%, 55%, 70%, 80% of field capacity (FC)], nitrogen application (0, 2.17, 5.43, 8.70, 10.87 g·plant−1), and phosphorus application (0, 0.96, 2.40, 3.85, 4.81 g·plant−1). The results indicated that: (1) Water-nitrogen and water-phosphorus interactions significantly affected ground diameter, chlorophyll content and specific leaf weight (SLW), while water-nitrogen interactions significantly affected plant height and photosynthesis; (2) Application of nitrogen (8.70 g·plant−1) and phosphorus (3.85 g·plant−1) fertilizers under appropriate irrigation conditions (40% FC and 70% FC) improved growth. Applying fertilizers containing either nitrogen (10.87 g·plant−1) or phosphorus (4.81 g·plant−1) under adequate irrigation (55% FC) increased the Chl content. However, high nitrogen levels (10.87 g·plant−1) reduced photosynthesis. Conversely, it was enhanced under appropriate phosphorus (4.81 g·plant−1) when the irrigation level was 55% FC, indicating the sensitivity of C. petelotii seedlings to nitrogen fertilizer. (3) Under specific conditions of 40% FC or 70% FC irrigation and 8.70 g·plant−1 or 2.17 g·plant−1 nitrogen fertilizer application, 3.85 g·plant−1 phosphorus addition boosted the SLW whereas, 0.96 g·plant−1 phosphorus addition inhibited it. Under W = 55% FC, deficiencies in either nitrogen (N = 0 g·plant−1) or phosphorus (P = 0 g·plant−1) significantly decreased leaf growth, affecting SLW. In summary, C. petelotii was more sensitive to nitrogen fertilizer at W = 55% FC, and nitrogen deficiency inhibited C. petelotii growth in terms of ground diameter more than phosphorus deficiency. The C. petelotii seedlings performed best when treated with 55% FC, 5.43 g·plant−1 nitrogen, 2.40 g·plant−1 phosphorus per plant. These parameter estimates could optimize water and fertilizer application for C. petelotii seedlings.Keywords
Camellia petelotii (Merr.) Sealy (family Theaceae) is an evergreen Chinese native shrub or tree species that belongs to Camellia sect. chrysantha Chang. It shows a high tolerance for shade and commonly inhabits shaded gullies, valleys, and under-forest environments. The International Union for Conservation of Nature (IUCN) Red List of Threatened Species has listed this species as Endangered (EN) [1,2]. The plant has a high aesthetic, medicinal, and nutritional value. It is an ornamental tree whose flowers are valued as a precious raw material for health drinks. It has recently spread significantly throughout southern China (including Guangdong, Yunnan, Fujian, and numerous other provinces) and a few others (such as Japan, USA, Australia, and France) [3,4]. Therefore, C. petelotii has high development value and immense market potential.
Proper applications of water and nutrients are the most significant factors during the cultivation of C. petelotii seedlings. Previous research has shown that C. petelotii requires high moisture levels and is drought intolerant at 15.04% to 16.54% of drought stress [5]. Sufficient water can increase the nutrient uptake of seedlings and plant cell elongation [6,7]. For example, proper irrigation (80%–85% field capacity, FC) can enhance the leaf water transport efficiency and photosynthesis of C. oleifera seedlings [8]. Furthermore, C. petelotii favors appropriate nutritional conditions in addition to medium-fertile soil. For example, potassium and compound nutrients [(N/P/K: 16/8/6) 150 g] could improve the growth of 24-month-old C. petelotii cutting seedlings [9]. Regarding nutrients, nitrogen is an essential constituent of proteins and chlorophyll, and it can also affect photosynthesis in some Camellia species. 22 g of nitrogen fertilizer [(NH4)2SO4] and 8 mmol·L−1 N (4 mmol·L−1 NH4Cl + 4 mmol·L−1 KNO3) per pot were found to enhance ideal leaf growth and photosynthetic conditions for Camellia sinensis and Camellia oleifera, respectively [10,11]. Another nutrient, phosphorus, was also shown to promote plant quality. Phosphorus fertilizer use boosted floral bud formation and fruit ripening in Camellia oleifera, significantly improving yield and quality [12]. The plant responses to phosphorus levels vary depending on the nitrogen level and species under consideration. C. oleifera may show higher nutrient accumulation with moderate phosphorus (0.4, 0.8 mmol·L−1) under nitrogen deficit, but the change was not significant with phosphorus under adequate nitrogen supply (8 mmol·L−1) [13]. Thus, nitrogen and phosphorus exhibit considerable complementarity, and promoting plant growth and fertilizer utilization requires a rational fertilizer mix. Additional research has shown that the interplay of water and nutrients can significantly affect growth, physiology, and economic yields. C. sinensis produced the highest amount of dry matter and grew optimally with 0.485 g·pot−1 N, 0.274 g·pot−1 P, and watering every 4~5 days [14]. To summarize, appropriate water-fertilizer coupling can promote water absorption, alleviate moisture stress, and enhance the nutritional status of seedlings. Water can improve the transport efficiency of nutrients in plants, and the right combination of water and nutrients is crucial to plant growth and development during the seedling stage.
C. petelotii cultivation research is currently focused on breeding [15,16], resistance [17,18], and fertilization studies [19,20]. However, it is still unclear how water-fertilizer coupling affects the growth of C. petelotii seedlings. C. petelotii requires more nitrogen fertilizer than other nutrients to promote leaf development and metabolite synthesis [21]. Research has shown that while the phosphorus content of tea is significantly lower than nitrogen content, the use of phosphorus fertilizer is highly correlated with improved tea growth and is crucial for respiration, photosynthesis, and other mechanism processes [22,23]. To summarize, it is critical to investigate appropriate water-fertilizer coupling conditions to promote growth and water utilization in C. petelotii and other Theaceae family species. Therefore, the objectives of this study were to (1) determine the effect of water and fertilizer coupling on growth characteristics and water use efficiency in the seedling stage of C. petelotii and (2) identify the best combination of water and fertilizer for seedling growth in this valuable shrub.
The experiment was conducted from 25 February to 25 December 2015, at the teaching nursery of the College of Forestry, Guangxi University (22°52′ N, 108°17′ E). The test site has a subtropical monsoon climate that is mild and humid, with a mean annual temperature of 21.6°C and a mean annual precipitation of 1304 mm, ideal for the growth of C. petelotii seedlings.
Guangxi State-owned Liuwan Forestry (Yulin, China) provided 24-month-old grafted seedlings of C. petelotii (plant height 16.38 ± 5.18 cm, ground diameter 3.71 ± 0.72 mm), with Camellia oleifera rootstock and C. petelotii scion.
Each pot measured 23 cm (top diameter) × 17 cm (bottom diameter) × 25 cm (height) and had a soil bulk density of 1.54 g·cm−3. Each pot included 9 kg of mixed substrate [fine sand: yellow soil = 1:2 (v/v)]. The College of Forestry’s Teaching Nursery supplied the Fine Sand and Yellow Soil. The basic physicochemical properties of the substrate were: soil bulk density of 1.54 g·cm−3, field water holding capacity (FC) of 25.1%, pH of 5, total nitrogen of 0.79 g·kg−1, total phosphorus of 0.17 g·kg−1, and total potassium of 1.03 g·kg−1.
Tianjin Damao Chemical Reagent Factory produced the fertilizers used. They included nitrogen (urea: N 46%, analytical pure reagent), phosphate (calcium superphosphate: 16% P2O5, experimental reagent), and potassium (potassium sulfate: K2O 60%, analytical pure reagent).
This experiment has three components, each with five levels. A full factorial design requires 125 treatments but an orthogonal experimental design involves 25 treatment groups. Therefore, the study employed a quadratic regression generalized rotational combinatorial design, widely acknowledged as the most efficient approach for examining multifactorial interaction effects. This approach can lower the number of required tests while maintaining efficiency [24,25]. The experiment used a three-factor (watering, nitrogen, and phosphorus), five-level quadratic rotational combination design. Five levels (−γ, −1, 0, 1, and γ) were established for each factor, with γ representing the highest level. The quadratic equation for the same can be stated as follows:
where m represents the experiment factor (m = 3 in this experiment), xj (j = 1, 2, ..., m) represents the independent variable, and y represents the experiment indication (dependent variable) [26].
The regression coefficients, denoted as a, bj, bkj, and bjj, are represented as 1 + m + m(m−1)/2 + m =
where mc = 2m is the experiment times of the orthogonal test with each factor at 2 levels (treatment 1–8 in Table 1), mγ = 2m is the experiment times of the orthogonal test related to level γ (treatment 9–14 in Table 1), and m0 is the experiment times of the zero-level trials. This experiment used five irrigation levels [30%, 40%, 55%, 70%, and 80% of the field capacity (FC, 25.1%)]. Nitrogen and phosphorus were each set at five levels, totaling 20 treatments. Each treatment had five biological replicates (one basin per replicate), totaling 100 plants (Tables 1–4). Phosphorus fertilizer was applied twice, at a constant rate of 0.75 g·plant−1 for each treatment. For the test, seedlings with uniform growth and good health, arranged in completely randomized blocks, were placed in a shed covered in a layer of shade net (Anhui Nongfeng Shade Equipment Co., Ltd., Hefei, China) with 67.5% natural light. The soil moisture content was determined using a TDR soil moisture tachymeter, and the average of the four directions of east, west, north, and south was measured every three days. If the results were less than the value set for the experiment, watering was replenished, with watering amount = (field capacity − soil moisture content) * potting soil weight.
The plant height and ground diameter were measured on 25 February 2015, and 25 December 2015, respectively, using a steel tape to within 0.1 cm and an electronic Vernier caliper to within 0.01 mm. All the seedlings should be measured (with three biological replicates for each plant). relative increments in plant height and ground diameter were calculated using the following formulas:
Rph and Rgd represent the relative increment of plant height and ground diameter, respectively. A1 and B1 represent the plant height and ground diameter before the experimental treatment, while A2 and B2 represent the plant height and ground diameter at the end of the experiment.
For each treatment, we selected three seedlings with similar growth status and collected the second to fifth mature functional leaves from the top bud of the upper branch of the plant. We used three biological replicates for each treatment. The leaf area (LA) was measured using a Li-3000A portable leaf area meter. After selecting the leaves, the fresh weight (M0) was measured using an electronic induction balance with an accuracy of 0.0001 g. The leaves were then left at room temperature and weighed at specific intervals (0.5, 4.5, 8.5, 20.5, 32.5, 44.5, 56.5, and 68.5 h) to determine their water-holding capacity. The leaves were dried at 65°C~70°C until a constant weight was reached. We measured the final dry mass (Md), and calculated the leaf water content (LWC). Leaf water loss rate, specific leaf weight (SLW) and LWC were calculated using the following formulas:
where M is the fresh weight of leaves measured at each time point [27–29].
The photosynthesis indexes were calculated using three biological replicates for each treatment. The chlorophyll content was determined using a combined acetone-ethanol extraction method [30]. The second to fifth mature functional leaves were randomly collected from the top bud of three seedlings with similar growth status. About 0.2 g of fresh leaf tissue (excluding the vein) was cut into pieces and added to the extraction solution (80% acetone: 95% ethanol = 1: 1 [v/v]) and placed in the dark for more than 24 h. We measured the absorbance at 645 and 663 nm and calculated the chlorophyll content in the samples using the formulas:
At the end of December 2015, the Pn, Gs, Tr, and Ci of C. petelotii seedlings were measured from 9.30 a.m. to 11.30 a.m. using the Lico-6400 Convenient Photosynthesis Analyser System.
Microsoft Excel 2019 was used to organize the experimental data and create tables. SAS 9.4 is employed to conduct main effects analysis and assess the relationships among factors using the response surface regression analysis (RSREG procedure). One-way analysis of variance (ANOVA) and Tukey’s test with a significance level set at 0.05 were used to conduct significance analysis and post hoc comparisons and perform significance analysis in IBM SPSS 26.0. Finally, Origin 2022 was used for data visualization and charting. The study employed the following regression analysis model to examine the relationship between several variables and soil moisture content, nitrogen application rate, and phosphorus application rate:
In the above equation, y is the response variable;
Distinct treatments showed highly significant differences (p < 0.01) in the relative increments of plant height and ground diameter. Water, nitrogen, and phosphorus exhibited significant effects on growth indexes (p < 0.01), with the water-nitrogen interaction affecting plant height and the water-nitrogen, water-phosphorus interaction affecting ground diameter (p < 0.01) (Fig. 1 and Table A2). T13 (W = 55% FC + N = 5.43 g·plant−1 + P = 4.81 g·plant−1) treatment resulted in the highest relative increments of plant height (52.8%, increase by 9.25 cm, 15% higher than the control). In comparison, T2 (W = 70% FC + N = 8.70 g·plant−1 + P = 0.96 g·plant−1) treatment resulted in the highest relative increments of ground diameter (53.7%, increase by 2.04 mm, 70.5% higher than the control). The relative growth increment initially increased and then decreased with increasing irrigation levels (T10→T15–T20→T9). While the relative increment of plant height first increased and then decreased, the relative increment of ground diameter gradually increased with the increase in nitrogen application (T12→T15–T20→T11). Increased phosphorus treatment resulted in a progressive increase in plant height but an increase followed by a decrease in ground diameter (T14→T15–T20→T13). In summary, 30%–40% FC restricted the growth of C. petelotii seedlings. However, nitrogen (8.7 g·plant−1) and phosphorus (3.85 g·plant−1) fertilizers with W = 40% or W = 70% can boost height growth. Nitrogen deficiency had a more significant impact on C. petelotii seedlings growth than phosphorus deficiency.
Figure 1: Relative increment of plant height and ground diameter under different coupling conditions of water and fertilizer
Note: Different lowercase letters indicate that the differences between treatments are significant at the p < 0.05 level, similarly hereinafter.
The regression models were examined and analyzed with soil moisture content (
The regression coefficients for
There were highly significant differences (p < 0.01) between the various treatments regarding Chl a, Chl b, and Chl a + b. Fig. 2 shows the results of the multifactor analysis of variance. T2 (W = 70% FC + N = 8.70 g·plant−1 + P = 0.96 g·plant−1) treatment resulted in maximum Chl a (4.52 mg·g−1, 35.7% higher than the control), and Chl a + b content (6.08 mg·g−1, 36.3% higher than the control), while T4 (W = 70% FC + N = 2.17 g·plant−1 + P = 0.96 g·plant−1) treatment resulted in the highest Chl b content (1.58 mg·g−1, 39.8% higher than the control). The chlorophyll content first increased and then decreased with the increasing irrigation levels (T10→T15–T20→T9). Chl a, Chl b, and Chl a+b content decreased and then increased when nitrogen application increased (T12→T15–T20→T11). Chl a and Chl a + b initially increased, then declined, while Chl b decreased and then increased as phosphorus application increased (T14→T15–T20→T13). Irrigation with 30% FC significantly inhibits the photosynthetic potential of C. petelotii seedlings. When irrigation level was adequate (55% FC), independently applying nitrogen (10.87 g·plant−1) or phosphorus (4.81 g·plant−1) fertilizer had a significant favorable effect on chlorophyll production. Nitrogen and phosphorus fertilizers have a complementary impact on chlorophyll synthesis. Applying nitrogen and phosphorus fertilizers simultaneously at constant water content (W = 40% FC or W = 70% FC) either insufficiently (N = 2.17 g·plant−1, P = 0.96 g·plant−1) or excessively (N = 8.70 g·plant−1, P = 3.85 g·plant−1) inhibited the synthesis of chlorophyll. However, an appropriate combination of nitrogen and phosphorus can help boost chlorophyll levels.
Figure 2: Changes of chlorophyll content under different coupling conditions of water and fertilizer
The regression model was examined and analyzed with soil moisture content (
The regression coefficients for
3.3 Photosynthetic Characteristics
Between the various treatments, Pn, Tr, Gs, and Ci showed highly significant differences (p < 0.01), and Fig. 3 displays the multifactor variance analysis results. The values of Tr (5.29 mmol·m−2·s−1, 163.2% higher than the control) and Gs (0.30 mol·m−2·s−1, 500% higher than the control) reached the maxima under T15 (W = 55% FC + N = 5.43 g·plant−1 + P = 2.40 g·plant−1) treatment. The values of Pn (5.59 umol·m−2·s−1, 133.9% higher than the control) and Ci (314.33 umol·mol−1, 58.2% higher than the control) reached the maxima under T12 (W = 55% FC + N = 0 g·plant−1 + P = 2.40 g·plant−1) and T18 (W = 55% FC + N = 5.43 g·plant−1 + P = 2.40 g·plant−1) treatments, respectively. Overall, Pn, Tr, and Gs first increased and then decreased while Ci showed a decreased-increased-decreased trend with increasing irrigation level (T10→T5–T8→T11–T20→T1–T4→T9). Tr, Gs, and Ci initially increased before decreasing, but Pn gradually and continuously declined as nitrogen application increased (T12→T15–T20→T11). However, increased phosphorus application caused Tr, Gs, Ci, and Pn to increase initially, then decrease (T14→T15–T20→T13). Higher nitrogen (8.7 g·plant−1) and phosphorus (3.85 g·plant−1) levels led to enhanced Pn, Gs, and Ci with 70% FC. Low irrigation levels (40% FC) inhibited the photosynthesis in C. petelotii seedlings. Though under W = 55% FC, adding more phosphorus fertilizer (4.81 g·plant−1) increased photosynthesis, adding nitrogen fertilizer (10.87 g·plant−1) decreased it. This suggests that C. petelotii seedlings need more phosphorus and are more sensitive to nitrogen fertilizers.
Figure 3: Effects of different water and fertilizer coupling on photosynthetic characteristics of leaves
The regression models were examined and analyzed with soil moisture content (
The regression coefficients for
3.4 Leaf Moisture Content and Specific Leaf Weight
The leaf water content (LWC) did not differ significantly (p > 0.05) across treatments, but we observed significant differences (p < 0.01) in specific leaf weight (SLW) between treatments. The multifactorial analysis of variance indicated that the water main effect significantly impacted the LWC of C. petelotii seedlings at 44.5 h (p < 0.05) (Fig. 4). The LWC reached its maximum under T15 (W = 55% FC + N = 5.43 g·plant−1 + P = 2.40 g·plant−1) treatment (64%, 18.5% higher than the control), while the SLW reached its maximum under T13 (W = 55% FC + N = 5.43 g·plant−1 + P = 4.81 g·plant−1) treatment (1.73%, 58.7% higher than the control). The LWC increased and then decreased with increasing soil water content (T10→T15–T19). The SLW increased and subsequently reduced as the irrigation level increased (T10→T15–T20→T9). At W = 17.57%, SLW increased progressively as nitrogen and phosphorus levels rose. C. petelotii seedlings had higher water-holding capacity in dry conditions (30% FC) than in settings with higher irrigation levels. When fertilizer levels were constant, the SLW increased with increasing irrigation levels. The SLW increased with increasing phosphorus application at a specific irrigation and nitrogen fertilizer application level. Leaf growth was severely restricted by insufficient nitrogen or phosphorus under appropriate irrigation, impacting the SLW (T11–T12, T13–T14).
Figure 4: Effects of different water-fertilizer coupling on leaf water content and specific leaf weight
The regression model was examined and analyzed using soil moisture content (
The regression coefficients for
We summarized and comprehensively analyzed the data using the membership function. The mean of the membership function of each growth index was used as a comprehensive evaluation index for C. petelotii seedlings under various water-fertilizer coupling treatments. Table 5 shows that T15 (W = 55% FC + N = 5.43 g·plant−1 + P = 2.40 g·plant−1) achieved the highest composite score, indicating that T15 is the optimal water and fertilizer condition for the growth of C. petelotii seedlings.
Fig. 5 depicts the correlation analysis among the indicators. Photosynthetic characteristics showed a highly positive significant link with a relative increment of plant height and ground diameter, as well as LWC and SLW. LWC indicators had a highly positive considerable influence on other indicators, except for Chl a + b. The leaf photosynthetic parameters showed a high correlation, with Gs having a more substantial impact on Pn than chlorophyll content. Higher Gs allowed more carbon dioxide into the leaves, resulting in an increased rate of photosynthesis. Higher rates of photosynthesis imply that the leaves may use water more efficiently to produce nutrients, which may help maintain or increase the LWC. On the other hand, increased of photosynthetic products can promote leaf growth and thickness, ultimately affecting SLW. Thicker leaf structure boosts photosynthetic efficiency, which aids overall plant growth and encourages plant height and ground diameter. The relative increment in plant height and ground diameter positively correlated with other indicators. The interactions between total chlorophyll, photosynthesis, LWC, and SLW promoted the growth in height and ground diameter of C. petelotii seedlings.
Figure 5: Correlation heat map of various indexes
Note: Orange (0 < r ≤ 1) indicates positive correlation, blue (−1 ≤ r < 0) indicates negative correlation, white indicates no correlation (r = 0), and the darker the colour indicates the stronger the correlation; * and ** indicate that the difference is significant at the levels of p < 0.05 and p < 0.01, respectively.
Principal component analysis was performed using relative increments of plant height and ground diameter, Chl a + b content, photosynthetic indexes, SLW, and LWC of C. petelotii (Fig. 6). The 20 treatment groups were scattered throughout the axes at various points, suggesting considerable treatment variability, with all nine markers positioned at the positive end of the x-axis. T18 had the most significant impact on the relative growth of plant height and ground diameter. T16 had the most significant impact on Ci, Gs, and Tr growth. T17 had the greatest effect on Pn, SLW, and LWC growth while T20 had the biggest impact on Chl a + b levels. The two primary components exhibited contribution rates of 52.6% and 20.1% for a cumulative contribution rate of 72.7%. It indicates that the two primary components listed above can cover most of the attributes associated with developing C. petelotii seedlings.
Figure 6: Principal component analysis of the growth characteristics
4.1 Effect of Water-Fertilizer Coupling on C. petelotii Growth
Plant height and ground diameter can reflect seedling growth and quality, whereas optimal water-nutrient interaction can boost plant growth at the seedling stage. This study found that water deficit (30%~40% FC) significantly decreased seedling growth. However, appropriate fertilization (N = 8.7 g·plant−1, P = 0.96 g·plant−1) might mitigate the adverse effects. Similar research on Robinia pseudoacacia and Gossypium hirsutum seedlings revealed severe growth limitations and biomass reduction when treated with water-fertilizer coupling under water deficiency at 30% FC and −0.2 MPa, respectively. G. hirsutum treated with 85 mmol·L−1 N effectively mitigated the inhibitory effects of drought on plant height and ground diameter [31,32]. We observed that water-nitrogen interaction significantly promoted seedling growth, and applying nitrogen fertilizer (10.87 g·plant−1) promoted relative increments in plant height and ground diameter in C. petelotii seedlings at reduced irrigation levels (55% FC). Seedlings of Populus tomentosa carr and Macleaya cordata (Willd) R.Br. also exhibit increased height and ground diameter growth under treatments of 55% FC + 5 g·plant−1 N and 30%~40% FC + 0.2 g·kg−1 N, respectively [14,33]. This shows that drought inhibits C. petelotii growth, whereas nitrogen application increases the available soil nitrogen. The absorption of this increased exogenous nitrogen by the root system promotes the growth and development of C. petelotii seedlings, thereby mitigating the damage to seedlings caused by drought stress [34]. Previous studies have shown that nitrogen promotes plant cell division and growth, whereas phosphorus accelerates cell division and stimulates aboveground growth [35]. Nutrients dissolved in water reach the root system’s surface via mass flow or diffusion, and the appropriate amount of irrigation and fertilizer application stimulates ion diffusion of K+ and H2PO4−, which promotes nutrient uptake and plant growth [36]. We hypothesized that C. petelotii seedlings reduced the energy required for survival by slowing plant height and ground diameter growth under low irrigation levels and that adding appropriate nitrogen/phosphorus fertilizers can alleviate the inhibitory effects of low irrigation levels on plant height and stem diameter. Meanwhile, fertilizer application may boost soil water potential and efficacy by transforming “ineffective” water for plant growth into “effective” water [37].
4.2 Effects of Water-Fertilizer Coupling on C. petelotii Photosynthesis
Photosynthesis is a source of energy and material for plant growth, and it plays a significant part in plant growth and development. In contrast, chlorophyll is the action ingredient for photosynthesis in plants, which impacts photosynthetic capacity [38,39]. Studies have indicated that providing plants with sufficient water and nutrients may promote a rise in chlorophyll levels, improving photosynthesis and promoting their nutritional development [40,41]. This study found that water and phosphorus, water-nitrogen. and nitrogen-phosphorus interactions significantly affected the chlorophyll content. Similar results were reported in a study on water and fertilizer in Accinium corymbosum seedlings [42]. Chlorophyll content decreased with decreasing irrigation levels, as demonstrated in the study on Aglaia duperreana [43]. Low water stress caused changes in the morphological structure of chloroplasts and photosynthetic parameters such as Pn, Ci, and Gs in C. petelotii seedlings [44]. It indicates that C. petelotii leaves that received adequate water and fertilizer had higher Gs leaves. Conversely, a low water environment reduced the Gs, chlorophyll content, and light energy absorption. It weakened the Pn of C. petelotii leaves, inhibiting the growth and development of the seedlings. The high nitrogen application group (8.7 g·plant−1) had a substantially higher chlorophyll content than the low nitrogen application group (2.17 g·plant−1) under low irrigation (40% FC) and low phosphorus application (0.96 g·plant−1). Similarly, high nitrogen (0.8 g·kg−1 N) treatment significantly reduced the inhibitory effects of drought and phosphorus deficit on the chlorophyll synthesis in Robinia pseudoacacia [45]. It implies that nitrogen can promote chlorophyll accumulation and that high nitrogen applications may reduce the inhibitory effect of low water and low phosphorus on chlorophyll production in C. petelotii leaves. Under low phosphorus conditions, the phosphorus uptake efficiency of seedlings may be higher, ensuring an adequate supply of phosphorus to the plant, which stimulates the nitrogen uptake required by C. petelotii. Low moisture inhibits chlorophyll biosynthesis and accelerates the decomposition of the original chlorophyll. Nitrogen application can boost chlorophyll synthesis and raise the nitrogen content of plant leaves, thus effectively regulating the light and performance, alleviating the light damage caused by drought stress on plants, and promoting C. petelotii leaf growth [46,47]. This study found that regardless of the irrigation level (40% FC or 70% FC), applying a decreased phosphorus administration rate (0.96 g·plant−1) resulted in higher chlorophyll content when supplemented with a relatively high nitrogen application rate (8.7 g·plant−1). In contrast, higher phosphorus administration (3.85 g·plant−1) increased chlorophyll content when the nitrogen application rate was lower (2.17 g·plant−1). Zea mays and Oryza sativa research followed the same trend as C. petelotii [48,49]. In Oryza sativa, lowering the phosphorus level below 45.0 kg·hm−2, the yield first increases and subsequently decreases as the nitrogen content increases. We postulated that the nitrogen-phosphorus interaction negatively affects the total chlorophyll content. Therefore, it is critical to maintain a proper balance between nitrogen and phosphorus applications. Our study found that lower moisture reduced photosynthetic characteristics regardless of nitrogen fertilizer content. Applying nitrogen fertilizer (8.7 g·plant−1) accelerated photosynthesis under sufficient water (70% FC) conditions but limited photosynthesis under low-water (W < 40%) conditions. A similar water-nitrogen coupling study in Picea asperata showed that nitrogen application boosts Pn under well-watered (W > 60% FC) conditions but has a detrimental under heavy water stress (40% FC) [50]. Nitrogen application can increase chlorophyll content, which leads to enhanced photosynthetic activity and light absorption by plant chloroplasts, resulting in higher Pn and increased photosynthetic capacity, which contributes to photosynthetic product accumulation and promotes plant growth [51]. Water significantly affected Pn, Tr, Gs, and Ci, and applying nitrogen fertilizer in low moisture conditions, enhanced water stress. This resulted in a drop in C. petelotii’s leaf water content (LWC) and increased water loss in the guard cells, closing the stomata and limiting CO2 entrance into the leaves. It subsequently increased in inter-cellular CO2 levels, limiting the rate of photosynthesis. T9 (W = 80% FC) did not exhibit a higher photosynthetic rate, most likely because the tissue was saturated with moisture and the stomata closed passively, which hindered the entry of CO2 into the chloroplasts, lowering the photosynthetic rate. Pn, Tr, and Gs values for T10 decreased. At the same time, Ci increased, which revealed that stomatal restriction values reduced at this moisture level, with non-stomatal limitation becoming the primary factor affecting C. petelotii photosynthesis. Consequently, both insufficient and excessive moisture significantly impacted photosynthesis and impeded the capacity to accumulate nutrients, limiting the development of C. petelotii seedlings.
4.3 Effects of Water-Fertilizer Coupling on Water Use Efficiency in C. petelotii
Plants respond to water stress by altering their water use efficiency, and LWC and SLW are key indices of plant adaptation to environmental changes [52]. This study demonstrated that water has a more significant impact than nitrogen. Treating the SLW of Populus tomentosa and Toona sinensis with water-fertilizer coupling yielded similar results. Their SLW increased with increasing irrigation levels but decreased when treated with drought conditions [33,53]. Low irrigation levels (30% FC) resulted in compacted soil due to the lack of moisture, causing cell membrane damage and lowering the SLW. Conversely, SLW significantly increased when irrigation was at 55% FC, indicating an increase in dry matter per unit leaf area and leaf thickness. These alterations are beneficial for resisting excessive transpiration and enhancing the water storage capacity of C. petelotii leaves. A positive correlation exists between the dry matter per unit leaf area (SLW), the CO2 conversion rate, and water usage efficiency [54]. This study showed that the water-nitrogen interaction significantly affected the SLW, with a trade-off effect. Applying nitrogen fertilizer (5.43 g/plant) at lower water content (55% FC) significantly increased the SLW, but decreased with the increase of nitrogen fertilizer level. Similarly, the SLW of Machilus pauhoi seedlings attained a maximum with the fertilizer application (N:P = 8) at 40% FC moisture condition and decreased after that as nitrogen levels increased [55]. We postulated that increases in nitrogen fertilizer cause the saturation of the plant’s total nitrogen absorption, resulting in increased nitrogen accumulation without commensurate rational usage. Applying nitrogen within the recommended range can stimulate cell division in leaf mesophyll cells, delay the formation and enlargement of spaces between cells, and enhance the leaf, palisade, and spongy tissue thickness. However, there was a significant positive correlation between leaf thickness, leaf dry matter content, and leaf nitrogen content [56]. Nitrogen fertilizer application facilitates nitrogen transport through the roots, increasing leaf nitrogen content. This enhances the plant’s photosynthetic efficiency and stimulates the production of a significant amount of chlorophyll and photosynthetic proteins in the leaves. Consequently, the leaf nitrogen content further increases, which causes the leaves to get thicker. Our study found that the nitrogen-phosphorus interaction considerably impacted the SLW. The administration of nitrogen, especially in combination with phosphorus fertilizer, had a similar impact on the SLW of Glycine max [57]. The simultaneous application of nitrogen and phosphorus improves phosphorus availability in the soil, resulting in less nitrogen consumption and a higher nitrogen content in plant leaves [58]. Hence, the proper application of nitrogen or phosphorus fertilizer improved the nutritional composition of the soil, resulting in increased nutrient transfer to the above-ground section. Consequently, this could amplify the buildup of organic matter in the leaves, boosting the SLW. The rise in nutrient content will simultaneously impact plant photosynthesis, enhancing the rate of photosynthesis and facilitating plant growth and development. Consequently, this will also lead to an increase in SLW. In the present study, neither low nor high moisture levels significantly affected the LWC of C. petelotii. A similar experiment on water-fertilizer coupling in Acer rubrum ‘Red Champions’ showed no significant differences among treatments in LWC during the early stage of color change [59]. All treatments showed higher LWC, which, according to a prior study, shows that maintaining higher LWC mitigates the negative effects of low water levels on seedling cell membranes. This indicates a high water-holding capacity and resistance to drought stress [60]. Nevertheless, C. petelotii naturally prefers a humid environment and has a high water requirement. Transgenic wheat exhibited higher LWC and water utilization efficiency when subjected to mild water stress [61]. This was attributed, in part, to higher levels of proline, soluble sugar, and soluble protein, along with lower malondialdehyde levels. The investigation of C. petelotii growth characteristics suffers from a few flaws due to the study’s restrictive focus. Thus, more research and investigation into the physiological features of LWC are required.
4.4 Shortcomings and Prospects
This study investigated the growth characteristics, leaf water utilization, and correlation between indicators of C. petelotii seedlings under various water-fertilizer coupling treatments. It reveals the growth mechanism of C. petelotii seedlings under different water and fertilizer conditions. It provides a theoretical basis and practical guidance for the future cultivation and production of C. petelotii seedlings. This work only examined the growth mechanisms for the growth indicators. Therefore, more research is needed to investigate the mechanisms of water and nutrient uptake and utilization. It failed to elucidate the molecular mechanisms of water and nutrient interactions. In-depth research into these aspects, such as plant hydraulics and nutrient uptake mechanisms, could be included in future studies. Due to the trial constraints, certain discrepancies were observed between the potting trials and the C. petelotii grown naturally outdoors. Future research should combine pot trials with field trials.
This study investigated the effects of water-fertilizer coupling on the growth and water use efficiency of 24-month-old potted C. petelotii seedlings. Applying an appropriate amount of water and fertilizer increased the plant height and ground diameter of C. petelotii. However, the different water fertilizer treatments did not significantly affect leaf water content and water-holding capacity. In summary, T15 (W = 55% FC + N = 5.43 g·plant−1 + P = 2.40 g·plant−1) was the optimum treatment, providing technical support for the investigation of the water-fertilizer coupling effect and cultivation of C. petelotii seedlings.
Acknowledgement: The authors acknowledge the following institutions for supporting this work: (1) College of Forestry, Guangxi University; (2) Key Laboratory of National Forestry and Grassland Administration on Cultivation of Fast-Growing Timber in Central South China; (3) Guangxi Key Laboratory of Forest Ecology and Conservation.
Funding Statement: Financial assistance project: Guangxi Forestry Science and Technology Project (Guilin kezi [2012] No. 25).
Author Contributions: All authors contributed to the study conception and design. Material preparation, data collection and analysis were performed by Liangyu Luo and Ru Li. Experiments were done by Ru Li and Yijin Wang. The first draft of the manuscript was written by Liangyu Luo and Daocheng Ma. Daocheng Ma and Linghui Wang revised the original manuscript. All authors commented on previous versions of the manuscript. All authors reviewed the results and approved the final version of the manuscript.
Availability of Data and Materials: The datasets generated during and/or analyzed during the current study are available from the corresponding author on reasonable request.
Ethics Approval: Not applicable.
Conflicts of Interest: The authors declare that they have no conflicts of interest to report regarding the present study.
References
1. IUCN. The IUCN red list of threatened species. 2023. Available from: https://www.iucnredlist.org/. [Accessed 2024]. [Google Scholar]
2. Wei X, Jiang Y, Wei J, Chen Z, Wang M, Zhao R. Investigation on the geographical distribution and habitat of Camellia nitidissima. Ecol Environ Sci. 2007;(3):895–9. [Google Scholar]
3. Li X, Li C, Bu Z, Huang Z, Huang C, Lu J. Analysis of amino acids composition and evaluation of nutritional value in flowers of 6 species of Camellia sect. Chrysantha Chang. Southwest China J Agric Sci. 2020;33(9):2062–8 (in Chinese). [Google Scholar]
4. Li X. On the protection and utilization of rare and endangered plant resources in Guangxi. Trop Geogr. 1995;(2):147–54. [Google Scholar]
5. Chai S, Tang J, Wang M, Chen Z, Jiang Y, Wei X. Photosynthetic and physiological characteristics of Camellia petelotii seedings under drought stress. Acta Botanica Boreali-Occidentalia Sinica. 2015;35(2):322–8 (In Chinese). [Google Scholar]
6. Duan M, Yang W, Mao X. Effects of water deficit on photosynthetic characteristics of spring wheat under plastic mulching and comparison of light response curve models. Trans Chin Soc Agric Mach. 2018;49(1):219–27 (In Chinese) doi:10.6041/j.issn.1000-1298.2018.01.027. [Google Scholar] [CrossRef]
7. Sun H, Chen X, Sun Z, Li M, Zhang M, Jing W. Rapid detection of moisture content in maize leaves based on transmission spectrum. Trans Chin Soc Agric Mach. 2018;49(3):173–8 (In Chinese) doi:10.6041/j.issn.1000-1298.2018.03.021. [Google Scholar] [CrossRef]
8. Chen Z, Ma L, Chen F, Zhou Y, Wang W, Zhang B, et al. Effects of soil water on gas exchange and water consumption of seedling leaves of Camellia oleifera. J Beijing Forest Univ. 2014;36(3):48–56 (In Chinese). [Google Scholar]
9. Zhang S, Chen C, Liu H, Ma F, Liao K, Li W, et al. Fertilization of Camellia nitidissima under Pinus massoniana Stands. Forest Sci Technol. 2016;(11):53–6 (In Chinese). [Google Scholar]
10. Xiang F, Li W, Liu H, Zhou L, Jiang C. Characteristics of photosynthetic and chlorophyll fluorescence of tea varieties under different nitrogen application levels. Acta Botanica Boreali-Occidentalia Sinica. 2018;38(8):1138–45 (In Chinese). [Google Scholar]
11. Cai D, Chen Q, Pei X, Zhang J, Gong S. Effects of different nitrogen forms on leaf anatomical structure and photosynthetic characteristics of Camellia oleifera seedlings. J Forest Environ. 2023;43(2):194–200. [Google Scholar]
12. Hu Y, Liu M, Li W, Yang X, Yang H. Effects of different fertilization methods on growth and economic characteristics of Camellia oleifera. Jiangsu Agric Sci. 2022;50(1):108–16. [Google Scholar]
13. Gai T, Zou Z, Ren Z, Xie S, Hu X, Liu J, et al. Effects of biomass and nutrient utilization of Camellia oleifera seedlings to phosphorus level under different nitrogen nutrients. Acta Agric Univ Jiangxiensis. 2022;44(3):626–34 (In Chinese). [Google Scholar]
14. Wang Y, Fu D, Pan L, Sun L, Ding Z. The coupling effect of water and fertilizer on the growth of tea plants [Camellia sinensis (L.) O. Kuntz]. J Plant Nutr. 2016;39(5):620–7. doi:10.1080/01904167.2015.1047521. [Google Scholar] [CrossRef]
15. Deng Y, Wu C, Guo J, Yao J. Experiment of cutting seedlings of Camellia nitidissima. Mol Plant Breed. 2017;15(7):2886–90. [Google Scholar]
16. Jiang H, Chai S, Xiong Z, Zou R, Yang Q, Deng L, et al. Growth, Photosynthetic characteristics and leaf morphological characteristics of Camellia nitidissima reintroduction plant. Guangxi Sci. 2023;30(6):1180–9 (In Chinese). doi:10.13656/j.cnki.gxkx.20240125.017. [Google Scholar] [CrossRef]
17. Chen J, Deng L, Li H, Long H, Xu C. Effects of shading on growth, development and physiology of two golden camellia species origined in Guizhou Province. J Nanjing Forest Univ (Nat Sci Ed). 2022;46(3):83–90 (In Chinese). [Google Scholar]
18. Tang JM, Chai SF, Zou R, Chen ZY, Shi YC, Jiang YS, et al. Effests of water stress on photosynthetic characteristics of Camellia tunghinensis seedlings. Guihaia. 2020;40(12):1764–72 (In Chinese). [Google Scholar]
19. Long HY, Deng LX, Xu CR, Chen R, Chen ZP. Effects of NPK formula fertilization on growth and root morphology of Camellia hunan. Guizhou Agric Sci. 2022;50(8):25–33 (In Chinese). [Google Scholar]
20. Chai SF, Tang JM, Zou R, Wei JQ, Tang H. Effects of different base fertilizers, and ratios of nitrogen, phosphorus and potassium topdressing on the growth of Camellia nitidissima seedlings. Hubei Agric Sci. 2020;59(7):41–5. doi:10.14088/j.cnki.issn0439-8114.2020.07.009. [Google Scholar] [CrossRef]
21. Luo J, Zhou X, Tian Y, Chen Y, Chen L. Distribution of nutrients in Camellia oleifera Abel. and their correlation with soil nutrients over the period of fruit maturation. Banglad J Bot. 2020;49(3):499–505. doi:10.3329/bjb.v49i3.49530. [Google Scholar] [CrossRef]
22. Ding Z, Jia S, Wang Y, Xiao J, Zhang Y. Phosphate stresses affect ionome and metabolome in tea plants. Plant Physiol Biochem. 2017;30(6):30–9. doi:10.1016/j.plaphy.2017.09.007. [Google Scholar] [PubMed] [CrossRef]
23. Salehi SY, Hajiboland R. A high internal phosphorus use efficiency in tea (Camellia sinensis L.) plants. Asian J Plant Sci. 2008;7(1):30–6. doi:10.1016/j.eurpolymj.2005.09.013. [Google Scholar] [CrossRef]
24. Yuan Y, Tan L, Xu Y, Dong J, Zhao Y. Optimization of processing parameters for lettuce vacuum osmotic dehydration using response surface methodology. Pol J Food Nutr Sci. 2018;68(1):15–23. doi:10.1515/pjfns-2017-0013. [Google Scholar] [CrossRef]
25. He Y, Bao YD. Study on rapeseed oil as alternative fuel for a single-cylinder diesel engine. Renew Energy. 2003;28(9):1447–53. doi:10.1016/S0960-1481(02)00223-9. [Google Scholar] [CrossRef]
26. Li Y, Hu C. Experiment design and data processing. 3rd edBeijing, China: Chemical Industry Press; 2017 (In Chinese). [Google Scholar]
27. Zheng S, ShangGuan Z. Comparison of photosynthetic physiological adaptability of leaves of Pinus tabulaeformis and robinia acacia in Loess Plateau. Chin J Appl Ecol. 2007;(1):16–22 (In Chinese). [Google Scholar]
28. Badr MA, Abou Hussein SD. Yield and fruit quality of drip-irrigated cantaloupe under salt stress conditions in an arid environment. Austr J Basic Appl Sci. 2008:141–8. [Google Scholar]
29. Jiang W, Cui S, Zhang Y, Wang S, Wang J, Hu S. Effects of KNO3, K2SO4 and their mixed salt stress on the physiological and biochemical characteristics of Pepper seedling. Acta Agric Boreali-Sinica. 2011;26(5):164–9 (In Chinese) doi:10.7668/hbnxb.2011.05.033. [Google Scholar] [CrossRef]
30. Ma D, Teng W, Yi B, Lin Y, Pan Y, Wang L. Effects of the nitrate and ammonium ratio on plant characteristics and Erythropalum scandens Bl. substrates. PLoS One. 2023;18(8):e0289659. doi:10.1371/journal.pone.0289659. [Google Scholar] [PubMed] [CrossRef]
31. Su B. Adaptation and trade-off of artificial Robinia pseudoacacia plantation to soil water and nutrient on the Loess Plateau. University of Chinese Academy of Sciences, Beijing, China; 2022. [Google Scholar]
32. Li Y. Response of growth aad chlorophyll fluorescence characteristics of cotton seedlings to coupling of water, nitrogen and phosphorus. Henan University, Zhengzhou, China; 2018. [Google Scholar]
33. Dong W. Study on coupling effects of water and fertilizer on Populus tomentosa seedlings. Beijing Forestry University, Beijing, China; 2011. [Google Scholar]
34. Tao C, Shao S, Shi W, Lin L, Tang Y, Ying Y. Effects of nitrogen deposition on biomass and protective enzyme activities of Phyllostachys edulits seedling under drought stress. Sci Silvae Sin. 2019;55(9):31–40. [Google Scholar]
35. Gentili F, Wall LG, Huss-Danell K. Effects of phosphorus and nitrogen on nodulation are seen already at the stage of early cortical cell divisions in Alnus incana. Ann Bot. 2006;98(2):309–15. doi:10.1093/aob/mcl109.doi:. [Google Scholar] [CrossRef]
36. Zhou X, Lyu T, Xing M, Song R, Fu X. Effects of water and fertilizer coupling on growth physiology and yield of Jun jujube under drip irrigation in Southern Xinjiang. J Huazhong Agric Univ. 2023;42(5):195–205 (In Chinese). doi:10.13300/j.cnki.hnlkxb.2023.05.022. [Google Scholar] [CrossRef]
37. Choi WJ, Chang SX, Allen HL, Kelting DL, Ro HM. Irrigation and fertilization effects on foliar and soil carbon and nitrogen isotope ratios in a loblolly pine stand. Forest Ecol Manag. 2005;213(1–3):90–101. doi:10.1016/j.foreco.2005.03.016. [Google Scholar] [CrossRef]
38. Hui H, Xu X, Li Q. Exogenous betaine improves photosynthesis of Lycium barbarum under salt stress. Acta Botanica Boreali-Occidentalia Sinica. 2003;23(12):2137–42. [Google Scholar]
39. Qiao Y, Liu K, Deng Z, Xu L, Su J, Rong J, et al. Photosynthetic characteristics of Phyllostachys edulis under different water and fertilizer coupling treatment. Chin J Trop Crops. 2020;41(11):2253–8 (In Chinese). [Google Scholar]
40. Wang J, Wen L, Chen P, Zhang D, Tian Y, Zeng J, et al. Effects of long-term fertilization on Soil pH, yield sustainability and quality of tea. Chin Agric Sci Bull. 2021;37(8):84–8 (In Chinese). [Google Scholar]
41. Li C, Yang W, Zhou B, Zhang Y, Lin Y, Xing S. Effects of biochar based fertilizer on soil nutrients, tea output and quality in an acidified tea field. Chin J Soil Sci. 2021;52(2):387–97 (In Chinese). [Google Scholar]
42. Luan J. Effects of water and fertilizer coupling on growth and physiological characteristics of Vaccinium corymbosum seedlings. Beihua University, Jilin, China; 2023. [Google Scholar]
43. Gan F, Zhao L, Huo C, Wang S. Effect of light and moisture on the growth of Aglaia duperreana seedlings. Chin J Appl Ecol. 2024;15(2):1–11 (In Chinese). [Google Scholar]
44. Chang Y, Yin G, Yan Y, Guo Y, Yu W, Peng Y, et al. Identification of PsaA/PsaB gene family in tobacco and its response to stress. J Shanxi Normal Univ (Nat Sci Ed). 2024;52(5):55–70 (In Chinese). [Google Scholar]
45. Su B, Wang L, Shangguan Z. Morphological and physiological responses and plasticity in Robinia pseudoacacia to the coupling of water, nitrogen and phosphorus. J Plant Nutr Soil Sci. 2021;184(2):271–81. doi:10.1002/jpln.202000465. [Google Scholar] [CrossRef]
46. Ji M, Peng L, Wen Z, Jiang H, Ge S, Jiang Y. Nitrogen and phosphorus absorption and utilization characteristics of apple rootstocks under different phosphorus levels. J Plant Nutr Fertilizers. 2015;21(4):1016–21. [Google Scholar]
47. Raese JT, Drake SR, Curry EA. Nitrogen fertilizer influences fruit quality, soil nutrients and cover crops, leaf color and nitrogen content, biennial bearing and cold hardiness of ‘Golden Delicious’. J Plant Nutr. 2007;30(10):1585–604. doi:10.1080/01904160701615483. [Google Scholar] [CrossRef]
48. Wang F, Wang S, Wang Z, Zuo Z. Effects of N, P, K fertilizer application on the production on of maize. J Arid Land Resour Environ. 2005;(4):167–71. [Google Scholar]
49. Wang W, Lu J, He Y, Li X, Li H. Effects of N, P, K fertilizer application on grain yield, quality, nutrient uptake and utilization of rice. Chin J Rice Sci. 2011;25(6):645–53 (In Chinese). [Google Scholar]
50. Tang B, Yin C, Yang H, Sun Y, Liu Q. The coupling effects of water deficit and nitrogen supply on photosynthesis, WUE, and stable isotope composition in Picea asperata. Acta Physiol Plant. 2017;39(7):375. doi:10.1007/s11738-017-2451-4. [Google Scholar] [CrossRef]
51. Zhong X, Ma X, H. LYU, Zhu C, Yang Y. Effect of nitrogen on growth and photosynthesis of Populus euphratica seedlings under drought stress. Chin J Ecol. 2017;36(10):2777–86 (In Chinese). doi:10.13292/j.1000-4890.201710.029. [Google Scholar] [CrossRef]
52. Chai H, Zhang W, Cai H. Study on the soil moisture stress level in regulated deficit irrigation experiment. Agric Sci Technol-Hunan. 2009;10(2):154–6 (In Chinese). doi:10.16175/j.cnki.1009-4229.2009.02.008. [Google Scholar] [CrossRef]
53. He R. Study on the coupling effect of water and fertilizer on Toona Sinensis at seedling stage. Central South University of Forestry and Technology, Changsha, China; 2019. [Google Scholar]
54. Reich PB, Ellsworth DS, Walters MB, Vose JM, Gresham C, Volin JC, et al. Generality of leaf trait relationships: a test across six biomes. Ecology. 1999;80(6):1955–69. doi:10.1890/0012-9658(1999)080[1955:GOLTRA]. [Google Scholar] [CrossRef]
55. Su B. Effects of different nitrogen and phosphorus ratio additions and water treatments on leaf relative water content and leaf mass area of Machilus pauho. South China Forest Sci. 2018;46(6):7–11+21 (In Chinese). [Google Scholar]
56. He C. Effects of grazing exclusion duration on plant functional traits, diversity and productivity in an alpine meadow. Lanzhou University: Lanzhou, China; 2023. [Google Scholar]
57. Zhang X. Temporal and spatial distribution of roots and feasibility study of furrow fertilization in soybean (Glycine Max L. Merril). Heilongjiang Bayi Agricultural University, Daqing, China; 2011. [Google Scholar]
58. Dong W, Zhang X, Liu X, Fu X, Chen F, Wang H, et al. Responses of soil microbial communities and enzyme activities to nitrogen and phosphorus additions in Chinese fir plantations of subtropical China. Biogeosciences. 2015;12(18):5537–46. doi:10.5194/bg-12-5537-2015 2015. [Google Scholar] [CrossRef]
59. Li L. Response of introduced Acer rubrum ‘Red Champions’ growth and coloration to water-fertilizer coupling. Southwest University: Chongqing, China; 2023. [Google Scholar]
60. Jongdee B, Fukai S, Cooper M. Leaf water potential and osmotic adjustment as physiological traits to improve drought tolerance in rice. Field Crops Res. 2002;76(2–3):153–63. doi:10.1016/S0378-4290(02)00036-9. [Google Scholar] [CrossRef]
61. Qin N. Responses to drought stress studies of transgenic wheat containing the maize C4 phosphoenolpyruvate carboxylase (PEPC) gene. Nanjing Agricultural University: Nanjing, China; 2017. [Google Scholar]
Appendix A
Cite This Article
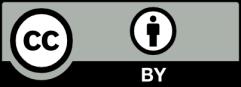
This work is licensed under a Creative Commons Attribution 4.0 International License , which permits unrestricted use, distribution, and reproduction in any medium, provided the original work is properly cited.