Open Access
ARTICLE
Critical Concentration of Zinc for Increasing Production and Quality of Mangosteen Fruit in West Sumatera, Indonesia
1 West Sumatera Mangosteen Association, Department of Plantation, Food Crops and Horticulture West Sumatera, Padang, 25157, Indonesia
2 Indonesian Agency for Agricultural Instrument Standardization, Jakarta, 12540, Indonesia
3 Research Center for Food Crops, National Research and Innovation Agency, Bogor, 16911, Indonesia
4 Research Center for Horticulture, National Research and Innovation Agency, Bogor, 16915, Indonesia
* Corresponding Author: Raden Heru Praptana. Email:
(This article belongs to the Special Issue: Influence of Biotic and Abiotic Stresses Signals on Plants and their Performance at Different Environments)
Phyton-International Journal of Experimental Botany 2024, 93(11), 2767-2786. https://doi.org/10.32604/phyton.2024.055686
Received 04 July 2024; Accepted 09 October 2024; Issue published 30 November 2024
Abstract
West Sumatera is one of the largest mangosteen production centers in Indonesia. After the 2019 harvest season, there were variations in fruit production and quality which indicated symptoms of zinc (Zn) deficiency. This study evaluates the status of Zn for increasing the production and quality of mangosteen fruit Ratu Kamang cv. The study was conducting using the observation method in Lima Puluh Kota, West Sumatera from January to December 2020. This study used ten mangosteen trees aged 23 years in three locations selected purposively. Parameters observed included soil properties, leaf nutrients, number of fruits; fruit, rind, aril and seed weights, percentage of fruit scars (FS) and percentage of fruit contaminated with yellow sap (YSC). The relationship between Zn concentration in leaves and fruit production and quality was analyzed using Pearson’s correlation and regression models. The results showed that Zn played a role in increasing the production and quality of mangosteen fruit at certain concentration ranges in the leaves. Zinc concentrations of 23.45–30.00 mg/kg play a role in increasing production, while Zn concentrations of 26.29–30.29 mg/kg play a role in increasing quality. The lowest incidence of FS and YSC was found in trees with Zn concentration in the leaves between 30.00–32.00 mg/kg. Zinc concentration in the leaves of 30.00 mg/kg was the critical concentration in increasing the production and quality of mangosteen fruit under local cultivation conditions. Zinc has an antagonistic relationship to iron (Fe) and copper (Cu) in leaf tissue.Keywords
Mangosteen (Garcinia mangostana L.) is the queen of fruits, which is endemic in tropical countries including Malaysia, Thailand, Indonesia [1], India, Myanmar, the Philippines and Sri Lanka [2]. Apart from being consumed as fresh fruit, mangosteen also has high pharmacological potential. Mangosteen rind contains abundant poly-phenolic compounds such as anthocyanins, tannins, xanthones and phenolic acids as antioxidants [3]. Besides, the compounds contained in mangosteen rind also have pharmacological activities such as antimicrobial, antimalarial, anti-inflammatory, antidiabetic [4], antiviral, analgesic, antihistamine, anti-obesity, antidepressant, anti-fungal, antimutagenic, antitumor, anticancer and antiproliferative [5]. The results of many investigations showed that the crude extract of mangosteen rind was able to reduce cholesterol and malondialdehyde levels [6], suppress type II diabetes, stress, liver and kidney injury [7], and effectively suppress carcinogenesis with a pleiotropic mechanism [8].
The commodity is one of the potential export fruits from Indonesia. Its planting centers are spread in several areas, such as West Java, West Sumatera, North Sumatera, Riau, East Java, East Kalimantan, Central Kalimantan and Bali [9]. West Sumatra is the province that produces the second largest mangosteen after West Java, with 28,833 t in 2019 [10]. However, mangosteen production is still experiencing many problems both in quantity and quality [11]. Mangosteen productivity in Indonesia ranges from 30–70 kg/tree and is still below other mangosteen-producing countries >100 kg/tree. Meanwhile, the quality of mangosteen for export is very low because the yellow sap reaches 20% and the fruit spots are 25% so only 10% is exportable from the total production [12]. Generally, mangosteen production is carried out in compound gardens, agroforestry, and home yards that have not been managed with optimal cultivation techniques [13]. The low production and quality of mangosteen can be caused by deficiencies of macronutrients [14] and soil physical properties. However, excessive application of fertilizers containing N, P, K, and Ca has the potential to reduce the availability of several micronutrients, especially zinc (Zn) [15], which plays an important role in plant growth and fruit production [16].
Zinc is an important micronutrient in plant growth, development and defense [17,18]. Zinc plays a role in regulating many enzymes, photosynthesis, protein metabolism, carbohydrate biosynthesis [19], maintenance of cell membrane integrity, auxin synthesis, and pollen formation [20]. Zinc acts as a major factor in abiotic defense by inducing enzymes involved in chlorophyll synthesis, maintaining stability for membrane permeability and integrity, and reducing the production of reactive oxygen species (ROS) [21]. Zinc is a key factor in reducing the formation of ROS and defending cells against ROS attacks [22]. Zinc can suppress drought stress by improving plant water relations, cell membrane stability, osmolyte accumulation, stomatal regulation, and water absorption [19]. Zinc deficiency in plants causes a decrease in physical function, reduces production and productivity, and reduces product quality [23]. Zinc deficiency also causes reduced catkin length, inhibits flowering and reduces the development and number of fruits [24].
The largest mangosteen production center in West Sumatera is at Regency of Lima Puluh Kota [25]. The production of the commodity in the regency was 20,980.90 t in 2020, and the main production center is Bukik Barisan District [26]. Information gathered from local farmers that after the 2019 harvest season there was a variation in productivity and a decrease in fruit quality in several locations. Observations showed that there were several trees showing symptoms of shrunken leaves and short petiole segments, indicating Zn deficiency symptoms. The most visible symptoms of Zn deficiency were shortening of the internodes (rosette) and decreased leaf size (small leaves), while severe Zn deficiency caused high-contrast chlorosis (mothed leaves) and dead shoots (die-back) [3]. Farmers carry out plant nutrient management by providing organic fertilizer from poultry manure, NPK fertilizer and dolomite, which is applied once a year after harvest. Zinc deficiency in plants occurs in various soil types [23], especially when the soil pH is high, organic matter in the soil is high, in calcareous soils with high bicarbonate concentration, and in soils that are planted intensively [27]. Currently, there is not enough information available about the correlation of Zn deficiency to decreased production and quality of mangosteen fruit. The purpose of this study was to evaluate the status of Zn for increasing the productivity and quality of mangosteen fruit. The novelty of this research is finding out the critical concentration of Zn that contributes to increasing the production and quality of mangosteen fruit as a reference in developing a sustainable mangosteen plant nutrient management strategy.
The study was conducted in three locations, namely Nagari Banja Laweh 1 (NBL 1), Nagari Banja Laweh 2 (NBL 2) and Maek (Mk), Bukik Barisan District, Lima Puluh Kota Regency, West Sumatera, Indonesia, from January to December 2020. This district is located at 00°13′25″S, 100°13′56″E, and the three locations are at an altitude of 532.73, 546.12, 260 m above sea level, respectively. The topography of the land belongs to the slightly sloping category with a slope of 10%–15% and is dominated by Ultisol soil types. The average rainfall throughout 2020 in Lima Puluh Kota is classified as the wet season (average monthly rainfall >200 mm), the lowest rainfall occurs in August (133 mm) and the highest in April (454.5 mm) [28]. Farmers control plant nutrition levels by applying organic fertilizer from animal waste (30 kg/tree), NPK fertilizer (0.05-0.10 kg/tree), and dolomite (3 kg/tree) once a year after harvest. Farmers has never been applied Zn fertilization at the study area. Mangosteen is planted at various distances, including 6 m × 6 m, 8 m × 8 m, 8 m × 10 m, and 10 m × 10 m. The spacing adapts to the local topography. This planting distance is adjusted to the local topography. Mangosteen plants in the research area range in height from 13–20 m and have an average age of >20 years.
This research was conducted using the observation method. The determination of plant samples was purposive in each location. The 23-year-old mangosteen Ratu Kamang cv trees are used as the object of this study. The plant material utilized in this study is a public domain cultivar rather than a commercial variety. The most productive period for mangosteen fruit is when it is 20–30-year-old [29]. At each site, 10 sample trees with the same tree height (15 m), the same spacing (8 × 10 m), and grown at the same elevation were selected. The soil, leaf and fruit samples were taken from each tree.
Soil samples were taken compositely at a depth of 0–30 cm and 30–60 cm around the root zone with a radius of 3 m from the base of the stem of each sampled tree, respectively. The soil samples were then dried and ground into two sizes, namely 2 mm for soil physical analysis and <2 mm for soil chemical analysis (pH, organic C, total nitrogen (N), phosphor (P), kalium (K), Zn, Cu, Fe and manganese (Mn). Soil sample analysis was carried out following the instructions for soil, water, and plant analysis by Marles [30]. The pH was measured based on the potential of the comparison electrode (AgCl). Ash concentration was obtained by saponification at 550°C–600°C. Total N was analyzed by the Kjeldahl method. C-organic was oxidized with dichromate and measured by spectrometry. The determination of P, K, Zn, Cu, Fe and Mn were wet oxidized with HNO3 and HClO4. P was measured spectrophotometrically, K was measured by atomic absorption flamefotometer, while Zn, Cu, Fe and Mn were measured by atomic absorption spectrometer. The chemical compounds employed in the study were produced by Merck KGaA, located in Darmstadt, Germany.
The leaf samples used were fully developed terminal leaves. For each tree, 16 leaves were taken from the bottom, middle and top of the crown from four sides. Leaf samples were cleaned of adhering dust and dried in an oven at 65°C for 48 h. The dried leaf samples were then crushed and prepared for analysis of their nutrient concentration. The nutrients measured included Zn, Fe, Cu and Mn. Analysis of leaf samples was carried out following instructions for soil, water, and plant analysis by Marles [30].
Fruit samples were taken when the fruit was physiologically ripe with an index maturity level of 2, according to Palapol et al. [31]. The number of fruits is counted on each tree to determine the productivity per tree. A total of 100 fruit samples were taken from each tree to determine the average weight per fruit, rind weight, aril weight, seed weight, percentage of fruit scar (FS) and percentage of fruit contaminated with yellow sap (YSC). Fruit productivity per tree is calculated by multiplying the average fruit weight by the number of fruits per tree.
Data were analyzed using the IBM SPSS Statistics 22.0, IBM Corp., Armong, NY, USA. Statistical testing of the data obtained at a 5% level of significance was performed using analysis of variance (F-test). Pearson’s correlation was carried out between nutrient concentration in leaves and production parameters. Meanwhile, the relationship between Zn concentration in leaves and fruit production and quality parameters was analyzed using a regression model.
The soil texture at the three locations was included in the category of clay at NBL 1 and Mk, and sandy clay at NBL 2. The texture at the two depths 0–30 cm, and 30–60 cm is the same. Soil pH at the three locations was lower at the depth of 30–60 cm. The soil in NBL 2 locations was classified as very acidic, while at location NBL 1 and Mk, they were classified as acidic at a depth of 0–30 cm and very acidic at a depth of 30–60 cm. Soil C-organic concentration was also lower at a depth of 30–60 cm in the three locations. At a depth of 0–30 cm, it was classified as moderate at locations NBL 1 and NBL 2, and high at location Mk, while at a depth of 30–60 cm it was classified as low at the three locations. Likewise, the nutrients concentration was generally lower at a depth of 30–60 cm except for K, which is higher at location Mk and Fe at location NBL 2 and Mk. The macronutrient concentration in the two layers of soil at the three locations was included in the criteria of low–medium (N), very low–very high (P), and low (K). While the criteria for micronutrients are very low–low (Zn), high (Cu), and medium–very high (Fe and Mn). Soil properties at the three locations are presented in Table 1.
3.2 Productivity of Mangosteen Fruits and Increasing Percentage
The results showed that the mangosteen trees in NBL 1 produced the highest number fruit and were significantly different from the other two locations. The total number of fruits in NBL 1, NBL 2 and Mk were 880, 638 and 591, respectively. Fruit weight ranged from 111.8–120.6 g and did not differ significantly between locations. While fruit productivity ranges from 71.6–89.5 kg/tree. The highest productivity was obtained in NBL 1 of 89.5 kg/tree and the lowest in NBL 2 of 71.6 kg/tree. The difference in mangosteen fruit productivity between NBL1 and the other two locations ranged from 24.21%–24.97%. The number of fruits, average fruit weight, and fruit productivity at the three locations are presented in Fig. 1.
Figure 1: The number of fruits, fruit weight and fruits productivity of mangosteen trees in NBL 1, NBL 2 and Mk in Bukik Barisan, Lima Puluh Kota, West Sumatera, 2020. Numbers followed by the same letter were not significantly different from the F-test at the 5% level
3.3 The Correlation between Zn Concentration in Leaves with Mangosteen Fruit Productivity
The data presented in Table 2 shows the mean nutrient concentrations (Zn, Fe, Cu, and Mn) in the leaves of three different samples (NBL 1, NBL 2, and Mk), along with the standard deviations for each nutrient. Zinc concentration varies across the samples, with NBL 1 showing the highest concentration, followed closely by NBL 2 and Mk having the lowest. Fe concentration shows significant variation, with Mk having the highest concentration, significantly higher than both NBL 1 and NBL 2. Cu concentrations are quite similar across all samples, with no significant differences. Mn concentration shows variability among the nutrients, with Mk having the highest concentration, followed by NBL 2 and NBL 1 having the lowest.
The concentration of Zn and Cu in the leaves is positively correlated with the number and production of fruit, while Fe and Mn are negatively correlated. Conversely, Zn and Cu have a negative correlation with YSC and FS, while Fe and Mn have a positive correlation. Fe and Mn are positively correlated with all production parameters except quantity and fruit production. The correlation between the concentration of micronutrients in the leaves with production and quality parameters is presented in Table 3.
The results of the regression analysis revealed that a relative fruit production value of 50% was obtained if the Zn concentration was 23.45 mg/kg. If the Zn concentration in the leaf ranges from 23.45–30.00 mg/kg, then the relative fruit production is between 50%–75%. Relative fruit production of 100% is achieved if the Zn concentration in the leaves was 30 mg/kg. Meanwhile, the relative production of fruit decreased if the Zn concentration in the leaves was >30 mg/kg (Fig. 2).
Figure 2: Relationship of leaf Zn concentration with relative production and total fruits per tree of mangosteen fruits
The total number of fruits per tree increased to 932 with a Zn concentration in the leaves of 37.38 mg/kg from trees with a Zn concentration in the leaves of 21.53 mg/kg with a total of 389 fruits. When the Zn concentration in the leaves increased to 47.84 mg/kg, the total number of fruits decreased to 379 fruits (Fig. 2).
3.4 The Correlation between Zn Levels in Leaves and Mangosteen Fruit Quality
The weight components of the mangosteen fruit were the weight of the rind, arils and seeds. Fruit weight (flesh) has a positive correlation with rind and aril weight. The rind, aril and seed weight at the three locations ranged from 56.31–88.11 g, 18.90–45.01 g and 1.91–2.94 g, respectively (Fig. 3).
Figure 3: The correlation between fruit weight with rind, aril and seed weight of mangosteen fruit
The highest aril weight was 45.01 g at a Zn concentration of 30.29 mg/kg and the lowest aril weight was 18.90 g at a Zn concentration of 47.84 mg/kg. If the Zn concentration exceeds 30.29 mg/kg, the aril weight tends to decrease. The relationship between Zn concentration in leaves and fruit rind weight follows the same pattern as the correlation between Zn concentration in leaves and fruit production. The highest rind weight was 86.11 g with a Zn concentration of 26.29 mg/kg, while the lowest rind weight was 56.31 g with a Zn concentration of 47.84 mg/kg (Fig. 4).
Figure 4: The correlation between Zn concentration in leaves with rind weight and aril weight of mangosteen fruit
3.5 The Correlation between Zn Concentration in Leaves with the Incidence of YSC and FS
The percentage of FS reached 80.86% in trees with Zn concentration <30 mg/kg and decreased to around 36.66%–37.77% at Zn levels between 30–32 mg/kg. The percentage of FS increased again at Zn levels >32 mg/kg, reaching 58.02%. The same pattern was also found in YSC, at leaf Zn levels <30 mg/kg, the incidence of YSC ranged from 1.60%–4.64%, then decreased at Zn levels between 30–32 mg/kg and increased again up to 2.40% at Zn concentration >32 mg/kg. The correlation between Zn levels in leaves and the incidence of FS and YSC is presented in Fig. 5.
Figure 5: The correlation between Zn concentration in leaves and the incidence of FS and YSC in mangosteen fruit
3.6 The Correlation of Zn with Fe, Cu and Mn
The Zn concentration in the leaves was significantly inversely proportional to the Fe and Mn levels. The increase in Zn concentration was followed by a decrease in Fe and Mn levels. In contrast to the relationship between Zn and Cu, Zn levels between 20–30 mg/kg were followed by an increase in Cu levels, but Zn levels >30 mg/kg were followed by a decrease in Cu levels. The correlation between Zn and Fe, Cu and Mn levels is presented in Fig. 6.
Figure 6: The correlation of Zn with Fe, Cu and Mn
The proportion of sand and clay was almost similar between that in 0–30 cm depth with that at 30–60 cm depth, so that the soil in the research site was classified as sandy clay soil. The increase in clay concentration was associated with an increase in acidity with increasing soil depth. Negatively charged clay will bind to H+ cations, so an increase in clay concentration will increase H+ concentration and soil acidity. However, the high clay concentration at a depth of 30–60 cm was thought to inhibit nutrient translocation. P2O5 accumulation with fertilizers and organic matter decomposition were limited to the topsoil, so the P2O5 concentration was lower at a depth of 30–60 cm.
Soil texture is important for Zn adsorption, clay soil has a higher Zn adsorption capacity than sandy soil [33]. The high clay concentration in the subsoil causes low soil porosity which prevents the addition of C-organic, resulting in low C-organic concentration in the 30–60 cm soil layer. Accessible Zn increases with increasing organic matter in the soil so low organic matter concentrations in the soil cause Zn deficiency [20]. Plants obtain Zn in ionic form in the soil solution. Soil properties such as pH, cation exchange capacity (CEC), texture and soil organic matter (SOM) concentration have a major effect on Zn adsorption [34]. Increasing the level of SOM in the soil will increase the amount of organic Zn fraction in the soil, which ultimately changes the solubility of Zn. However, SOM is affected by pH, CEC and specific surface area, which varies between soils, thereby affecting the availability of Zn in the soil [35]. Zinc forms soluble complexes with chloride (Cl−), phosphate (ZnHPO4), nitrate (NO3−) and sulfate (SO42−) ions, but neutral sulfate (ZnSO4) and phosphate most importantly contribute to the total zinc concentration in solution [36]. The total concentration and activity of Zn in the soil solution increases with increasing Zn concentration in the soil and increasing soil acidity, whereas it decreases with increasing C-organic and clay particle concentration [37]. This phenomenon indicates that the Zn concentration in the soil at the three locations is in the very low-low category. Nandal et al. [38] state that the recommended concentration of Zn in the soil is between 0.6–1.2 mg/kg.
4.2 Productivity of Mangosteen Fruits
Soil properties play an important role in mangosteen productivity. Mangosteen plants grow well in soils that are moderately heavy to light, deep and moist but well-drained, slightly acidic and rich in organic matter [39] but can also survive in sandy loam or clay soils containing organic matter [40]. The total productivity of mangosteen fruit per tree in the three locations did not reach 100 kg/tree, but it was higher than the productivity of mangosteen fruit in Thailand, which was 55 kg/tree in the season and 30 kg/tree in the low season [41]. Weather conditions affect the productivity of mangosteen fruit, when the weather is favorable, mangosteen fruit production will increase. The flowering phase requires relatively dry conditions followed by rainfall for fruit formation and development. The wet season throughout the year in the Lima Puluh Kota is thought to influence the lack of flowering. Several types of fruit, such as mango and longan, require drying time to reduce vegetative growth and stimulate flowering [42]. Clay loam texture and nutrient concentration at NBL 1 location were more suitable for the growth and development of mangosteen plants so fruit productivity was higher than the other two locations.
4.3 The Correlation between Zn Concentration in Leaves with Mangosteen Fruit Productivity
The correlation between the Zn concentration in the leaves and the number of fruits and other production components showed that the adequacy of Zn was related to the number of fruits and productivity. Fruit, rind, aril and seed weights were negatively correlated with leaf Zn concentrations as indicated by a decrease in metrics with too high Zn concentration. This showed that Zn needed to increase the number of mangosteen fruit within certain concentration limits in the leaves.
Foliar spraying with 250 mL of ZnSO4 (0.5%) in 10 L of water fortnightly until deficiency symptoms disappear is the best dose per unit area on plants showing deficiency symptoms [43]. Spraying mango trees with 1 g/L nano-zinc before flowering can increase yield and fruit quality and increase resistance to malformations [44]. Foliar spraying with 0.6% ZnSO4 four months before harvest can reduce pre-harvest fruit de-cline, increase production and quality of kinnow fruit [45]. An increase in the number of fruits per bunch, the percentage of fruit formation, individual fruit weight, number of fruits per plant and fruit yield per plant was seen after Zn spraying on tomato plants [46]. Spraying 100 mg/kg ZnO-NPs can increase the quantity and quality of tomatoes in the greenhouse [47]. Spraying Zn or boron (B) or a combination of both effectively increased flowering and fruit set of mandarins [48]. Zinc application by foliar spraying supports the establishment of nutrient management techniques in fruit production [49].
The application of Zn to soil and leaves only fulfills 30%–40% of plant needs, while the rest is partially absorbed in colloidal clay and, becomes immobile and partly escapes into the environment due to soil and edaphic factors. ZnSO4 application of 0.2%–0.5% can withstand temporary deficiency if there is a severe Zn deficiency in the leaves [36]. Zinc in the soil is more relevant for affecting uptake by plants than ZnSO4, so it is necessary to pay attention to the dynamics of Zn in the soil so that it is effective in fertilizing management [50]. Makarenko et al. [51] stated that soils in Ukraine are characterized by a low level of transition of Zn from soil to crop production with an average transition coefficient of 0.10, which is thought to be due to the low Zn concentration. One of the effective efforts to regulate the mechanism of Zn inflow into the soil and increase its mobility is the fertilization system, especially Zn, which is contained in phosphorus fertilizers. Management of soil fertility with NPK minerals, organic fertilizers and Zn can increase the supply of Zn and promote the Zn cycle in cropping systems. However, geospatial variations in initial Zn concentration and Zn requirements per individual plant need to be considered together with a cost-benefit analysis of input costs [52]. Therefore, in areas with high adsorption capacity, high Zn fertilizer application is required, while soils with low absorption capacity require lower Zn fertilizer to minimize Zn accumulation and reduce production input. This model will help calculate the amount of potential Zn available in the soil and can be used to develop recommendations for Zn fertilization in stages in various soil conditions [15].
4.4 The Correlation between Zn Concentration in Leaves with Mangosteen Fruit Quality
Fruit quality is related to the Zn concentration in the leaves as indicated by the relationship between the Zn concentration in the leaves and the weight of the fruit arils and rind. The correlation of Zn concentration in leaves to fruit quality showed the same pattern as the correlation to relative fruit production. Zinc was needed to improve the quality of mangosteen fruit within certain concentration limits in the leaves, namely 26.29–30.29 mg/kg.
Some studies showed that Zn has a significant effect on fruit quality. Application of Zn fertilizer at the appropriate dose can significantly improve fruit quality [53]. Application of 0.6% ZnSO4 on leaves can increase fruit weight, percentage of sap, total dissolved solids, ascorbic acid and sugar concentration, total antioxidant (TAO) and total phenolic concentration (TPC) in mandarin kinnow fruit [43]. Foliar spraying with 200 mg/kg ZnSO4 was most effective in improving the quality of tomato fruit [54]. Zinc application significantly increased fruit size, rind thickness, fruit weight at harvest and after ripening, total soluble solids (TSS), flesh color, aroma and taste in mangoes [55]. Tahir et al. [56] stated that Zn was the main limiting factor for citrus production, and spraying Zn on the leaves significantly increased the growth, yield, production and quality of oranges.
Kumari et al. [57] stated that post-flowering Zn and B spraying played an important role in improving the quality of pineapples. The combined application of Zn and B can reduce mineral deficiencies in the leaves and more efficiently improve the quality of mangoes (taste, aroma, texture, aroma) than individual applications [58]. Applications of Zn and B to leaves have been shown to be more effective in increasing the production and quality of citrus fruits than applications to soil [59]. However, fruit quality is also strongly influenced by the supply of Zn and Mn in the soil. Plants treated with high concentrations of Zn and Mn can cause a reduction in total dissolved solids concentration in fruit, fruit diameter and chlorophyll and carotene concentration in leaves [60]. Likewise, unsuitable cultivation conditions such as poor drainage and mineral deficiencies, especially Zn and calcium (Ca), can affect fruit quality [61].
4.5 The Correlation between Zn Concentration in Leaves with the Incidence of FS and YSC
Fruit scars on the mangosteen fruit are shown by the fruit rind, which is silvery, pale, discolored to yellow or brown, scars that are elongated and hardened and scars that cover the entire surface of the fruit [62]. Fruit scar and YSC on the rind of the mangosteen fruit can be caused by damage to the rind due to insect bites or punctures and friction of the fruit rind during high winds. Weeks 3–10 after the fall of the calyx is a critical phase of the mangosteen fruit against thrips [63]. Most FS symptoms appeared in fruit aged 2 and 3 weeks after anthesis (WAA), and a thrips density of 10.6 individuals could cause scar symptoms in mangosteen fruits aged 2 WAA [64]. Kaur et al. [65] reported that the scars on kinnow mandarins were caused by thrips and mites. Fruit scars caused by thrips averaged 20% for all citrus species and citrus varieties in Tunisia [66]. Likewise, scars on guava fruit are caused by thrips and mites, but the damage only occurs in the epidermis of the pericarp of the fruit, which becomes brown and thickens [67]. Bagging, sanitation, neon yellow sticky traps, and a combination of sanitation and neon yellow sticky traps effectively reduced the intensity and percentage of scars on mangosteen fruit [68].
Yellow latex contamination occurs when the latex contaminates the surface of the fruit rind or arils due to the rupture of the yellow latex ducts. The rupture of the yellow sap duct is related to the presence of Ca in the fruit pericarp. Giving Ca from both dolomite (CaMg(CO3)2) and calcite (CaO) can reduce YSC in aril and mangosteen rind [69]. Treatment of calcium chloride (CaCl2) in the vegetative phase can be an effective way to reduce the incidence of YSC in mangosteen fruit [70]. The blooming phase is the best time for Ca application because the critical period for Ca uptake occurs between the first week and the fourth week after flowering. Application of Ca at anthesis and four WAA can reduce YSC in arils and fruit rind [71]. Application of 90 g of sub-micron dolomite per plant can increase Ca levels in mangosteen leaves and reduce YSC by up to 19.78% [72]. Treatment of Ca 4.8 kg/tree during flower blooming can increase the Capectate concentration in the pericarp, which causes a decrease in YSC in the segments, arils and rind of the mangosteen fruit [73]. Application of dolomite at doses of 2, 3, 4, 5, and 6 kg/tree can reduce YSC in arils, while at a dose of 5 kg/tree it can reduce YSC on mangosteen rind [74]. However, Zn negatively interacts with Ca because it competes at the same adsorption point on both the particles and the root surface [75], so Zn application is recommended through spraying on leaves.
The correlation of Zn concentration in leaves to the incidence of FS and YSC was the same as the correlation to the relative production and quality of mangosteen fruit. The incidence of FS and YSC was low in plants with Zn concentration in the leaves between 30.00–32.00 mg/kg. Several studies reported that Zn plays a role in protecting plants from attackers by direct Zn toxicity or by increasing Zn triggers for organic defense [76]. Zinc has the potential to be toxic to organisms at concentrations that exceed physiological limits and can affect insect reproduction [77]. Zinc can also reduce the symptoms of pests and diseases in plants [78]. The application of Zn to leaves can increase the total phenolic concentration, antioxidant activity and sugar concentration, especially when the application is carried out before flowering [79]. Plant phenols are a group of defensive compounds that play a major role in plant resistance to insects, microorganisms and plant competitors [80]. Changes in phenol levels are a strategy for plant defense against pest attacks [81]. Based on the range of Zn concentration in the leaves which affects the relative increase in production, quality and the incidence of FS and YSC, the Zn concentration in the leaves of 30.00 mg/kg was the critical concentration in increasing the production and quality of mangosteen fruit under local cultivation conditions. This information can be used as a reference in conducting regular monitoring in mangosteen development areas with the same agroecology as an effort to improve efficient and sustainable fertilization management.
4.6 The Correlation of Zn with Fe, Cu and Mn
The analysis showed that there was an antagonistic relationship between Zn, Fe and Cu in leaf tissue. If Zn is applied at high enough levels it will compete with other micronutrients, thereby limiting its uptake by plant roots which can lead to deficiency [82]. Excess Zn is often found in growing media that competes with P, Fe, Mn and Cu uptake and can cause Zn deficiency in plant tissues. High concentrations of Cu in the soil solution will reduce Zn availability to plants due to competition at the same site into plant roots. Zinc deficiency causes Fe deficiency, due to inhibition of transfer from the roots to the shoot system [83]. Martias et al. [84] state that there is a negative correlation between Mn in soil and leaves and mangosteen fruit quality, where the critical value of Mn in mangosteen leaves is around 425 mg/kg in the soil ranging from 22–24 mg/kg.
In pistachio, the concentration of Cu is strongly influenced by the application of Zn, as well as the concentrations of Fe and Mn. Zn application has a negative effect on Cu concentrations in plant tissues [85]. Copper concentrations in leaves and stems decreased significantly in Zn-treated plants compared to control plants, but Cu concentrations in roots increased with increasing Zn concentrations. The addition of 10 mg/kg Zn to the soil will reduce Cu concentrations in leaves and stems by 18%. Ramzan et al. [86] found that the application of Zn could reduce Cu concentrations due to its antagonistic effect on rice and wheat plants. Therefore, the application of a complete and balanced fertilizer containing all micronutrients is recommended.
The Zn concentration in the soil at the three locations was in the very low-low category. Mangosteen fruit productivity at NBL 1 location was higher than the other two locations because it has a clay texture and relatively more suitable nutrient concentration for the growth and development of mangosteen plants. Zinc levels play a role in increasing the production and quality of mangosteen fruit at a certain concentration range in the leaves. Zinc concentration in the leaves of 30.00 mg/kg is the critical concentration in increasing the production and quality of mangosteen fruit under local cultivation conditions. Zinc has an antagonistic relationship to Fe and Cu in leaf tissue, so to reduce antagonism between other micronutrients, it is recommended to use a balanced micronutrient fertilizer as needed. The suggested recommendation for mangosteen plants in Indonesia is the surface application using Zn fertilizers.
Acknowledgement: Thank you to our colleagues and reviewers who provided valuable suggestions for this publication.
Funding Statement: The research funded by Indonesian Agency for Agricultural Research and Development through the Indonesian Tropical Fruit Research Institute, West Sumatera Indonesia for this work through a research project entitled Technology and Innovation for Increasing Production of Horticultural Plants, grant number SP DIPA-018.09.2.412050/2020.
Author Contributions: The authors confirm contribution to the paper as follows: study conception and design: Martias, Dewi Fatria, Raden Heru Praptana, Antonius Kasno, Riska, Affandi, Ellina Mansyah; data collection: Martias, Dewi Fatria, Adha Fatmah Siregar, Raden Heru Praptana, Antonius Kasno, Riska, Tri Budiyanti, Sri Hadiati, Ellina Mansyah, Irwan Muas, Jumjunidang, Mizu Istianto, Djoko Mulyono, Triyani Dewi, Bambang Nuryanto; analysis and interpretation of results: Martias, Raden Heru Praptana, Antonius Kasno, Muhammad Prama Yufdy, Riska, Ni Luh Putu Indrayani, Affandi, Intan Gilang Cempaka, Agus Supriyo; draft manuscript preparation: Martias, Dewi Fatria, Adha Fatmah Siregar, Raden Heru Praptana, Antonius Kasno, Muhammad Prama Yufdy, Riska, Ni Luh Putu Indrayani, Affandi, Tri Budiyanti, Sri Hadiati, Ellina Mansyah, Irwan Muas, Jumjunidang, Mizu Istianto, Djoko Mulyono, Intan Gilang Cempaka, Triyani Dewi, Agus Supriyo, Bambang Nuryanto. All authors reviewed the results and approved the final version of the manuscript.
Availability of Data and Materials: The datasets used and/or analyzed during the current study are available from the corresponding author upon reasonable request.
Ethics Approval: Not applicable.
Conflicts of Interest: The authors declare that they have no conflicts of interest to report regarding the present study.
References
1. Aizat WM, Ahmad-Hashim FH, Jaafar SNS. Valorization of mangosteen, the queen of fruits, and new advances in postharvest and in food and engineering applications: a review. J Adv Res. 2019;20:61–70. doi:10.1016/j.jare.2019.05.005. [Google Scholar] [PubMed] [CrossRef]
2. Rizaldy D, Ramadhita NK, Nadhifa T, Fidrianny I. Mangosteen (Garcinia mangostana L.evaluation of in vitro antioxidant activities. Pharmacogn J. 2022;14:633–40. doi:10.5530/pj.2022.14.82. [Google Scholar] [CrossRef]
3. Viégas IJM, Cordeiro RAM, de Almeida GM, Silva DAS, da Silva BC, Okumura RS, et al. Growth and visual symptoms of nutrients deficiency in mangosteens (Garcinia mangostana L.). Am J Plant Sci. 2018;9:1014–28. doi:10.4236/ajps.2018.95078. [Google Scholar] [CrossRef]
4. Febrina D, Milanda T, Muchtaridi. Pharmacological activity Garcinia mangostana Linn: a review. Int J Curr Med Sci. 2018;8:430–3. doi:10.24327/ijcmes2018.08050047. [Google Scholar] [CrossRef]
5. Ansori ANM, Fadholly A, Hayaza S, Susilo RJK, Inayatillah B, Winarni D. A review on medicinal properties of mangosteen (Garcinia mangostana L.). Res J Pharm Technol. 2020;13:974–82. doi:10.5958/0974-360X.2020.00182.1. [Google Scholar] [CrossRef]
6. Husen SA, Winarni D, Khaleyla F, Kalqutny SH, Ansori ANM. Activity assay of mangosteen (Garcinia mangostana L.) pericarp extract for decreasing fasting blood cholesterol level and lipid peroxidation in type-2 diabetic mice. AIP Conf Proc. 2017;1888:020026. doi:10.1063/1.5004303. [Google Scholar] [CrossRef]
7. Elmund B, Hartrianti P. Evaluation of mangosteen (Garcinia mangostana) antioxidant activity in clinical trials and in vivo animal studies: a systematic review. J Appl Pharm Sci. 2020;10:114–29. doi:10.7324/JAPS.2020.101216.https://japsonline.com/abstract.php. [Google Scholar] [CrossRef]
8. Mahendra J, Sharma V, Mahendra L, Janani M, Hussain ST, Janarthan S, et al. Mangosteen (Garcinia mangostanacompositional profile and usage in cancer molecular docking studies. Oncol Radiot. 2021;15:42–7. Available from: https://www.oncologyradiotherapy.com/archive/oar-volume-15-issue-12-year-2021.html. [Accessed 2024]. [Google Scholar]
9. Mamondol MR. Investment feasibility and marketing of mangosteen commodity in Central Sulawesi, Indonesia. J Socioecon Dev. 2020;3(2):115–25. doi:10.31328/jsed.v3i2.1433. [Google Scholar] [CrossRef]
10. Yudha EP, Sari EY, Nugraha A. Analysis of indonesian mangosteen competitiveness in Thailand, Hong Kong and Malaysia. Agricore: Jurnal Agribisnis dan Sosial Ekonomi Pertanian Unpad. 2022;7:79–87. doi:10.24198/agricore.v7i1.40432. [Google Scholar] [CrossRef]
11. Uji T. Diversity, distribution, and potential of Garcinia species in Indonesia. Berk Penel Hayati. 2007;12:129–35. doi:10.23869/340. [Google Scholar] [CrossRef]
12. Purwandari H. Mangosteen (Garcinia mangostana L.) agribusiness development strategy in trenggalek regency, east java province. Magister Agribisnis. 2018;18:43–53. doi:10.32503/AGRIBISNIS.V18I2.982. [Google Scholar] [CrossRef]
13. Tanari Y, Jayanti KD. Application of technology to increase mangosteen (Garcinia mangostana L.) production in angele village, pamona pusalemba district, poso regency. Mosintuwu: Jurnal Pengabdian Kepada Masyarakat. 2021;1:32–5. Available from: https://ojs.unsimar.ac.id/index.php/mosintuwu/article/view/439. [Accessed 2024]. [Google Scholar]
14. Brunetto G, Wellington G, Melo BD, Toselli M, Quartieri M, Tagliavini M. The role of mineral nutrition on yields and fruit quality in grapevine, pear and apple. Rev Bras Frutic. 2015;37(4):1089–104. doi:10.1590/0100-2945-103/15. [Google Scholar] [CrossRef]
15. Desta MK, Broadley MR, McGrath SP, Hernandez-Allica J, Hassall KL, Gameda S, et al. Plant available zinc is influenced by landscape position in the Amhara Region. Ethiop Plants. 2021;10(2):254. doi:10.3390/plants10020254. [Google Scholar] [PubMed] [CrossRef]
16. Ojeda-Barrios D, Abadi’a J, Lombardini L, Abadi’a A, Va’zquez S. Zinc deficiency in field-grown pecan trees: changes in leaf nutrient concentrations and structure. J Sci Food Agric. 2012;92(8):1672–8. doi:10.1002/jsfa.5530. [Google Scholar] [PubMed] [CrossRef]
17. Cabot C, Martos S, Llugany M, Gallego B, Tolrà R, Poschenrider C. A role for zinc in plant defense against pathogens and herbivores. Front Plant Sci. 2019;10:1171. doi:10.3389/fpls.2019.01171. [Google Scholar] [PubMed] [CrossRef]
18. Hacisalihoglu G. Zinc (Znthe Last Nutrient in the alphabet and shedding light on Zn efficiency for the future of crop production under suboptimal Zn. Plants. 2020;9(11):1471. doi:10.3390/plants9111471. [Google Scholar] [PubMed] [CrossRef]
19. Hassan MU, Aamer M, Chattha MU, Haiying T, Shahzad B, Barbanti L, et al. The critical role of zinc in plants facing the drought stress. Agriculture. 2020;10(9):396. doi:10.3390/agriculture10090396. [Google Scholar] [CrossRef]
20. Babar H, Yusof MK, Saleem M. Role of zinc in plant nutrition—a review. Am J Exp Agric. 2013;3:374–91. doi:10.9734/AJEA/2013/2746. [Google Scholar] [CrossRef]
21. Ganguly R, Sarkar A, Dasgupta D, Acharya K, Keswani C, Popova V, et al. Unravelling the efficient applications of zinc and selenium for mitigation of abiotic stresses in plants. Agriculture. 2022;12(10):1551. doi:10.3390/agriculture12101551. [Google Scholar] [CrossRef]
22. Gonzalez JG, Ojeda-Barrios D, Hernandez-Rodriguez A, Abadia J, Sanchez E, Parra-Quezada R, et al. Zinc nutritional status of Pecan trees influences physiological and nutritional indicators, the metabolism of oxidative stress, and yield and fruit quality. Not Bot Horti Agrobot Cluj-Napoca. 2019;47:531–7. doi:10.15835/nbha47211389. [Google Scholar] [CrossRef]
23. Tayyiba L, Zafar H, Gondal AH, Farooq Q, Mukhtar MM, Hussain R, et al. Efficiency of zinc in plants, its deficiency and sensitivity for different crops. Curr Res Agric Sci. 2021;8(2):128–34. doi:10.18488/journal.68.2021.82.128.134. [Google Scholar] [CrossRef]
24. Walworth JL, White SA, Comeau MJ. Soil-applied Zn EDTA: vegetative growth, nut production, and nutrient acquisition of immature pecan trees grown in an alkaline, calcareous soil. HortScience. 2017;52(2):301–5. doi:10.21273/HORTSCI11467-16. [Google Scholar] [CrossRef]
25. Fadhilah M, Rochdiani D. Analysis of income farming of mangosteen in simpang sugiran village, guguak sub-district, limapuluh kota district. Jurnal Pemikiran Masyarakat Ilmiah Berwawasan Agribisnis. 2021;7:796–804. doi:10.25157/ma.v7i1.4790. [Google Scholar] [CrossRef]
26. Statistics of West Sumatera. West Sumatera Province in Figs. West Sumatera, Indonesia: Statistics of West Sumatera; 2021. p. 971. [Google Scholar]
27. Barman H, Das SK, Roy A. Zinc in soil environment for plant health and management strategy. Univers J Agric Res. 2018;6(5):149–54. doi:10.13189/ujar.2018.060501. [Google Scholar] [CrossRef]
28. Statistics of Lima Puluh Kota Regency. Bukik Barisan District in Figs. West Sumatera, Indonesia: Statistics of Lima Puluh Kota Regency; 2021. p. 93. [Google Scholar]
29. Jaluardi H. Sukabumi mangosteen center enters a productive period. 2012. Available from: https://regional.kompas.com/read/2012/01/25/23235731/~Regional~Jawa. [Accessed 2022]. [Google Scholar]
30. Marles RJ. Mineral nutrient composition of vegetables, fruits and grains: the context of reports of apparent historical declines. J Food Compost Anal. 2017;56(1S):93–103. doi:10.1016/j.jfca.2016.11.012. [Google Scholar] [CrossRef]
31. Palapol YS, Ketsa S, Stevenson D, Cooney JM, Allan AC, Ferguson IB. Colour development and quality of mangosteen (Garcinia mangostana L.) fruit during ripening and after harvest. Postharvest Biol Technol. 2009;51:349–53. doi:10.1016/j.postharvarbio.2008.08.003. [Google Scholar] [CrossRef]
32. Eviati, Sulaeman. Technical guide edition 2: chemical analysis of soil, plants, water and fertilizers. Bogor, West Java, Indonesia: Soil Research Institute; 2009. p. 234. Available from: https://kikp-pertanian.id/bpsitanahpupuk/opac/detail-opac?id=5. [Accessed 2024]. [Google Scholar]
33. Hippler FWR, Boaretto RM, Quaggio JA, Boaretto AE, Abreu-Junior CH, Mattos Jr D. Uptake and distribution of soil applied zinc by citrus trees-addressing fertilizer use efficiency with 68 Zn labeling. PLoS One. 2015;10(3):e0116903. doi:10.1371/journal.pone.0116903. [Google Scholar] [PubMed] [CrossRef]
34. Fan TT, Wang YJ, Li CB, H. JZ, Gao J, Zhou DM, et al. Effect of organic matter on sorption of Zn on soil: elucidation by Wien effect measurements and EXAFS spectroscopy. Environ Sci Tech. 2016;50(6):2931–7. doi:10.1021/acs.est.5b05281. [Google Scholar] [PubMed] [CrossRef]
35. McBride M, Sauve S, Hendershot W. Solubility control of Cu, Zn, Cd and Pb in contaminated soils. Eur J Soil Sci. 2005;48:337–46. doi:10.1111/j.1365-2389.1997.tb00554.x. [Google Scholar] [CrossRef]
36. Arunachalam P, Kannan P, Prabukumar G, Govindaraj M. Zinc deficiency in Indian soils with special focus to enrich zinc in peanut. Afr J Agric Res. 2013;8:6681–8. doi:10.5897/AJARx12.015. [Google Scholar] [CrossRef]
37. Rutkowska B, Szulc W, Bomze K, Gozdowski D, Spychaj-Fabisiak E. Soil factors affecting solubility and mobility of zinc in contaminated soils. Int J Environ Sci Technol. 2015;12(5):1687–94. doi:10.1007/s13762-014-0546-7. [Google Scholar] [CrossRef]
38. Nandal V, Solanki M. Status of Zinc in the soils of Faridabad District. India Eco Env Cons. 2021;27:286–9. Available from: https://www.envirobiotechjournals.com/issues/article_abstract.php?aid=11370&iid=329&jid=3. [Accessed 2024]. [Google Scholar]
39. International Centre for Underutilized Crops. Fruits for the future: mangosteen. Factsheet. 2003;8:1–2. Available from: https://www.doc-developpement-durable.org/file/Culture/Arbres-Fruitiers/FICHES_ARBRES/mangoustan/Factsheet-Garcinia-Mangosteen.pdf. [Accessed 2024]. [Google Scholar]
40. Grant A. What is mangosteen: how to grow mangosteen fruit trees. 2021. Available from: https://www.gardeningknowhow.com/edible/fruits/mangosteen/mangosteen-fruit-trees.htm. [Accessed 2022]. [Google Scholar]
41. Apiratikorn S, Sdoodee S, Lerslerwong L, Rongsawat S. The impact of climatic variability on phenological change, yield and fruit quality of mangosteen in Phatthalung Province, Southern Thailand. Kasetsart J (Nat Sci). 2012;46:1–9. Available from: https://li01.tci-thaijo.org/index.php/anres/article/view/242773. [Accessed 2024]. [Google Scholar]
42. Sdoodee S. The influence of global warming on phenological change of mangosteen (Garcinia mangostana L.) in Songkhla. In: The 33rd 90 Congress on Science & Technology, Thailand (STT33) “Science & Technology for Global Sustainability”, 2007 Oct 18–20; Nakhon Si Thammarat, Thailand: Walailak University. [Google Scholar]
43. Vadlamudi K, Upadhyay H, Singh A, Reddy M. Influence of Zinc application in plant growth: an overview. European J Mol Clin Med. 2020;7:2321–7. Available from: https://www.ejmcm.com/issue-content/influence-of-zinc-application-in-plant-growth-an-overview-10693. [Accessed 2024]. [Google Scholar]
44. Zagzog OA, Gad MM. Improving growth, flowering, fruiting and resistance of malformation of mango trees using Nano-Zinc. Middle East J Agric Res. 2017;6:673–81. Available from: https://curresweb.com/mejar/mejar/2017/673-681.pdf. [Accessed 2024]. [Google Scholar]
45. Liaquata M, Alib I, Ahmadc S, Malika AM, Ashrafc HMQ, Parveend N, et al. Efficiency of exogenous zinc sulfate application reduced fruit drop and improved antioxidant activity of ‘Kinnow’ mandarin fruit. Braz J Biol. 2023;83(5):e244593. doi:10.1590/1519-6984.244593. [Google Scholar] [PubMed] [CrossRef]
46. Ahmed R, Samad MYA, Uddin MK, Quddus MA, Hossain MAM. Recent trends in the foliar spraying of zinc nutrient and zinc oxide nanoparticles in tomato production. Agronomy. 2021;11(10):2074. doi:10.3390/agronomy11102074. [Google Scholar] [CrossRef]
47. Ahmed R, Uddin MK, Quddus MA, Samad MYA, Hossain MAM, Haque ANA. Impact of foliar application of zinc and zinc oxide nanoparticles on growth, yield, nutrient uptake and quality of tomato. Horticulturae. 2023;9(2):162. doi:10.3390/horticulturae9020162. [Google Scholar] [CrossRef]
48. Ruchala OK, Pandeya SR, Regmia R, Regmib R, Magrati BB. Effect of foliar application of micronutrient (zinc and boron) in flowering and fruit setting of mandarin (Citrus Reticulata Blanco) in Dailekh. Nepal Malaysian J Sustain Agric (MJSA). 2020;4(2):94–8. doi:10.26480/mjsa.02.2020.94.98. [Google Scholar] [CrossRef]
49. Boaretto R, Hippler FWR, Teixeira LAJ, Fornari RC, Quaggio JA, de Mattos-Jr D. Zinc fertilizers for Citrus production: assessing nutrient supply via fertigation or foliar application. Res Sq. 2022;1–31. doi:10.21203/rs.3.rs-2256772/v1. [Google Scholar] [CrossRef]
50. Recena R, García-López AM, Delgado A. Zinc uptake by plants as affected by fertilization with Zn sulfate, phosphorus availability, and soil properties. Agronomy. 2021;11(2):390. doi:10.3390/agronomy11020390. [Google Scholar] [CrossRef]
51. Makarenko N, Bondar V, Makarenko V, Symochko L. Zinc deficiency in soils of Ukraine: possible causes and regulatory mechanisms. Int J Ecosyst Ecol Sci. 2021;11(4):857–66. doi:10.31407/ijees11.424. [Google Scholar] [CrossRef]
52. Manzeke-Kangara MG, Joy EJM, Mtambanengwe F, Chopera P, Watts MJ, Broadley MR, et al. Good soil management can reduce dietary zinc defciency in Zimbabwe. CABI Agric Biosci. 2021;2(1):36. doi:10.1186/s43170-021-00057-4. [Google Scholar] [CrossRef]
53. Davarpanah S, Tehranifar A, Davarynejad G, Abadía J, Khorasani R. Effects of foliar applications of zinc and boron nanofertilizers on pomegranate (Punica granatum cv. Ardestani) fruit yield and quality. Scientia Hort. 2016;10:57–64. doi:10.1016/j.scienta.2016.07.003. [Google Scholar] [CrossRef]
54. Nagar V, Kumar S, Bagri UK, Bunker RR, Rana H. Assessment of effect of foliar spray of zinc sulphate on fruit quality of tomato under polyhouse. Int J Curr Microbiol App Sci. 2021;10(2):2376–83. doi:10.20546/ijcmas.2021.1002.282. [Google Scholar] [CrossRef]
55. Masroor HM, Anjum MA, Hussain S, Ejaz S, Ahmad S, Ercisli S, et al. Zinc ameliorates fruit yield and quality of mangoes cultivated in Calcareous soils. Erwerbs-Obstbau. 2016;58(1):1–7. doi:10.1007/s10341-015-0258-2. [Google Scholar] [CrossRef]
56. Tahir R, Adnan M, Bilal HM, Saeed MS, Tampubolon K, Rehman F, et al. Impact of foliar application of Zn on growth yield and quality production of citrus: a review. Ind J Pure App Biosci. 2020;8(6):529–34. doi:10.18782/2582-2845.8496. [Google Scholar] [CrossRef]
57. Kumari U, Deb P. Effect of foliar application of zinc and boron on quality of pineapple cv. Mauritius J Pharmacogn Phytochem. 2018;7:1166–8. Available from: https://www.phytojournal.com/archives/2018.v7.i6.6353/effect-of-foliar-application-of-zinc-and-boron-on-quality-of-pineapple-cv-mauritius. [Accessed 2024]. [Google Scholar]
58. Ahmad I, Bibi F, Ullah H, Munir TM. Mango Fruit yield and critical quality parameters respond to foliar and soil applications of zinc and boron. Plants. 2018;7(4):97. doi:10.3390/plants7040097. [Google Scholar] [PubMed] [CrossRef]
59. Noor Y, Shah Z, Tariq M. Effect of zinc and boron using different application methods on yield of citrus (sweet orange) in calcareous coils. Sarhad J Agric. 2019;35(4):1247–58. doi:10.17582/journal.sja/2019/35.4.1247.1258. [Google Scholar] [CrossRef]
60. Casierra-Posada F, Poveda J. Manganese and zinc toxicity reduce dry matter production, leaf pigment and fruit quality in strawberries (Fragaria sp. cv. Camarosa). Agronomía Colombiana. 2005;23:283–9. Available from: https://revistas.unal.edu.co/index.php/agrocol/article/view/19973. [Accessed 2024]. [Google Scholar]
61. Le Bellec F, Imbert E. Mangosteen: a delicacy in a fine case. In: Denis Loeillet, Eric Imbert, editors. Fruitrop. France: Cirad; 2013. p. 34–8. [Google Scholar]
62. Affandi, Octriana L, Fatria D, Purnama T. Quality improvement of mangosteen for export through drip irrigation system and yellow fluorescent sticky trap installation. AGRIVITA. 2011;33:2018–26. doi:10.17503/agrivita.v33i3.71. [Google Scholar] [CrossRef]
63. Affandi, Emilda D. Effect of mulching, sanitation and yellow fluorescent sticky trap application on population fluctuation and critical attack period of Thrip on mangosteen. AGRIVITA. 2010;3:277–84. doi:10.17503/agrivita.v32i3.25. [Google Scholar] [CrossRef]
64. Fardedi, Maryana N, Manuwoto S, Poerwanto R. Trips (Thysanoptera: thripidae) on mangosteen flowers and fruit and their relationship with ccar incidence. J Hort. 2012;22:119–28. doi:10.21082/jhort.v22n2.2012.p120-129. [Google Scholar] [CrossRef]
65. Kaur R, Bhullar MB, Sharma DR, Arora PK, Mahajan BVC. Rind scarring caused by biotic and abiotic factors influences kinnow fruit quality. Indian J Entomol. 2022;e21101. doi:10.55446/IJE.2022.495. [Google Scholar] [CrossRef]
66. Belaam-Kort I, Boulahia-Kheder S. Assessment of thrips damage in citrus orchards in Tunisia. Tunis J Plant Prot. 2019;14:55–68. Available from: http://www.tjpp.tn/SiteWeb/CurrentIssue/TJPP14_1/4ImenKort.pdf. [Accessed 2024]. [Google Scholar]
67. Musyarofah N, Susanto S, Aziz SA, Suketi K, Dadang. Fruit scar incidence and its effect on guava ‘Kristal’ fruit quality (Psidium guajava L.) at low- and middle-altitude orchards in Bogor. Indonesia J Trop Crop Sci. 2021;8(2):101–9. doi:10.29244/jtcs.8.02.101-109. [Google Scholar] [CrossRef]
68. Affandi, Emilda D, Jawal MAS. Application of fruit bagging, sanitation, and yellow sticky trap to control thrips on mangosteen. Indones J Agric Sci. 2008;9:19–23. doi:10.21082/ijas.v9n1.2008.19-23. [Google Scholar] [CrossRef]
69. Kurniadinata OF, Poerwanto R, Efendi D, Wachjar A. The effect of calcium and bio-pores absorption holes technology to reduce yellow sap contamination in mangosteen (Garcinia mangostana). J Hort. 2016a;26:59–66. doi:10.21082/jhort.v26n1.2016.p59-66. [Google Scholar] [CrossRef]
70. Dorly, Wulandari I, Tjitrosemito S, Poerwanto R, Efendi D. Study of calcium application to reduce gamboge on mangosteen fruits (Garcinia mangostana L.). J Agron Indones. 2011;39:49–55. doi:10.24831/jai.v39i1.13188. [Google Scholar] [CrossRef]
71. Kurniadinata OF, Depari SOS, Poerwanto R, Efendi D, Wachjar A. Solving yellow sap contamination problem in mangosteen (Garcinia mangostana) with Ca2+ application based on fruit growth stage. Commun Biom Crop Sci. 2016b;11:105–13. Available from: https://repository.unmul.ac.id/handle/123456789/3227. [Accessed 2024]. [Google Scholar]
72. Martias, Sparta A, Hendri, Fatria D, Marlina L, Mansyah E. The potency of sub-micron dolomite in reducing yellow latex contamination in mangosteen fruits. IOP Conf Series: Earth Environ Sci. 2021;648:012139. doi:10.1088/1755-1315/648/1/012139. [Google Scholar] [CrossRef]
73. Tanari Y, Efendi D, Poerwanto R, Sopandie D, Suketi K. Application of calcium to decrease yellow sap contamination at different positions of Gancinia mangostana L. Adv Hort Sci. 2018;32:169–75. doi:10.13128/ahs-22018. [Google Scholar] [CrossRef]
74. Yuniarti S, Lestari S, Hadiatry MC, Purba R. The calcium application to control yellow latex in mangosteen fruit (Garcinia mangostana). IOP Conf Series: Earth Environ Sci. 2021;807(4). doi:10.1088/1755-1315/807/4/042070. [Google Scholar] [CrossRef]
75. Prasad R, Shivay YS, Kumar D. Interactions of zinc with other nutrients in soils and plants—a review. Indian J Fertilisers. 2016;12:16–26. Available from: https://www.researchgate.net/publication/234062115. [Accessed 2024]. [Google Scholar]
76. Fones H, Preston GM. The impact of transition metals on bacterial plant disease. FEMS Microbiol Rev. 2013;37:495–519. doi:10.1111/1574-6976.12004. [Google Scholar] [PubMed] [CrossRef]
77. Shu Y, Gao Y, Sun H, Zou Z, Zhou Q, Zhang G. Effect of zinc exposure on the reproduction of Spodoptera litura Fabricius (Lepioptera: noctuidae). Ecotoxicol Environ Saf. 2009;72:2130–6. doi:10.1016/j.ecoenv.2009.06.004. [Google Scholar] [PubMed] [CrossRef]
78. Machado PP, Steiner F, Zuffo AM, Machado RA. Could the supply of boron and zinc improve resistance of potato to early blight? Potato Res. 2018;61:169–82. doi:10.1007/s11540-018-9365-4. [Google Scholar] [CrossRef]
79. Kürşat K, Kırlı A, Akgün M, Dede O. Effects of different levels of foliar zinc and application time on total phenolic content and antioxidant activity of potato. Fresenius Environ Bulletin. 2018;27:4192–7. Available from: https://www.researchgate.net/publication/327800846. [Accessed 2024]. [Google Scholar]
80. Belete T. Defense mechanisms of plants to insect pests: from morphological to biochemical approach. Trends Tech Sci Res. 2018;2(2):555584. doi:10.19080/TTSR.2018.02.555584. [Google Scholar] [CrossRef]
81. Pratyusha S. Phenolic compounds in the plant development and defense: an overview. plant stress physiology—perspectives in agriculture. IntechOpen. 2022. doi:10.5772/intechopen.102873. [Google Scholar] [CrossRef]
82. Bloodnick Ed. Role of zinc in plant culture. 2022. Available from: https://www.pthorticulture.com/en/training-center/role-of-zinc-in-plant-culture/. [Accessed 2023]. [Google Scholar]
83. Seeda MAA, El-Nour EAAA, Yassen AA, Mervat MG, Zaghloul SM. Interaction of copper, zinc, and their importance in plant physiology: review, acquisition and transport. Middle East J Appl Sci. 2020;10:407–34. doi:10.36632/mejas/2020.10.3.37. [Google Scholar] [CrossRef]
84. Martias, Hariyanto B, Purnama T, Nofiarli, Emilda D, Hendri, et al. Critical level of manganese in soil and leaves: it’s relationship to fruit quality of mangosteen (Garcinia mangostana L.). Ann Res Rev Biol. 2021;36:75–85. doi:10.9734/ARRB/2021/v36i930427. [Google Scholar] [CrossRef]
85. Shahriaripour R, Tajabadipour A. Zinc nutrition of pistachio: interaction of zinc with other trace elements. Commun Soil Sci Plant Anal. 2010;41:1885–8. doi:10.1080/00103624.2010.492445. [Google Scholar] [CrossRef]
86. Ramzan Y, Hafeez MB, Khan S. Biofortification with zinc and iron improves the grain quality and yield of wheat crop. Int J Plant Prod. 2020;14(3):501–10. doi:10.1007/s42106-020-00100-w. [Google Scholar] [CrossRef]
Cite This Article
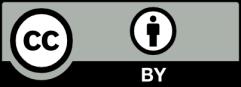
This work is licensed under a Creative Commons Attribution 4.0 International License , which permits unrestricted use, distribution, and reproduction in any medium, provided the original work is properly cited.