Open Access
ARTICLE
An Efficient Plant Regeneration System for Different Explants of Rare and Endangered Plants in Mussaenda anomala
1 School of Life Science, Guizhou Normal University, Guiyang, 550025, China
2 Qiandongnan Forestry Scientific Research Institute, Kaili, 556000, China
* Corresponding Author: Yingliang Liu. Email:
# These authors contributed equally to this study
(This article belongs to the Special Issue: Microbial Biotechnology Applications on Plant Performance and Physiology for Sustainable Production )
Phyton-International Journal of Experimental Botany 2024, 93(10), 2597-2611. https://doi.org/10.32604/phyton.2024.056875
Received 01 August 2024; Accepted 18 September 2024; Issue published 30 October 2024
Abstract
To establish an efficient regeneration method for the rare and endangered plant Mussaenda anomala to address problems regarding its reproductive obstacles and scarce populations. In this study, the terminal buds, axillary buds, stem segments with two axillary buds, stem segments with two axillary buds and one terminal bud, and leaves of M. anomala were used as explants. The effects of different explants and disinfection methods, plant growth regulators and substrates on plant regeneration were explored. The following results were obtained: (1) The terminal bud was a suitable explant for M. anomala tissue culture, and the disinfection method utilized was treatment with 0.2% HgCl2 for 8 min. (2) Initiate medium: MS basic medium supplemented with 0.5 mg/L 6-BA and 0.2 mg/L IBA for the high germination rate (100%) and the maximum bud height (1.70 cm) of the terminal bud. (3) Proliferation medium: MS basic medium supplemented with 3.0 mg/L 6-BA and 0.2 mg/L IBA for a high proliferation rate (96%) and proliferation time (6.0) of terminal buds. (4) Proliferation medium supplemented with 0.7 mg/L GA3 significantly increased the bud heights of multiple buds. (5) Rooting medium: MS basic medium supplemented with 0.5 mg/L IBA and 0.5 mg/L IAA for a high rooting rate (88%), root number (12.0) and root length (5.07 cm). (6) The optimal substrate for seedling acclimation and transplanting was perlite: vermiculite (1:1), which resulted in the highest survival rate (97%) and plant height (5.89 cm), as well as better growth potential for seedlings. The surfaces of M. anomala explants are densely covered with trichome, which increased the difficulty of disinfection; the plant growth regulators directly affected the growth and development in the regeneration process of M. anomala, and the substrate significantly affected the survival rate and height growth for seedling acclimation.Keywords
Supplementary Material
Supplementary Material FileMussaenda anomala, an evergreen trailing shrub of the family Rubiaceae and genus Mussaenda, is only distributed in the Dayao Mountains of Guangxi Province and Southeastern Guizhou Province in China and is a rare and endangered plant classified for national I key protection [1]. The population is extremely small in number and has a narrow distribution. There are 5 sepals on the inflorescence, which grow to resemble leaves in shape. Its flowers are clustered at the tops of the branches, and the flower colour and shape are extremely beautiful, with high ornamental value and broad horticultural prospects [1]. Mussaenda pubescens, a member of the same genus, contains some medicinal ingredients, such as triterpenoid saponins, cycloartane saponin and flavonoids, which have the effects of relieving heat, cooling the blood and detoxifying toxins and have been utilized in a variety of medicinal agents [2,3]. However, the study of the medicinal ingredients of M. anomala is still lacking, mainly due to the scarcity of its population, which has hindered further exploration. Its elongated calyx tube with densely covered with trichome and a short, concealed pistil hinder pollination, and the seeds are difficult to obtain in the wild. M. anomala is a minimal population plant in Guizhou Province and is an important feature of the phytogeography in this region, an irreplaceable basal source of the regional flora and its biodiversity components, and a germplasm resource with extensive use and ecological value. Therefore, there is an urgent need to conserve, rescue and propagate M. anomala at the present time. A previous study obtained a small number of regenerated plants through cuttings [4]. However, the tissue culture technique has the advantages of using less material, causing less damage to the mother plant, and featuring a high reproduction coefficient and a shorter cultivation cycle for M. anomala propagation, with its scarcity of germplasm resources. However, the establishment of the system is influenced by both internal and external factors.
The surfaces of young stem segments and leaves of M. anomala are densely covered with elongated trichomes, which increase the disinfection difficulty, making it challenging to choose a disinfectant type and concentration. Among them, alcohol, NaClO and HgCl2 are widely used in disinfection processes for plant tissue culture explants; however, alcohol and NaClO are more damaging to young explants [5]. In addition, cytokinin and auxin are crucial for the dedifferentiation and redifferentiation of explants [6,7]. Gibberellin promoted internode elongation and the high growth of plant buds [8] and had a significant effect on bud height growth in tissue cultures of Zenia insignis [9], Camellia sinensis [10], Pyrus spp. [11] and Dendrobium officinale [12]. Zhang et al. [13] initially investigated a rapid propagation system using young stem segments as explants for M. anomala, but the proliferation time was only 2; thus, attempts to establish large-scale and industrialized applications in gardening and forestry have not been successful. To date, there have also been no reports on the establishment of a mature propagation and breeding system for M. anomala.
Therefore, on the basis of previous studies, this paper evaluated the effects of different explant types, disinfection methods, and plant growth regulators on plant regeneration of M. anomala and screened for the optimal substrate for seedling acclimation, aiming to provide technical support for the establishment of an efficient and stable regeneration system for M. anomala. The results are of great significance for the promotion of M. anomala tissue culture technology, propagation, germplasm conservation, potential value development, and subsequent basic research.
The plant materials were taken from the nursery of Qiandongnan Institute of Forestry in May 2022, and one-year-old well-grown, disease-free and pest-free branches of M. anomala were collected and brought back to the laboratory for pretreatment at Guizhou Normal University in Guiyang, Guizhou Province, China (longitude 106°38′15″, latitude 26°23′19″, altitude 1139 m), which is a subtropical plateau monsoon humid climate zone with an annual average temperature of 15.8°C and precipitation of 1100 mm.
After pruning the branches, they were transferred to a container filled with 0.01% Tween-80 (Tianjin Kemiou Chemical Reagent Co., Ltd., Tianjin, China), and the stem segments and leaves were brushed with a brush and rinsed under tap water for 2 h. Five kinds of explants (Fig. 1), including terminal buds (a), axillary buds (b), stem segments with two axillary buds (c), stem segments with two axillary buds and one terminal bud (d), and 1 cm ×1 cm leaves (e), were sterilized with 0.1%–0.2% HgCl2 containing 0.01% Tween-80 for 6, 8, and 10 min, respectively, followed by five rinses in sterile distilled water, and inoculated into MS medium without plant growth regulators and then placed in an incubator at 25 ± 2°C with a light intensity of 1200 lux for 16 h/d. The pH value of the medium was adjusted to 5.90 ± 0.1 before sterilization (121°C, 20 min). Thirty explants were inoculated in each treatment, and each experiment was replicated three times. After 30 days of inoculation, the pollution rate, survival rate and growth were counted (the same below). The leaf calluses were stained with haematoxylin and observed and photographed under a DM-3000 microscope (Leica, Germany).
Figure 1: Different explant types of M. anomala
Notes: a. Terminal bud; b. Axillary buds; c. Stem segment with two axillary buds; d. Stem segment with two axillary buds and one terminal bud; e. Young leaf. Bars = 1.0 cm.
2.3 Initiate Culture in Terminal Buds
The terminal buds of young stem segments of M. anomala were used as explants and inoculated into MS medium containing 30 g/L sucrose, 6 g/L agar, and 0.2 g/L PVP (Chengdu Jinshan Chemical Reagent Co., Ltd., Chengdu, China). The effects of 6-BA, KT (0.5, 1.0, 2.0, and 4.0 mg/L), or TDZ (0.1, 0.3, 0.5, 0.7 and 1.0 mg/L) combined with IBA or NAA (0.05, 0.1, 0.2 and 0.4 mg/L) (Shanghai aladdin Biochemical Technology Co., Ltd., Shanghai, China) on the growth and germination of the terminal buds were investigated. Each treatment was inoculated with 30 explants, and the experiment was repeated three times, with plants incubated at 25 ± 2°C, 1200 lux, 16 h/d for 30 d. The growth and germination of terminal buds were recorded.
2.4 Proliferation Culture in Terminal Buds
The germinated terminal buds as explants were inoculated into MS basic medium supplemented with different PGRs: 6-BA, KT or TDZ (1.0, 2.0, 3.0, and 4.0 mg/L) in combination with IBA (0.05, 0.1, 0.2 and 0.4 mg/L) for proliferation induction. Each treatment was inoculated with 30 explants, the experiment was repeated three times, and the growth and germination of terminal buds were recorded at 25 ± 2°C, 1200 lux, and 14 h/d for 45.
2.5 Effect of GA3 on the Height Growth of Multiple Buds
After terminal bud proliferation, the height growth rate was slow, which was not conducive to further rooting induction. Multiple buds from the experiments described above were cut into single buds and inoculated into MS basic medium supplemented with 30 g/L sucrose, 6 g/L agar, 0.2 g/L PVP, 3.0 mg/L 6-BA and 0.2 mg/L IBA. The effects of different concentrations of GA3 (0.0, 0.5, 0.7, and 1.0 mg/L) on bud proliferation and height growth were investigated (GA3 was added to the medium by the filtration sterilization method). Each treatment was inoculated with 30 explants, and the experiment was repeated three times. Bud proliferation and height growth were recorded at 25 ± 2°C, 1200 lux, and 14 h/d for 45 d.
Multiple buds with strong growth and more than 2 cm in height were divided into single buds and inoculated into 1/2 MS basic medium supplemented with 30 g/L sucrose, 6 g/L agar, 0.2 g/L PVP, and medium supplemented with different PGRs: IBA (0.0, 0.1, 0.5, 1.0 and 1.5 mg/L) in combination with IAA (0.0, 0.1, 0.5, 1.0 and 1.5 mg/L) for rooting induction. 30 explants were inoculated in each treatment, the experiment was repeated three times, and the rooting rate and growth of the buds were recorded in the incubation at 25 ± 2°C, 1200 lux, 14 h/d for 30 d. The root indicators of the plantlets were scanned using a root scanner (SCAN-GXY-A, Beijing, China).
The plantlets were acclimatized for adaptation to the external environment by opening the bottle caps in the culture room for 7 d, the medium on the roots was cleaned off, and the plantlets were subsequently transplanted into different substrates (s1: vermiculite; s2: perlite; s3: vermiculite: perlite (1:1, v/v); s4: vermiculite: perlite: peat (1:1:1, v/v/v)), and there were sterilised with 800X solution of carbendazim for 30 min. 30 plantlets were transplanted for each treatment, and the experiment was repeated three times. The plants were placed in a greenhouse for insulation and moisturizing management at 18°C–35°C and 55%–80% air humidity. The survival rate and growth of the plants were observed and counted after transplanting for 60 days.
2.8 Statistics and Analysis of Data
Experimental data were processed using SPSS 25.0 software to determine significant differences by Tukey’s test (p ≤ 0.05). Graphs were created using the software Origin 19.0 and Photoshop 2018.
Contamination Rate (%) = (Number of contaminated explants/Number of inoculations) × 100%
Proliferation Rate (%) = (Number of proliferative colonies/Number of inoculations) × 100%
Proliferation Times = (Number of proliferative colonies – Number of inoculations)/Number of inoculations.
3.1 Explants and Sterilization Methods
The surfaces of the five types of explants in M. anomala were densely covered with trichomes, which made the sterilization process extremely difficult (Fig. 1). There was a significant difference (p < 0.05) in the contamination and mortality rates of different explants according to the disinfectants (Table 1); the contamination and mortality rates of I and II explants were low, V explants were higher, III and IV explants had a contamination rate of more than 80%; and the mortality rate of II explants was significantly higher than those of I, III, IV and V (11.50, 14.43, 7.76 and 3.02 times, respectively). Therefore, the terminal buds were suitable explants for M. anomala tissue culture. In addition, the contamination rate of the explants showed a decreasing trend with increasing HgCl2 concentration and time, while the mortality rate showed an increasing trend. Therefore, it was determined that the appropriate disinfection method was to treat the terminal buds with 0.2% HgCl2 for 8 min and the leaves with 0.1% HgCl2 for 10 min (Table S1). The calluses were induced by the leaves, which were yellow–white, loosely arranged, and had prominent nodular structures on their surfaces (Fig. 2). Staining of the calluses revealed deep obvious nuclei and a tight intercellular arrangement by haematoxylin staining, exhibiting embryonic callus characteristics (Fig. 2). However, the calluses failed to yield regenerated plants after a large number of differentiation experiments.
Figure 2: Cultivation processes of M. anomala explants
Notes: a. Inflorescence in M. anomala; b. Fungal contamination of stem segments with two axillary buds and one terminal bud; c. Uncontaminated stem segment with two axillary buds and one terminal bud; d. A nodular callus induced from a leaf; e. Microscopic observation of embryonic calluses. Bars = 1.0 cm.
3.2 Effects of Different PGRs on Terminal Bud Initiate Induction in M. anomala
Treatments of 6-BA with NAA, KT with IBA, and TDZ with IBA resulted in relatively poor growth on terminal buds (Table S2), especially medium supplemented with NAA at different concentrations. A large number of calluses were induced at the base of the terminal bud, though terminal bud growth deteriorated with the cultivation process (Fig. 3b,c). There were no significant differences between 6-BA and IBA in terms of the terminal bud germination rate and leaf length (p > 0.05), though there was a significant difference in bud height in 16 treatments (p < 0.05) (Table 2). Groups a3 and a5 had the highest bud heights, and a3 had a longer leaf length, showing strong growth potential and green leaves (Fig. 3a). In medium supplemented with a high concentration of 6-BA, the terminal bud leaves were yellowish white and curled easily. Therefore, the medium supplemented with 0.5 mg/L 6-BA and 0.2 mg/L IBA (a3) was suitable for initiate culture in M. anomala terminal buds.
Figure 3: Initiate culture and proliferation induction of M. anomala terminal buds
Notes: a. Terminal bud growth in a 3 medium for 15 d; b. Terminal bud cultured in MS medium supplemented with 1.0 mg/L 6-BA and 0.05 mg/L NAA for 15 d, which induced a small number of calluses at the base; c. Terminal bud cultured in MS medium supplemented with 1.0 mg/L 6-BA and 0.2 mg/L NAA for 15 d, which induced a large number of calluses at the base and petiole; d. Growth of germinating terminal buds in proliferation medium (b11) for 45 d. Bars = 1.0 cm.
3.3 Effects of the Combination of 6-BA and IBA on the Proliferation Induction of Terminal Buds
There were significant differences between 6-BA and IBA on the proliferation rate and proliferation times of terminal buds in 16 treatments (p < 0.05). The proliferation rates and proliferation times of b1–b4 and b5–b8 and the proliferation rates of b9–b12 first increased and then decreased with increasing IBA concentration, while the proliferation rates of b9–b12 and the proliferation rate and proliferation times of b13–b16 gradually increased with increasing IBA concentration (Table 3). The proliferation rate was the highest in b12, followed by b11 and b10, which were higher than 90%; the proliferation times were the highest in b10 (6.62), followed by b11 (6.0), which were significantly higher than those of b1–b8 and b12–b16, and the lowest proliferation time was in b8 (1.50). Moreover, the proliferation effects of terminal buds were extremely good in b9–b12 when the 6-BA concentration was 3.0 mg/L, the leaves were green, and the buds were vigorous (Fig. 3d). The growth of terminal buds was poor when the 6-BA concentration was 4.0 mg/L. Therefore, MS medium supplemented with 3.0 mg/L 6-BA and 0.2 mg/L IBA (b11) was suitable for the proliferation induction of M. anomala terminal buds.
3.4 Effects of GA3 on the Proliferation and Height Growth of Terminal Buds
When terminal buds proliferate, their height growth is slow, and they need to stay in the strong seedling stage for a longer time, thus delaying the rooting culture process and prolonging the culture time. Compared with the control (b11), medium supplemented with GA3 could significantly increase the bud height, but there was no significant difference in the proliferation rate (Fig. 4); the bud height first increased and then decreased with increasing GA3 concentration and was highest in b11-2, which was 1.54 times that of b11 (Fig. 5). Therefore, medium supplemented with 3.0 mg/L 6-BA, 0.2 mg/L IBA, and 0.7 mg/L GA3 was suitable for the height growth induction of M. anomala terminal buds.
Figure 4: Effects of different concentrations of GA3 on the height growth of terminal buds
Figure 5: Effects of different concentrations of GA3 on the high growth of terminal buds during proliferation. Bars = 2.0 cm
3.5 Rooting Induction in Proliferating Seedlings
The rooting rate, root number and root length were significantly different (p < 0.05) in the 25 treatments (Table 4). Compared with the control (c0), the rooting rate and root number of c1–c24 were higher than those of c0. The rooting rate was the highest in c8, followed by c12 and c9 (>80%), which were 71.91%, 71.35%, and 66.35% higher than that of c0, respectively. The root number was the highest at c12, followed by c7, c11, and c20 (>11), which were 5.15, 5.04, 4.94, and 4.86 times higher than that of c0, respectively. In contrast, the root length was the highest in c0 and significantly higher than those in c1–c24, while c12, c10, c6, and c13 had longer root lengths, and the root lengths of c15–c24 were shorter at relatively high concentrations of auxin. These results indicated that medium supplemented with IBA and IAA promoted the rooting rate and root number in multiple buds, and high auxin concentrations were unfavourable to root elongation. It is worth noting that the adventitious roots extended out of the medium against root geotropism and grew upwards with prolonged culture time during rooting induction (Fig. 6). A comprehensive analysis revealed that medium supplemented with 0.5 mg/L IBA and 0.5 mg/L IAA (c12) was suitable for rooting induction of M. anomala multiple buds.
Figure 6: Rooting induction processes of M. anomala multiple buds
Notes: a. Multiple buds were incubated in c12 for 13 d; b. Multiple buds were incubated in c12 for 21 d; c. Multiple buds were incubated in c12 for 48 d; d. Root system analysis of regenerated plants. Bars = 1.0 cm.
There were significant differences in the survival rates and plant heights of the seedlings in the 4 types of transplantation substrates (p < 0.05) (Table 5). Among them, the survival rate and plant height were the highest in s3, and the survival rate of s3 was significantly higher than those of s1 and s4, while the plant height of s3 was significantly higher than those of s1, s3 and s4, and s3 and s4 seedlings had better growth potentials and green leaves (Fig. 7). Therefore, it can be concluded that perlite: vermiculite (1:1, v/v) was a suitable mixed substrate for seedling acclimatization and transplanting.
Figure 7: Acclimatization and transplantation process of M. anomala seedlings
Notes: a. The seedlings were transplanted into perlite: vermiculite (1:1, v/v) for 1 d; b. The seedlings were transplanted into perlite: vermiculite (1:1, v/v) for 32 d; c. The seedlings were repotted 45 d after transplantation; d. The 60th day after the seedlings were repotted. Bars = 2.5 cm.
4.1 Explants and Sterilization
The types and disinfection methods of explants are the main factors influencing the success of plant tissue culture technology and are of great significance for the establishment of a tissue culture system to select disinfection methods for difficult explants [14,15]. The surfaces of stem segments, leaves and buds for M. anomala are densely covered with trichomes, and a large number of small air bubbles adhere to the explant surface when they are sterilized in disinfectant solutions, which makes it extremely difficult to sterilize the explants due to the small surface tension of these bubbles, as the disinfectant solutions cannot saturate the area. To solve this problem, the disinfectant solutions contained different concentrations of antifoaming agent for explant disinfection; however, the solutions failed to reduce the contamination rate, and further mixing the explants and the disinfectant solution in different intensities of ultrasonic treatment resulted in the death of most of the explants. It was found that tender explants were successfully disinfected with 75% alcohol or 2% NaClO, though the surfaces of the explants gradually turned brown and deepened after inoculation for 2 h, and the mortality rate was higher. The disinfection effect of HgCl2 alone was better, and the terminal buds were disinfected with 0.2% HgCl2 for 8 min, showing a higher germination rate and lower contamination. Similar results were found in Mussaenda erythrophylla [16], Juglans regia [17] and Platycladus orientalis [18], while the treatment of leaves with 0.1% HgCl2 for 10 min was a suitable disinfection method, but the embryonic calluses induced by the leaves failed to differentiate in differentiation medium, a finding that should be further investigated. However, Das et al. [19] verified somatic embryogenesis and development into whole plants, in callus originating from internodes of M. erythrophylla.
4.2 Effects of Different PGRs on Initial Culture
In the initial culture stage, cytokinin and auxin play important roles in explant growth and development [6,7]. In this study, we found that a large number of calluses could be induced by terminal bud inoculation in medium supplemented with NAA, indicating that the callus induction of M. anomala is extremely sensitive to NAA. In fact, NAA has been proven to be effective in the induction of callus formation in a variety of plants, such as Eucalyptus robusta [20], Moringa oleifera [21], Paeonia suffruticosa [22], Paeonia lactiflora [23] and Asparagus officinalis [24], because the application of exogenous PGRs alters the levels of other endogenous hormones and influences plant developmental processes [25]. Fehér [26] reported that NAA has a dual role in the initiation of calluses and somatic embryos, one related to auxin signal transduction and the other related to the stress response, which can alter the endogenous auxin content. However, the addition of NAA to the culture medium reduced the number of shoots [27]. The germinated terminal buds had poor growth, and the leaves curled significantly with continued culture. In contrast, the combination of 6-BA and IBA had a better effect on top bud germination than the other combinations. One possible mechanism for these results is the regulatory input of the auxin precursor indole-3-butyrate (IBA) into the active auxin IAA library, which regulates plant cell division and cell proliferation. One of the mechanisms may involve the regulated input of the auxin precursor indole-3-butyric acid (IBA) to the active auxin IAA pool, which regulates plant cell division and proliferation [28].
4.3 Effects of Different PGRs on Adventitious Buds Proliferation
In the proliferation stage, 6-BA promoted the differentiation of adventitious buds, and the terminal bud proliferation rates and times increased continuously with increasing cytokinin concentration and were highest in medium supplemented with 3.0 mg/L 6-BA and 0.2 mg/L IBA. Aygun et al. [29] reported that the bud proliferation rates of Pyrus ussuriensis were the highest (>80%) in medium supplemented with 9.0 µM BA and 0.5 µM IBA. Similarly, the buds of Garcinia mangostana formed a large number of multiple buds on MS medium supplemented with 5.0 µM 6-BAP and 1.0 µM IBA [30]. Maximum shoot proliferation rates were achieved on medium containing 1.5–2.0 mg/L BA, 50 mg/L ADS, and 0.25 mg/L IAA in the culture of Mussaenda [31]. However, the height growth of multiple buds was slow during M. anomala proliferation, which was not conducive to rooting induction and hindered the rapid propagation process. Gibberellins, which were discovered in the 1930s, promote cell proliferation and division and are important PGRs in the process of plant growth and development. They are synthesized via the terpene route from geranylgeranyl diphosphate and feature a basic structure formed by an ent-gibberellane tetracyclic skeleton. To date, more than 130 types of gibberellins have been identified [32]; among them, only four have biological activity (GA1, GA3, GA4, and GA7), and GA3 acts as a natural PGR, regulating stem elongation, seed germination, and increased fruit size [33]. In this study, the heights of multiple buds first increased and then decreased with increasing GA3 concentration and were highest at 0.7 mg/L GA3 (>3.5 cm). Similarly, the bud elongation rate of Glycine max was affected by different GA3 concentrations, and bud elongation rates of 34% and 26% were achieved when using the combination of 1.0 mg/L GA3 and 0.1 mg/L IAA for Jack Purple and Tianlong 1, respectively [34]. Regenerated buds of Zenia insignis elongated successfully in medium containing 1.20 μM GA3 [9]. This is related to the fact that GA3 induces the upregulated expression of the NAC and MYB transcription factors CESA, PAL, and GA oxidase, which are related to secondary wall biosynthesis [8], and increases the activity of cell wall-transforming enzymes [35], which trigger plant lignification and stem elongation. In contrast, GA3 negatively affected the number and length of buds and generally caused the generation of red leaves in Pyrus boissieriana. [11], which may be related to the differences in sensitivity to GA3 in different tree species.
4.4 Effects of Different PGRs on Adventitious Buds Rooting
In the rooting stage, the addition of exogenous auxin can effectively promote the induction of adventitious rooting and improve the rooting quality of seedlings [36]. However, there are significant differences in the types and concentrations of auxin for rooting induction in different plants. In this study, it was found that the combination of 0.5 mg/L IBA and 0.5 mg/L IAA had a better effect on inducing adventitious rooting than IBA or IAA alone and was a suitable medium for adventitious rooting induction in M. anomala. Similar findings have been reported in Monochasma savatieri [37]. A possible mechanism explaining this effect is that exogenous auxin affects the endogenous auxin content [38], while IAA is a predominant form of active auxin in plants. In addition to de novo biosynthesis and release from its conjugate forms, IAA can be converted from its precursor IBA [39]. IBA, as an endogenous storage auxin, is essential for maintaining appropriate IAA levels, thereby influencing primary root elongation and lateral root development [40]. Medium supplemented with high concentrations of auxin inhibited the root elongation of multiple buds, which may be related to auxin-stimulated ethylene production, and its synergistic effect reduced the cell length in the epidermal layer and thus inhibited root elongation [41]. In addition, we found that a large number of adventitious roots could break through the medium and grow upwards against geotropism during the rooting process, which may be related to conditions in the culture environment, such as humidity, light intensity, and medium composition. This phenomenon needs to be further investigated.
Acclimatization and transplantation are the keys to obtaining viable regenerated plants, and substrates that are loose and breathable, with water retention and fertilizer preservation properties are the basis for cultivating high-quality seedlings and play important roles in strengthening, preserving and promoting the roots of seedlings [42]. The physical properties of different substrates are different: nutrient soil has high organic matter content, rich mineral elements, and reasonable soil structure but with more microbial species; perlite has good aeration and drainage capacity, but its water retention capacity is average; vermiculite is light and porous, with good aeration, water absorption, and water-holding capacity, as well as containing Mg2+ and K+ that can be absorbed and utilized by plants [43,44]. Therefore, each substrate can be mixed in certain proportions according to plant needs so that it has good physical properties (i.e., less dense, appropriate porosity and permeability) and suitable chemical properties (i.e., suitable nutrients and an acid-base environment) to promote the growth and development of plants [42,45]. This study showed that the survival rate and plant height of plantlets were the highest and that the growth was better using a mixed substrate of perlite: vermiculite (1:1, v/v). Similar results were reported in Ribes nigrum [46], while regenerated seedlings of Pseudocydonia inensis [47], Vernicia fordii [48] and Pongamia pinna [49] had higher survival rates in different proportions of nutrient soil, vermiculite and perlite. The results indicated that different plants had different substrate requirements.
Terminal buds were used as suitable explants for an efficient regeneration system for M. anomala. The surfaces of explants were densely covered with trichomes, which increased the disinfection difficulty, and the best disinfection treatment for the terminal buds was 0.2% HgCl2 for 8 min. Plant growth regulators directly affected growth and development in the regeneration process of M. anomala. In initiate medium: MS basic medium supplemented with 0.5 mg/L 6-BA and 0.2 mg/L IBA for the high germination rate (100%) and the maximum bud height (1.70 cm) of the terminal bud. In proliferation medium: MS basic medium supplemented with 3.0 mg/L 6-BA and 0.2 mg/L IBA for a high proliferation rate (96%) and proliferation time (6.0) of terminal buds. Proliferation medium supplemented with 0.7 mg/L GA3 significantly increased the bud heights of multiple buds. In Rooting medium: MS basic medium supplemented with 0.5 mg/L IBA and 0.5 mg/L IAA for a high rooting rate (88%), root number (12.0) and root length (5.07 cm). The optimal substrate for seedling acclimation and transplanting was perlite: vermiculite (1:1), which resulted in the highest survival rate (97%) and plant height (5.89 cm). The results are of great significance for the promotion of M. anomala tissue culture technology, propagation, germplasm conservation, potential value development, and subsequent basic research.
Acknowledgement: We would like to thank the the Institute of Biology of Guizhou Province and Qiandongnan Forestry Scientific Research Institute of Guizhou Province for providing the plant material.
Funding Statement: This research was funded by the National Natural Science Foundation of China–Guizhou Provincial People’s Government Karst Science Research Center Project (U1812401), the National Natural Science Foundation of China (31760124), the Department of Education of Guizhou Province (grant code qianjiaoji (2022) 136), and the New Seedling Program of Guizhou Normal University (grant code 2021-B05).
Author Contributions: The authors confirm contribution to the paper as follows: study conception and design: Gaoyin Wu and Ya Tan; data collection: Ya Tan, Yundou Pang, Shuanggui Geng, Zhongcheng Peng, Qiuying Li, Shuang Wang, Lihong Zhang and Quanyan Tian; analysis and interpretation of results: Ya Tan, Yundou Pang; draft manuscript preparation: Gaoyin Wu and Yundou Pang; source of plant resources: Guanglin Tao; supervision, project administration, funding acquisition: Yingliang Liu. All authors reviewed the results and approved the final version of the manuscript.
Availability of Data and Materials: All data are available in the main text or the supplementary materials.
Conflicts of Interest: The authors declare no conflicts of interest to report regarding the present study.
Supplementary Materials: The supplementary material is available online at https://doi.org/10.32604/phyton.2024.056875.
References
1. Feng ZZ. Mussaenda anomaia. Encyclopedic Know. 2011;22:41 (In Chinese). [Google Scholar]
2. Wang YH, Wang HS, Wu SS, Li DS, Chen SN. Effect of Gelsemium elegans and Mussaenda pubescens, the components of a detoxification herbal formula, on disturbance of the intestinal absorptions of indole alkaloids in Caco-2 cells. Evid Based Compl Alternat Med. 2017;2017:6947948. doi:10.1155/ecam.v2017.1. [Google Scholar] [CrossRef]
3. Huong LT, Thao TP, Trang DT, Lee JH, Tai BH, Kiem PV, et al. Anti-osteoclastogenic cycloartane saponins from Mussaenda pubescens. Nat Prod Res. 2022;36(18):4597–604. [Google Scholar] [PubMed]
4. Zhang WQ, Wang DJ, Yang HY, Yang JW, Tao GL, Yuan MQ. Cutting propagation technology of Mussaenda anomaia Li. J Anhui Agri Sci. 2016;44(6):41–2+46 (In Chinese). [Google Scholar]
5. Gong ZH, Shen SX. Plant tissue culture. Beijing, China: Chemical Industry Press; 2022 (In Chinese). [Google Scholar]
6. Cheng ZJ, Zhu SS, Gao XQ, Zhang XS. Cytokinin and auxin regulates WUS induction and inflorescence regeneration in vitro in Arabidopsis. Plant Cell Rep. 2010;29(8):927–33. doi:10.1007/s00299-010-0879-8. [Google Scholar] [PubMed] [CrossRef]
7. Zhai N, Xu L. Pluripotency acquisition in the middle cell layer of callus is required for organ regeneration. Nat Plants. 2021;7(11):1453–60. doi:10.1038/s41477-021-01015-8. [Google Scholar] [PubMed] [CrossRef]
8. Guo HY, Wang YC, Liu HZ, Hu P, Jia YY, Zhang CR, et al. Exogenous GA3 application enhances xylem development and induces the expression of secondary wall biosynthesis related genes in Betula platyphylla. Int J Mol Sci. 2015;16(9):22960–75. doi:10.3390/ijms160922960. [Google Scholar] [PubMed] [CrossRef]
9. Zhou YQ, Zhang MJ, Zhang D, Zhang JJ, Li JJ, Chen XY. In vitro plant regeneration of Zenia Insignis Chun. Open Life Sci. 2018;13:34–41. doi:10.1515/biol-2018-0005. [Google Scholar] [PubMed] [CrossRef]
10. Gonbad RA, Rani Sinniah U, Aziz MA, Mohamad R. Influence of cytokinins in combination with GA3 on shoot multiplication and elongation of tea clone Iran 100 (Camellia sinensis (L.) O. Kuntze). Scientif World J. 2014;2014:943054. [Google Scholar]
11. Zakavi M, Askari H, Irvani N. Optimizing micropropagation of drought resistant Pyrus boissieriana Buhse. Physiol Mol Biol Plants. 2016;22(4):583–93. doi:10.1007/s12298-016-0387-6. [Google Scholar] [PubMed] [CrossRef]
12. Wang MJ, Zhao QL, Bai BY, Xiang SZ, Hong CH. Research progress on tissue culture and planting technology of Dendrobium officinale. South-Central Agri Sci Tech. 2023;44:217–21 (In Chinese). [Google Scholar]
13. Zhang WQ, Wang DJ. A preliminary study on tissue culture of rare and endangered plant Mussaenda anomala. J Central South Univ Forest Tech. 2016;36(10):12–15+47 (In Chinese). [Google Scholar]
14. Wu GY, Wei XL, Wang X, Wei Y. Induction of somatic embryogenesis in different explants from Ormosia henryi Prain. Plant Cell Tiss Org Cult. 2020;142:229–40. doi:10.1007/s11240-020-01822-5. [Google Scholar] [CrossRef]
15. Han S, Liu M, Wang Y, Chen J. Tissue culture and rapid propagation technology for Gentiana rhodantha. Open Life Sci. 2023;18(1):20220565. doi:10.1515/biol-2022-0565. [Google Scholar] [PubMed] [CrossRef]
16. Maity SK, De KK, Kundu AK. In vitro propagation of Mussaenda erythrophylla Schum and Thom cv. scarlet through multiple shoot regeneration. Indian J Exp Bio. 2001;39(11):1188–90. [Google Scholar] [PubMed]
17. Yang H, Chen WJ, Yang B, Li XF, Chen T, Liu ZZ, et al. Preliminary study on tissue culture of stem segments in Carya illinoensis. Non-Wood Forest Res. 2017;35(2):220–4 (In Chinese). [Google Scholar]
18. Chang EM, Jiang ZP, Liu JF, Jia ZR, Li X, Zhang JQ, et al. Establishment of tissue culture system of ancient Platycladus orientalis L. Forest Res. 2023;36(4):41–9 (In Chinese). [Google Scholar]
19. Das P, Rout GR, Das AB. Somatic embryogenesis in callus culture of Mussaenda erythrophylla cvs. Queen Sirikit and Rosea. Plant Cell Tiss Org Cult. 1993;35:199–201. doi:10.1007/BF00032970. [Google Scholar] [CrossRef]
20. Corredoira E, Ballester A, Ibarra M, Vieitez AM. Induction of somatic embryogenesis in explants of shoot cultures established from adult Eucalyptus globulus and E. saligna × E. maidenii trees. Tree Physiol. 2015;35(6):678. doi:10.1093/treephys/tpv028. [Google Scholar] [PubMed] [CrossRef]
21. Adugna AY, Feyissa T, Tasew FS. Optimization of growth regulators on in vitro propagation of Moringa stenopetala from shoot explants. BMC Biotechnol. 2020;20(1):60. doi:10.1186/s12896-020-00651-w. [Google Scholar] [PubMed] [CrossRef]
22. Zhu XT, Zhu HJ, Ji W, Hong EM, Lu ZY, Li BL, et al. Callus induction and transcriptomic analysis of in vitro embryos at different developmental stages of peony. Front Plant Sci. 2022;13:1046881. doi:10.3389/fpls.2022.1046881. [Google Scholar] [PubMed] [CrossRef]
23. Duan SY, Xin RJ, Guan SX, Li XT, Fei RW, Cheng W, et al. Optimization of callus induction and proliferation of Paeonia lactiflora Pall. and Agrobacterium-mediated genetic transformation. Front Plant Sci. 2022;13:996690. doi:10.3389/fpls.2022.996690. [Google Scholar] [PubMed] [CrossRef]
24. Qahtan AA, Faisal M, Alatar AA, Abdel-Salam EM. Callus-mediated high-frequency plant regeneration, phytochemical profiling, antioxidant activity andgenetic stability in Ruta chalepensis L. Plants. 2022;11(12):1614. doi:10.3390/plants11121614. [Google Scholar] [PubMed] [CrossRef]
25. Ashokhan S, Othman R, Abd Rahim MH, Karsani SA, Yaacob JS. Effect of plant growth regulators on coloured callus formation and accumulation of azadirachtin, an essential biopesticide in Azadirachta indica. Plants. 2020;9:352. doi:10.3390/plants9030352. [Google Scholar] [PubMed] [CrossRef]
26. Fehér A. Why somatic plant cells start to form embryos? In: Mujib A, Šamaj J, editors. Somatic embryogenesis. Berlin: Heidelberg; 2005. p. 85–101. [Google Scholar]
27. Cramer CS, Bridgen MP. Somatic embryogenesis and shoot proliferation of Mussaenda cultivars. Plant Cell Tiss Org Cult. 1997;50:135–8. doi:10.1023/A:1005900110956. [Google Scholar] [CrossRef]
28. Frick EM, Strader LC. Roles for IBA-derived auxin in plant development. J Exp Bot. 2018;69(2):169–77. doi:10.1093/jxb/erx298. [Google Scholar] [PubMed] [CrossRef]
29. Aygun A, Dumanoglu H. In vitro shoot proliferation and in vitro and ex vitro root formation of Pyrus elaeagrifolia Pallas. Front Plant Sci. 2015;6:225. [Google Scholar] [PubMed]
30. Harahap F, Poerwanto R, Suharsono, Suriani C, Rahayu S. In vitro growth and rooting of Mangosteen (Garcinia mangostana L.) on medium with different concentrations of plant growth regulator. Hayati J Biosci. 2014;21(4):151–8. doi:10.4308/hjb.21.4.151. [Google Scholar] [CrossRef]
31. Das P. Mass cloning of Rose and Mussaenda, popular garden plants, via somatic embryogenesis. Hort Sci. 2010;37:70–8. doi:10.17221/57/2009-HORTSCI. [Google Scholar] [CrossRef]
32. Li J, Li CY. Seventy-year major research progress in plant hormones by Chinese scholars. Sci Sin Vitae. 2019;49:1227–81 (In Chinese). [Google Scholar]
33. Camara MC, Vandenberghe LPS, Rodrigues C, Oliveira J, Faulds C, Bertrand E, et al. Current advances in gibberellic acid (GA3) production, patented technologies and potential applications. Planta. 2018;248(5):1049–62. doi:10.1007/s00425-018-2959-x. [Google Scholar] [PubMed] [CrossRef]
34. Li SX, Cong YH, Liu YP, Wang TT, Shuai Q, Chen NN, et al. Optimization of Agrobacterium-mediated transformation in Soybean. Front Plant Sci. 2017;8:246. [Google Scholar] [PubMed]
35. Wu LL, Mitchell JP, Cohn NS, Kaufman PB. Gibberellin (GA3) enhances cell wall invertase activity and mRNA levels in elongating dwarf pea (Pisum sativum) shoots. Int J Plant Sci. 1993;154(2):280–9. doi:10.1086/297108. [Google Scholar] [PubMed] [CrossRef]
36. Zheng L, Xiao Z, Song W. Effects of substrate and exogenous auxin on the adventitious rooting of Dianthus caryophyllus L. HortScience. 2020;55(2):170–3. doi:10.21273/HORTSCI14334-19. [Google Scholar] [CrossRef]
37. Tang Y, Liu SZ, Wu CL, Si FF, Zeng MS, Lu YS, et al. Optimization of rooting medium for in Monochasma savatieri. Mol Plant Breed. 2022;20:6433–40 (In Chinese). [Google Scholar]
38. Zhou LiXX, Shen RY, Yang HL, L. Effect of exogenous plant growth regulators and rejuvenation measures on the endogenous hormone and enzyme activity responses of Acer mono Maxim in cuttage rooting. Int J Mol Sci. 2023;24(15):11883. doi:10.3390/ijms241511883. [Google Scholar] [PubMed] [CrossRef]
39. Zhao H, Wang YT, Zhao S, Fu Y, Zhu L. Homeobox protein 24 mediates the conversion of indole-3-butyric acid to indole-3-acetic acid to promote root hair elongation. New Phytol. 2021;232(5):2057–70. doi:10.1111/nph.v232.5. [Google Scholar] [CrossRef]
40. Jawahir V, Zolman BK. Long chain acyl CoA synthetase 4 catalyzes the first step in peroxisomal indole-3-butyric acid to IAA conversion. Plant Physiol. 2021;185(1):120–36. doi:10.1093/plphys/kiaa002. [Google Scholar] [PubMed] [CrossRef]
41. Alarcón MV, Lloret PG, Salguero J. Synergistic action of auxin and ethylene on root elongation inhibition is caused by a reduction of epidermal cell length. Plant Signal Behav. 2014;9(3):e28361. doi:10.4161/psb.28361. [Google Scholar] [PubMed] [CrossRef]
42. Cuesta B, Vega J, Viuar-salvador P. Root growth dynamics of Aleppo pine (Pinus halepensis Mill.) seedings in relation to shoot elongation, plant size and tissue nitrogen concentration. Trees. 2010;24:899–908. doi:10.1007/s00468-010-0459-0. [Google Scholar] [CrossRef]
43. Chen SY, Xiong YP, Yu XC, Pang JH, Zhang T, Wu KL, et al. Adventitious shoot organogenesis from leaf explants of Portulaca pilosa L. Sci Rep. 2020;10:3675. doi:10.1038/s41598-020-60651-w. [Google Scholar] [PubMed] [CrossRef]
44. Akwatulira F, Gwali S, Bosco J, Okullo L, Ssegawa P, Mbwambo JR, et al. Influence of rooting media and indole-3-butyric acid (IBA) concentration on rooting and shoot formation of Warburgia ugandensis stem cuttings. Afr J Plant Sci. 2011;5:421–9. [Google Scholar]
45. Chen JL, Xie J, Sun Y, Shi FH, Shen YB, Zhang YH, et al. Effects of container size and substrate composition on the growth of ×Taxodiomera peizhongii container seedlings. J Northeast Forestry Univ. 2023;51(7):33–8 (In Chinese). [Google Scholar]
46. Jiao HY. Rapid tissue culture and direct regeneration of Ribes nigrum L. ‘Braude’ (Master’s Thesis). Tianjin Agricultural University: Tianjin, China; 2020 (In Chinese). [Google Scholar]
47. Liu YH. Study on tissue culture and cutting technology of Chaenomeles Sinensis Koehen (Master’s Thesis). Henan Agricultural University: China; 2019 (In Chinese). [Google Scholar]
48. Zhang HJ, Qin SF, Cao H, Zhang QW, Luo KM. Plant regeneration in Vernicia fordii from apical bud. Heliyon. 2020;6(5):e03884. doi:10.1016/j.heliyon.2020.e03884. [Google Scholar] [PubMed] [CrossRef]
49. Tan SN, Tee CS, Wong HL. Multiple shoot bud induction and plant regeneration studies of Pongamia pinnata. Plant Biotechnol. 2018;35(4):325–34. doi:10.5511/plantbiotechnology.18.0711a. [Google Scholar] [PubMed] [CrossRef]
Cite This Article
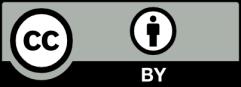
This work is licensed under a Creative Commons Attribution 4.0 International License , which permits unrestricted use, distribution, and reproduction in any medium, provided the original work is properly cited.