Open Access
ARTICLE
Effects of Forest Types on Soil Available Nutrients and Carbon Contents in Coastal Areas, China
1 Co-Innovation Center for Sustainable Forestry in Southern China, College of Soil and Water Conservation, Nanjing Forestry University, Nanjing, 210037, China
2 National Positioning Observation and Research Station of Forest Ecosystem in Changjiang River Delta, Nanjing, 210037, China
* Corresponding Author: Haijun Sun. Email:
Phyton-International Journal of Experimental Botany 2024, 93(10), 2557-2569. https://doi.org/10.32604/phyton.2024.056868
Received 01 August 2024; Accepted 14 September 2024; Issue published 30 October 2024
Abstract
Clarifying the soil nutrient dynamics caused by forest type variations in the coastal region helps scientifically to apply fertilizer to forest plantations and enhance the carbon (C) sink capacity. Pure forests of Ligustrum and Metasequoia, as well as their mixed forests, in a coastal region of China were investigated by collecting 0–20 and 20–40 cm soil samples and analyzing their differences in bulk density, water content, pH, soil organic matter (SOM), ammonium (NH4+–N), nitrate (NO3––N) and total nitrogen (TN), available phosphorus (AP) and potassium (AK), microbial biomass C (MBC) and N (MBN), and enzyme activity. The results demonstrated that different forest types had no significant (p ≥ 0.05) effect on 0–20 cm soil bulk density, water content, pH, NH4+–N, and SOM. However, the surface soil NO3––N, TN, AP, and AK contents as well as enzyme activity changed significantly (p < 0.05), in which the soil AK content of the ligustrum × metasequoia mixed forest was 47.5% and 65.5% higher than that of the ligustrum and metasequoia pure forest, respectively. The mixed forest soil had the highest MBN content, which was significantly (p < 0.05) 25.1% higher than that in the pure metasequoia forest. Meanwhile, soil phosphatase activities in ligustrum and metasequoia pure forests were significantly (p < 0.05) lower than those in the mixed forests by 17.4% and 43.1%, respectively. However, soil NO3––N and AP contents in the metasequoia pure forest were significantly (p < 0.05) higher than those in the ligustrum pure forest and mixed forests. Soil MBC content and reductase NO3––N activity were significantly (p < 0.05) higher in ligustrum pure forest than in metasequoia pure forest and mixed forests. In addition, the results of two-way ANOVA showed that there were no significant (p ≥ 0.05) differences in nutrient contents (e.g., NH4+–N, AP, AK, and SOM) in different soil layers (0–20 and 20–40 cm) within the same forest type, except for NO3––N. However, forest types had a significant (p < 0.05) impact on NO3––N and AP contents in 20–40 cm soil layer. Combining the two factors of forest stand and soil layer, there was a significant (p < 0.05) interaction effect for their soil NH4+–N, NO3––N, AP, and AK contents. In conclusion, significant (p < 0.05) differences were observed in nutrient contents in 0–20 cm soil layer from different forest types, with soil fertility indices inmixed forests generally higher than in pure forests. Therefore, establishing mixed forests in coastal saline region is recommended to retain soil fertility and to enhance the C sink capacity of forestry.Keywords
Soil salinization greatly impedes the sustainable development of saline-alkaline ecological environments and agroforestry production [1]. There are approximately 9.9 × 107 hm2 of saline-alkaline land in China, and there are more than 6.8 × 105 hm2 of silty coastal saline-alkaline land in the coastal area of northern Jiangsu, which are important potential resources for artificial afforestation [2,3]. Therefore, enhancing and utilizing coastal saline–alkaline land resources for alleviating the current situation of land resource utilization, ensuring national economic development and food security, and safeguarding the ecological environment [4]. Over the past decades, saline-alkaline land has been improved through traditional engineering methods, such as swidden and ditching, and developed and utilized saline-alkaline land by using mulching, tillage, straw return, and the cultivation of salt-tolerant plants [5]. Moreover, large-scale ecological protection and reserve forests are frequently established in coastal areas to enhance the ecological environment [5,6]. The primary afforestation species include Ulmus pumila, Ligustrum, Metasequoia, Zelkova, Taxodium, and Apocarya, all of which exhibit a degree of salt and moisture tolerance, thrive in coastal saline and alkaline soils, and offer significant economic and aesthetic value [7,8].
Coastal saline area exhibits adverse conditions, such as high soil salinity and low nutrient content, therefore, soil fertility is a critical factor in establishing basal forest belts and supporting forest tree growth. Research indicates planting Tamarisk on saline soils alters soil salinity and moisture [9], while planting Suaeda glauca facilitates salt desalination of coastal soils and alters soil physicochemical and microbial properties as well as enzyme activities [10]. Understory soil microbial communities and enzyme activities considerable variation among different forest types, attributable to differences in tree species growth patterns or site conditions. Previous research has demonstrated that forest type significantly influences soil carbon (C), nitrogen (N) and phosphorus (P) contents as well as their stoichiometric ratios [11]. For instance, a study of mixed Picea abies × Fagus sylvatica forests revealed that nutrient levels in leaves and soils were higher in the mixed coniferous forests than the pure conifer forests [12]. Numerous studies have demonstrated that mixed forests help to enhance soil fertility, improve the forest floor nutrient status, and increase forest stability and productivity [13]. Specifically, the soil total N (TN), available P (AP), and organic C (SOC) concentrations were increased by 58%, 17%, and 66%, respectively, in mixed stands compared to monoculture types [14]. Moreover, even under identical site conditions, variations in soil nutrients persist among different forest types. For instance, in plantation forests, the rapid growth of trees during the young forest period results in high fertilizer demand, and fertilizer application can significantly change the spatial heterogeneity of soil nutrients. Zheng et al. [15] found that P fertilization may be an effective measure to increase both forest soil C and P effectiveness in the young forests. Song et al. [16] applied N fertilization to plantation forests of varying ages along the coastal area of China to increase the N resorption efficiency of leaf and decrease soil P accumulation factor of root in young forests. Therefore, plantation forests of different types require targeted fertilization to maximize the promotion of forest growth, increase soil C sequestration, and promote the C sink capacity of plantation forests.
The extensive and complex root systems of forests stabilize the soil, thereby influencing the spatial distribution of soil nutrients. Different vegetation types can spatially influence soil fertility, including soil nutrients such as total P (TP), TN, AP and available N [17]. Moreover, variations in apoplastic litter and root systems among tree species, leading to significant differences in the fertility status of the understory soil [18]. Variations in the type and amount of litter across different forest types, leading to differences in the nutrients returned, as most of the litter exists in the 0–20 cm topsoil under the forest, which is known as the surface polymerization. Surface litter has a high content and rapid decomposition rate, resulting in distinct nutrient characteristics in the surface soil compared to the subsurface soil, with most available nutrients decrease with the depth of the soil profile [19]. For instance, research indicates that the C/P in the soil surface layer of mixed forests with Masson pine and Erythrophloeum ferdii is higher than that of the pure Masson pine forests, and soil N/P was also higher than at both the surface and subsurface layers of mixed forests [20]. Thus, investigating the vertical profile distributions of selected soil nutrients under forest is essential.
The impact of forest type on soil nutrients, the underlying causes of nutrient differences between mixed and pure types, and the effects on deep soil nutrients are worth exploring. We hypothesized that surface soil nutrients would differ significantly among forest types, with mixed forests exhibiting the highest nutrient content. This study examined the soil nutrient status under three forest types in a typical coastal area to elucidate differences in understory soil fertility among these forest types. Additionally, comparisons were made between the 0–20 cm and 20–40 cm soil layers to assess the influence of profile depth and forest type on coastal forest soil fertility. The results of our study offer valuable insights for scientific fertilization, tree species selection, and the enhancement of C sink capacity in plantation forests located in coastal saline regions.
2.1 Overview of Experimental Forest
The sampling sites were situated in a typical coastal forest at Dafeng District, Yancheng City, Jiangsu Province (120°78′ E, 33°06′ N), China, at an altitude of 0–10 m. The forest was reclaimed in 1991, and the southern portion of the site adjoins the Dafeng Elk Nature Reserve, a significant coastal protection forest in Jiangsu Province, China. The forest farm is a state-owned. After 40 years of tidal inundation, the tide was blocked by the construction of the seawall, leading to the complete reclamation of the land and its conversion to Poplar or metasequoia protection forest. The climate of the study area is characterized as transitional, maritime, and monsoonal. The average annual precipitation is 1042 mm, which is considerable; however, it is unevenly distributed, with the rainy season primarily occurring from June to August. The average annual temperature and evaporation are 14.1°C and 1417 mm, respectively. The frost–free period lasts approximately 230 days [21]. The soil is representative of silt alluvial saline soil, derived from marine sediments, with parent material consisting of modern sediment that has been accumulating for approximately 60 years. Through long–term natural action and anthropogenic improvement, most of the heavy saline soils have gradually evolved into medium and light saline soils and have been reclaimed for agricultural and forestry use. The soils at the experimental site are homogeneous throughout the profile, with the following properties: pH 8.1–9.0, electric conductivity 128.8~205 μs/cm, salt content 1.3%–1.5%, soil bulk density 1.3–1.6 g/cm3, and porosity 40%–50%.
The forest types investigated included pure forests of ligustrum (Ligustrum lucidum Ait.) and metasequoia (Metasequoia glyptostroboides) as well as their mixed forests, which were afforested in Mar 2019. The proportion of mixed forest was approximately 50%. Prior to afforestation, the land was grassland, resulting in uniform initial soil conditions (Table 1). During afforestation, each tree received 0.25 kg P fertilizer and 1.5 kg of organic fertilizer. Additionally, management practices, including fertilization and weeding, adhered to local forest management protocols.
2.2 Soil Sample Collection and Nutrient Determination
Sample plots measuring 5.0 m × 5.0 m were established in each forest type, with three replicates per type. Soil samples from 0–20 and 20–40 cm depths in ligustrum, metasequoia pure, and their mixed forests, as well as samples from 0–20 cm in the cutting ring, were collected on 26 February, 2023, using a five–point method. Soil samples that collected from the same site and depth profile were thoroughly mixed after removing impurities such as fallen leaves, roots, and stones, and were subsequently transported to the laboratory in self–sealing bags. Approximately 500 g of fresh soil was seriously air–dried and thereafter ground to pass through 2 and 0.149 mm nylon sieves for the analysis of forest soil nutrients contents and enzyme activities, respectively. The remaining fresh soil was stored at 4°C for the determinations of available N, microbial biomass C and N.
Soil bulk density was measured using the cutting ring method. Gravimetric water content (GWC) was determined by drying at 105°C for 48 h. Soil pH was measured with air-dried samples at a soil: water ratio of 1:5 using a potentiometric method. Soil organic matter (SOM) was determined by oxidation–external heating method with potassium dichromate (K2Cr2O7). Approximate 1.0 g of air-dried soil sample (0.15 mm) was prepared, adding with 2 g of accelerator and purified water. Subsequently, 5 mL of H2SO4 was added to the mixture, which was then transferred to a digestion tube for digestion. Soil TN content was determined by the Kjeldahl N determination after digestion. Inorganic N including NH4+–N and NO3––N were extracted from moist soil with 2 mol/L KCl solution and then determined by indophenol blue colorimetry and UV spectrophotometry. Soil AP was determined using the 0.5 mol/L NaHCO3 extraction–molybdenum antimony colorimetric method. Soil available potassium (AK) was determined using the 1 mol/L NH4OAc extraction–flame photometric method. Microbial biomass C (MBC) and N (MBN) were measured using the chloroform fumigation–K2SO4 extraction method [22,23]. Urease (URE) activity was assessed using the colorimetric method with phenol-sodium hypochlorite and a multifunctional enzyme marker; alkaline phosphatase (ACP) activity was determined by diphenyl disodium phosphate colorimetry. Approximately 0.2 g of fresh soil was weighed, ground with liquid N, and extracted with 25 mmol/l PBS, the mixture was centrifuge for 10 min, and the supernatant was used to measure nitrate reductase (NR) activity using the sulfonamide colorimetric method. All the above methods were referred as Soil Agrochemical Analysis [24].
Data were organized and analyzed using the software of Excel 2010 and SPSS 26.0. Differences among different forest types were analyzed using one-way ANOVA, followed by multiple comparisons with Duncan’s method. The effects of forest type, soil depth, and their interactions on the soil properties were assessed using two-way ANOVA. Graphs were generated using Origin 2022.
3.1 Soil Bulk Density, Gravimetric Water Content, and pH
There were no significant (p ≥ 0.05) differences in the soil bulk density, GWC, and pH among three forest types, which ranged from 1.15–1.23 g/cm3, 25.0%–28.0%, and 8.38–8.53, respectively (Fig. 1A–C). Of these, the metasequoia pure forest had the lowest soil bulk density. Compared with the pure forest, the soil pH in the ligustrum × metasequoia mixed forests decreased by 0.15 units.
Figure 1: Differences in 0–20 cm soil bulk density (A), gravimetric water content (B), and pH (C) across forest types. The data in the figure are expressed as the mean ± standard deviation (n = 3). Different lowercase letters denote significant differences between treatments (p < 0.05). The absence of lowercase letters indicates that differences are not significant (p ≥ 0.05)
3.2 Nutrient Contents of 0–20 cm Soil
No significant (p ≥ 0.05) differences were observed in NH4+–N and SOM contents in 0–20 cm surface soil that sampled from three forest types, with only 0.34–0.42 mg/kg and 12.4–14.9 g/kg, respectively (Fig. 2A,D). For different forest types, there were significant (p < 0.05) differences in soil NO3––N, TN, AP, and AK contents. The soil NO3––N content in metasequoia pure forests was significantly (p < 0.05) 23 times higher than in both ligustrum pure forests and ligustrum × metasequoia mixed forests (Fig. 2B). In contrast, soil TN content in metasequoia pure forest was 42.8 mg/kg, which was 24.4% and 26.1% lower than that in ligustrum pure forest and mixtures, respectively (Fig. 2C). Soil AP content decreased in the following order: metasequoia pure forest > mixed forest > ligustrum pure forest, with the AP content in ligustrum pure forest was 40.9% lower than that in metasequoia pure forest (Fig. 2E). Conversely, AK content was 47.4% to 65.5% higher in mixed forests compared to the corresponding pure forests (Fig. 2F).
Figure 2: (Continued)
3.3 Soil Microbial Biomass C, N
Significant (p < 0.05) differences were observed in the 0–20 cm soil MBC and MBN contents among the three forest types (Fig. 3A,B). The highest MBC content of 279.7 mg/kg was recorded in ligustrum pure forest, which was significantly (p < 0.05) higher by 5.0% and 15.1% compared to ligustrum × metasequoia mixed forests and metasequoia pure forests, respectively. However, the highest MBN content was found in the mixed forests, which was 25.1% significantly (p < 0.05) higher than that in the metasequoia pure forests.
Figure 3: Differences in MBC (A) and MBN (B) contents of 0–20 cm topsoil from different forest types. The data in the figure are expressed as the mean ± standard deviation (n = 3). Different lowercase letters denote significant differences between treatments (p < 0.05)
There was no significant difference (p ≥ 0.05) in the 0–20 cm soil URE enzyme activity between different forest types, whereas significant (p < 0.05) differences were noted in both ACP and NR enzyme activities. ACP enzyme activities of soils from ligustrum × metasequoia mixed forests were significantly (p < 0.05) 17.4% and 43.1% higher than in ligustrum and metasequoia pure forests, respectively. Conversely, soil NR enzyme activity was highest in ligustrum pure forest at 0.19 mg/g ± 24 h, which was significantly (p < 0.05) higher by 18.9% compared to the mixed forest and 58.3% in the metasequoia pure forest (Table 2).
3.5 Soil Properties across Different Forest Types and Soil Layers
Table 3 reveals significant (p < 0.05) differences in soil properties between the two layers across different forest types. The contents of AP and SOM increasing soil depth, while pH decreased. In the 0–20 cm soil layer, NO3––N content in metasequoia pure forests was approximately 23 times higher than in ligustrum pure forests and ligustrum × metasequoia mixture forests. Additionally, AK content in the mixture forests was significantly (p < 0.05) higher than in both pure forests, and AP content of metasequoia pure forests was significantly (p < 0.05) higher than in ligustrum and the mixture forests. In contrast, NH4+–N content in the 20–40 cm layer soil of mixed forests was significantly (p < 0.05) 14.8% and 34.1% higher than that of ligustrum and metasequoia pure forests, respectively. Moreover, ligustrum pure forests and mixed forests were with 28.1%–35.3% significantly (p < 0.05) higher soil AP contents than that of metasequoia pure forests. No significant difference (p ≥ 0.05) was observed in pH, NO3––N, SOM, and AK contents in the 20–40 cm soil layer.
Additionally, comparing of soil properties across different depth layers within the same forest type, revealed that the NH4+–N, NO3––N, and AP contents in the mixed forests were higher in the 20–40 cm soil layer compared to the 0–20 cm soil layer. In contrast, the AP content in the 20–40 cm soil layer of ligustrum pure forests was significantly (p < 0.05) higher than that in the 0–20 cm soil layer. Furthermore, AK content in the 20–40 cm soil layer of both ligustrum and metasequoia pure forests was higher than in the 0–20 cm soil layer. Two-way ANOVA results indicated a significant (p < 0.05) effect of forest type on NO3––N and AK content, and a significant (p < 0.05) effect of soil layer on pH, NO3––N and AP content. Moreover, forest type and soil layer exhibited a significant (p < 0.05) interaction effect on NH4+–N, NO3––N, AP and AK contents in forest soil.
4.1 Effects of Different Forest Types on Soil Nutrients
Community biomass, species cover, and microbial decomposition of plant roots and litter within different forest types influence soil nutrient fixation, transformation, and cycling processes to varying degrees [25]. The study revealed significant (p < 0.05) differences in soil nutrients among various forest types in the coastal region. Soil nutrients in pure forests varied considerably among tree species, with metasequoia pure forests exhibiting higher soil NH4+–N, NO3––N, SOM, and AP contents. In contrast soil nutrients in the ligustrum × metasequoia mixed forest were generally more stable and balanced compared to the pure forests. Specifically, soil TN and AK contents were higher in the mixed forest compared to the two pure forests, suggesting that the mixed forest structure helps to balance soil fertility [26].
Soil properties across different forest types in this study varied considerably but generally exhibited a certain pattern. There were no significant (p ≥ 0.05) differences in soil bulk density, GWC, and pH among the three forest types. Soil bulk density was slightly lower in metasequoia pure forest and mixed forest compared to ligustrum pure forest, indicating that the root penetration ability of metasequoia and the mixed forest was greater than that of ligustrum, thereby reducing soil bulk density and increasing soil porosity. However, GWC in ligustrum pure and mixed forests was higher than in metasequoia pure forest, as ligustrum has a larger leaf area and higher coverage of the soil surface, which can retain water to a large extent, whereas metasequoia litter leaves have weaker water–holding capacity. Under saline conditions, surface soil pH was similar across forest types, explaining that the forest type had a weak influence on soil pH. In addition, the pH of soil in the mixed forest was slightly lower than in pure forests, suggesting that the mixed forest can slightly reduce soil pH in saline conditions and mitigate soil salinity. Previous studies have demonstrated that planting salt-tolerant plants on saline soils can alter soil properties and reduce soil pH [27], a finding that is corroborated by our results. Yang et al. [28] reported that planting salt–tolerant species or plants could reduce the salt content of coastal saline soils and increased nutrients availability. Consequently, reclamation and planting mixed forests in coastal saline areas can help regulate soil salinity and maintain soil fertility [29]. In this research, the contents of available N, AP and SOM in the surface soil of metasequoia pure forests were significantly (p < 0.05) higher than those in ligustrum pure forests and the mixed forests. This can be attributed to the fact that metasequoia litter decomposes rapidly due to its fine shredding, which is more conducive to microbial activity. Consequently, urease activity is relatively high, leading to greater accumulation of nutrients, including available N. Therefore, the content of NO3––N in the surface soil of metasequoia pure forest was significantly higher compared to the other two forest types. NO3––N serves as a form of available N fertilizer, converted from NH4+–N through nitrification. Since ligustrum is an evergreen broad–leaved species with less understory litter, it results in increased leaching of soil NO3––N [30], thus reducing the conversion of soil NO3––N into NH4+–N. In addition, metasequoia pure forests with high depression conditions promote the accumulation of soil C and N nutrients. Surface litter also reduces the rate of soil C and N nutrient loss by impeding surface runoff [31,32]. Compared with metasequoia pure forests, the AP content of surface soil in metasequoia pure forests was significantly (p < 0.05) higher than that in ligustrum pure forests and mixed forests, indicating that the roots of metasequoia pure forests could fix more AP. Soil organic matter is a crucial indicator of soil fertility, and SOM did not differ significantly (p < 0.05) among the different forest types, illustrating that SOM is a relatively stable measure of soil nutrients and is not easily influenced by forest type. However, the highest SOM content in the surface layer of metasequoia pure forests is attributable to the fact that coniferous species with low quality, high litter content decompose slowly, and most of the organic matter produced accumulates in the litter layer [33]. Therefore the SOM content in the soil of 0–20 cm layer is higher, which in turn increases the effective soil N and P nutrients [11]. Compared with pure forests, the spatial structure, root distribution, and litter quality of mixed forests are superior, and the combination of various litter types produces a synergistic effect that enhances soil microorganisms activity, accelerates litter decomposition, and improves nutrient cycling and utilization [34]. In this study, the surface soil TN and AK contents in the mixed forests were significantly (p < 0.05) higher than those in the pure forests, while other soil fertility indices remained relatively stable. The level of soil TN content is to evaluate the overall level of soil fertility, and the establishment of mixed forests helps maintain higher soil N content and improve soil fertility. Meanwhile, the soil AK content in mixed forests exceeded the medium level (100–150 mg/kg), indicating a higher K content. In contrast, AK content in ligustrum and metasequoia pure forests was lower, ranging from 50–100 mg/kg, indicating a deficiency [35]. Therefore, the camping pattern of mixed forests within the stand is superior to that of pure forests. In addition, the relatively high AK but low AP contents in the 0–20 cm soil across different forest types indicate that P the limiting factor for forest growth in the study area. From a practical perspective, the application of P fertilizer in the study area could improve forestry productivity.
4.2 Effects of Different Forest Types on Soil Microbial Biomass C and N
Soil MBC and MBN are crucial sources of soluble organic C and N [36]. Microbial biomass in various forest types is greatly influenced by soil properties, litter quality, and fine root inputs [37]. The effects of different tree species on soil properties through a variety of mechanisms, such as litter quality and nutrient return, root nutrient uptake, canopy sequestration, and alterations to the soil biome on topsoil microbial C and N are different [38] and are also an important reason for the differences in soil nutrients across different forest types in the coastal region. In this research, the MBN content in the topsoil layer was highest in the mixed forest, while the MBC content was highest in the ligustrum pure forest, consistent with Kooch et al.’s study [39]. This suggests that mixed forests benefit from nutrient restitution due to the diversity of litter species, root secretion and root turnover. This diversity influences soil MBC through root C input, promoting the synthesis of soil MBC and MBN, and effectively enhancing topsoil fertility [40,41]. Additionally, there was a positive correlation between soil MBC, MBN, and TN concentrations [42]. Soil TN was significantly higher in the mixed forest than in the pure forest. These findings suggest that mixed forests may promote microbial growth, enhance soil C pool utilization, and increase C sink capacity.
4.3 Effects of Different Forest Types on Soil Enzyme Activity
Soil enzyme activity has long been recognized as an indicator of soil quality, as it regulates plant nutrient supply and microbial growth [43]. Additionally, soil enzymes, which possess specific catalytic abilities, reflect microbial activity and indicate soil nutrient capacity [44]. Among them, soil URE enzyme effectively promotes the conversion of organic N into available inorganic N. URE enzyme activity is generally higher in broad-leaved evergreen forests compared to coniferous forests and is also higher in mixed forests than in pure forests [45]. The results of the present study align with these findings. Specifically, URE enzyme activities were higher in ligustrum pure forest and mixed forest were higher, indicating that mixed forests significantly increased soil ACP enzyme activity [46]. The dense root system in the topsoil of mixed forest facilitates greater fixation of AP content. Meanwhile, a variety of root exudates promote the hydrolysis of soil organophosphorus, converting it into forms of P that can be directly absorbed and utilized by plants and microorganisms [47], thereby increased P uptake by forest trees. However, NR enzyme activity was significantly (p < 0.05) higher in ligustrum pure forests compared to metasequoia pure forests and mixed forests. Ligustrum pure forests and mixed forests exhibited very low NO3––N content but relatively higher NR enzyme activity. This is because NO3––N serves as the substrate for NR reduction, and NR is the primary enzyme in the NO3––N assimilation process [48]. Its activity directly impacts the utilization rate of inorganic N in the soil [49], suggesting that ligustrum pure forests and mixed forests can efficiently utilize soil NO3––N. Therefore, different forest types affect soil enzyme activity, and the establishment of mixed forests promotes improved soil enzyme activity.
4.4 Effects of Different Forest Types on Soil Fertility in Different Soil Layers
This study analyzed properties including pH, NH4+–N, NO3––N, available P, K, and SOM at different soil depth. Results indicated significant (p < 0.05) differences in NO3––N, AP, and AK contents among the three forest types in the 0–20 cm soil layer. While only NH4+–N and AP content reached significant (p < 0.05) differences in the 20–40 cm soil layer. This indicates that surface soil nutrients are strongly influenced by the type of forest, with differences in litter species and root system. Since the root system is primarily concentrated in the soil surface layer, decomposition of litter and humus layer leads to increased nutrient levels in the surface soil, whereas deeper layers receive comparatively fewer nutrients [50]. The contents of NH4+–N, NO3––N, and AP in the 20–40 cm soil layer of ligustrum × metasequoia mixed forest were higher than those in the 0–20 cm soil layer, indicating that nutrient levels increased with soil depth, and soil fertility remained elevated up to 40 cm. However, pH decreases with the increase of soil depth, reflecting a reduction in alkalinity in deeper soil layers, suggesting that tree roots may ameliorate saline-alkali conditions. Moreover, examining only the 0–40 cm soil layer is insufficient, deeper soil fertility properties require further investigated. Two-way ANOVA results indicated significant (p < 0.05) effects of forest type on NO3––N and AK contents, and significant effects of soil depth on NO3––N and AP contents, confirming that both forest type and soil depth influence partial soil nutrient content. Additionally, forest type and soil layers had significant (p < 0.05) interaction effects on soil NH4+–N, NO3––N, AP and AK contents. These results indicate a strong correlation between forest type and soil layer with respect to soil fertility.
(1) Significant (p < 0.05) differences in the available nutrient contents were observed among soils from different forest types, indicating that variations in forest types contribute to differences in soil nutrient levels.
(2) Surface soil TN and AK contents, MBN, MBC and ACP enzyme activities were significantly (p < 0.05) higher in ligustrum × metasequoia mixed forests compared to the corresponding pure forests, and other nutrients remained at elevated levels, which were beneficial to soil nutrient accumulation and C sink capacity.
(3) Differences were observed in certain properties of the 0–20 cm and 20–40 cm soil layers across different forest types. Meanwhile, significant (p < 0.05) interaction effects were found between forest type and soil layer on soil NH4+–N, NO3––N, AP and AK contents. Thus, further investigation into the relationship between deep soil layers and forest type is warranted.
Acknowledgement: None.
Funding Statement: This study was funded by the Technology Innovation Special Fund of Jiangsu Province for Carbon Dioxide Emission Peaking and Carbon Neutrality (BE2022307) and the National Positioning Observation and Research Project of Forest Ecosystem in Changjiang River Delta, Supported by State Forestry and Grassland Administration of China (2023132077).
Author Contributions: Conceptualization and methodology, Haijun Sun; investigation and data curation, Zirui Chen and Jiale Liu; writing—original draft preparation: Zirui Chen and Haijun Sun; writing—review and editing, Haijun Sun; supervision and funding acquisition, Haijun Sun. All authors reviewed the results and approved the final version of the manuscript.
Availability of Data and Materials: The data that support the findings of this study are available from the corresponding authors upon reasonable request.
Ethics Approval: Not applicable.
Conflicts of Interest: The authors declared that they have no conflicts of interest to report regarding the present study.
References
1. Xia JB, Ren JY, Zhang SY, Wang YH, Fang Y. Forest and grass composite patterns improve the soil quality in the coastal saline–alkali land of the Yellow River Delta, China. Geoderma. 2019;349:25–35. doi:10.1016/j.geoderma.2019.04.032. [Google Scholar] [CrossRef]
2. Liao YWK, Zhang PY, Zhang YQ, Zhang YQ, Li XG. Advances in salt-tolerant mechanisms of trees and forestation techniques on saline-alkali land. Nanjing for Univ (Nat Sci Ed). 2022;46(6):96–104 (In Chinese). doi:10.12302/j.issn.1000-2006.202209010. [Google Scholar] [CrossRef]
3. Shao TY, Zhao JJ, Liu AH, Long XH, Rengel Z. Effects of soil physicochemical properties on microbial communities in different ecological niches in coastal area. Appl Soil Ecol. 2020;150:103486. doi:10.1016/j.apsoil.2019.103486. [Google Scholar] [CrossRef]
4. Ding JN, Li B, Sun ML, Li X. Different cropping patterns to restore saline-alkali soils in Northeast China affect the abundance of functional genes in the soil nitrogen cycle. Sustainability. 2023;15(8):6592. doi:10.3390/su15086592. [Google Scholar] [CrossRef]
5. Liu LP, Long XH, Shao HB, Liu ZP, Ya T, Zhou QS, et al. Ameliorants improve saline-alkaline soils on a large scale in northern Jiangsu Province. China Ecol Eng. 2015;81:328–34. doi:10.1016/j.ecoleng.2015.04.032. [Google Scholar] [CrossRef]
6. Li N, Shao TY, Zhou YJ, Cao HY, Hu HY, Sun QK, et al. Effects of planting Melia azedarach L. on soil properties and microbial community in saline-alkali soil. Land Degrad Dev. 2021;32(10):2951–61. doi:10.1002/ldr.3936. [Google Scholar] [CrossRef]
7. Wang L, Wen YX, Tong R, Zhang H, Chen H, Hu T, et al. Understanding responses of soil microbiome to the nitrogen and phosphorus addition in Metasequoia glyptostroboides plantations of different ages. Microb Ecol. 2021;84(2):1–15. doi:10.1007/s00248-021. [Google Scholar] [CrossRef]
8. Weand MP. Chinese privet (Ligustrum sinense Lour.) alters the timing of litterfall and nutrient quality of leaf litter inputs in invaded riparian forests. Biol Invasions. 2020;22(6). doi:10.1007/s10530-020-02335-0. [Google Scholar] [CrossRef]
9. Feng XH, An P, Li XG, Guo K, Yang C, Liu XJ. Spatiotemporal heterogeneity of soil water and salinity after establishment of dense-foliage Tamarix chinensis on coastal saline land. Ecol Eng. 2018;2017:104–13. doi:10.1016/j.ecoleng.2017.06.031. [Google Scholar] [CrossRef]
10. Jing CL, Xu ZC, Zou P, Tang Q, Li YQ, You XW, et al. Coastal halophytes alter properties and microbial community structure of the saline soils in the Yellow River Delta, China. Appl Soil Ecol. 2019;134:1–7. doi:10.1016/j.apsoil.2018.10.009. [Google Scholar] [CrossRef]
11. Bo FJ, Zhang YX, Chen HYH, Wang PG, Ren XM, Guo JP. The C:N:P Stoichiometry of planted and natural Larix Principis-Rupprechtii stands along altitudinal gradients on the loess plateau, China. Forests. 2020;11(4):363. doi:10.3390/f11040363. [Google Scholar] [CrossRef]
12. Berger WT, Untersteiner H, Toplitzer M, Neubauer C. Nutrient fluxes in pure and mixed stands of spruce (Picea abies) and beech (Fagus sylvatica). Plant Soil. 2009;322(1–2):317–42. doi:10.1007/s11104-009. [Google Scholar] [CrossRef]
13. Lu JZ, Scheu S. Response of soil microbial communities to mixed beech-conifer forests varies with site conditions. Soil Biol Biochem. 2021;155:108155. doi:10.1016/j.soilbio.2021.108155. [Google Scholar] [CrossRef]
14. Li WT, Liu QH, Xie LL, Yin CY. Interspecific plant-plant interactions increase the soil microbial network stability, shift keystone microbial taxa, and enhance their functions in mixed stands. Forest Ecol Manage. 2023;533:120851. doi:10.1016/j.foreco.2023.120851. [Google Scholar] [CrossRef]
15. Zheng MH, Huang J, Chen H, Wang H, Mo J. Responses of soil acid phosphatase and beta-glucosidase to nitrogen and phosphorus addition in two subtropical forests in southern China. Eur J Soil Biol. 2015;68:77–84. [Google Scholar]
16. Song R, Tong R, Zhang H, Wang GG, Wu TG, Yang XQ. Effects of long-term fertilization and stand age on root nutrient acquisition and leaf nutrient resorption of metasequoia glyptostroboide. Front Plant Sci. 2022;13:905358. doi:10.3389/fpls.2022.905358. [Google Scholar] [PubMed] [CrossRef]
17. Fu BJ, Chen LD, Ma KM, Zhou HF, Wang J. The relationships between land use and soil conditions in the hilly area of the loess plateau in northern Shaanxi, China. Catena. 2000;39(1):69–78. doi:10.1016/S0341-8162(99)00084-3. [Google Scholar] [CrossRef]
18. Chen LL, Deng Q, Yuan ZY, Mu XM, Kallenbach RL. Age-related C:N:P stoichiometry in two plantation forests in the loess plateau of China. Ecol Eng. 2018;120:14–22. doi:10.1016/j.ecoleng.2018.05.021. [Google Scholar] [CrossRef]
19. Su L, Du H, Zeng FP, Peng WX, Rizwan M, Núnez-Delgado A, et al. Soil and fine roots ecological stoichiometry in different vegetation restoration stages in a karst area, southwest China. J Environ Manage. 2019;252:109694. doi:10.1016/j.jenvman.2019.109694. [Google Scholar] [PubMed] [CrossRef]
20. Xiao ZR, Teng JQ, Qin JQ, Liang ZL, He J, Qin L. Differential responses of soil carbon, nitrogen and phosphorus eco-stoichiometric ratio to monoculture and mixed coniferous-broadleaved plantations. J West China Forest Sci. 2024;53(2):56–63. doi:10.16473/i.cnki.xblykx1972.2024.02.008. [Google Scholar] [CrossRef]
21. Han JJ, Yang SJ, Yao RJ, Chen XM. Effects of adjustment measure of water-salinity on soil salinization in the Coastal Area of North Jiangsu province. Soils. 2012;44(4):658–64. doi:10.13758/j.cnki.tr.2012.04.021. [Google Scholar] [CrossRef]
22. Vance ED, Brookes PC, Jenkinson DS. An extraction method for measuring soil microbial biomass C. Soil Biol Biochem. 1987;19.6:703–7. doi:10.1016/0038-0717(87)90052-6. [Google Scholar] [CrossRef]
23. Wu J, Joergensen RG, Pommerening B, Chaussod R, Brookes PC. Mea-surement of soil microbial biomass C by fumigation-extraction—an automated procedure. Soil Biol Biochem. 1990;22(8):1167–9. doi:10.1016/0038-0717(90)90046-3. [Google Scholar] [CrossRef]
24. Bao SD. Analysis of soil agrochemistry. Beijing: China Agriculture Press; 2000. p. 14–111. [Google Scholar]
25. Iost S, Landgraf D, Makeschin F. Chemical soil properties of reclaimed marsh soil from Zhejiang Province PR China. Geoderma. 2007;142(3–4):245–50. doi:10.1016/j.geoderma.2007.08.001. [Google Scholar] [CrossRef]
26. Vesterdal L, Clarke N, Sigurdsson BD, Gundersen P. Do tree species influence soil carbon stocks in temperate and boreal forests? For Ecol Manage. 2013;309:4–18. doi:10.1016/j.foreco.2013.01.017. [Google Scholar] [CrossRef]
27. Ladenburger CG, Hild AL, Kazmer DJ, Munn LC. Soil salinity patterns in Tamarix invasions in the Bighorn Basin, Wyoming, USA. J Arid Environ. 2006;65:111–28. doi:10.1016/j.jaridenv.2005.07.004. [Google Scholar] [CrossRef]
28. Yang HJ, Xia JB, Cui Q, Liu JT, Wei SC, Feng L, et al. Effects of different Tamarix chinensis–grass patterns on the soil quality of coastal saline soil in the Yellow River Delta, China. Sci Total Environ. 2021;772:145501. doi:10.1016/j.scitotenv.2021.145501. [Google Scholar] [PubMed] [CrossRef]
29. Georgea SJ, Harper RJ, Hobbs RJ, Tibbett M. A sustainable agricultural landscape for Australia: a review of interlacing carbon sequestration, biodiversity and salinity management in agroforestry systems. Agric Ecosyst Environ. 2012;163:28–36. doi:10.1016/j.agee.2012.06.022. [Google Scholar] [CrossRef]
30. Zhang JB, Müller C, Zhu TB, Cheng Y, Cai ZC. Heterotrophic nitrification is the predominant NO3– production mechanism in coniferous but not broad-leaf acid forest soil in subtropical China. Biol Fertil Soils. 2011;47(5):533–42. doi:10.1007/s00374-011. [Google Scholar] [CrossRef]
31. Rothe A, Binkley D. Nutritional interactions in mixed species forests: a synthesis. Can J For Res. 2001;31(11):1855–70. doi:10.1139/cjfr-31. [Google Scholar] [CrossRef]
32. Deng JF, Li JH, Deng G, Zhu HY, Zhang RH. Fractal scaling of particle-size distribution and associations with soil properties of Mongolian pine plantations in the Mu Us Desert, China. Sci Rep. 2017;7(1):6742–2. doi:10.1038/s41598-017. [Google Scholar] [CrossRef]
33. Liu XT, Li HK, Cao L, Zhang YR. Analysis on the heterogeneity of forest soil nutrients in Guangdong province of southern China. J Beijing Forest Univ. 2021;43(2):90–101. doi:10.12171/j.1000. [Google Scholar] [CrossRef]
34. Niu D, Wang SL, Ouyang ZY. Comparisons of carbon storages in Cunninghamia lanceolata and Michelia macclurei plantations during a 22-year period in southern China. J Environ Sci. 2009;21(6):801–5. doi:10.1016/S1001-0742(08)62344-x. [Google Scholar] [PubMed] [CrossRef]
35. Yang XJ, Wang HY, Liu L, Li X, Li WS. Soil fertility under four forest stand types in low hills of Eastern Jilin province. Bull Soil Water Conserv. 2013;33(4):142–8. doi:10.13961/j.cnki.stbctb.2013.04.059. [Google Scholar] [CrossRef]
36. Bowles TM, Acosta-Martínez V, Calderón F, Jackson LE. Soil enzyme activities, microbial communities, and carbon and nitrogen availability in organic agroecosystems across an intensively-managed agricultural landscape. Soil Biol Biochem. 2014;68:252–62. doi:10.1016/j.soilbio.2013.10.004. [Google Scholar] [CrossRef]
37. Wang M, Cui J, Liu HY, Xu XN. Characterization of soil microbial biomass carbon and nitrogen in four forest types of Shushan Urban Forest park. Forests. 2023;14(7):1498. doi:10.3390/f14071498. [Google Scholar] [CrossRef]
38. Jiang HL, Yuan CX, Wu QQ, Heděnec P, Zhao ZM, Yue K, et al. Effects of transforming multiple ecosystem types to tree plantations on soil microbial biomass carbon, nitrogen, phosphorus and their ratios in China. Appl Soil Ecol. 2024;193:105145. doi:10.1016/j.apsoil.2023.105145. [Google Scholar] [CrossRef]
39. Kooch Y, Sanji R, Tabari M. Increasing tree diversity enhances microbial and enzyme activities in temperate Iranian forests. Trees. 2018;32:809–22. doi:10.1007/s00468-018-1674-3. [Google Scholar] [CrossRef]
40. Brant JB, Myrold DD, Sulzman EW. Root controls on soil microbial community structure in forest soils. Oecologia. 2006 Jul;148:650–9. doi:10.1007/s00442-006-0402-7. [Google Scholar] [PubMed] [CrossRef]
41. Zhang W, Gao DX, Chen ZX, Li H, Deng J, Qiao WJ, et al. Substrate quality and soil environmental conditions predict litter decomposition and drive soil nutrient dynamics following afforestation on the Loess Plateau of China. Geoderma. 2018;325:152–61. doi:10.1016/j.geoderma.2018.03.027. [Google Scholar] [CrossRef]
42. Teng YM, Zhan JY, Liu W, Zhang F, Wang C, Dong SK. Larch or Mongolian pine? Effects of tree species on soil properties and microbial biomass with the consideration of afforestation time. Ecol Eng. 2020;158:106074. doi:10.1016/j.ecoleng.2020.106074. [Google Scholar] [CrossRef]
43. Da Silva DKA, de Oliveira Freitas N, de Souza RG, da Silva FSB, de Araujo ASF, Maia LC. Soil microbial biomass and activity under natural and regenerated forests and conventional sugarcane plantations in Brazil. Geoderma. 2012;189:257–61. doi:10.1016/j.geoderma.2012.06.014. [Google Scholar] [CrossRef]
44. Cao D, Shi FC, Koike T, Lu ZH, Sun JK. Halophyte plant communities affecting enzyme activity and microbes in saline soils of the Yellow River Delta in China. Clean–Soil, Air, Water. 2014;42(10):1433–40. doi:10.1002/clen.201300007. [Google Scholar] [CrossRef]
45. Dong QQ, Wang HY, Du X, Zou JH, Cui X, Zhao H. Characteristics of soil urease activity in typical stand types in low mountainous area of Northeast China. Chin J Appl Environ Biol. 2023;29(3):690–5 (In Chinese). doi:10.19675/j.cnki.1006-687x.2022.03050. [Google Scholar] [CrossRef]
46. Ding K, Zhang YT, Wang L, Ge SY, Zhang YM, Yang Q. Forest conversion from pure to mixed Cunninghamia lanceolata plantations enhances soil multifunctionality, stochastic processes, and stability of bacterial networks in subtropical southern China. Plant Soil. 2023;488(1–2):411–29. doi:10.1007/s11104-023. [Google Scholar] [CrossRef]
47. Kotroczó Z, Veres Z, Fekete I, Krakomperger Z, Tóth JA, Lajtha K, et al. Soil enzyme activity in response to long-term organic matter manipulation. Soil Biol Biochem. 2014;70:237–43. doi:10.1016/j.soilbio.2013.12.028. [Google Scholar] [CrossRef]
48. Navarro FJ, Perdomo G, Tejera P, Medina B, Machín F, Guillén RM, et al. The role of nitrate reductase in the regulation of the nitrate assimilation pathway in the yeast Hansenula polymorpha. FEMS Yeast Res. 2003;4(2):149–55. doi:10.1016/S1567-1356(03)00163-6. [Google Scholar] [PubMed] [CrossRef]
49. Vidal EA, Gutiérrez RA. A systems view of nitrogen nutrient and metabolite responses in Arabidopsis. Curr Opin Plant Biol. 2008;11(5):521–9. doi:10.1016/j.pbi.2008.07.003. [Google Scholar] [PubMed] [CrossRef]
50. Lei J, Du HL, Duan AG, Zhang JG. Effect of stand density and soil layer on soil nutrients of a 37-year-old Cunninghamia lanceolata plantation in Naxi, Sichuan province, China. Sustainability. 2019;11(19):5410. doi:10.3390/su11195410. [Google Scholar] [CrossRef]
Cite This Article
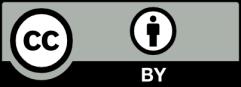
This work is licensed under a Creative Commons Attribution 4.0 International License , which permits unrestricted use, distribution, and reproduction in any medium, provided the original work is properly cited.