Open Access
ARTICLE
The Impact of Varied Fertilizers on the Yield and Quality of Panicum miliaceum under Low Nitrogen Conditions
1 Center for Agricultural Genetic Resources Research, Shanxi Agricultural University, Taiyuan, 030031, China
2 College of Agriculture, Shanxi Agricultural University, Jinzhong, 030801, China
3 Key Laboratory of Crop Genetic Resources and Germplasm Development in Loess Plateau, Ministry of Agriculture and Rural Affairs, Taiyuan, 030031, China
* Corresponding Authors: Zhijun Qiao. Email: ; Xiaoning Cao. Email:
(This article belongs to the Special Issue: Integrated Nutrient Management in Cereal Crops)
Phyton-International Journal of Experimental Botany 2024, 93(10), 2629-2644. https://doi.org/10.32604/phyton.2024.056157
Received 15 July 2024; Accepted 10 September 2024; Issue published 30 October 2024
Abstract
To clarify the response characteristics of broomcorn millet yield and quality to various fertilizers under low nitrogen conditions, the present study investigated the effects of different fertilization treatments, including no fertilization (HCK, CCK), urea (HF1, CF1), phosphate fertilizer (HF2, CF2), compound fertilizer (HF3, CF3), and organic fertilizer (HF4, CF4), on the agronomic traits, quality, and starch pasting properties of proso millet. As experimental materials, non-waxy proso millet (Hequ red proso millet, denoted as HQH) and waxy proso millet (Chishu 1, denoted as CS1) were utilized. The results showed that under low nitrogen conditions, urea treatment (HF1) significantly increased plant height and yield, and phosphate fertilizer treatment (HF2) significantly increased thousand-grain weight (TW). For waxy proso millet Chishu NO.1 (CS1), urea treatment (CF1) significantly increased yield, phosphate fertilizer treatment (CF2) significantly influenced the height of the plants, and compound fertilizer treatment (CF3) significantly increased thousand-grain weight. In terms of quality, phosphate fertilizer treatment (CF2) and organic fertilizer treatment (CF4) resulted in a substantial augmentation of both the total starch content and amylose content of waxy proso millet Chishu NO.1 (CS1), respectively, and organic fertilizer treatment (HF4, CF4) resulted in a notable enhancement of the protein content of proso millet. Organic fertilizer treatment (HF4) increased peak viscosity (PV), hot viscosity (HV), setback viscosity (SV), final viscosity (FV), breakdown viscosity (BV), pasting time (PTim), and pasting temperature (PTemp) of non-waxy proso millet. Phosphate fertilizer treatment (CF2) increased the peak viscosity (PV), hot viscosity (HV), breakdown viscosity (BV), final viscosity (FV), pasting time (PTim), and pasting temperature (PTemp) of waxy proso millet. Organic fertilizer treatment (HF4) and phosphate fertilizer treatment (CF2) under low nitrogen were the most effective in improving the comprehensive quality of non-waxy and waxy proso millet, respectively. The findings of our research elucidated the impacts of various fertilizers on the productivity and quality of non-waxy and waxy proso millet in nitrogen-deficient environments. Furthermore, this research offered a conceptual underpinning for attaining enhanced cultivation outcomes, encompassing both improved yield and quality, of proso millet in drought-prone regions.Keywords
Proso millet (Panicum miliaceum L.) originates from China and has a long planting history [1]. As a gluten-free food, numerous vitamins, minerals, and amino acids can be found in abundance in proso millet. At the same time, proso millet has the characteristics of drought tolerance, barren tolerance and short growth period [2], A common planting in arid and semi-arid regions, it plays a vital role in both the security of food and the sustainable growth of agriculture.
Nitrogen fertilizer can promote root growth [3], affect nutrient absorption and transport, prolong grain filling time, and increase crop yield [4], Meanwhile, the application of nitrogen fertilizer under low nitrogen conditions promoted the increase of protein content and amylose content of rice [5]. The increase in protein content directly affects the quality of crops. The change in protein content will affect the water absorption of starch to a certain extent, further regulate the combination of starch and water, and then influence the paste formation characteristics of starch [6]. Phosphorus fertilization enhances the activity of enzymes involved in nitrogen metabolism in root tissues, promotes root growth, increases rhizosphere soil nutrient absorption [7], and increases grain biomass by increasing sucrose content and sucrose synthase (SUS) activity [8]. At the same time, phosphate fertilizer can activate the high-affinity transporter of rice [9], thereby promoting starch degradation, which reduces the hardness of rice and increases its viscosity [10]. Compound fertilizer promotes soil nitrification, increases soil inorganic nitrogen content [11], provides abundant nutrients to increase protoplast composition accelerates cell division and elongation [12], and ultimately increases crop leaf area index and yield. The employment of a composite fertilizer led to an augmentation in both total starch content and amylose content but had no significant effect on pasting properties [13]. The utilization of organic fertilizer effectively ameliorates soil physical structure and augments the organic carbon content in the soil [14], promotes the increase of soil total nitrogen, and improves nitrogen utilization efficiency [15], so as to improve soil fertility. Jia et al. [16] observed that the utilization of organic fertilizer positively influences the starch content in wheat, elevating its PV, SV, and FV, thereby enhancing the quality of the wheat.
Proso millet is mainly planted in dry and thin areas, Soil nitrogen deficiency is one of the main problems in the production process of proso millet [17], Insufficient nitrogen supply will inhibit root growth. The synergistic effect of nitrogen (N), phosphorus (P), and potassium (K) fertilization can enhance the proliferation of roots, enhance the ability of roots to capture nitrogen, and promote the transport of nitrogen from roots to stems and grains [18], thereby increasing biomass and yield. At present, the research on low nitrogen in proso millet mainly focuses on resource evaluation [19], the physiological mechanism of low nitrogen tolerance [20], and so on. The underlying mechanisms governing the influence of various fertilizer types on the yield and quality of non-waxy and waxy proso millet under low nitrogen conditions are not clear.
The experimentation took place at the Hequ Research Facility at Shanxi Agricultural University, on 15 May 2020. The experimental plot did not apply nitrogen fertilizer for 4 consecutive years, and the previous crop was millet-maize rotation. The soil nutrients were: pH 8.51, organic matter 8.16 g/kg, total nitrogen 0.61 g/kg, available phosphorus 5.87 mg/kg, available potassium 97.07 mg/kg, and alkali-hydrolyzable nitrogen 53.59 mg/kg. The meteorological information about the experimental location is presented in Table 1. The average monthly precipitation during the growth period of proso millet is 60 mm, the average temperature is 18°C. The test cultivars were selected from the local production to promote the large area of non-waxy proso millet Hequhong proso millet (HQH) and waxy proso millet Chishu NO.1 (CS1). Hequ Red Millet is a local farmer-cultivated variety that has been planted for a long time in the region, and it covers a relatively large cultivation area. Chishu NO.1 is bred by the local red millet system. The experiment was conducted utilizing a randomized block methodology repeated 5 times, with row spacing of 45 cm, density of 300,000 plants/hm2, and plot area of 5 m × 6 m. Five distinct experimental conditions were set up for HQH, including HF1 with urea (150 kg/hm2), HF2 with phosphate fertilizer (120 kg/hm2), HF3 with compound fertilizer (375 kg/hm2), HF4 with farmyard manure (30,000 kg/hm2), and HCK as a control with no fertilizer. Similarly, five distinct experimental conditions were also established for CS1, namely CF1 with urea (150 kg/hm2), CF2 with phosphate fertilizer (120 kg/hm2), CF3 with compound fertilizer (375 kg/hm2), CF4 with farm manure (30,000 kg/hm2), and CCK as a control with no fertilizer. Compound fertilizer was ≥40% potassium phosphate nitrate (N-P2O5-K2O: 17-20-4), phosphate fertilizer (P2O5) was 12.5% calcium superphosphate, nitrogen fertilizer was urea (N: 46.4%), and farm manure was sheep manure (0.46% N, 0.29% P2O5, 0.24% K2O, 12.6% organic matter). Management practices were implemented by the locally specific technical regulations for cultivation.
2.2 Indicators and Methods of Determination
2.2.1 Determination of Agronomic Traits
After the plants matured, 15 samples of millet were selected from each treatment for the determination of agronomic traits. Following natural air drying, the plants were subject to cultivar evaluation. The height of the millet plant (cm) was quantified from the initial node of the elongated stem, extending up to the apical growth tip, using a ruler. The ear length (cm) was measured from the attachment point of the first spikelet to the top of the ear. The stem diameter (cm) at the second node from the base of the plant was determined using a vernier caliper. Upon maturity, the plants were manually harvested, excluding the two border rows. Each plot was harvested and threshed individually, and the yield was recorded after drying.
2.2.2 Determination of Nutritional Quality
The protein content was conducted in accordance with the procedure outlined by Li et al. [21]. After harvest, the grain was naturally air-dried, shelled, and ground into powder. Subsequently, The protein content in the millet was performed utilizing an automated Kjeldahl apparatus for nitrogen analysis.
For the determination of total starch content, an approach outlined by Zhuang et al. [22] was adopted, utilizing the Detection Kit provided by Solarbio Company (Beijing, China). After grinding 0.05 g of the sample, reagents were added and processed through multiple steps including water bath, centrifugation, and shaking. Ultimately, the absorbance was quantified at a wavelength of 620 nanometers, subsequently allowing for the computation of starch content through the utilization of a predefined standard curve.
The amylose content was determined using the iodine reagent method [23]. A volume of 10 milliliters of 0.5 molar potassium hydroxide solution (KOH) was administered to the starch specimen, and thoroughly mixed, Subsequently, 5 milliliters of 1 molar hydrochloric acid (HCl) and 0.5 milliliters of an iodine-based reagent were introduced into the mixture. The volume of the mixture was adjusted to 100 milliliters through dilution, Subsequently, Following this, the absorbance of the mixture was quantified at a wavelength of 620 nanometers using a spectrophotometer. A calibration curve was constructed, and the amylose content was calculated accordingly.
2.2.3 Determination of Pasting Properties
The pasting properties were determined according to the methodology outlined by Li et al. [24]. Specifically, 3.0 grams of the sample with a moisture content of 14% were precisely weighed and mixed with 25.0 milliliters of purified water. Following this, the mixture was introduced into the RVA-4500 Rapid Viscosity Analyzer, manufactured by Perten Instruments, Sweden, for testing. The program settings were as follows: maintaining at 50°C for 1 min, heating from 50°C to 95°C in 3.45 min, maintaining at 95°C for 2.4 min, cooling to 50°C in 3.45 min, and finally maintaining at 50°C for 1 min. The final results included peak viscosity, hot viscosity, breakdown viscosity, final viscosity, setback viscosity, pasting time, and pasting temperature.
Data were organized using Microsoft Excel 2021 software, and statistical analysis and graph plotting were performed using Origin Pro 2021 software. The Least Significant Difference (LSD) test was utilized for statistical analysis and for conducting multiple comparisons, with a significance level set at p < 0.05. All index data are presented as the mean ± standard error of three replicates.
3.1 The Impact of Varied Fertilizer Treatments on the Agronomic Traits
Distinct fertilizers exert notable influences on the agronomic characteristics of proso millet (Table 2). In contrast to the control group HCK, the plant heights of HQH under HF1, HF2, HF3, and HF4 treatments increased by 6.22%, 2.63%, 5.02%, and 5.74%, respectively, with HF1 treatment resulting in the highest plant height. Compared with HCK, HF2 treatment led to the thickest stem and the heaviest TW, increasing by 11.07% and 14.34%, respectively, while HF3 treatment resulted in the longest spike, increasing by 11.35%. In comparison with the control group CCK, the plant heights of CS1 under CF1, CF2, CF3, and CF4 treatments increased by 5.63%, 18.31%, 16.9%, and 17.6%, respectively, with CF2 treatment resulting in the highest plant height. Compared to the CCK treatment group, CF3 treatment led to the longest spike and the heaviest TW, increasing by 26.59% and 30.34%, respectively. In contrast to the CCK treatment group, CF4 treatment resulted in the thickest stem, increasing by 24.46%. There were significant differences in plant height, spike length, stem thickness, and TW between different varieties.
3.2 The Impact of Varied Fertilizer Treatments on the Yield
Different fertilizers can have significant effects on the yield of proso millet (Fig. 1). Compared with the control HCK, the yields of non-waxy proso millet HQH increased by 19.66%, 6.26%, 15.71%, and 11.08% under HF1, HF2, HF3, and HF4 treatments, with HF1 treatment resulting in the highest yield. In comparison with the control CCK, the yields of CS1 increased by 25.7%, 12.35%, 20.96%, and 17.13% under CF1, CF2, CF3, and CF4 treatments, with CF1 treatment achieving the highest yield.
Figure 1: Impact of varied fertilizer treatments on the yield. Different letters indicate significant differences between means (p < 0.05)
3.3 The Impact of Varied Fertilizer Treatments on the Quality
Different fertilizer treatments can significantly influence the quality attributes of proso millet, resulting in variations in amylose content, total starch content, and protein content (Fig. 2). Compared with the control HCK, the non-waxy proso millet HQH under HF1 treatment had the highest amylose content. The protein content of HQH treated with HF1, HF3, and HF4 increased significantly by 4.52%, 3.56%, and 5.17%, respectively, with HF4 treatment showing the highest protein content. In comparison with the control CCK, the total starch content of CS1 under CF1 and CF2 treatments increased significantly by 20.39% and 34.6%, respectively, with CF2 treatment exhibiting the highest total starch content. CF2, CF3, and CF4 treatments led to significant increases in amylose content by 7.93%, 8.62%, and 13.02%, respectively. CF1 and CF4 treatments also significantly increased protein content by 1.78% and 3.61%, respectively, with CF4 treatment showing the highest amylose and protein content.
Figure 2: Impact of varied fertilizer treatments on the nutritive value. (a) Amylose content of proso millet, (b) total starch content, (c) protein content. Different letters indicate that there is a significant difference between the mean ( p < 0.05), and the same letter indicates no significant difference
3.4 The Impact of Varied Fertilizer Treatments on the Starch Pasting Properties
Different fertilizer treatments can significantly influence the pasting properties of proso millet starch (Fig. 3). Compared to the control group HCK, under the HF1 treatment, the PV, HV, BV, FV, SV, PTim, and PTemp of the HQH starch all showed a downward trend. The HF2 treatment resulted in the highest BV. The HF3 treatment led to an extended PTim, while the HF4 treatment increased the RVA value of the starch. In contrast to the control group CCK, the CF1 treatment led to an upward trend in the HV, FV, SV, PTim, and PTemp of the CS1 starch. Under the CF2 treatment, all indicators except the SV showed an increase, with significant increases in PV and HV by 5.60% and 8.45%, respectively. The CF3 treatment increased the PTim and PTemp of the CS1 starch, while the CF4 treatment only improved the PTemp.
Figure 3: Impact of varied fertilizer treatments on RVA values. In the figures, distinct lettering denotes statistically significant variations among means, with a significance level of p < 0.05. (a) PV; (b) HV; (c)BV; (d) FV; (e) SV; (f) PTim; (g) PTemp
The comprehensive analysis of the agronomic and quality characteristics of diverse proso millet genotypes under low nitrogen conditions disclosed noteworthy patterns. Specifically, in HQH (Fig. 4a), a highly positive correlation was observed between HV and BV of starch, both being strongly associated with PV. Furthermore, SV exhibited a robust positive correlation with FV (r = 0.92), and PTim showed a significant positive relationship with both PV and HV. Additionally, PTemp displayed a substantial positive correlation with PTim (r = 0.71) and a notable positive correlation with HV. Conversely, stem diameter was negatively correlated with PTim and PTemp. Plant height was significantly positively correlated with yield (r = 0.7). Total starch content showed a positive correlation with FV and SV. Amylose content, on the other hand, had a negative correlation with PV and HV. Finally, protein content exhibited a highly positive correlation with both plant height and yield.
Figure 4: Correlation analysis of proso millet traits under different fertilizer treatments. (a) Correlation analysis of thetraits of HQH proso millet; (b) Correlation analysis of thetraits of CS1 proso millet; PV: peak viscosity; HV: hot viscosity; BV: breakdown viscosity; FV: final viscosity: SV: setback viscosity; Ptim: pasting time; Ptem: pasting temperature; PH: plant height; SL: spike length; SD: stem diameter; TW: thousand-grain weight; Yld: yield; TSC: total starch content; AC: amylose content; PC: protein content
In CS1 (Fig. 4b), the HV and BV of starch exhibited a robust positive correlation with PV. Additionally, FV showed a significant positive relationship with PV. PTim displayed a highly significant positive correlation with both PV and HV. Notably, PTemp had a strong positive correlation with PTim (r = 0.68) and a significant negative correlation with SV. Interestingly, plant height demonstrated a significant negative correlation with PV, HV, and PTim. On the other hand, stem diameter was significantly positively correlated with plant height (r = 0.85) and exhibited a notable negative correlation with PTim and PTemp. Furthermore, TW had a significant positive association with HV and a negative correlation with plant height, spike length, and stem diameter. Lastly, protein content showed a significant positive correlation with PTemp and a highly significant negative correlation with stem diameter (r = −0.7).
3.6 Principal Component Analysis
A principal component analysis (PCA) was conducted to evaluate the 15 measured traits., five primary components with eigenvalues exceeding the threshold of 1 were selected for further analysis. This approach ensures that the selected components are statistically significant and contribute meaningfully to the overall variance (Table 3). The contribution rates of the remaining components were relatively small and negligible. The contribution rates of these five principal components were 34.808%, 27.414%, 12.659%, 9.17%, and 6.902%. The selected five primary components collectively accounted for a cumulative contribution rate of 90.953%, satisfying the criterion that the cumulative contribution rate exceeds 90%. Through the analysis of these five principal components, the first principal component had a high loading on PV, the second principal component had a high loading on stem diameter, the third principal component had a high loading on plant height, spike length, HV, and PTim, the fourth principal component had a high loading on amylose content, TSC, and protein content, and the fifth principal component had a high loading on TW, yield, BV, FV, SV, and PTemp.
3.7 Fuzzy Membership Function Analysis and Comprehensive Evaluation
Based on the fuzzy membership function method, a comprehensive evaluation was conducted on 15 traits of proso millet (Table 4). The comprehensive evaluation values (D values) for different fertilizer treatments were calculated, ranging from 0.293 to 0.784. The fertilizer treatments were ranked according to their comprehensive evaluation values, from smallest to largest: CF4 < CF3 < HF1 < HF2 < CCK < HF3 < CF1 < HCK < HF4 < CF2. This indicates that under low nitrogen conditions, The utilization of organic fertilizer yielded the supreme comprehensive evaluation value for the HQH, while the application of phosphate fertilizer led to the highest comprehensive evaluation value for CS1.
Based on the clustering heatmap analysis of the 15 measured traits, the results show that: The upper clustering tree represents different fertilizer treatment groups. For HQH in Fig. 5a, the fertilizer treatments are divided into three groups: Group 1, represented by HF2, is characterized by lower plant height, protein content, yield, and pasting properties, but higher amylose content, stem diameter, spike length, TW, and BV. Group 2, represented by HCK, exhibits higher pasting property values, as well as good amylose content, total starch content, and stem diameter. Group 3, including HF1, HF3, and HF4, shows better performance in plant height, yield, and protein content. The left clustering tree, representing the quality traits of proso millet, can be grouped into three categories: Group 1 consists of amylose content and stem diameter; Group 2 comprises yield, protein, plant height, spike length, TW, and BV; and Group 3 includes TSC, SV, PTemp, FV, PTim, HV, and PV.
Figure 5: Heat map of cluster analysis of the impact of varied fertilizer treatments on various indicators. (a) Clustering of various indicators of HQH; (b) Clustering of each index of CS1
For CS1 in Fig. 5b, the fertilizer treatments are divided into three groups: Group 1, including CF3 and CF4, is characterized by lower stem diameter, total starch content, and pasting property values. Group 2, represented by CCK, has low nutritional component content. Group 3, comprising CF1 and CF2, exhibits higher total starch content, thousand-grain weight, and pasting property values. The left clustering tree of the quality traits of proso millet can be grouped into four categories: Group 1 consists of stem diameter, spike length, and plant height; Group 2 comprises protein content, amylose content, and PTemp; Group 3 includes yield, TW, and PTim; and Group 4 comprises SV, FV, BV, TSC, HV, and PV.
Nitrogen deficiency is one of the most significant issues in the production of proso millet [17], which can limit plant growth, nutrient accumulation, and yield formation. Our study found that under low nitrogen conditions, the HF1 and CF1 treatments significantly increased proso millet yield and reached a consistent conclusion with the research conducted by Sainju et al. [25], This may be because the application of nitrogen fertilizer promotes the activity of key enzymes such as urease, phosphatase, and invertase in the soil, which directly participate in the biochemical reactions of the soil, enabling nutrients to be directly absorbed by crops [26]. Furthermore, nitrogen fertilizer also provides sufficient nitrogen. Further promoting the richness and activity of soil microbial communities [27], contributing to the storage of more dry matter in leaves and delaying leaf senescence [28], increasing leaf area index, improving photosynthetic rate [29], promoting the efficient transport of photosynthetic products during grain formation [30], thereby enhancing the accumulation of dry matter [31], and ultimately increasing yield. The HF2 treatment was able to increase the stem diameter, TW, and yield of HQH, while the CF2 treatment increased the yield of CS1. This could potentially be attributed to the phosphate fertilizer application, which likely enhanced the crop’s carbon metabolism and subsequent carbohydrate production [32], enhanced photosynthetic phosphorylation and carbon assimilation processes [33], and also increased the expression levels of sucrose transporter genes GhSusA and GhSusB, thereby increasing sucrose content and promoting sucrose hydrolysis through elevating the activities of sucrose synthase (SUS) and sucrose-phosphate synthase (SPS) enzymes can be observed, providing sufficient nutrients [8], and ultimately increasing grain yield. The HF3 and CF3 treatments promoted the increase in proso millet yield, possibly because compound fertilizer can slowly release nutrients to meet plant growth needs, convert them into organic matter and energy through metabolism [34], increase root number and aboveground biomass [18], and ultimately promote yield increase. The HF4 and CF4 treatments increased the yield of proso millet, possibly because organic fertilizer can promote nutrient absorption by reducing mineral leaching [35], activate nutrients in the soil, improve soil physical and chemical properties [14], strengthen plant nutrient absorption, and thereby promote plant height growth and yield improvement [36].
The present study revealed that the HF1 treatment led to a notable elevation in the amylose concentration and protein content within the HQH cultivar. This phenomenon might be ascribed to the utilization of nitrogen-based fertilizer, which increases the sucrose content in grains, ensuring an adequate supply for source organs [37]. This treatment increased the expression of sucrose synthase(SUS), promoting the hydrolysis of sucrose into UDP-glucose, thereby promoting the synthesis of cell wall polysaccharides and starch [38]. Meanwhile, nitrogen also increased the activities of key enzymes such as SPS and NR in carbon and nitrogen metabolism in maize plants [39], regulating the hormonal harmony between IAA/GA3 and Z+ZR/GA3, Applying nitrogen fertilizer can up-regulate the gene encoding cytosolic isoenzyme 1-1 of glutamine synthetase, thereby enhancing nitrogen assimilation and utilization [40], ultimately promoting the biosynthesis of starch and protein in maize [5]. The CF2 treatment significantly increased the starch content of CS1, while the HF2 treatment boosted protein content. likely due to the phosphate fertilizer enhancing the expression levels of starch synthesis-related genes such as AGP1 and GBSS1, as well as the transcriptional abundance of the endosperm edge amylase gene [41], The GBSSI encoded by the Wx gene is not only responsible for the biosynthesis of amylose but also participates in the biosynthesis of long chains of amylopectin [42], thus resulting in an increase in grain starch content. Additionally, the application of phosphorus fertilizer optimizes root distribution while promoting nitrogen accumulation and translocation in plants [43]. It also enhances the activities of enzymes such as nitrate reductase, glutamine synthetase, endopeptidase, and carboxypeptidase, which in turn improves the nitrogen assimilation and redistribution capacity of leaves, ultimately increasing protein content [44]. The CF4 treatment promoted an increase in the amylose content and protein content of CS1, while also enhancing the protein content of HQH. This observation can be ascribed to the provision of ample organic substrate by the organic fertilizer, fostering microbial proliferation. This, in turn, enhances the overall microbial activity within the soil or plant system, establishing a stable rhizosphere microbial community [42]. This treatment enhanced urease activity and acid phosphatase activity, accelerating soil nitrogen cycling and phosphorus supply levels [45,46]. Consequently, it increased the chlorophyll content in leaves [35], contributing to the accumulation of photosynthetic products, and ultimately increasing starch and amylose content. Simultaneously, it improved nitrogen use efficiency, and the increased nitrogen in leaves induced the production of plant hormones IAA and indole-3-acetic acid [47], stimulating the photosynthetic process and enhancing protein content. Although the starch content under HCK treatment was higher than that of the treatment groups (HF1, HF2, HF3, HF4), the difference was not significant. This could be attributed to the fact that the average precipitation was relatively low, which affected the activity of starch phosphorylase [48]. Although starch phosphorylase is not the key enzyme in starch synthesis, it is related to the accumulation of dry matter in grains and grain development and can promote the conversion of glucose into starch [49], ultimately affecting the starch content.
The pasting properties of starch are an essential indicator for evaluating starch quality. This study has found that the HF1 treatment can reduce the RVA value of HQH. This reduction is likely attributed to the increase in glutelin content in the grains caused by nitrogen fertilizer, which subsequently enhances the formation of disulfide bonds in glutelin during the pasting process. This, in turn, further inhibits starch pasting and expansion [6], Additionally, the application of nitrogen fertilizer significantly increases the content of amylose. Crops with high amylose content tend to be more difficult to completely dissolve and expand during pasting [50], resulting in decreased pasting viscosity, longer PTim, and higher PTemp. in the end, resulting in a lower RVA value. Nitrogen fertilizer enhances the activity of enzymes such as soluble starch synthase and starch branching enzyme [51]. The ALK gene encodes soluble starch synthase II, and alterations in amino acids within this gene can potentially lead to changes in the activity of starch synthase, thereby influencing the synthesis of medium-length branched chains in amylopectin. This, in turn, modifies the crystalline layer structure and ultimately controls the gelatinization temperature of crops [52]. The CF2 treatment can lower the PTemp of CS1, likely because phosphate fertilizer facilitates the binding of phosphorus in the form of phosphate groups to starch molecules. The hydrophilicity of phosphate groups is greater than the hydroxyl groups in starch molecules, which favors rapid water absorption and expansion of starch, thus altering the viscosity of starch and leading to a certain degree of reduction in the PTemp [53]. Additionally, phosphorus fertilizer promotes starch degradation in rice grains, reducing rice hardness and increasing viscosity, thereby improving the eating quality of rice [10]. The HF3 treatment increased the RVA value of HQH, likely due to the application of compound fertilizer, which resulted in grains with lower water content and higher levels of nutrients such as protein, starch, and fiber, thus enhancing the PV and BV [54]. The HF4 treatment was able to increase the RVA value of HQH, likely due to the organic fertilizer regulating the retention and release process of nitrogen to modulate nitrogen supply [55], which in turn affects the branching crystallinity of amylopectin [56], The crystallinity of starch is closely related to the viscosity of the gelatinization properties of crops [57] and subsequently the RVA value of wheat. This study found that amylose content was negatively correlated with pasting viscosity, positively correlated with PTim and PTemp, while protein content was negatively correlated with pasting viscosity. This could be because amylose inhibits granule expansion [50], leading to a decrease in pasting viscosity and an increase in pasting time and pasting temperature. Therefore, appropriately reducing amylose and protein content is crucial for improving the palatability of proso millet, consistent with the research by Li et al. [24].
This study explored the impact of varied fertilizer treatments on the yield and quality of HQH and CS1 under low nitrogen conditions. The results indicated that urea significantly increased the yield, followed by compound fertilizer. Different fertilizers had varying effects on amylose, TSC, and protein content. Urea favored the accumulation of amylose in HQH, while organic fertilizer benefited the amylose content in CS1. Phosphorus fertilizer enhanced the total starch content of CS1. Compound fertilizer promoted protein synthesis in both varieties. Organic fertilizer improved the RVA value of HQH, whereas phosphorus increased the peak viscosity, hot paste viscosity, breakdown value, and final viscosity of CS1. Urea, on the other hand, improved the setback viscosity and pasting temperature of CS1. Based on the comprehensive evaluation value obtained from the membership function, organic fertilizer, and phosphorus fertilizer were found to have the highest comprehensive evaluation values for the yield and quality of HQH and CS1 under low nitrogen conditions. This study furnishes scientific evidence for the cultivation of proso millet that achieves both elevated yields and superior quality under low nitrogen conditions, thereby holding significant value in advancing organic dry farming techniques and theories.
Acknowledgement: None.
Funding Statement: This research was funded by the earmarked fund for CARS (CARS-06-14.5-A16), the earmarked fund for Modern Agro-Industry Technology Research System (2024CYJSTX03-23), the Shanxi Provincial Key Research and Development Program (Program No. 2022ZDYF110), the Central Guiding Local Science and Technology Development Funds (YDZJSX2022A044).
Author Contributions: The contribution of the authors to the paper is as follows: Mengyao Wang, Jiao Mao and Xiaoning Cao were responsible for the study’s conception and design; data collection was conducted by Yuanmeng Xu, Shu Wang, Yuhan Liu, Jiangling Ren, Zhijun Qiao and Sichen Liu; Mengyao Wang, Yuanmeng Xu and Shu Wang analyzed and interpreted the results; the draft manuscript was prepared by Mengyao Wang, Jiao Mao and Xiaoning Cao. All authors reviewed the results and approved the final version of the manuscript.
Availability of Data and Materials: For access to the data utilized in this research, readers are encouraged to reach out to the corresponding author.
Ethics Approval: Not applicable.
Conflicts of Interest: The authors declare that they have no conflicts of interest to report regarding the present study.
References
1. Wang RY, Hunt HV, Qiao ZJ, Wang L, Han YH. Diversity and cultivation of broomcorn millet (Panicum miliaceum L.) in China: a review. Econ Bot. 2016;70(3):332–42. doi:10.1007/s12231-016-9357-8. [Google Scholar] [CrossRef]
2. Diao XM. Production and genetic improvement of minor cereals in China. Crop J. 2017;5(2):103–14. doi:10.1016/j.cj.2016.06.004. [Google Scholar] [CrossRef]
3. Liu SJ, Yang XW, Ma G, Feng HX, Han ZD, Han XJ, et al. Effects of water and nitrogen application on root characteristics and nitrogen utilization in winter wheat. Acta Agron Sin. 2023;49(8):2296–307 (In Chinese). doi:10.3724/SP.J.1006.2023.21051. [Google Scholar] [CrossRef]
4. Li Q, Du LJ, Feng DJ, Ren Y, Li ZX, Kong FL, et al. Grain-filling characteristics and yield differences of maize cultivars with contrasting nitrogen efficiencies. Crop J. 2020;8(6):990–1001. doi:10.1016/j.cj.2020.04.001. [Google Scholar] [CrossRef]
5. Liu QL, Wang XL, Wu YW, Lan TQ, Liu F, Wei G, et al. Improved yield by optimizing carbon, nitrogen metabolism and hormone balance in apical kernels under low nitrogen conditions using the low nitrogen-tolerant maize variety. Field Crops Res. 2024;310:109358. doi:10.1016/j.fcr.2024.109358. [Google Scholar] [CrossRef]
6. Li LN, Shi SJ, Cheng B, Zhao D, Pan KQ, Cao CG, et al. Association between rice protein components and eating quality traits of different rice varieties under different nitrogen levels. J Cereal Sci. 2023;113:103760. doi:10.1016/j.jcs.2023.103760. [Google Scholar] [CrossRef]
7. Zhou QY, Tang JG, Liu CM, Huang KF, Huang XY. Effects of phosphate fertilizer application on the growth and yield of tartary buckwheat under low-nitrogen condition. Agron J. 2023;13:1886. doi:10.3390/agronomy13071886. [Google Scholar] [CrossRef]
8. Wang JW, Li HJ, Wang Q, Huang XL, Hu W, Wang SS, et al. Effects of phosphorus application on carbohydrate metabolism in cottonseed kernel during the key development period provided a new insight for phosphorus management in cotton production. Ind Crop Prod. 2023;191:115972. doi:10.1016/j.indcrop.2022.115972. [Google Scholar] [CrossRef]
9. Zhang WL, Yang QH, Xia MJ, Bai WM, Wang PK, Gao XL, et al. Effects of phosphate fertiliser on the physicochemical properties of Tartary buckwheat (Fagopyrum tataricum (L.) Gaertn.) starch. Food Chem. 2020;307:125543. doi:10.1016/j.foodchem.2019.125543. [Google Scholar] [PubMed] [CrossRef]
10. Ma ZH, Zhang WB, Cheng HT, Lu LJ, Song WW, Lv WY, et al. Regulating endosperm composition of rice through nutrient management for improved taste. Plant Nutr Fert Sci. 2022;28(1):45–57 (In Chinese). doi:10.11674/zwyf.2021283. [Google Scholar] [CrossRef]
11. Li N, Yang Y, Wu YJ, Liu BM, Tao LZ, Zhan Y, et al. Better performance of compound fertilizers than bulk-blend fertilizers on reducing ammonia emission and improving wheat productivity. Agr Ecosyst Environ. 2022;335:108018. doi:10.1016/j.agee.2022.108018. [Google Scholar] [CrossRef]
12. Arun S, Soumya TM, Hugar AY, Salimath SB. Effect of crop specific blended fertilizers on growth and yield of maize (Zea mays L.). Int J Environ Clim Change. 2023;13(12):357–66. doi:10.9734/ijecc/2023/v13i123691. [Google Scholar] [CrossRef]
13. Duan WX, Li GH, Zhang HY, Wang QM, Xie BT, Zhang LM. The impact of humic acid compound fertilizer on sweet potato variety Jishu 25: a comprehensive study on dry matter, root yield, and starch properties. SCI Horticamsterdam. 2024;327:112822. doi:10.1016/j.scienta.2023.112822. [Google Scholar] [CrossRef]
14. Dai ZS, Zhang Y, Wei YJ, Cai CF. Impacts of long-term organic manure inputs on cultivated soils with various degradation degrees. Soil Till Res. 2024;236:105950. doi:10.1016/j.still.2023.105950. [Google Scholar] [CrossRef]
15. Ning LYR, Xu XP, Zhang YT, Zhao SC, Qiu SJ, Ding WC, et al. Effects of chicken manure substitution for mineral nitrogen fertilizer on crop yield and soil fertility in a reduced nitrogen input regime of North-Central China. Front Plant Sci. 2022;13:1050179. doi:10.3389/fpls.2022.1050179. [Google Scholar] [PubMed] [CrossRef]
16. Jia J, Meng FG, Zhu ZX, Zhang YL, Liu S, Liu ST, et al. Combination of high organic and low nitrogen fertilizers improvesgrain nutrition and grinding quality of strong gluten wheat. Plant Nutr Fert Sci. 2024;30(1):190–98 (In Chinese). doi:10.11674/zwyf.2023300. [Google Scholar] [CrossRef]
17. Wang Y, Wang JS, Dong EW, Liu QX, Wang LG, Chen EY, et al. Foxtail millet [Setaria italica (L.) P. Beauv.] grown under nitrogen deficiency exhibits a lower folate contents. Front Nutr. 2023;10:1035739. doi:10.3389/fnut.2023.1035739. [Google Scholar] [PubMed] [CrossRef]
18. Duncan EG, O’Sullivan CA, Roper MM, Palta J, Whisson K, Peoples MK. Yield and nitrogen use efficiency of wheat increased with root length and biomass due to nitrogen, phosphorus, and potassium interactions. J Soil Sci Plant Nutr. 2018;181(3):364–73. doi:10.1002/jpln.201700376. [Google Scholar] [CrossRef]
19. Zhang MJ, Qiao ZJ, Yang WD, Feng MC, Xiao LJ, Wang G, et al. Biological response of different cultivars of millet to low nitrogen stress. Plant Nutr Fert Sci. 2014;20(3):661–69 (In Chinese). doi:10.11674/zwyf.2014.0318. [Google Scholar] [CrossRef]
20. Chen L, Wang JJ, Wang HG, Cao XN, Liu SC, Tian X, et al. Screening of broomcorn millet varieties tolerant to low nitrogen stress and the comprehensive evaluation of their agronomic traits. Sci Agric Sin. 2020;53(16):3214–25 (In Chinese). doi:10.3864/j.issn.0578-1752.2020.16.002. [Google Scholar] [CrossRef]
21. Li CF, Ji Y, Li EP, Gilbert RG. Interactions between leached amylose and protein affect the stickiness of cooked white rice. Food Hydrocoll. 2023;135:108215. doi:10.1016/j.foodhyd.2022.108215. [Google Scholar] [CrossRef]
22. Zhuang KY, Kong FY, Zhang S, Meng C, Yang MM, Liu ZB, et al. Whirly1 enhances tolerance to chilling stress in tomato via protection of photosystem II and regulation of starch degradation. New Phytol. 2018;221(4):1998–2012. doi:10.1111/nph.15532. [Google Scholar] [PubMed] [CrossRef]
23. Chen ZK, Chen HY, Jiang Y, Wang JP, Khan A, Li P, et al. Metabolomic analysis reveals metabolites and pathways involved in grain quality traits of high-quality rice cultivars under a dry cultivation system. Food Chem. 2020;326:126845. doi:10.1016/j.foodchem.2020.126845. [Google Scholar] [PubMed] [CrossRef]
24. Li DM, Wang HL, Gong Y, Cao ZW, Li JY, Feng BL. Effects of amylose and protein contents on visual appearance and eating quality of proso millet. Food Sci. 2023;44(4):115–21. doi:10.7506/spkx1002-6630-20220406-063. [Google Scholar] [CrossRef]
25. Sainju MU, Ghimire R, Pradhan G. Nitrogen fertilization I: impact on crop, soil, and environment. In: Rigobelo EC, Serra AP, editors. Nitrogen fixation. London, UK: IntechOpen; 2019. p. 1–19. doi:10.5772/intechopen.86028. [Google Scholar] [CrossRef]
26. Sun JB, Li WB, Li CQ, Chang WJ, Zhang SQ, Zeng YB, et al. Effect of different rates of nitrogen fertilization on crop yield, soil properties and leaf physiological attributes in banana under subtropical regions of China. Front Plant Sci. 2020;11:613760. doi:10.3389/fpls.2020.613760. [Google Scholar] [PubMed] [CrossRef]
27. Guo W, Zhou YP, Chen MQ, Li DD, Wang QX, Ma DH, et al. Effects of long-term different N application rates on aggregate distribution and fungal community composition in fluvo-aquic soil. Acta Pedologica Sinica. 2024;61(3):824–35 (In Chinese). doi:10.11766/trxb202210090556. [Google Scholar] [CrossRef]
28. Wang W, Li MY, Zhou R, Mo F, Khan A, Batool A, et al. Leaf senescence, nitrogen remobilization, and productivity of maize in two semiarid intercropping systems. Eur J Agron. 2023;150:126943. doi:10.1016/j.eja.2023.126943. [Google Scholar] [CrossRef]
29. Noor H, Yan ZZ, Sun PJ, Zhang LM, Ding PC, Li LH, et al. Effects of nitrogen on photosynthetic productivity and yield quality of wheat (Triticum aestivum L.). Agron J. 2023;13(6):1448. doi:10.3390/agronomy13061448. [Google Scholar] [CrossRef]
30. Muhammad Y, Li XK, Zhang Z, Ren T, Cong RH, Syed STA, et al. Nitrogen fertilizer management for enhancing crop productivity and nitrogen use efficiency in a rice-oilseed rape rotation system in China. Front Plant Sci. 2016;7:1496. doi:10.3389/fpls.2016.01496. [Google Scholar] [PubMed] [CrossRef]
31. Ma SY, Wang YY, Liu YA, Yao KJ, Huang ZL, Zhang WJ, et al. Effect of sowing date, planting density, and nitrogen application on dry matter accumulation, transfer, distribution, and yield of wheat. Chin J Eco-Agric. 2020;28(3):375–85 (In Chinese). doi:10.13930/j.cnki.cjea.190691. [Google Scholar] [CrossRef]
32. Fu GZ, Yan ML, Cai RG, Jia XL, Tian L, Cao HM, et al. Effects of application of phosphorus combined with nitrogen fertilizer on changes of grain protein components and characteristics of dough rheological in wheat. Sci Agric Sin. 2008;6:1640–48 (In Chinese). doi:10.3864/j.issn.0578-1752.2008.06.009. [Google Scholar] [CrossRef]
33. Wang F, Liu L, Wu YY, Li X, Sun ZG, Yin F, et al. Mechanism of maize intercropping peanut improving iron nutrition to increase photosynthetic performance of peanut. Plant Nutr Fert Sci. 2020;26(5):901–13. doi:10.11674/zwyf.19156. [Google Scholar] [CrossRef]
34. Sudding AF, Maintang, Asri M, Rauf AW, Syam A, Adriani WA. The effect of NPK 15-15-6-4 compound fertilizer on corn growth and yield. IOP Conf Ser: Earth Environ Sci. 2021;911(1):12047. doi:10.1088/1755-1315/911/1/012047. [Google Scholar] [CrossRef]
35. Muhammad NS, Wright DL, Hussain S, Koutroubas SD, Seepaul R, George S, et al. Organic fertilizer sources improve the yield and quality attributes of maize (Zea mays L.) hybrids by improving soil properties and nutrient uptake under drought stress. J King Saud Univ Sci. 2023;35(4):102570. doi:10.1016/j.jksus.2023.102570. [Google Scholar] [CrossRef]
36. Kamaei R, Faramarzi F, Parsa M, Jahan M. The effects of biological, chemical, and organic fertilizers application on root growth features and grain yield of Sorghum. J Plant Nutr. 2019;42(18):2221–33. doi:10.1080/01904167.2019.1648667. [Google Scholar] [CrossRef]
37. Xin L, Fu YY, Ma ST, Li CX, Wang HB, Gao Y, et al. Effects of post-anthesis irrigation on the activity of starch synthesis-related enzymes and wheat grain quality under different nitrogen conditions. Plants. 2023;12(24):4086. doi:10.3390/plants12244086. [Google Scholar] [PubMed] [CrossRef]
38. Wang HL, Zhang H, Liu JJ, Ma Q, Wu EG, Gao JF, et al. Transcriptome analysis reveals the mechanism of nitrogen fertilizers in starch synthesis and quality in waxy and non-waxy proso millet. Carbohyd Polym. 2024;323:121372. doi:10.1016/j.carbpol.2023.121372. [Google Scholar] [PubMed] [CrossRef]
39. Zhang ZQ, Hu YX, Tung SA, Yang L, Wang Y, Zhou XB. Evaluating the effects of water-nitrogen interactions on carbon and nitrogen accumulation as well as related metabolic enzymes activity in autumn maize. J Soil Sci Plant Nut. 2023;23(4):5245–56. doi:10.1007/s42729-023-01398-x. [Google Scholar] [CrossRef]
40. Yan WY, Qin JH, Jian YQ, Liu JG, Bian CS, Jin LP, et al. Analysis of potato physiological and molecular adaptation in response to different water and nitrogen combined regimes. Plants. 2023;12(8):1671. doi:10.3390/plants12081671. [Google Scholar] [PubMed] [CrossRef]
41. Zhang RQ, Li C, Fu KY, Li C, Li CY. Phosphorus alters starch morphology and gene expression related to starch biosynthesis and degradation in wheat grain. Front Plant Sci. 2017;8:2252. doi:10.3389/fpls.2017.02252. [Google Scholar] [PubMed] [CrossRef]
42. Wu HK, Wang SY, Wu M. The waxy gene has pleiotropic effects on hot water-soluble and-insoluble amylose contents in rice (Oryza sativa) grains. Int J Mol Sci. 2024;25:6561. doi:10.3390/ijms25126561. [Google Scholar] [PubMed] [CrossRef]
43. Chen XY, Liu P, Cheng Y, Dong ST, Zhang JW, Zhao B, et al. The root-layer regulation based on the depth of phosphate fertilizer application of summer maize improves soil nitrogen absorption and utilization. Acta Agron Sin. 2020;46(2):238–48 (In Chinese). doi:10.3724/SP.J.1006.2020.93029. [Google Scholar] [CrossRef]
44. Wang P, Yin YP, Fu GZ, Guo Y, Cai RG, Liang TB, et al. Effect of phosphorus on activities of enzymes related to nitrogen metabolism in flag leaves and protein content of wheat grains. J Plant Nutr. 2009;15(1):24–31 (In Chinese). doi:10.11674/zwyf.2009.0104. [Google Scholar] [CrossRef]
45. Enebe MC, Babalola OO. Effects of inorganic and organic treatments on the microbial community of maize rhizosphere by a shotgun metagenomics approach. Ann Microbiol. 2020;70(1):70–8. doi:10.1186/s13213-020-01591-8. [Google Scholar] [CrossRef]
46. Fan L, Chai RS, Liu LJ, Huang LD, Zhang YS. Effects of the rice straw-pig manure fermentation residues inoculated with microorganisms on improvement of continuous cropping soil in greenhouse. Plant Nutr Fert Sci. 2013;19(2):437–44 (In Chinese). doi:10.11674/zwyf.2013.0221. [Google Scholar] [CrossRef]
47. Cakmakci R, Erat M, Erdogan U, Donmez MF. The influence of plant growth-promoting rhizobacteria on growth and enzyme activities in wheat and spinach plants. J Plant Nutr Soil Sci. 2007;170:288–95. doi:10.1002/jpln.200625105. [Google Scholar] [CrossRef]
48. Chen N, Haung YF, Kong LB, Li N, Zhang CC, Ma HY. Study on fine agricultural climate zoning of high quality wheat in heze city based on GIS. Anhui Agric Sci Bull. 2018;24(6):134–6 (In Chinese). doi:10.16377/j.cnki.issn1007-7731.2018.06.052. [Google Scholar] [CrossRef]
49. Shen B, Chen N, Li TG, Luo YK. Effect of temperature on rice chalkiness formation and changes of materials in endosperm. Chin J Rice Sci. 1997;11(3):183–6 (In Chinese). doi:10.16819/j.1001-7216.1997.03.012. [Google Scholar] [CrossRef]
50. Li GT, Wang SA, Zhu F. Physicochemical properties of quinoa starch. Carbohydr Polym. 2016;137:328–38. doi:10.1016/j.carbpol.2015.10.064. [Google Scholar] [PubMed] [CrossRef]
51. Ban WH, Wang XQ, Liu QJ, Sun JB, Lv KY, Kang JH. Effects of nitrogen application rate on potato yield, starch content and related enzyme metabolic during tuber formation after high temperature stress. J Nucl Agric Sci. 2022;36(2):422–34 (In Chinese). doi:10.11869/j.issn.100-8551.2022.02.0422. [Google Scholar] [CrossRef]
52. Gao ZY, Zeng DL, Cui X, Zhou YH, Yan MX, Huang DN, et al. Map-based cloning and sequence analysis of rice gelatinization temperature control gene ALK. Sci Sin. 2003;33(6):481–7 (In Chinese). doi:10.1360/zc2003-33-6-481. [Google Scholar] [CrossRef]
53. Tang ZH, Li HM, Zhang AJ, Shi XM, Sun J, Zhu H, et al. Effects of long-term phosphorus fertilization on quality and starch RVA characters in sweetpotato. Plant Nutr Fert Sci. 2011;17(2):391–96 (In Chinese). doi:10.11674/zwyf.2011.0313. [Google Scholar] [CrossRef]
54. Lu DL, Wang X, Sun XL, Xu RC, Yan FB, Lu WP. Effects of basic fertilizer ratio and nitrogen top-dressing at jointing stage on pasting properties of waxy maize flour. Sci Agric Sin. 2013;46(5):909–16 (In Chinese). doi:10.3864/j.issn.0578-1752.2013.05.005. [Google Scholar] [CrossRef]
55. Zhou TY, Chen L, Wang WL, Xu YJ, Zhang WY, Zhang H, et al. Effects of application of rapeseed cake as organic fertilizer on rice quality at high yield level. J Sci Food Agric. 2022;102(5):1832–41. doi:10.1002/jsfa.11518. [Google Scholar] [PubMed] [CrossRef]
56. Cao XM, Sun HY, Wang CG, Ren XJ, Liu HF, Zhang ZJ. Effects of late-stage nitrogen fertilizer application on the starch structure and cooking quality of rice. J Sci Food Agric. 2018;98(6):2332–40. doi:10.1002/jsfa.8723. [Google Scholar] [PubMed] [CrossRef]
57. Jiang Y, Zhao C, Chen Y, Liu GM, Zhao LT, Liao PQ, et al. Effects of nitrogen panicle fertilizer application on physicochemical properties and fine structure of japonica rice starch and its relationship with eating quality. Acta Agron Sin. 2023;49(1):200–10 (In Chinese). doi:10.3724/SP.J.1006.2023.12083. [Google Scholar] [CrossRef]
Cite This Article
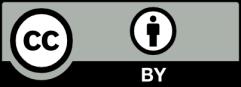
This work is licensed under a Creative Commons Attribution 4.0 International License , which permits unrestricted use, distribution, and reproduction in any medium, provided the original work is properly cited.