Open Access
ARTICLE
Enhanced Ultraviolet‑B Radiation Suppresses Magnaporthe oryzae Infection and Alleviates Its Damage to the Photosynthesis of Rice Leaves
1 College of Resources and Environment, Yunnan Agricultural University, Kunming, 650201, China
2 College of Horticulture and Landscape, Yunnan Agricultural University, Kunming, 650201, China
* Corresponding Authors: Zuran Li. Email: ; Yongmei He. Email:
(This article belongs to the Special Issue: Photosynthetic Responses to Biotic and Abiotic Stress)
Phyton-International Journal of Experimental Botany 2024, 93(10), 2613-2628. https://doi.org/10.32604/phyton.2024.056014
Received 12 July 2024; Accepted 11 September 2024; Issue published 30 October 2024
Abstract
In the present study, an indoor potting experiment was conducted to study the effects of enhanced UV-B radiation and Magnaporthe oryzae on the growth, stomatal structure, photosynthesis, and endogenous hormone contents of a traditional rice cultivar Baijiaolaojing in the Yuanyang terraces of Yunnan Province. In addition, the relationships between these parameters and disease indices were analyzed. We aimed to clarify the response of the photosynthetic physiology of rice under the combined stress of UV-B radiation and M. oryzae. Compared with the M. oryzae infection treatment, all the treatments, including M. oryzae infection before (MBR), simultaneously with (MSR), and after (MAR) UV-B radiation significantly increased the rice height and biomass by 4%–11% and 30%–111%, respectively, and the stomatal structure and carotenoids content of leaves, while decreasing the contents of chlorophyll a and b, by 21%–41% and 63%–73%, respectively. Both the MSR and MBR treatments significantly increased the photosynthetic rate and transpiration rate of rice leaves. The MAR treatment weakened chlorophyll fluorescence parameters, including the actual photosystem II (PS II) photochemical efficiency, electron transport rate, photochemical quenching, and nonphotochemical quenching by 40%, 39%, 43%, and 24%, respectively. Moreover, the treatments of MAR, MSR, and MBR decreased the phytohormones content and the M. oryzae disease index by 27%–62% in rice leaves. Thus, the enhanced UV-B radiation contributed to suppressing the M. oryzae infection and alleviating its damage to the photosynthesis of rice leaves. This study is valuable for the control of rice blast fungus and offers important insights into plant pathology.Keywords
Rice constitutes one of the most crucial food crops on a global scale, and the development and output of rice are impacted by multiple biotic and abiotic stresses, including ultraviolet B (UV-B) radiation and infection by Magnaporthe oryzae (M. oryzae). UV-B radiation directly damages biomolecules, such as DNA and proteins, and negatively affects the evolution and growth of plants [1]. Rice blast is caused by a pathogenic fungus and leads to symptoms such as leaf yellowing and a reduction in spike grain yield, thus affecting the yield and quality of rice [2,3]. In recent years, many studies have shown that the combined stress of UV-B radiation and M. oryzae has a complex influence on the development and growth of rice [4].
UV-B radiation has a wide range of biological effects on pathogenic bacteria [5], and UV-B radiation affects the production, survival, propagation, and invasive power of the conidia of pathogens. UV-B radiation can inhibit the germination of pathogen spores, change the size of pathogen colonies, and limit the propagation of spores, thus reducing the occurrence of disease [6]. For M. oryzae, increased UV-B radiation reduces the reproductive ability of the pathogen, slows the growth rate of mycelium, inhibits spore germination, leads to a decrease in spore production, intensifies the color of the pathogen colonies, and significantly reduces the invasive power of M. oryzae [7]. UV-B radiation can harm the DNA structure of pathogens in a straightforward manner, leading to damage to and mutation of genetic material [8], thus affecting the biological characteristics and pathogenicity of pathogens [9]. In addition, UV-B radiation can interfere with the cell wall synthesis and membrane lipid composition of pathogens, leading to cell membrane disruption and leakage, which in turn affects the physiological functions of pathogens [10].
Rice plants exhibit unique physiological responses to increased UV-B radiation and pathogenic bacterial stresses. Among them, methyl jasmonate (ME-JA) is a key factor in the acquisition of resistance in the plant system, and as an endogenous growth regulator in plants, it can stimulate the defense response or directly stimulate the manifestation of defense-related genes in the plant, produce a series of secondary metabolites with defense effects, and increase resistance to adversity [11,12]. Multiple hormone-mediated defense mechanisms play important roles in external stress, and these complex physiological processes may be interrelated and independent [13], constituting a complex hormonal regulatory network in rice itself.
Photosynthesis is one of the basic processes of plant growth, development, and production; it is an important way for plants to synthesize organic substances, and it is also one of the most important indices for determining the biological effects of UV-B [14]. The impact of enhanced UV-B radiation on photosynthetic physiology is characterized by damage to photosystem II (PSII), which causes a reduction electron transfer efficiency and photoinhibition, resulting in a decrease in chloroplast oxygen-excreting activity [15], blockage of photosynthetic pigment synthesis, and disruption of the chloroplast system [16]. Changes in photosynthetic parameters such as the net photosynthetic rate and stomatal conductance also occur [17]. The impaired activity of the key enzymes involved in photosynthesis in plants is another consequence [18]. Triggering the production of oxygen radicals in plants [19] leads to the oxidation of intracellular proteins and damage to the structure and function of photosynthesis-related proteins. And affect the normal growth and development of plants [20–23], resulting in plant stunting and reduced biomass. M. oryzae leads to disease symptoms such as spots and sores on rice leaves [24]. After infestation by M. oryzae, the leaf tissue structure is damaged by the destruction of leaf epidermal cells and chloroplasts. The spores and mycelia that form on the leaf surface severely block the stomata, impeding gas exchange and transpiration. This in turn affects leaf photosynthesis. Moreover, the toxins produced by M. oryzae during infestation cause damage to leaf vesicle membranes and disruption of reaction centers [25]. They also have toxic effects on chloroplasts and other photosynthetic organs, further inhibiting the photosynthetic activity of plants [4].
Research has shown that the physiological photosynthetic characteristics of rice exhibit a positive response to increased UV-B radiation or rice blast fungus stress. However, studies on the response of rice physiological photosynthetic characteristics to the combined stress of enhanced UV-B radiation and M. oryzae are limited to field experiments. Field conditions have many sources of external interference. Compared with field experiments, pot experiments can more easily control external interferences. To further clarify the effects of photosynthetic physiological properties of rice on UV-B radiation and rice blast fungus systems. Therefore, in the present study, we conducted pot experiments with traditional rice-Baijiaolaojing-old japonica from Yuanyang terraced fields and used a UV-B lamp radiation enhancement (5.0 kJ·m−2) treatments at different stages of rice leaf infestation by the rice blast fungus (before infestation, during infestation, and after succumbing to the disease) to select rice plants with the same growth and the same part of the plant to study the reactions of the physiological photosynthetic characteristics of rice to UV-B enhancement and the stress caused by M. oryzae. We propose the following hypotheses: 1. Increased UV-B radiation or M. oryzae infection alone had significant negative effects on growth, development and physiological photosynthetic characteristics in rice. 2. Increased UV-B radiation can inhibit M. oryzae infection to some extent. 3. The time of application of UV-B radiation can reduce the damage caused by M. oryzae infection to a certain extent, and the effect is most significant when UV-B radiation is applied before M. oryzae infection.
The rice variety employed in this experiment was the traditional rice variety of the Yuanyang terrace “Baijiaolaojing”, and plastic pots with an inner diameter of 33 cm were used for planting. A UV-B radiation meter was manufactured by Beijing Normal University Optoelectronic Instrument Factory, Beijing, China, and a 40 W UV-B lamp (wavelength 280–320 nm) was made by Shanghai Gucun Instrument Factory, Shanghai, China. Additionally, the rice blast strain (PEX22A-PCB1004) used for the test was supplied by the Yunnan Provincial Key Laboratory of Plant Pathology, Kunming, China and has stable pathogenicity. The soil utilized in the experiment was obtained from the experimental farm of Yunnan Agricultural University, Kunming, China, and the environmental background values of this soil are shown in Table 1.
The test rice seeds were sterilized by being soaked in a carbendazim solution that was diluted by a factor of 1000 for 20 min. The samples were subsequently placed in trays, overlaid with two layers of sterile water-moistened gauze, and maintained in an incubator at 28°C for 46 days. Once the seeds became dewy and white, they were directly sown in 20 pots, each containing 20 kilograms of soil. When the seedlings reached the three-leaf stage, 18 pots with uniform growth were selected for UV-B radiation and inoculation with M. oryzae.
When the seedlings turned green, the rice plants were exposed to increased UV-B radiation under natural light. This was achieved by employing 40 W UV-B lamps (with wavelengths spanning 280–320 nm) on each plot, facilitated by a freely pull-up lamp holder. This arrangement ensured that one row of rice was directly beneath the lamps. The irradiation intensity, particularly at the upper part of the plants, was regulated by adjusting the height of the lamps and assessed by a UV-B radiation tester (Beijing 720). The radiation intensity was set at natural light and augmented by 5.0 kJ·m−2. Throughout the UV-B radiation intensity treatments, with plants growth, the height of the lamps was constantly modulated to ensure that the plants received an equal intensity of UV-B radiation. And in the control group, no UV fluorescent lights were placed on top of the plants. For each treatment, three replicates were irradiated the UV lamp for the same amount of time each day, depending on weather conditions, except during rainy and cloudy day, until the determination of the rice morphological indices, including plant height, biomass, and disease indices.
The experimental design was divided into six different treatments: (1) illumination by natural light (CK), (2) enhanced UV-B radiation (R), (3) inoculation of M. oryzae (M), (4) three days before M. oryzae inoculation, enhanced UV-B radiation was supplied, and the indicators were measured at seven days (MBR), (5) concurrently treated with enhanced UV-B radiation and M. oryzae, and the indicators were measured at seven days (MSR), and (6) enhanced UV-B radiation was used three days after M. oryzae inoculation, and the indicators were measured on the seventh day (MAR).
The test strain was purified and grown on yeast media at 26°C for 7 days. A perforator was used to cut mycelial pieces with a diameter of 2 mm, which were then transferred to potato dextrose medium (PDA) and incubated for 8 to 10 days at 26°C under light (10 h·d−1). Microscopy was used to observe the spore production of M. oryzae. The content of the spore suspension was controlled at approximately 3 × 105 mL−1 through a hemocyte counting plate as a seedling spray inoculum. A total of 250 mL of inoculum solution of the same concentration of M. oryzae was added, and each rice leaf was allowed to adhere uniformly to the inoculum solution via a high-pressure water gun during spraying and infestation.
2.4.1 Plant Height and Biomass
Plant height: The distance from the tip of the tallest leaf to the surface of the soil was measured for 5 randomly selected plants in each pot.
Biomass: When harvest was complete, the entire pot of rice was extracted. The pot was delicately rinsed with tap water and subsequently allowed to dry naturally in a ventilated area without direct sunlight. The rice plants were inserted into kraft paper bags and dried at 80°C until a steady weight was obtained. While drying, the weight was measured every 24 h until a steady weight was achieved, and the mass of the dried plants was promptly weighed.
2.4.2 Photosynthetic Pigment Content and Photosynthetic Physiological Measurements in Rice Leaves
The photosynthetic pigments were extracted via the acetone method, and the absorbance values at 440, 630, 644, 662, 664, and 750 nm were determined via a UV spectrophotometer with 90% acetone solution as a reference. The photosynthetic pigment content was computed in accordance with the abovementioned formula.
The photosynthetic properties of the rice leaves were measured via the LCPRO portable intercellular CO2 concentration (Ci), stomatal conductance (Gs), photosynthesis rate (Tr), and photosynthetic rate (Pn).
The fluorescence characteristics were determined using the modulated chlorophyll fluorometer MINI-PAM-II. The measured indicators included the actual PSII photochemical efficiency, maximum quantum yield, potential photosynthetic activity, electron transport rate, photochemical quenching, and nonphotochemical quenching. After the leaves were incubated in the dark for 30 min, the light intensity was measured as 191 µmol·m−2·s−1.
With reference to the Standard Evaluation System for Rice, disease severity was assessed via a 9-level grading system. The disease index (DI) was calculated as follows: DI = [(number of leaves in each disease grade × numeric value representing the grade)/total number of leaves] × numeric value representing the severity of the disease grade × 100%.
Level 0: No disease spots.
Level 1: Only small brown disease spots.
At Level 2, the diameter of the brown spot ranged from 0.5–1 mm.
At Level 3, small, round or slightly elongated oval brown ring necrotic spots with a diameter of 1–2 mm were observed.
Level 4: Typical blast spots on rice that are oval in shape and measuring 1–2 cm in length.
Level 5: Typical rice blast spots with less than 10% of the affected area.
Level 6: Typical rice blast spot with 10%–25% of the affected area.
Level 7: Typical rice blast spot with 26%–50% of the affected area.
Level 8: Typical rice blast spot with 51%–75% of the affected area.
Level 9: All dead leaves.
2.4.4 Determination of Hormone Contents in Rice Leaves
Fresh rice leaves were weighed, ground in 80% methanol under low-temperature conditions, and centrifuged to collect the supernatant. The residue was supplemented with 80% methanol and concentrated to the aqueous phase at 40°C, with the pH adjusted to 2.5–3. The organic phases were combined and concentrated to dryness by adding 1–2 drops of ammonia and then further concentrated to dryness at 40°C through a rotary evaporator. A total of 0.5 mL of 0.1 mol·mL−1 glacial acetic acid was dissolved and filtered, and the filtered liquid was measured by a Shimadzu superior-efficiency liquid chromatograph featuring a diode array detector. The chromatographic conditions were as follows: C18 reversed-phase column, 38°C; detection wavelength, 254 nm; and injection volume, 10 μL. Phytohormones were qualitatively determined via comparison with standard samples of gibberellins (GA), auxin (IAA), abscisic acid (ABA), and methyl jasmonate (ME-JA) and quantified via the peak area external standard method.
2.5 Data Processing and Statistical Analysis
Using the SPSS 22.0 statistical software package (SPSS Inc., Chicago, IL, USA), the data from the different treatments were analyzed via least significant difference (Duncan) tests.
3.1 Effects of UV-B Radiation and M. oryzae Stress on the Growth of Rice Plants
As shown in Fig. 1, both the R and M treatments significantly reduced the rice plant height by 10% and 23%, respectively. However, the effects of UV-B radiation treatment on rice plant height vary depending on the timing of pathogen invasion. The MSR and MBR significantly influenced the rice plant height, with decreases of 14%, 16%, and 19%, respectively. Compared with the M treatment, the MAR, MSR, and MBR treatments significantly influenced the rice plant height, with increases of 12%, 9%, and 5%, respectively. Compared with the R treatment, the MAR, MSR, and MBR treatments significantly influenced the rice plant height, with decreases of 4%, 7%, and 10%, respectively.
Figure 1: Effect of M. oryzae and UV-B radiation at different times on plant height (A) and biomass (B). CK treatment, be illuminated by natural light; R treatment, enhanced UV-B radiation treatment; M treatment, the inoculating of M. oryzae; MAR treatment, Inoculated M. oryzae treatment three days after UV radiation.; MSR treatment, Enhanced UV-B radiation was provided concurrently with M. oryzae inoculating; MBR treatment, UV radiation three days before inoculating M. oryzae. Each value symbolizes the mean ± error (SE), n = 3. Different lowercase letters imply significant differences between treatments (p < 0.05)
The R treatment did not significantly affect the rice biomass, whereas the M treatment significantly affected the rice biomass, resulting in a significant influence of 53%. Treating rice with enhanced UV-B radiation at different stages of pathogen invasion had varying effects on rice biomass: MAR did not significantly reduce the rice biomass. However, both the MSR and the MBR decreased the rice biomass by 17% and 37%, respectively. Moreover, compared with the M treatment, the MAR, MSR, and MBR treatments significantly influenced the rice biomass, with decreases of 114%, 78%, and 34%, respectively.
3.2 Effects of UV-B Radiation and M. oryzae Stress on the Stomatal Structure of Rice Leaves
As shown in Table 2, in comparison with the CK treatment, both the R treatment and the fungus treatment significantly reduced the stomatal area of the rice leaves by 56% and 83%, respectively. Compared with the M treatment, the MAR, MSR, and MBR treatments significantly influenced the stomatal area of the rice leaves, with increases of 122%, 297%, and 156%, respectively. Compared with the R treatment, the MSR treatment had a significant influence on the stomatal area of the rice leaves, with an increase of 57%.
Compared with the CK treatment, the M treatment significantly influenced the stomatal density of the rice leaves, with a decrease of 64%. The R treatment did not significantly affect the stomatal density of the rice leaves. Compared with the M treatment, the MAR, MSR, and MBR treatments significantly influenced the stomatal density of the rice leaves, with increases of 205%, 162%, and 108%, respectively. Compared with the R treatment, the MBR treatment significantly influenced the stomatal density of the rice leaves, with a decrease of 30%.
Compared with the CK treatment, the R treatment resulted in a significant influence on the number of stomatal mastoids, with decreases of 38%. Compared with the M treatment, both the MAR and MSR significantly influenced the number of stomatal mastoids, with an increase of 25%. Compared with the R treatment, the MBR treatment had a significant influence on the number of stomatal mastoids, with a decrease of 27%.
3.3 Effects of UV-B Radiation and M. oryzae Stress on the Photosynthetic Pigments of Rice Leaves
As shown in Table 3, compared with the CK treatment, the M treatment significantly influenced the chlorophyll a and b contents of the rice leaves, with increases of 33% and 214%, respectively. M treatment also resulted in a significant 35% influence on carotenoids in rice leaves. The R treatment significantly influenced the chlorophyll b content of the rice leaves, with an increase of 12%, and significantly influenced the chlorophyll a and carotenoid contents of the rice leaves, with increases of 7% and 9%, respectively. Compared with the M treatment, the MAR treatment had a significant influence on the chlorophyll a and carotenoid contents of the rice leaves, with increases of 6% and 12%, respectively. MSR had a significant influence on chlorophyll a and b in rice leaves, with decreases of 22% and 16%, respectively, as well as a significant influence on carotenoids in rice leaves, with increases of 8%. MBR had a significant influence on the chlorophyll a and b and carotenoid contents of rice leaves, with increases of 6%, 17% and 14%, respectively.
3.4 Effects of UV-B Radiation and M. oryzae Stress on Photosynthetic Indicators in Rice Leaves
As shown in Table 4, the results were compared with those of the CK treatment. The R treatment resulted in a significant influence on the Pn of 170%, and the M treatment resulted in a significant influence on the Pn of 78%. Compared with the effects of the M treatment, the effects of the UV-B radiation treatment on the Pn during different periods of M. oryzae infestation were different. Compared with the M treatment, the MAR, MSR and MBR treatments significantly influenced the Pn, with increases of 65%, 155% and 672%, respectively.
Compared with the CK treatment, The R treatment resulted in a significant influence on Gs, with an increase of 28%. Treatment resulted in a significant influence on the Gs of 57%. Compared with that in the M treatment group, the amount of Gs in the MAR treatment group decreased significantly at different periods of M. oryzae infestation and resulted in the most significant influence on the Pn, with a decrease of 68%.
Compared with the CK treatment, UV-B and M treatments had a significant influence on Ci, with increases of 8% and 48%, respectively. Compared with the M treatment, the MAR and MSR treatments had significant influences on Ci, with decreases of 6% and 5%, respectively.
In comparison with the CK treatment, UV-B and M treatments resulted in a significant influence on the Tr, with decreases of 10% and 63%, respectively. Compared with the M treatment, the MBR and MSR significantly influenced the Tr, with increases of 28% and 31%, respectively.
3.5 Effects of UV-B Radiation and M. oryzae Stress on Chlorophyll Fluorescence Parameters in Rice
As shown in Fig. 2, in comparison with the CK treatment, the M treatment significantly influenced the actual PSII photochemical efficiency (ΦPSII), whereas the R treatment had no significant effect on ΦPSII. Compared with the M treatment. MBR, MSR and MAR significantly influenced ΦPSII, with decreases of 28%, 20% and 40%, respectively.
Figure 2: Effect of UV-B radiation and M. oryzae stress on chlorophyll fluorescence parameters in rice. The actual PSII photochemical efficiency (A), Maximum quantum yield (B), Potential photosynthetic activity (C), Electron transport rate (D), Photochemical quenching (E), Nonphotochemical quenching (F). CK treatment, be illuminated by natural light; R treatment, enhanced UV-B radiation treatment; M treatment, the inoculating of M. oryzae; MAR treatment, Inoculated M. oryzae treatment three days after UV radiation.; MSR treatment, Enhanced UV-B radiation was provided concurrently with M. oryzae inoculating; MBR treatment, UV radiation three days before inoculating M. oryzae. Each value symbolizes the mean ± error (SE), n = 3. Different lowercase letters imply significant differences between treatments (p < 0.05)
The maximum quantum yield (Fv/Fm) was significantly increased by UV-B radiation at different periods of M. oryzae infection, and compared with M. oryzae inoculation alone, the combined effect of M. oryzae and UV-B radiation resulted in a significant increase in the Fv/Fm. The R treatment significantly increased the maximum light energy conversion efficiency of the rice leaves. The trend in the potential photosynthetic activity (Fv/Fo) ratio was similar to that in the Fv/Fo ratio. The Fv/Fo ratio was significantly increased by UV-B radiation during different periods of M. oryzae wilt infestation.
In contrast to the CK treatment, the R treatments resulted in a significant influence on the electron transport rate (ETR), with decreases of 3%, and the M treatments resulted in a significant influence on the ETR, with increases of 24%. Compared with the M treatment, the MBR, MSR, and MAR treatments had significant influences on the ETR, with decreases of 25%, 19%, and 39%, respectively.
Compared with the CK treatment, the R treatment resulted in a significant influence on photochemical quenching (qP), with a decrease of 7%, and the M treatment resulted in a significant influence on qP, with an increase of 25%. Compared with the M treatment, the MBR and MAR significantly influenced the qP, with decreases of 43% and 29%, respectively.
Compared with the CK treatment, the R treatment had a significant effect on nonphotochemical quenching (NPQ), with a decrease of 5%, and the M treatment had a significant influence on NPQ, with an increase of 18%; however, compared with the M treatment, the MBR and MAR treatments had a significant influence on NPQ, with decreases of 16% and 24%, respectively.
3.6 Effects of UV-B Radiation and M. oryzae on Rice Leaf Phytohormones at Different Time Points
Fig. 3 shows the results of comparison with those of the CK treatment. The R treatment had a significant influence on ABA, GA, and ME-JA, with increases of 37%, 26%, and 31%, respectively, whereas the M treatment had a significant influence on ABA, GA, IAA, and ME-JA, with increases of 80%, 62%, 64%, and 105%, respectively. Compared with the M treatment, the MAR treatment had a significant influence on ABA, GA, IAA, and ME-JA, with decreases of 42%, 27%, 40%, and 41%, respectively. MSR significantly influenced ABA, GA, IAA and ME-JA, with decreases of 40%, 38%, 46%, and 62%, respectively; MBR significantly influenced ABA, GA, IAA, and ME-JA, with decreases of 29%, 26%, 19%, and 47%, respectively.
Figure 3: Effect of UV-B radiation and M. oryzae at different time points on ABA (A), GA (B), IAA (C), and ME-JA (D). CK treatment, be illuminated by natural light; R treatment, enhanced UV-B radiation treatment; M treatment, the inoculating of M. oryzae; MAR treatment, Inoculated M. oryzae treatment three days after UV radiation.; MSR treatment, Enhanced UV-B radiation was provided concurrently with M. oryzae inoculating; MBR treatment, UV radiation three days before inoculating M. oryzae. Each value symbolizes the mean ± error (SE), n = 3. Different lowercase letters imply significant differences between treatments (p < 0.05)
3.7 Effects of UV-B Radiation for Different Periods on the Disease Index of M. oryzae
As shown in Fig. 4, increased UV-B radiation significantly suppressed the occurrence of M. oryzae disease. Specifically, the inhibition of rice blast disease development on rice leaves was most pronounced when the rice plants were subjected to UV-B radiation before inoculation with M. oryzae. MBR, MSR, and MAR had inhibitory effects on rice blast disease of 27%, 40%, and 62%, respectively.
Figure 4: Effect of UV-B radiation on the disease index of rice blast. CK treatment, be illuminated by natural light; R treatment, enhanced UV-B radiation treatment; M treatment, the inoculating of M. oryzae; MAR treatment, Inoculated M. oryzae treatment three days after UV radiation.; MSR treatment, Enhanced UV-B radiation was provided concurrently with M. oryzae inoculating; MBR treatment, UV radiation three days before inoculating M. oryzae. Each value symbolizes the mean ± error (SE), n = 3. Different lowercase letters imply significant differences between treatments (p < 0.05)
As shown in Fig. 5, plant height was significantly positively correlated with leaf surface area, stomatal conductance, and transpiration rate but negatively correlated with the disease severity index. Biomass was significantly positively correlated with the leaf surface area, photosynthetic rate, and transpiration rate but negatively correlated with the disease severity index and chlorophyll a and b contents. These findings suggest that inoculation with M. oryzae and increased UV-B radiation may influence rice plant height and biomass by affecting the leaf surface area, photosynthetic rate, chlorophyll content, stomatal conductance, and transpiration rate.
Figure 5: Correlation analysis of UV-B radiation and inoculated M. oryzae on rice leaf parameter. *In (double side) significant correlation (p < 0.05); **In (double side) means highly significant related (p < 0.01)
4.1 Response of Rice Growth to Combined Stressors of Increased UV-B Radiation and M. oryzae
UV-B radiation and M. oryzae, as environmental and biological stressors, respectively, have an impact on rice growth and development [26]. Under R treatment, the morphological changes in the mycelium of M. oryzae result in a decrease in spore production, leading to a reduction in the rice disease index and alleviating the pathogenicity of M. oryzae [4]. UV-B radiation can cause damage to the cellular structure of M. oryzae. High-energy photons can disrupt the integrity of the fungal cell wall and membrane, leading to leakage of the cellular contents and ultimately inhibiting the growth and reproduction of the pathogen [27]. The results showed that UV-B radiation significantly reduced plant height and biomass due to the morphological adjustment of plants to form a compact structure to adapt to excessive UV-B radiation, which resulted in shorter rice plant phenotypes [28], a reduced leaf area, and reduced number of tillers, thereby increasing resistance to UV-B and leading to a reduction in rice biomass. In our research, we found that both UV-B radiation and inoculation with M. oryzae reduced rice plant height and biomass, and the degree of decline in rice biomass and plant height varied with radiation enhancement treatments at different stages of M. oryzae infestation of rice leaves (before infestation, during infestation, and after succumbing to disease), with the lowest decline occurring in the radiation enhancement treatments before infestation and the greatest decline occurring in the radiation enhancement treatments after susceptibility to disease. This occurred because, under UV-B radiation, the activity of the phenylalanine ammonia-lyase enzyme in rice increased, the flavonoid content increased, and stress tolerance increased; however, the phenylalanine ammonia lyase enzyme activity in rice decreased in response to inoculation with M. oryzae, and the flavonoid content decreased, decreasing stress tolerance [29–31]. The disease index was highly significantly and negatively correlated with plant biomass and the photosynthetic rate, likely because plants with higher disease indices lost more photosynthetic capacity, leading to lower rice biomass.
4.2 Response of the Photosynthetic Characteristics of Rice to Combined Stressors of Increased UV-B Radiation and M. oryzae
Leaves are plant organs that are sensitive to environmental stresses and respond to various environmental changes through changes in their morphology and structure [32]. Leaf photosynthetic characteristics directly or indirectly affect plant growth and development [33]. Increased UV-B radiation causes a decrease in rice leaf size, an increase in thickness, and an increase in the number of leaf surface epidermises [34]. Chlorophyll is the basis of plant photosynthesis, determines the strength of photosynthesis, and is closely related to plant growth and physiology [35]. The significant increase in the rice photosynthetic rate caused by UV-B radiation in this study occurred because the increase in UV-B radiation in this study was weakly stressful and stimulated the functioning of the photosynthetic structures, thus causing an increase in the photosynthetic rate of rice [36]. In this study, inoculation with M. oryzae led to an increase in rice chlorophyll content, which was highly significantly negatively correlated with rice plant height and biomass; this occurred because external stress leads to a short-term increase in chlorophyll synthesis in rice [37], which enhances photosynthesis and provides more energy and metabolites for the plant’s defense response. All treatments decreased the leaf transpiration rate, and the degree of decrease varied with UV-B radiation at different stages of disease susceptibility because M. oryzae obstructed the closure of rice stomata, and UV-B radiation changed the mycelial morphology of M. oryzae, which opened leaf stomata and led to an increase in the leaf transpiration rate. External stress can damage the photosynthetic system in chloroplasts, especially photosystem II, which affects the photosynthetic electron transport chain and reduces the production of ATP and NADPH [38], thereby affecting the photosynthetic characteristics of plant leaves.
4.3 Response of Leaf Endogenous Phytohormones in Rice to Combined Stressors of Increased UV-B Radiation and M. oryzae
Phytohormones are key signaling compounds that regulate processes such as plant growth, opening and closing of stomata, and chlorophyll synthesis and significantly affect leaf photosynthesis [39]. ABA and ME-JA interact with IAA and GA to regulate plant defense responses to pathogens and abiotic stresses [40]. The increase in hormone content in rice under all the treatments in this study was attributed to damage to the chloroplast membrane system due to external stress and the accumulation of ABA in the plant [41]. The synthesis of antioxidant substances and heat-excited proteins is induced to reduce the damage of reactive oxygen species to the plant body [42]. Pathogen infestation induces the upregulation of the expression of ME-JA biosynthetic genes [43]. ME-JA has important regulatory functions in plants. It promotes the synthesis of plant defense compounds, initiates the expression of genes involved in pathogenesis related to systemic acquired resistance and local resistance, and participates in systemic defense responses against pathogenic bacteria [44]. The synthesis of antimicrobial peptides and other disease-fighting substances, as well as the regulation of programmed death of plant cells against pathogenic bacterial infections, is recommended [45]. This regulatory mechanism allows plants to respond effectively to pathogen infection and enhances their resistance. IAA induces the rapid expression of genes implicated in the plant response to adverse stress in response to adverse conditions [46]. Multiple phytohormones act synergistically in a complex network structure to guide normal plant growth and increase plant resistance to stresses [47]. In this study, we found that MBR, MSR, and MAR resulted in increased phytohormone contents, with the smallest change occurring in the MAR treatment. This may be because UV-B radiation promotes the synthesis of polyphenolic compounds in plants, thereby increasing their stress tolerance [48]. Additionally, UV-B radiation significantly reduces the expression of pathogenic genes of M. oryzae [9], decreasing its pathogenicity and alleviating the toxicity of M. oryzae to rice, thus reducing the synthesis of phytohormones. Compared with the M treatments, the MBR, MSR, and MAR treatments resulted in lower levels of photosynthetic pigments and growth hormones, resulting in greater plant height and biomass. This may be related to the survival strategies of plants in the face of external stresses [49], where plants need to mobilize many resources to cope with adversity. Plants may allocate more resources to antiretroviral responses, resulting in higher levels of hormones in the plant, while allocating fewer resources to growth [50,51].
The increase in UV-B and M. oryzae infection impaired rice growth and physiological photosynthetic characteristics and reduced rice plant height and biomass. The increase in UV-B radiation contributed to the suppression of M. oryzae infection and alleviated its damage to the photosynthesis of rice leaves and was most effective when it was administered before infection. In conclusion, this study provides new insights into the response of plant photosynthetic physiology to biotic and abiotic stresses and offers new ideas for research in plant pathology, agriculture and other related fields.
Acknowledgement: None.
Funding Statement: This work was funded by the National Natural Science Foundation of China (32060287) and the Scientific Research Fundation Project of Yunnan Provincial Department of Science and Technology, Yunnan, China (202301BD070001-014).
Author Contributions: Qinghao Zhang, Zuran Li and Yongmei He designed the experiments. Qinghao Zhang wrote the manuscript. Yijie Yang, Jiong Wu and Hongru Li conducted the experiments. Yuan Li and Yongmei He provided the funding. All authors reviewed the results and approved the final version of the manuscript.
Availability of Data and Materials: All related information is available in this manuscript.
Ethics Approval: None.
Conflicts of Interest: The authors declare that they have no conflicts of interest to report regarding the present study.
References
1. Fu G, Shen ZX. Effects of enhanced UV-B radiation on plant physiology and growth on the Tibetan Plateau: a meta-analysis. Acta Physiol Plant. 2017;39:85. doi:10.1007/s11738-017-2387-8. [Google Scholar] [CrossRef]
2. Samalova M, Meyer AJ, Gurr SJ, Fricker MD. Robust anti-oxidant defences in the rice blast fungus Magnaporthe oryzae confer tolerance to the host oxidative burst. New Phytol. 2014;201(2):556–73. doi:10.1111/nph.2013.201.issue-2. [Google Scholar] [CrossRef]
3. Wilson RA, Talbot NJ. Under pressure: investigating the biology of plant infection by Magnaporthe oryzae. Nat Rev Microbiol. 2009;7(3):185–95. doi:10.1038/nrmicro2032. [Google Scholar] [PubMed] [CrossRef]
4. He YM, Li HR, Wu J, Li X, Zu YQ, Zhan FD, et al. Enhanced ultraviolet-B radiation alleviates structural damages on rice leaf caused by Magnaporthe oryzae infection. Protoplasma. 2024;261(1):161–71. doi:10.1007/s00709-023-01867-1. [Google Scholar] [PubMed] [CrossRef]
5. Zhao CJ, Gu HG, Xu JY, Li ZT. Effects of enhanced UV-B irradiation on the antioxidant enzymes and cell wall degrading enzymes of Rhizoctonia solani. Acta Phytophy Sin. 2021;51(4):549–58. [Google Scholar]
6. Xu XQ, Zuo J, Wan QQ, Cao RH, Xu HN, Li K, et al. Effective inactivation of fungal spores by the combined UV/PAA:Synergistic effect and mechanisms. J Hazard Mater. 2022;430:128515. doi:10.1016/j.jhazmat.2022.128515. [Google Scholar] [PubMed] [CrossRef]
7. Li X, Huang LL, He YM, Xie CM, Zhan FD, Zu YQ, et al. Effects of enhanced UV-B radiation on the interaction between rice and Magnaporthe oryzae in Yuanyang terrace. Photoch Photobio Sci. 2019;18:2965–76. doi:10.1039/c8pp00556g. [Google Scholar] [PubMed] [CrossRef]
8. Shafaei S, Klamerth N, Zhang YY, McPhedran K, Bolton JR, Ei-Din MG. Impact of environmental conditions on bacterial photoreactivation in wastewater effluents. Environ Sci: Proc IMP. 2017;19(1):31–7. [Google Scholar]
9. Huang LL, Mei XY, Li X, Zu YQ, He YM, Li Y. Effects of UV-B radiation on the expression of four pathogenic genes in the infection stage of Magnaporthe grisea. J Agro-Environ Sci. 2019;38(3):494–501 (In Chinese). [Google Scholar]
10. Gomes MC, Silva S, Faustino MAF, Neves MGPMS, Almeida A, Cavaleiro JAS, et al. Cationic galactoporphyrin photosensitisers against UV-B resistant bacteria: oxidation of lipids and proteins by 1O2. Photochem Photobiol Sci. 2013;12(2):262–71. doi:10.1039/c2pp25149c. [Google Scholar] [PubMed] [CrossRef]
11. Scheer JM, Ryan CJ. The systemin receptor SR160 from Lycopersicon peruvianum is a member of the LRR receptor kinase family. Proc Natl Acad Sci U S A. 2002;99(14):9585–90. doi:10.1073/pnas.132266499. [Google Scholar] [PubMed] [CrossRef]
12. Li CQ, Duan GH, Ma XQ, Qian LW, Ping T, Jing Y. Effects of exogenous jasmonic acids on defense response of resistant rice during the formation of brown necrotic spots caused by Magnaporthe oryzae infection. J Southern Agricult. 2020;51(5):1053–61 (In Chinese). [Google Scholar]
13. Zhi TT, Zhou Z, Han CY, Ren CM. PAD4 mutation accelerating programmed cell death in Arabidopsis thaliana tyrosine degradation deficient mutant sscd1. Chin Bull Bot. 2022;57(3):288–98 (In Chinese). [Google Scholar]
14. Allorent G, Lefebvre-Legendre L, Chappuis R, Kuntz M, Truong TB, Niyogi KK, et al. UV-B photoreceptor-mediated protection of the photosynthetic machinery in Chlamydomonas reinhardtii. Proc Natl Acad Sci U S A. 2016;113(51):14864–9. doi:10.1073/pnas.1607695114. [Google Scholar] [PubMed] [CrossRef]
15. El-Sheekh MM, Alwaleed EA, Ibrahim A, Saber H. Detrimental effect of UV-B radiation on growth, photosynthetic pigments, metabolites and ultrastructure of some cyanobacteria and freshwater chlorophyta. Int J Radiat. 2021;97(2):265–75. doi:10.1080/09553002.2021.1851060. [Google Scholar] [PubMed] [CrossRef]
16. Kreslavski VD, Huang X, Semenova G, Khudyakova A, Shirshikova A, Hummatov N, et al. Linking sensitivity of photosystem II to UV-B with chloroplast ultrastructure and UV-B absorbing pigments contents in A. thaliana L. phyAphyB double mutants. Plant Growth Regul. 2020;91:13–21. doi:10.1007/s10725-020-00584-6. [Google Scholar] [CrossRef]
17. Wu XJ, Chen BC, Xiao JP, Huachun G. Different doses of UV-B radiation affect pigmented potatoes’ growth and quality during the whole growth period. Front Plant Sci. 2023;14:1101172. doi:10.3389/fpls.2023.1101172. [Google Scholar] [PubMed] [CrossRef]
18. Reyes RT, Scartazza A, Castagna A, Cosio EG, Ranieri A, Guglielminetti L. Physiological effects of short acute UVB treatments in Chenopodium quinoa Willd. Sci Rep. 2018;8(1):371. doi:10.1038/s41598-017-18710-2. [Google Scholar] [PubMed] [CrossRef]
19. Zhang HY, Cheng KS, Gao X, Zhang XG, Liu T. Influence on antioxidants and alkaloid content of Phellodendron amurense seedlings grown under supplementary UV-B radiation. J Beijing For Univ. 2018;40(1):27–36 (In Chinese). [Google Scholar]
20. Vanhaelewyn L, Prinsen E, Straeten DVD, Vandenbussche F. Hormone-controlled UV-B responses in plants. J Exp Bot. 2016;67(15):4469–82. doi:10.1093/jxb/erw261. [Google Scholar] [PubMed] [CrossRef]
21. Arróniz-Crespo M, Núñez-Olivera E, Martínez-Abaigar J, Becker H, Scher J, Zapp J, et al. Physiological changes and UV protection in the aquatic liverwort Jungermannia exsertifolia subsp. cordifolia along an altitudinal gradient of UV-B radiation. Funct Plant Biol. 2006;33(11):1025–36. doi:10.1071/FP06096. [Google Scholar] [PubMed] [CrossRef]
22. Rusaczonek A, Czarnocka W, Willems P, Sujkowska-Rybkowska M, Breusegem FV, Karpiński S. Phototropin 1 and 2 influence photosynthesis, UV-C induced photooxidative stress responses, and cell death. Cells. 2021;10(2):200. doi:10.3390/cells10020200. [Google Scholar] [PubMed] [CrossRef]
23. Piccni C, Cai G, Disa MC, Romi R, Longo R, Cantini C. UV-B radiation affects photosynthesis-related processes of two Italian Olea europaea (L.) varieties differently. Plants. 2020;9(12):1712. doi:10.3390/plants9121712. [Google Scholar] [PubMed] [CrossRef]
24. Liang ML, Feng JQ, Wang WJ, Yang JY, Zhu XY, Su J. Baicalin affects the cell development of Magnaporthe oryzae and its control efficacy on rice blast. J Plant Prot Res. 2023;50(5):1176–83 (In Chinese). [Google Scholar]
25. Hu GY, Yang GT, Tang LQ, Zhang L, Ma K, Guo LA. Effect of Magnaporthe oryzae crude toxin on photosystemIIof hybrid rice. J Northwest A&F Univ. 2014;42(8):45–50 (In Chinese). [Google Scholar]
26. Pescheck F, Rosenqvist E, Bilger W. Plants and UV-B radiation. Physiol Plant. 2021;173(3):661–2. doi:10.1111/ppl.v173.3. [Google Scholar] [CrossRef]
27. Barrera A, Hereme R, Ruiz-Lara S, Larrondo LF, Gundel PE, Pollmann S, et al. Fungal endophytes enhance the photoprotective mechanisms and photochemical efficiency in the Antarctic Colobanthus quitensis (Kunth) Bartl. exposed to UV-B radiation. Front Ecol Ecol. 2020;8:122. doi:10.3389/fevo.2020.00122. [Google Scholar] [CrossRef]
28. He YM, Zhan FD, Gao ZH, Zu YQ, Li Y. Differences of UV-B radiation sensitivity of rice. Ecology Env Sci. 2012;21(3):489–95. [Google Scholar]
29. Liu XQ, Xie ZY, Xin JJ, Yuan SQ, Liu S, Sun YY, et al. OsbZIP18 is a positive regulator of phenylpropanoid and flavonoid biosynthesis under UV-B radiation in rice. Plants. 2024;13(4):498. doi:10.3390/plants13040498. [Google Scholar] [PubMed] [CrossRef]
30. Dong LY, Liu SF, Tian WK, Zhou WM, Zhang XW, Li XD, et al. Pathogenicity and mating type of rice blast fungus Magnaporthe oryzae isolatesin Yunnan Province. J Plant Dis Protect. 2023;50(2):316–24. [Google Scholar]
31. Yu W, Gong FS, Xu HW, Zhou XF. Molecular mechanism of exogenous ABA to enhance UV-B resistance in Rhododendron chrysanthum Pall. by modulating flavonoid accumulation. Int J Mol Sci. 2024;25(10):5248. doi:10.3390/ijms25105248. [Google Scholar] [PubMed] [CrossRef]
32. Liu WS, Zheng L, Qi DH. Variation in leaf traits at different altitudes reflects the adaptive strategy of plants to environmental changes. Ecol Evol. 2020;10(15):8166–75. doi:10.1002/ece3.v10.15. [Google Scholar] [CrossRef]
33. Miao YY, Zhang YR, Song B, Liu XT, Zhang AQ, Liu JZ, et al. Effects of Suaeda glauca rhizobacteria and endophytic bacterial strains on alfalfa growth under salt-alkaline stress. Acta Prataculturae Sinica. 2022;31(9):107–17 (In Chinese). [Google Scholar]
34. Wang D, Sun YC, Tu M, Zhang PP, Wang XQ, Wang TX, et al. Response of Zebrina pendula leaves to enhanced UV-B radiation. Funct Plant Biol. 2021;48(9):851–9. [Google Scholar] [PubMed]
35. Wang JQ, Zhang YF, Xue Y. Influence of ultraviolet radiation on physiological characteristics of Potamogeton crispus. Acta Ecologica Sinica. 2015;35(18):5975–83 (In Chinese). [Google Scholar]
36. Liu X, He JF, Xue M. Response of primary energy transfer process of photosystem II to low dose ultraviolet-B radiation studied with fluorescence dynamics technique. Acta Biochim Biophys Sin. 2011;27(10):839–48. [Google Scholar]
37. Sanchez-Zabala J, González-Murua C, Marino D. Mild ammonium stress increases chlorophyll content in Arabidopsis thaliana. Plant Signal Behav. 2015;10(3):e991596. doi:10.4161/15592324.2014.991596. [Google Scholar] [PubMed] [CrossRef]
38. Li C, Liu JT, Fan YX, Zhao XH, Xiao W, Chen XD, et al. Effects of UV-B on photosynthetic function and chloroplast ultrastructure of peach leaves grown in greenhouse. Chin Bull Bot. 2022;57(4):434–43 (In Chinese). [Google Scholar]
39. Waatd R, Seller CA, Hsu PK, Takahashi Y, Munemasa S, Schroeder JI. Plant hormone regulation of abiotic stress responses. Nat Rev Mol Cell Biol. 2022;23(10):680–94. doi:10.1038/s41580-022-00479-6. [Google Scholar] [PubMed] [CrossRef]
40. Verma V, Ravindran P, Kumar PP. Plant hormone-mediated regulation of stress responses. BMC Plant Biol. 2016;16(1):86. doi:10.1186/s12870-016-0771-y. [Google Scholar] [PubMed] [CrossRef]
41. Wang CL, Chen S, Dong YP, Ren RJ, Chen DF, Chen XW. Chloroplastic Os3BGlu6 contributes significantly to cellular ABA pools and impacts drought tolerance and photosynthesis in rice. New phytol. 2020;226(4):1042–54. doi:10.1111/nph.v226.4. [Google Scholar] [CrossRef]
42. Zhang XJ, Qiao QH, Yang XY, Lv ZW, Zhang WH. Response of growth and antioxidant properties of winter wheat seedlings to the UV-B stress and the regulation of exogenous ABA. Crop J. 2014;34(8):1098–103. [Google Scholar]
43. Liu F, Zhao Q, Jia ZH, Zhang SY, Wang J, Song SS, et al. N-3-oxo-octanoyl homoserine lactone primes plant resistance against necrotrophic pathogen Pectobacterium carotovorum by coordinating jasmonic acid and auxin-signaling pathways. Front Plant Sci. 2022;13:886268. doi:10.3389/fpls.2022.886268. [Google Scholar] [PubMed] [CrossRef]
44. Yu XX, Zhang WJ, Zhang Y, Zhang XJ, Lang DY, Zhang XH. The roles of methyl jasmonate to stress in plants. Funct Plant Biol. 2018;46(3):197–212. [Google Scholar]
45. Liu LJ, Sonbol FM, Huot B, Gu YN, Withers J, Mwimba M, et al. Salicylic acid receptors activate jasmonic acid signalling through anon-canonical pathway to promote effector-triggered immunity. Nat Commun. 2016;7:13099. doi:10.1038/ncomms13099. [Google Scholar] [PubMed] [CrossRef]
46. Hou XL, Lee LYCL, Xia KF, Yan YY, Yu H. DELLAs modulate jasmonate signaling via competitive binding to JAZs. Dev Cell. 2010;19(6):884–94. doi:10.1016/j.devcel.2010.10.024. [Google Scholar] [PubMed] [CrossRef]
47. Park J, Kim YS, Kim SG, Jung JH, Woo JC, Park CM. Integration of auxin and salt signals by the NAC transcription factor NTM2 during seed germination in Arabidopsis. Plant Physiol. 2011;156(2):537–49. doi:10.1104/pp.111.177071. [Google Scholar] [PubMed] [CrossRef]
48. Gong FH, Yu W, Zeng QP, Dong JW, Cao K, Xu HW, et al. Rhododendron chrysanthum’s primary metabolites are converted to phenolics more quickly when exposed to UV-B radiation. Biomolecules. 2023;13(2):1700. [Google Scholar] [PubMed]
49. Wilkinson SW, Magerøy MH, López, Sánchez A, Smith LM, Furci L, et al. Surviving in a hostile world: plant strategies to resist pests and diseases. Annu Rev Phytopathol. 2019;57(1):505–29. doi:10.1146/phyto.2019.57.issue-1. [Google Scholar] [CrossRef]
50. Golan G, Weiner J, Zhao YS, Schnurbusch T. Agroecological genetics of biomass allocation in wheat uncovers genotype interactions with canopy shade and plant size. New Phytol. 2024;242(1):107–20. doi:10.1111/nph.v242.1. [Google Scholar] [CrossRef]
51. Sparks EE, Rasmussen A. Trade-offs in plant responses to the environment. Plant Cell Environ. 2023;46(10):2943–5. doi:10.1111/pce.v46.10. [Google Scholar] [CrossRef]
Cite This Article
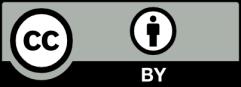
This work is licensed under a Creative Commons Attribution 4.0 International License , which permits unrestricted use, distribution, and reproduction in any medium, provided the original work is properly cited.