Open Access
ARTICLE
Comparative Analysis of Organic and Compound Fertilizers on the Yield and Metabolites of Platostoma palustre
1 Guangxi Key Laboratory of Medicinal Resources Protection and Genetic Improvement/Guangxi Engineering Research Center of TCM Resource Intelligent Creation, National Center for TCM Inheritance and Innovation, Guangxi Botanical Garden of Medicinal Plants, Nanning, 530023, China
2 College of Pharmacy, Guangxi Medical University, Nanning, 530021, China
3 National Engineering Research Center for Southwest Endangered Medicinal Materials Resources Development, Guangxi Botanical Garden of Medicinal Plants, Nanning, 530023, China
4 School of Traditional Chinese Medicine, China Pharmaceutical University, Nanjing, 210000, China
5 Apaxfon (Baotou) Biological Science and Technologies Co., Ltd., Baotou, 014100, China
* Corresponding Authors: Lijun Shi. Email: ; Danfeng Tang. Email:
(This article belongs to the Special Issue: Plant Secondary Metabolism and Functional Biology)
Phyton-International Journal of Experimental Botany 2024, 93(10), 2645-2662. https://doi.org/10.32604/phyton.2024.053492
Received 01 May 2024; Accepted 27 August 2024; Issue published 30 October 2024
Abstract
To explore the effect of fertilizers on the yield and quality of Platostoma palustre, in this study, P. palustre was utilized as the research material, and field experiments were conducted with different application rates of compound fertilizer and organic fertilizer and non-targeted metabolomics analysis was further employed to compare and analyze the differences in the metabolic components between the compound fertilizer and organic fertilizer treatments. The results of field experiments demonstrated that both compound and organic fertilizers could promote the fresh weight, shade dry weight, and dry weight of P. palustre, with 450 kg hm−2 compound fertilizer and 4500 kg hm−2 organic fertilizer presenting the optimum effects. Non-targeted metabolomics revealed that 1096 metabolites were identified in 450 kg hm−2 compound fertilizer and 4500 kg hm−2 organic fertilizer, and 885 metabolites were annotated in the Human Metabolome Database (HMDB). There were 318 differential metabolites (DMs) found between the two treatments, and 263 metabolites were annotated in HMDB. The abundance of 2 phenolic compounds and 12 organic oxygen compounds in the treatment of 4500 kg hm−2 organic fertilizer was significantly higher than that of the 450 kg hm−2 compound fertilizer, while the abundance of 21 organic oxygen compounds, 14 flavonoids, 3 phenolic compounds, and 5 cinnamic acids and their derivatives was significantly up-regulated in 450 kg hm−2 compound fertilizer treatment. In addition, 5 metabolic pathways were significantly enriched, and the flavone and flavonol biosynthesis was the most significantly differential metabolic pathway. These results suggested that the application of both compound fertilizers and organic fertilizers can increase the yield of P. palustre, but their effects on metabolites were different. This study has considerable implications for the planting and cultivation of P. palustre, furnishing a scientific foundation for an efficient and rational application of fertilizer.Keywords
Supplementary Material
Supplementary Material FilePlatostoma palustre (Blume) A. J. Paton (formerly known as Mesona chinensis Benth, also known as Xiancao in Chinese, Lamiaceae family) is one of the most important medicinal and edible plants. P. palustre contains various bioactive substances such as polysaccharides, amino acids, phenols, flavonoids, terpenes, etc., which are known to possess a variety of beneficial effects, like antioxidant, anti-inflammatory, hypolipidemic, hypotensive activity, and so on [1–6]. P. palustre is widely used to create a variety of cool and refreshing foods and beverages. According to incomplete statistics, in China, the domestic industry’s demand for P. palustre is high, with a yearly demand of more than 100,000 tons, yet the domestic production of the crop is inadequate, with an annual output of between 25,000 and 35,000 tons, necessitating imports from countries in Southeast Asia [7]. Therefore, improving the yield of P. palustre can relieve or meet domestic industry’s demand and improve economic benefits.
Fertilization is a critical factor in influencing the yield and quality of P. palustre. Appropriate utilization of fertilizer can significantly increase the yield of P. palustre [8]. Chemical fertilizers play an important role in agricultural production and are the main source of nutrients for plant growth [9]. Reasonable application of chemical fertilizers can increase plant yield (more than 20%) in a short period, increase soil available nutrients, and improve plant quality [10,11]. However, the unreasonable application of chemical fertilizers can lead to various of environmental problems, such as soil acidification and nutrient imbalance, decreased fertility, environmental pollution, etc. [12]. The Ministry of Agriculture and Rural Affairs of the People’s Republic of China has proposed the “Action Plan for Zero Growth in Fertilizer Usage”, which emphasizes the importance of replacing chemical fertilizer use with organic fertilizer as a viable response strategy [13]. Organic fertilizers are fortified with essential macronutrients such as potassium, nitrogen, phosphorus, and trace elements, as well as organic compounds, including amino acids and humic substances [14]. Studies have shown that the use of organic fertilizers minimizes adverse environmental impacts. Long-term application not only enhances soil nutrient content and improves soil physical and chemical properties but also enhances the nutritional value of crops [15]. Nevertheless, the total amount of organic fertilizer nutrients is relatively insufficient and decomposes slowly, which cannot meet the nutrient needs of crops in time. A single application of organic fertilizers will, to a certain extent, influence crop yield and quality [16]. Although fertilization can increase plant productivity, increased productivity may not necessarily improve quality. Mostly, with an increase in fertilizer application, the plant yield and quality improved. However, Yuan et al. [17] found that the seed yield of Kengyilia melanthera increased with increasing nitrogen fertilizer application, but the quality was not influenced by increasing nitrogen fertilizer input. P. palustre grows on the edge of the ditch and in the grass on dry sandy land, which is a plant that is extremely tolerant to poor soil [18]. In the production and planting process, the seedlings of P. palustre are mainly local domestic varieties. Till now, there are very few reports on its fertilizer-loving characteristics and fertilizer tolerance. Huang et al. [19] employed the “3414” experimental design to investigate the impact of nitrogen (N), phosphorus (P), and potassium (K) fertilizers on the yield and gum content of P. palustre. This involved 14 treatments at three factors and four levels of N, P, and K. The study findings revealed that the dependency of P. palustre on K, N, and P fertilizers was 30.09%, 27.98%, and 17.28%, respectively. The optimal fertilization regimen consisted of 468 kg hm−2 of N, 272.25 kg hm−2 of P, and 267.75 kg hm−2 of K fertilizers, resulting in a gel yield of 36.2%. Chen [20] demonstrated that P. palustre had a large demand for N, followed by K and P, with a ratio of 1:0.5:0.8 for N, P, and K. It can be seen that these reports focus on the application of compound fertilizers, therefore, in the context of ecological and environmental protection, the rational application of compound fertilizers and organic fertilizers for P. palustre is very necessary.
Metabolomics is the systematic analysis of metabolites present in a biological sample using high-throughput detection technologies, such as untargeted and targeted metabolomics [21]. Non-targeted metabolomics analysis presents a cost-effective and time-saving approach for examining a broad spectrum of metabolites in large sample sizes, gaining widespread adoption in plant science research [22,23]. Shi et al. [24] demonstrated that bioorganic fertilizer could alter soil metabolism and rhizosphere bacterial microbiota to improve soil environment and Panax notoginseng plant growth. Rao et al. [25] found that different microbial fertilizers could increase the yield of saffron (Crocus sativus L.) by regulating the accumulation of different metabolites. Applying metabolomics to analyze the relationship between fertilizers and plants is beneficial for providing a reference for practical production. However, research investigating the influence of varying application rates of organic and compound fertilizers on the yield, quality, and metabolic profiles of P. palustre remains unreported. Therefore, a hypothesis that both compound fertilizers and organic fertilizers could increase the yield, quality, and metabolites of P. palustre was proposed.
In the present study, the field experiment was performed, and the yield of P. palustre between different organic and compound fertilizer treatments was compared, and the differences in the quality and metabolites were also analyzed by using non-targeted metabolomics methods. By analyzing the effect of fertilizers on yield and metabolic differences, we sought to provide scientific evidence for the reasonable application of fertilizers in the planting and production of P. palustre.
2.1 Site Description and Experimental Design
The field experiment was conducted in Xixiangtang District, Nanning City, China, at a precise location of 22°50′48″N, 108°7′16″E, and an altitude of 80 m. The purplish soil in the study area exhibited an organic matter content of 22.57 g kg−1, an alkaline hydrolysis nitrogen content of 80.42 mg kg−1, an available phosphorus content of 15.70 mg kg−1, and an available potassium content of 149.50 mg kg−1, with a pH of 7.84. During planting, the mean temperature was from 24°C to 32°C, and the mean rainfall was 87.2 mm. The organic fertilizers were applied at the dosages of 2250, 4500, and 6750 kg hm−2, designated as YJF150, YJF300, and YJF450, respectively. The compound fertilizers were applied at the dosages of 300, 450, and 600 kg hm−2, designated as FHF20, FHF30, and FHF40, respectively. Each treatment was with three replicates, and the control group (CK) had no fertilization treatment (Fig. 1). The organic fertilizer was purchased from Yuantaifeng (Baotou) Biotechnology Co., Ltd., Baotou, China (N + P2O5 + K2O ≥ 4%, organic matter ≥ 30%). The compound fertilizer was bought from Stanley Agricultural Group Co., Ltd. (N + P2O5 + K2O ≥ 45%). On 22 April 2022, the P. palustre cutting seedlings purchased from a commercial company (Guangxi Runda Biotechnology Co., Ltd., Lingshan County, Qinzhou City, China) were transplanted into the field at a planting density of 45,000 plants per hectare. Throughout the growth period, routine field management practices were implemented.
Figure 1: (A) The morphology of P. palustre. (B) The schematic of the experimental treatments
The whole P. palustre plants were sampled (collected) on 20 June 2022 (about two months after transplanting), to estimate the yield and quality. For each treatment, we collected the data of the fresh weight, shade dry weight, and dry weight. Five plants were randomly collected per treatment. 5 fresh plants were randomly harvested and weighed to record their fresh weight. Randomly harvested 5 fresh plants were dried in the shade for 7 days, and then weighed their shade dry weight. 5 fresh plants were placed in an oven set at a temperature of 60°C until a constant weight was achieved to accurately measure and record their dry weight. The dried plants were crushed into powder with a mill for subsequent metabolome analysis.
Metabolite extraction was performed by combining 50 mg samples with a 6 mm diameter grinding bead, 400 μL of extraction solution (methanol:water = 4:1 (v:v)) that included 0.02 mg/mL of internal standard (L-2-chlorophenylalanine) in a 2 mL centrifuge tube. The sample solution was ground using a frozen meat grinder at a temperature of −10°C and a frequency of 50 Hz for 6 min, followed by extraction using cryogenic ultrasound at 5°C and 40 kHz for 30 min. Subsequently, the processed samples were stored at −20°C for 30 min and then centrifuged at 4°C and 13,000 g for 15 min to separate the fractions. The resulting supernatant obtained after centrifugation was carefully transferred to sample vials to undergo machine analysis.
We used a Q Exactive HF-X ultra-high-performance liquid chromatography-tandem Fourier transform mass spectrometry system, manufactured by Thermo Fisher (USA) for LC-MS analysis. LC-MS analysis was performed following the method of Liu et al. [26]. The 2 μL sample was separated by the HSS T3 chromatography column (100 mm × 2.1 mm i.d., 1.8 µm) and then subjected to mass spectrometry detection. Mobile phase A composed of a mixture of water and ethyl acetate in a ratio of 95:5 (v/v), while mobile phase B consisted of a mixture of ethyl acetate, isopropanol, and water in a ratio of 47.5:47.5:5 (v/v). Both mobile phases A and B were supplemented with 0.1% formic acid. The flow rate was set at 0.40 mL/min, while the column temperature was controlled at a temperature of 40°C.
The Thermo UHPLC-Q Exactive HF-X Mass Spectrometer, equipped with an electrospray ionization (ESI) source and operating in positive/negative ion scanning mode with a mass range of 70–1050 m/z, was utilized to analyze the samples. Mass spectrometry conditions were set as follows: the sheath gas and auxiliary gas flow rates were maintained at 50 and 13 psi, respectively, while the temperature of the auxiliary gas was heated to 425°C. The positive ion spray voltage and negative ion spray voltage were adjusted to 3500 and −3500 V, respectively. The auxiliary gas temperature and ion transfer tube temperature were 425°C and 325°C, respectively. The normalized collision energy was adjusted to 20, 40, and 60 V in a normalized manner during the experiment. The primary mass spectrum was obtained with a high resolution of 60,000, while the secondary mass spectrum had a lower resolution of 7500. Both spectra were acquired using a data-dependent acquisition (DDA) mode.
2.5 Data Preprocessing and Database Searching
The MS and MSMS spectra were matched against the Human Metabolome Database (HMDB, http://www.hmdb.ca/, accessed on 04 November 2022), Metlin (https://metlin.scripps.edu/, accessed on 04 November 2022), and Majorbio’s proprietary database to retrieve metabolite information. After querying the database, the data matrix is uploaded to the Majorbio Cloud Platform (https://cloud.majorbio.com, accessed on 04 November 2022) for subsequent data analysis.
We preprocessed the raw data and validated the overall data applicability by estimating the relative standard deviation (RSD) of a certain ion in the quality control (QC). The Spearman correlation between samples was calculated using the ‘cor’ function in the R package “ropls” (Version 1.6.2) and presented in a heat map. Furthermore, we utilized the R package “ropls” (Version 1.6.2) to perform Principal Component Analysis (PCA) and Orthogonal Partial Least Squares Discriminant Analysis (OPLS-DA). The model’s stability was evaluated by using a 7-fold cross-validation. The selection of DMs was determined according to the Variable Importance in the Projection (VIP) scores and p-values from the Student’s t-test within the OPLS-DA model. Metabolites with a VIP value greater than 1 (VIP > 1) and a p-value less than 0.05 (p < 0.05) were identified and designated as DMs. The significance of the difference was assessed using analysis of variance (ANOVA) with SPSS 8.0 software (version 22, IBM).
3.1 Influence of Various Fertilization Treatments on the Yield of P. palustre
It can be seen from Fig. 2 that the utilization of compound fertilizer and organic fertilizer could significantly promote the fresh weight, dry weight, and shade dry weight of P. palustre compared to the control (p < 0.05). Among the compound fertilizer treatments, the fresh weight, shade dry weight, and dry weight of P. palustre treated with 450 kg hm−2 compound fertilizer were the highest, which were 71.0%, 91.0%, and 61.5% higher than the control, respectively (Figs. 2A–2C). Among the organic fertilizer treatments, the fresh weight of P. palustre treated with 6750 kg hm−2 organic fertilizer presented the highest, while the highest shade dry weight and dry weight were found in the 4500 kg hm−2 treatment. Among the organic fertilizer treatments, the fresh weight, shade dry weight, and dry weight of P. palustre were no significant differences (p > 0.05) (Figs. 2D–2F). In summary, it could be concluded that the 450 kg hm−2 compound fertilizer and 4500 kg hm−2 organic fertilizer were the two optimal fertilization treatments. The fresh weight of P. palustre exhibited a statistically significant difference between the two treatments (p < 0.05), whereas no significant difference was observed in either the shade dry weight or the dry weight, as depicted in Fig. 3.
Figure 2: The effect of different levels of organic and compound fertilizers on the yield of P. palustre. (A–C) The influence of various compound fertilizer treatments on the fresh weight, shade dry weight, and dry weight of P. palustre. (D–F) The influence of various organic fertilizer treatments on the fresh weight, shade dry weight, and dry weight of P. palustre. Each histogram was the mean +/− standard error (SE) of n = 5. Different lowercase letters above histograms within each panel indicated significant differences (p < 0.05) among fertilizer doses within each fertilizer. Vertical bars above histograms indicated standard error
Figure 3: Comparative analysis of the optimal compound fertilizer and organic fertilizer treatments on the yield of P. palustre. Each histogram was the mean +/− standard error (SE) of n = 5. Different lowercase letters above histograms within each weight indicator indicated significant differences (p < 0.05) between the 450 kg hm−2 of compound fertilizer (FHF30) and 4500 kg hm−2 of organic fertilizer (YJF300) treatments. Vertical bars above histograms indicated standard error
3.2 Data Quality Analysis of Samples
To conduct a comparative analysis of the impact of organic fertilizer and compound fertilizer on the quality and metabolites of P. palustre, we performed untargeted metabolomics on the two selected treatments: 450 kg hm−2 compound fertilizer (FHF30) and 4500 kg hm−2 organic fertilizer (YJF300). The metabolomics analysis identified a total of 1096 metabolites, with 506 detected in negative ion mode and 590 in positive ion mode.
The RSD of the overall data was less than 30%, and data with a cumulative proportion of peaks greater than 70% were qualified and could be used for subsequent analysis (Fig. S1A). Correlation analysis revealed that the correlation coefficients were consistent within the FHF30 and YJF300 treatment groups, but varied between the groups. The correlation coefficients of the samples were approaching 1, suggesting a strong similarity in metabolite expression across samples, indicating a positive correlation (Fig. S1B).
PCA revealed that the two principal components PC1 (50.30%) and PC2 (8.96%) could well indicate the main characteristic information of the samples (Fig. S2A). Venn analysis showed 590 common metabolites in positive ion mode and 506 in negative ion mode between FHF30 and YJF300 (Fig. S2B). The PLS-DA intuitively revealed that the classification effect of the model was significant (Fig. S2C). Permutation testing uncovered that the original model demonstrated strong effectiveness and reliability. Additionally, the PLS-DA model exhibited excellent predictive ability without suffering from over-fitting issues (Fig. S2D).
After data processing, 885 metabolites were obtained and annotated into the HMDB, and these metabolites were matched and classified into 14 categories, including 352 lipids and lipid-like molecules, 119 organic acids and derivatives, 111 organoheterocyclic compounds, 108 organic oxygen compounds, 86 phenylpropanoids and polyketides, 66 benzenoids, 18 organic nitrogen compounds, 16 nucleosides, nucleotides and analogues, 3 hydrocarbons, 2 alkaloids and derivatives, 1 homogeneous non-metal compound, 1 hydrocarbon derivative, 1 lignans, neolignans and related compound, 1 organosulfur compound (Fig. S3).
OPLS-DA (Component 1 was 65.00% and Orthogonal Component 1 was 4.54%) could be better able to distinguish between groups (Fig. 4A). The validity of the OPLS-DA model was checked through the permutation tests (Fig. 4B). These results indicated that over-fitting phenomenon was not presented in the model. Based on the fold change of relative content ≥1 (up-regulation) or ≤1 (down-regulation), p-value <0.05, and VIP value ≥1, a total of 318 significant DMs were screened out. Among these, 161 DMs were detected in positive ion mode, with 74 up-regulated and 87 down-regulated, while 157 DMs were found in negative ion mode, comprising 101 up-regulated and 56 down-regulated DMs (Fig. 4C,D). HMDB compound classification revealed that a total of 263 DMs were identified and annotated into HMDB 4.0. These DMs were matched and classified into 9 categories, including 116 lipids and lipid-like molecules, 33 organic oxygen compounds, 32 organic acids and derivatives, 29 phenylpropanoids and polyketides, 24 orrganoheterocyclic compounds, 16 benzenoids, 6 organic nitrogen compounds, 6 nucleosides, nucleotides, and analogues, 1 homogeneous non-metal compounds (Fig. S4, Table S1). Among these significantly different metabolites, a total of 33, 14, 5, and 5 secondary metabolites were identified as organic oxygen compounds, flavonoids, phenolic compounds, and cinnamic acids and derivatives (Table 1), respectively, which were associated with essential active ingredients (polysaccharides, phenols, and flavonoids) of P. palustre.
Figure 4: Analysis of the differential metabolites between FHF30 and YJF300. (A) OPLS-DA scatter plot with large and small plots representing positive and negative ion modes, respectively. (B) Permutation test diagram showcasing 200 iterations for the positive ion mode. (C) Volcano map under positive ion mode. (D) Volcano map under negative ion mode
P. palustre is rich in polysaccharides, which are a very important quality evaluation indicator [48]. The polysaccharides of P. palustre contained 8 kinds of monosaccharides (glucose, galacturonic acid, ribose, rhamnose, glucuronic acid, galactose, arabinose, and xylose) [49]. In this study, we found 33 significantly differential organic oxygen compounds (12 up-regulated in YJF300 and 21 down-regulated in FHF30) (Fig. 5A). Among these, the abundance of 2-Deoxy-D-Ribose significantly increased in the YJF300 treatment relative to the FHF30 treatment (Fig. 5B). In addition, oligosaccharides refer to the compounds containing 2–10 monosaccharides polymerized by glycosidic bonds. Here, 4 kinds of differential oligosaccharides (galactose-beta-1,4-xylose, sucrose, raffinose, stachyose) and 1 polysaccharide (levan) were identified between the two treatments. The abundance of these five saccharides was significantly increased in the FHF30 treatment compared to the YJF300 treatment (Figs. 5C–5G).
Figure 5: The expression profile and VIP values of the 33 differential organic oxygen compounds and the abundance of the six saccharides between the YJF300 and FHF30 treatments. (A) The expression profile and VIP of the 33 differential organic oxygen compounds. (B–G) The abundance of the six saccharides. Note: ***indicates the statistical significance at a p-value of 0.001. Different shades of blue and red in Fig. 5A indicated the expression abundance and VIP values of the DMs
A total of 14 significantly differential flavonoids were detected in this study. As shown in Fig. 6A, the FHF30 treatment exhibited a higher abundance of the 14 differential flavonoids compared to the YJF300 treatment. Of these, quercetin, quercetin 3-O-glucoside, kaempferol, and rutin are the flavonoids that have been identified in P. palustre, and these four kinds of flavonoids had a significantly high abundance expression in the FHF30 treatment (Fig. 6B–E). This indicated that FHF30 treatment was a suitable treatment to improve the pharmacological effects of P. palustre.
Figure 6: The expression profile and VIP of the 14 differential flavonoids and the abundance of the four flavonoids. (A) The expression profile and VIP of the 14 differential flavonoids. (B–E) The abundance of the four flavonoids. Note: *** indicates statistical significance at a p-value of 0.001. Different shades of blue and red in Fig. 6A indicated the expression abundance and VIP values of the DMs
3.7 Phenolic Compounds and Cinnamic Acid and Its Derivatives
In the present study, 5 significantly differential phenolic compounds and 5 significantly differential cinnamic acids and their derivatives were detected between the FHF30 and YJF300 treatments. The abundance of [7]-Paradol, sinapyl alcohol, and isoeugenol in YJF300 treatment was higher than in FHF300 treatment, while the abundance of grevilline B and (5a,6a,8a,11a)-8-Hydroxy-2-oxo 1(10),3-guaiadien-12,6-olide-15-al 8-(4-hydroxyphenylacetate) in FHF300 treatment was higher (Fig. 7A). Meanwhile, in the FHF30 treatment, there was a higher abundance of five cinnamic acids and their derivatives compared to the YJF300 treatment (Fig. 7B). These two phenolic compounds sinapyl alcohol and isoeugenol were found to be a higher abundance in the YJF300 treatment (Fig. 7C,D). In addition, caffeic acid and ferulic acid are essential active ingredients of P. palustre, and the abundance of these two compounds in FHF30 was significantly higher (Fig. 7E,F).
Figure 7: The expression profile and VIP of the phenolic compounds and cinnamic acid and its derivatives and the abundance of two phenolic compounds and two cinnamic acid and its derivatives. (A) The expression profile and VIP of the 5 differential phenolic compounds. (B) The expression profile and VIP of the 5 differential cinnamic acid and its derivatives. (C and D) The abundance of Sinapyl alcohol and isoeugenol, respectively. (E and F) The abundance of caffeic acid and ferulic acid, respectively. Note: *** indicates statistical significance at a p-value of 0.001. Different shades of blue and red in Fig. 7A,B indicated the expression abundance and VIP values of the DMs
3.8 Metabolic Pathway Analysis for the Key DMs
Based on the selected 33 organic oxygen compounds, 14 flavonoids, 5 phenolic compounds, and 5 cinnamic acids and derivatives, there were 5 significantly enriched metabolic pathways, including flavone and flavonol biosynthesis, flavonoid biosynthesis, galactose metabolism, starch and sucrose metabolism, and phenylpropanoid biosynthesis (Fig. 8A). In addition, a metabolic map was developed based on these five metabolic pathways (Fig. 8B), in which a total of 14 DMs were involved, including 6 organic oxygen compounds (dulcitol, raffinose, sucrose, levan, stachyose, and chlorogenic acid), 4 flavonoids (kaempferol, quercetin, quercetin 3-O-glucoside, and rutin), 2 phenolic compounds (sinapyl alcohol and isoeugenol), and 2 cinnamic acids and derivatives (caffaric acid and ferulic acid). In particular, flavone and flavonol biosynthesis emerged as the most significantly differential metabolic pathway, which was associated with compounds such as quercetin, quercetin 3-O-glucoside, kaempferol, and rutin.
Figure 8: The KEGG topology analysis and metabolic map based on five metabolic pathways. (A) The KEGG topology analysis. (B) The metabolic map based on five metabolic pathways. Metabolisms in orange, gray, green, blue, and yellow were involved in galactose metabolism, starch, and sucrose metabolism, phenylpropanoid biosynthesis, flavonoid biosynthesis, and flavone and flavonol biosynthesis, respectively. Red metabolites mean higher abundance in the YJF300 treatment and blue metabolites mean higher abundance in the FHF30 treatment
Fertilization is a very important part of the cultivation and management of P. palustre. In this study, the results demonstrated that both organic and compound fertilizers could dramatically improve the yield of P. palustre (Fig. 2). However, excessively low fertilization rates could not significantly achieve the goal of increasing yield. Similarly, excessively high fertilization rates not only increased soil pressure but also violated economic considerations. Therefore, it was recommended to use a reasonable amount of fertilizer during the planting process of P. palustre. In addition, as mentioned above, both compound fertilizers and organic fertilizers could increase the yield of P. palustre, but there were significant differences in the yield between the two fertilizer types (Fig. 3). Thus, the selection and use of different fertilizer types also had a crucial effect on the P. palustre yield.
The primary active components extracted from P. palustre include flavonoids, polysaccharides, phenols, etc., all of which exhibit antioxidant effects [2,5]. Among these, the polysaccharide is the highest and most versatile active ingredient in P. palustre, accounting for about 20%, and their compositions are relatively complex and heteroglycans with antioxidant, immunomodulatory, antitumor, and intestinal microbiota-regulating effects [4,6,48,50,51]. In the present study, the YJF300 treatment significantly increased the monosaccharide content of P. palustre, while the FHF30 treatment increased the content of oligosaccharides and polysaccharides (Fig. 5). It was indicated that the application of different types of fertilizers could potentially alter the biological activity of polysaccharides in P. palustre.
P. palustre is also rich in flavonoids, accounting for 5.47%–6.21% [52]. P. palustre flavonoids, mainly including kaempferol, quercetin, isoquercetin, rutin, and scutalpin [52,53] have strong scavenging ability against hydroxyl radicals and 1,1-diphenyl-2-picrylhydrazine (DPPH·) [52]. Among these, quercetin is the main flavonoid component with bioactive functions of anti-cancer, anti-oxidation, anti-inflammatory, and antibacterial effects [42,52,54,55]. Isoquercetin reduces hyperlipidemia and inflammation by generating free radicals and regulating antioxidant genes [40,56]. Kaempferol possesses antioxidant, antiviral, and antiallergic effects [43,57,58]. Rutin improves antioxidant activity, increases insulin sensitivity, and lowers blood sugar and blood lipids [41]. In our study, the abundance of the 14 flavonoid differential metabolites, including the most important flavonoid substances in P. palustre (quercetin, isoquercetin, kaempferol, and rutin) were significantly up-regulated in the 450 kg hm−2 compound fertilizer treatment (Fig. 6). These suggested that the compound fertilizers could significantly improve the level of flavonoids in P. palustre and have the potential to enhance the bioactivity of flavonoids in P. palustre. In addition, flavone and flavonol biosynthesis emerged as the most notable differential metabolic pathway, which was associated with quercetin, isoquercetin, kaempferol, and rutin (Fig. 8). This also confirmed that the 450 kg hm−2 compound fertilizer treatment could promote the accumulation of the metabolites in the flavone and flavonol biosynthesis pathway.
Additionally, phenolic compounds are mainly composed of phenolic acids, flavonoids, and tannins, which play a critical role in plant growth and have very strong protective effects on various organs of the human body, with the biological activities of antioxidation, antibiosis, and anti-inflammatory [52,59]. In our investigation, [7]-Paradol, sinapyl alcohol, and isoeugenol presented a higher abundance in 4500 kg hm−2 organic fertilizer treatment, while grevilline B and (5a,6a,8a,11a)-8-Hydroxy-2-oxo 1(10),3-guaiadien-12,6-olide-15-al 8-(4-hydroxyphenylacetate) exhibited a higher abundance in 450 kg hm−2 compound fertilizer treatment (Fig. 7). This implied that we could choose the type of fertilizer to use depending on the actual situation of the phenolic compounds. Cinnamic acid and its derivatives are primary phenolic acid compounds found in various medicinal plants, exhibiting hypoglycemic, antioxidant, anti-inflammatory, and anticancer properties [60–64]. Caffeic acid and ferulic acid are the representative compounds of cinnamic acid and its derivatives in P. palustre [52]. Caffeic acid is one of the main signature components of the quality difference of P. palustre and is the material basis for biological activities such as anti-inflammatory, antioxidant, antibacterial, and metabolic regulation [3,65,66]. Ferulic acid reduces free radical levels and increases antioxidant levels to produce antioxidant effects [47]. Here, five differential cinnamic acids and their derivatives in the 450 kg hm−2 compound fertilizer treatment were significantly more abundant than those in the 4500 kg hm−2 organic fertilizer treatment (Fig. 7). It was inferred that the 450 kg hm−2 compound fertilizer treatment might have a positive effect on improving the antioxidant and pharmacological health effects of P. palustre.
It was determined that the optimal fertilization treatments for this study were 450 kg hm−2 compound fertilizer and 4500 kg hm−2 organic fertilizer. Organic fertilizers increased the abundance of 2 phenolic compounds and 12 organic oxygen compounds (including 1 monosaccharide), while compound fertilizers could increase the abundance of 21 organic oxygen compounds (including 4 oligosaccharides and 1 polysaccharide), 14 flavonoids, 2 phenolic compounds, and 5 cinnamic acid and its derivatives. This study provided valuable guidance for the cultivation and production of P. palustre. Additionally, in the context of global efforts to reduce pollution and protect the environment, the utilization of both compound fertilizers and organic fertilizers together could potentially be an effective strategy for enhancing the yield and quality of P. palustre.
Acknowledgement: None.
Funding Statement: This study was funded by the Fund Projects of the Central Government in Guidance of Local Science and Technology Development (GuiKeZY22096020), Guangxi Key R&D Plan Project (2023AB23078), National Natural Science Foundation of China (82260750), and Appropriate Technology Development and Promotion Project of Guangxi Traditional Chinese Medicine Administration (GZSY23-07).
Author Contributions: The authors confirm their contribution to the paper as follows: Write the draft manuscript: Suhuang Huang; Collect samples and data: Hao Chen, Fan Wei, and Meihua Xu; Analyze data: Jingchun Li, Zhining Chen, and Changqian Quan; Providing experimental organic fertilizer: Hongyu Li; Design and guide the experiments: Lijun Shi; Design and guide the experiments and revise the manuscript: Danfeng Tang. All authors reviewed the results and approved the final version of the manuscript.
Availability of Data and Materials: The data can be provided on demand.
Ethics Approval: Not applicable.
Conflicts of Interest: The authors declare that they have no conflicts of interest to report regarding the present study.
Supplementary Materials: The supplementary material is available online at https://doi.org/10.32604/phyton.2024.053492.
References
1. Tang DF, Huang Q, Wei KH, Yang X, Wei F, Miao JH. Identification of differentially expressed genes and pathways involved in growth and development of Mesona chinensis benth under red- and blue-light conditions. Front Plant Sci. 2021;12:761068. doi:10.3389/fpls.2021.761068. [Google Scholar] [CrossRef]
2. Lin L, Xie J, Liu S, Shen M, Tang W, Xie M. Polysaccharide from Mesona chinensis: extraction optimization, physicochemical characterizations and antioxidant activities. Int J Biol Macromol. 2017;99:665–73. doi:10.1016/j.ijbiomac.2017.03.040. [Google Scholar] [CrossRef]
3. Xiao L, Lu X, Yang H, Lin C, Li L, Ni C, et al. The antioxidant and hypolipidemic effects of Mesona chinensis benth extracts. Molecules. 2022;27(11):3423. doi:10.3390/molecules27113423. [Google Scholar] [CrossRef]
4. Huang L, Shen M, Wu T, Yu Y, Yu Q, Chen Y, et al. Mesona chinensis Benth polysaccharides protect against oxidative stress and immunosuppression in cyclophosphamide-treated mice via MAPKs signal transduction pathways. Int J Biol Macromol. 2020;152:766–74. doi:10.1016/j.ijbiomac.2020.02.318. [Google Scholar] [CrossRef]
5. Lim J, Adisakwattana S, Henry CJ. Effects of grass jelly on glycemic control: hydrocolloids may inhibit gut carbohydrase. Asia Pac J Clin Nutr. 2018;27(2):336–40. [Google Scholar] [PubMed]
6. Lu H, Shen M, Chen T, Yu Y, Chen Y, Yu Q, et al. Mesona chinensis Benth polysaccharides alleviate DSS-induced ulcerative colitis via inhibiting of TLR4/MAPK/NF-κB signaling pathways and modulating intestinal microbiota. Mol Nutr Food Res. 2022;66(15):e2200047. doi:10.1002/mnfr.v66.15. [Google Scholar] [CrossRef]
7. Tang DF, Wei F, Xie JX, Zhu YX, Wei KH, Miao JH. Key technologies and problems in conservation of Mesona chiensis benth in Guangxi. Mod Chin Med. 2022;24(3):407–11 (In Chinese). [Google Scholar]
8. Shi YL, Wang LL, Liu SB, Nie HG. Development of chemical fertilizer industry and its effect on agriculture of China. Acta Pedol Sin. 2008;5:852–64. [Google Scholar]
9. Li T, Zhang ZH, Guo YW, Tian X, Xu XW, Qiu LY. Research progress and prospect of microbial fertilizer at domestic and abroad. Jiangsu Agric Sci. 2019;47(10):37–41 (In Chinese). [Google Scholar]
10. Kang XH, An JJ, Wang LQ, Zhou H, Yang J. Effects of partial substitution of chemical fertilizer by organic fertilizer on welsh onion yield and quality. J Changjiang Veg. 2023;6:65–8 (In Chinese). [Google Scholar]
11. Yao WW, Zhang FJ. Effect of combined application of rice husk and compound fertilizer on physiological characteristics of tulips and weed control. J Nucl Agri Sci. 2023;37(5):1022–9. [Google Scholar]
12. He T, Dai HF, Li H, Zhang C. Effects of different fertilization methods on soil fertility in paddy field. Shanghai Agri Sci Tech. 2022;5:108–9 (In Chinese). [Google Scholar]
13. Wei HK. The action plan for reducing the use of chemical fertilizers and pesticides needs to be accelerated. In: The People’s Congress of China; 2018. [Google Scholar]
14. Chen LJ, Jiang YJ, Wang HT, Zhao QG, Sun B. Effects of long-term application of organic materials on phosphorus fractions and availability in red soil. Soils. 2020;52(3):451–7. [Google Scholar]
15. Li YQ. Study on agronomic and environmental effects of combined application of different organic manures with chemical fertilizer (Doctor Thesis). Chinese Academy of Agricultural Sciences: Beijing, China; 2016. [Google Scholar]
16. Wan LJ, Li JJ, Zhang J, Zheng YQ, Deng L, Yi SL. Research progress on the technology of organic fertilizer partial substituted for chemical fertilizer. North Hortic. 2021;11:133–42. [Google Scholar]
17. Yuan S, Ling Y, Xiong Y, Zhang CL, Sha LN, You MH, et al. Effect of nitrogen fertilizer on seed yield and quality of Kengyilia melanthera (Triticeae, Poaceae). PeerJ. 2022;10:e14101. doi:10.7717/peerj.14101. [Google Scholar] [CrossRef]
18. Tang DF, Quan CQ, Wei KH, Lin Y, Huang QF, Wei F, et al. Physio-morphological, biochemical and transcriptomic analyses provide insights into drought stress responses in Mesona chinensis Benth. Front Plant Sci. 2022;13:203. [Google Scholar]
19. Huang RS, Li XH, Deng J, Zhang M, Li LB, Zhang PG. Effects of nitrogen, phosphorus and potassium on the yield and gum content of Mesona chinensis Benth. In: Summit Forum on Traditional Chinese Medicine and Natural Medicine and the 12th National Symposium on Traditional Chinese Medicine and Natural Medicine, 2012; China. [Google Scholar]
20. Chen XB. Key points of Xiancao fertilization technology. Fujian Agri. 2012;1:23 (In Chinese). [Google Scholar]
21. Hong J, Yang L, Zhang D, Shi J. Plant metabolomics: an indispensable system biology tool for plant science. Int J Mol Sci. 2016;17(6):767. doi:10.3390/ijms17060767. [Google Scholar] [CrossRef]
22. Fernie AR. The future of metabolic phytochemistry: larger numbers of metabolites, higher resolution, greater understanding. Phytochemistry. 2007;68(22–24):2861–80. [Google Scholar] [PubMed]
23. Gong YH, Zhou GH, Peng SJ, Wu ZM, Liu J, Huang H, et al. Differential analysis of the metabolites on citrus pericarp brownspot based on untargeted metabolomics. J Chin Inst Food Sci Technol. 2022;22:316–24 (In Chinese). [Google Scholar]
24. Shi R, Wang S, Xiong B, Gu H, Wang H, Ji C, et al. Application of bioorganic fertilizer on Panax notoginseng improves plant growth by altering the rhizosphere microbiome structure and metabolism. Microorganisms. 2022;10(2):275. doi:10.3390/microorganisms10020275. [Google Scholar] [CrossRef]
25. Rao JF, Lyu WD, Song T. Effect of different microbial fertilizer on the growth and metabolites in Crocus sativus. J Zhejiang Univ (Sci Ed). 2019;46(6):731–6 (In Chinese). [Google Scholar]
26. Liu DJ, Liu Z, Yan YY, Zhang WH, Wang F, Yu YS, et al. Differential metabolites identification of Ma bamboo shoots under different low-temperature conditions based on non-target metabolomics. J Food Saf Qual. 2023;14(9):212–20. [Google Scholar]
27. Koh G, Lee DH, Woo JT. 2-Deoxy-D-ribose induces cellular damage by increasing oxidative stress and protein glycation in a pancreatic β-cell line. Metabolism. 2010;59(3):325–32. doi:10.1016/j.metabol.2009.07.028. [Google Scholar] [CrossRef]
28. Fontana MB, de Bastos MC, Brandelli A. Bacteriocins Pep5 and epidermin inhibit Staphylococcus epidermidis adhesion to catheters. Curr Microbiol. 2006;52(5):350–3. doi:10.1007/s00284-005-0152-5. [Google Scholar] [CrossRef]
29. He X, Zheng S, Sheng Y, Miao T, Xu J, Xu W, et al. Chlorogenic acid ameliorates obesity by preventing energy balance shift in high-fat diet induced obese mice. J Sci Food Agr. 2021;101(2):631–7. doi:10.1002/jsfa.v101.2. [Google Scholar] [CrossRef]
30. Tanyeri-Bayraktar B, Bayraktar S, Hepokur M, Güzel Kıran G. Comparison of two different doses of sucrose in pain relief. Pediatr Int. 2019;61(8):797–801. doi:10.1111/ped.v61.8. [Google Scholar] [CrossRef]
31. Whitaker BD, Saftner RA. Temperature-dependent autoxidation of conjugated trienols from apple peel yields 6-methyl-5-hepten-2-one, a volatile implicated in induction of scald. J Agr Food Chem. 2000;48(6):2040–3. doi:10.1021/jf991107c. [Google Scholar] [CrossRef]
32. Ferreira VF, de Carvalho AS, Ferreira PG, Lima CGS, de C da Silva F. Quinone-based drugs: an important class of molecules in medicinal chemistry. Med Chem. 2021;17(10):1073–85. doi:10.2174/1573406416666201106104756. [Google Scholar] [CrossRef]
33. He L, Zhang F, Jian Z, Sun J, Chen J, Liapao V, et al. Stachyose modulates gut microbiota and alleviates dextran sulfate sodium-induced acute colitis in mice. Saudi J Gastroentero. 2020;26(3):153–9. doi:10.4103/sjg.SJG_580_19. [Google Scholar] [PubMed] [CrossRef]
34. Nyssen P, Franck T, Serteyn D, Mouithys-Mickalad A, Hoebeke M. Propofol metabolites and derivatives inhibit the oxidant activities of neutrophils and myeloperoxidase. Free Radical Bio Med. 2022;191(4–9):164–75. doi:10.1016/j.freeradbiomed.2022.08.039. [Google Scholar] [CrossRef]
35. Suzuki E, Yamamura N, Ogura Y, Nakai D, Kubota K, Kobayashi N, et al. Identification of valproic acid glucuronide hydrolase as a key enzyme for the interaction of valproic acid with carbapenem antibiotics. Drug Metab Dispos. 2010;38(9):1538–44. doi:10.1124/dmd.110.032938. [Google Scholar] [CrossRef]
36. Lin S, Li L, Li M, Gu H, Chen X. Raffinose increases autophagy and reduces cell death in UVB-irradiated keratinocytes. J Photoch Photobio B. 2019;201(9):111653. doi:10.1016/j.jphotobiol.2019.111653. [Google Scholar] [CrossRef]
37. Lin XL, Li K, Yang Z, Chen B, Zhang T. Dulcitol suppresses proliferation and migration of hepatocellular carcinoma via regulating SIRT1/p53 pathway. Phytomedicine. 2020;66(9):153112. doi:10.1016/j.phymed.2019.153112. [Google Scholar] [CrossRef]
38. Gamal AA, Abbas HY, Abdelwahed NAM, Kashef MT, Mahmoud K, Esawy MA, et al. Optimization strategy of Bacillus subtilis MT453867 levansucrase and evaluation of levan role in pancreatic cancer treatment. Int J Biol Macromol. 2021;182(5):1590–601. doi:10.1016/j.ijbiomac.2021.05.056. [Google Scholar] [CrossRef]
39. Parichatikanond W, Pinthong D, Mangmool S. Blockade of the renin-angiotensin system with delphinidin, cyanin, and quercetin. Planta Med. 2012;78(15):1626–32. doi:10.1055/s-00000058. [Google Scholar] [CrossRef]
40. Jayachandran M, Wu Z, Ganesan K, Khalid S, Chung SM, Xu B. Isoquercetin upregulates antioxidant genes, suppresses inflammatory cytokines and regulates AMPK pathway in streptozotocin-induced diabetic rats. Chem-Biol Interact. 2019;303:62–9. doi:10.1016/j.cbi.2019.02.017. [Google Scholar] [CrossRef]
41. Sun C, Wang L, Sun J, Wang Z, Tang Z. Hypoglycemic and hypolipidemic effects of rutin on hyperglycemic rats. J Tradit Chin Med. 2020;40(4):640–5. [Google Scholar] [PubMed]
42. Hisaka T, Sakai H, Sato T, Goto Y, Nomura Y, Fukutomi S, et al. Quercetin suppresses proliferation of liver cancer cell lines in vitro. Anticancer Res. 2020;40(8):4695–700. doi:10.21873/anticanres.14469. [Google Scholar] [PubMed] [CrossRef]
43. Diao M, Liang Y, Zhao J, Zhao C, Zhang J, Zhang T. Enhanced cytotoxicity and antioxidant capacity of kaempferol complexed with α-lactalbumin. Food Chem Toxicol. 2021;153:112265. doi:10.1016/j.fct.2021.112265. [Google Scholar] [CrossRef]
44. Wang W, Liu Y, Che F, Li H, Liu J, Wu N, et al. Isolation and purification of flavonoids from Euonymus alatus by high-speed countercurrent chromatography and neuroprotective effect of rhamnazin-3-O-rutinoside in vitro. J Sep Sci. 2021;44(24):4422–30. doi:10.1002/jssc.v44.24. [Google Scholar] [CrossRef]
45. Choi J, Shin KM, Park HJ, Jung HJ, Kim HJ, Lee YS, et al. Anti-inflammatory and antinociceptive effects of sinapyl alcohol and its glucoside syringin. Planta Med. 2004;70(11):1027–32. doi:10.1055/s-2004-832642. [Google Scholar] [CrossRef]
46. Zhang LL, Zhang LF, Xu JG, Hu QP. Comparison study on antioxidant, DNA damage protective and antibacterial activities of eugenol and isoeugenol against several foodborne pathogens. Food Nutr Res. 2017;61(1):1353356. doi:10.1080/16546628.2017.1353356. [Google Scholar] [CrossRef]
47. Erbil G, Sacik U, Yilmaz F, Kisaoglu H, Erbayraktar Z, Pekcetin C, et al. The effect of ferulic acid on experimental traumatic brain damage in rats. Bratisl Med J. 2019;120(5):372–9. doi:10.4149/BLL_2019_061. [Google Scholar] [PubMed] [CrossRef]
48. He CB, Deng T, Wei HC, Wu GH, Shangguan YC, Xiong HJ. Protective effect of polysaccharide from Mesona blumes on oxidative damage of cells. Food Sci. 2020;41(13):160–8. [Google Scholar]
49. Qi GM. Analysis of polysaccharide hydrolysate monosaccharide in Mesona chinensis by HPLC-UV. Fujian Anal Test. 2022;31(3):28–33 (In Chinese). [Google Scholar]
50. Niu R, Han NJ, Fang HL. Research progress on active ingredients and efficacy of Mesona chinensis Beath. Mod Commun. 2018;13:254–5. [Google Scholar]
51. Chen X, Xiao W, Shen M, Yu Q, Chen Y, Yang J, et al. Changes in polysaccharides structure and bioactivity during Mesona chinensis Benth storage. Curr Res Food Sci. 2022;5:392–400. doi:10.1016/j.crfs.2022.01.024. [Google Scholar] [CrossRef]
52. Lin LH, Huang LX, Xie JH. Review on main chemical constituents and biological activities of Mesona chinensis. Sci Technol Food Ind. 2016;37(20):356–9. [Google Scholar]
53. Wei ZL. Study on chemical constituents and quality evaluation of herba mesona (Master Thesis). Guangzhou University of Chinese Medicine: China; 2016 (In Chinese). [Google Scholar]
54. Yin J, Peng X, Lin J, Zhang Y, Zhang J, Gao H, et al. Quercetin amelioratesAspergillus fumigatuskeratitis by inhibiting fungal growth, toll-like receptors and inflammatory cytokines. Int Immunopharmacol. 2021;93:107435. doi:10.1016/j.intimp.2021.107435. [Google Scholar] [CrossRef]
55. Yoshikawa T, Mifune Y, Inui A, Nishimoto H, Yamaura K, Mukohara S, et al. Quercetin treatment protects the Achilles tendons of rats from oxidative stress induced by hyperglycemia. BMC Musculoskeletal Disord. 2022;23(1):563. doi:10.1186/s12891-022-05513-4. [Google Scholar] [CrossRef]
56. Shi Y, Chen X, Liu J, Fan X, Jin Y, Gu J, et al. Isoquercetin improves inflammatory response in rats following ischemic stroke. Front Neurosci. 2021;15:555543. doi:10.3389/fnins.2021.555543. [Google Scholar] [CrossRef]
57. Li L, Wang R, Hu H, Chen X, Yin Z, Liang X, et al. The antiviral activity of kaempferol against pseudorabies virus in mice. BMC Vet Res. 2021;17(1):247. doi:10.1186/s12917-021-02953-3. [Google Scholar] [CrossRef]
58. Cao J, Li C, Ma P, Ding Y, Gao J, Jia Q, et al. Effect of kaempferol on IgE-mediated anaphylaxis in C57BL/6 mice and LAD2 cells. Phytomedicine. 2020;79:153346. doi:10.1016/j.phymed.2020.153346. [Google Scholar] [CrossRef]
59. Huang JJ, Huang ZL, Chen HG, Liu K, Wang LK, Zhi H, et al. Analysis of polyphenols from Mesona chinensis by UPLC-Q -TOF-MS/MS. Nat Prod Res Dev. 2021;33(5):758–66. [Google Scholar]
60. Sova M. Antioxidant and antimicrobial activities of cinnamic acid derivatives. Mini-Rev Med Chem. 2012;12(8):749–67. doi:10.2174/138955712801264792. [Google Scholar] [CrossRef]
61. De Cássia da Silveira e Sá R, Andrade LN, Dos Reis Barreto de Oliveira R, De Sousa DP. A review on anti-inflammatory activity of phenylpropanoids found in essential oils. Molecules. 2014;19(2):1459–80. doi:10.3390/molecules19021459. [Google Scholar] [CrossRef]
62. Alam MA, Subhan N, Hossain H, Hossain M, Reza HM, Rahman MM, et al. Hydroxycinnamic acid derivatives: a potential class of natural compounds for the management of lipid metabolism and obesity. Nutr Metab. 2016;13(1):27. doi:10.1186/s12986-016-0080-3. [Google Scholar] [CrossRef]
63. Anantharaju PG, Gowda PC, Vimalambike MG, Madhunapantula SV. An overview on the role of dietary phenolics for the treatment of cancers. Nutr J. 2016;15(1):99. doi:10.1186/s12937-016-0217-2. [Google Scholar] [CrossRef]
64. Sun YM, Wu Q, Gao J, Zhang X, Zhao L, Dong SQ. Determination of cinnamic acid and its derivatives by dispersive solid phase microextraction of graphene quantum dots magnetic composite nanoparticles coupled with capillary electrophoresis. Chin J Chromatogr. 2017;35(3):325–31 (In Chinese). doi:10.3724/SP.J.1123.2016.10017. [Google Scholar] [CrossRef]
65. Huang GJ, Liao JC, Chiu CS, Huang SS, Lin TH, Deng JS. Anti-inflammatory activities of aqueous extract of Mesona procumbens in experimental mice. J Sci Food Agr. 2012;92(6):1186–93. doi:10.1002/jsfa.v92.6. [Google Scholar] [CrossRef]
66. Xie P, Chen QH, Xu XX, Shen JH, Hao CL, Lin LL, et al. Study on quality control of Mesona chinensis Benth. Based on HPLC Fingerprint combined with chemical pattern recognition and TOPSIS analysis. Chin J Inf Tradit Chin Med. 2022;29(5):98–104 (In Chinese). [Google Scholar]
Cite This Article
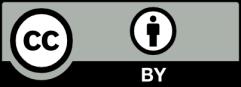
This work is licensed under a Creative Commons Attribution 4.0 International License , which permits unrestricted use, distribution, and reproduction in any medium, provided the original work is properly cited.