Open Access
ARTICLE
In Vitro Synthetic Seed Production of Potato under Different Fungicide Levels and Storage Intervals
1 Department of Horticulture, PMAS-Arid Agriculture University, Rawalpindi, 46300, Pakistan
2 Department of Agronomy, Sher-e-Bangla Agricultural University, Dhaka, 1207, Bangladesh
3 Department of Agronomy, PMAS-Arid Agriculture University, Rawalpindi, 46300, Pakistan
4 Department of Plants and Crops, Ghent University, Ghent, 9000, Belgium
* Corresponding Authors: Mirza Hasanuzzaman. Email: ; Mukhtar Ahmed. Email:
Phyton-International Journal of Experimental Botany 2023, 92(8), 2429-2450. https://doi.org/10.32604/phyton.2023.030846
Received 09 February 2023; Accepted 04 May 2023; Issue published 25 June 2023
Abstract
Potato propagation through tubers and seed is an inefficient way of propagation thus we propose an alternative method in this study which is in vitro propagation. Plantlets were raised from True Potato Seeds (TPS) in test tubes containing 10 mL of Murashige Skoog (MS) medium. Subculturing was done after every 21 days and after four subsequent subculturing, when there was an adequate quantity of stock material, uninodal cuttings of about 3–4 mm in length were prepared from in vitro proliferated potato plants by excising leaves of 1–2 mm on each side of the node. Prepared uninodal cuttings were cultured on ½ strength MS medium for root primordia development. The selected uninodal cuttings with root primordia were encapsulated with sodium alginate solution (3.5% (w/v), mixed with 1.25% charcoal and different concentration of fungicide (thiophanate methyl, sigma) to enhance the viability percentage of synthetic seed. Furthermore, these encapsulated uninodal cuttings were subjected to different storage intervals to check maximum storage. Results showed that optimum dose for fungicide is 150 mg/L as it resulted in good viability percentage, shoot number, shoot length, rooting percentage, root number, root length and ideal for bead formation with sodium alginate. Similarly, results revealed that encapsulated uninodal cuttings can be stored at a temperature of 4°C up to 45 days with a survival rate of 63.33%. Encapsulated uninodal cutting stored for 60 days showed a poor viability percentage of about 43.03%. It can be concluded that optimum dose of 150 mg/L with storage time of 45 days should be used to get a better outcome for synthetic potato seed production.Keywords
Potato (Solanum tuberosum), the annual herb of the family Solanaceae, is the fourth most important food crop grown worldwide with annual production approaching 370 MT [1]. Owing to its nutritional benefits, it is a high-value cash crop. Potato contains carbohydrates, proteins, minerals, vitamins (B and C), and dietary fiber [2–4]. In conventional systems, seed potato tubers are utilized for propagation [5], being an inefficient approach with a low rate of multiplication along with a high risk of catching various diseases and pests. Therefore, a rapid multiplication system like in vitro propagation can solve some of the problems associated with conventional propagation methods [6,7]. Tissue culture has revolutionized seed potato production in some countries by increasing potato yield and mass multiplication of virus-free potato seedlings [8,9]. Among in vitro propagation techniques, synthetic seed technology can eliminate approximately 100% of viruses in seed production [10–12]. Similarly, this technique could be a viable option under changing climates, where the occurrence of extreme events is more prevalent [13].
Synthetic seeds are defined as artificially encapsulated somatic embryos, shoot buds, cell aggregates, or any other tissue that can be used for sowing as a seed. They should possess the ability to transform into plants under in vitro or ex-vitro conditions and retain this potential even after storage [14]. There has been a growing interest in the production and use of synthetic seed technology due to the advantages of producing genetically identical planting material for easy handling, transportation, and storage (<1 cm), as well as offering a new option for the maintenance of elite germplasm [15]. The use of synthetic seeds has been long considered a promising alternative to conventional potato propagation, but little has been accomplished to develop synthetic seeds in this crop [14,16]. Therefore, the present study was designed to investigate the effect of different concentrations of thiophanate methyl (TM) fungicide and storage interval on synthetic seed production of potato using alginate-mediated nutrient encapsulation of in vitro grown uninodal shoot segments. Key objectives of this study were the evaluation of the optimum dose of fungicide for the encapsulation and the appropriate storage period for potato synthetic seeds.
2.1 In Vitro Synthetic Seed Production
In vitro established cultures are required for the preparation of synthetic seeds. Plantlets were raised from True Potato Seeds (TPS), and 5% (v/v) NaOCl was used to eradicate the chances of contamination in TPS. After sterilization, seeds were sown in test tubes containing 10 mL of Murashige Skoog (MS) medium (MS macro and microelements) (Sigma-Aldrich, Saint Louis, MO, USA) supplemented with 100 mg/L myo-inositol, 0.5 mg/L nicotinic acid, 0.5 mg/L pyridoxine, 0.1 mg/L thiamine, 30 g/L sugar, and 7 g/L agar. Germination percentage, vigor, and growth rate of seedlings were recorded after emergence. From in vitro grown plantlets, shoots of about 1.5 cm in length were excised and cultured in jars containing 40 mL MS medium supplemented with 150 mg/L myo-inositol, 0.5 mg/L nicotinic acid, 0.5 mg/L pyridoxine, 0.5 mg/L thiamine-HCl, 0.5 mg/L biotin, 1.0 mg/L Ca-pantothenate, 2 mg/L glycine, 1 mg/L GA3, 30 g/L sugar, and 7 g/L agar. Subculturing was done after every 21 days and after four subsequent subculturing, when there was an adequate quantity of stock material, uninodal cuttings of about 3–4 mm in length were prepared from in vitro proliferated potato plants by excising leaves of 1–2 mm on each side of the node. Prepared uninodal cuttings were cultured on ½ strength MS medium for root primordia development. The medium was supplemented with 0.25 mg/L IBA, 100 mg/L brilliant black, 10 g/L sucrose, 5 g/L glucose, and 7 g/L agar. The selected uninodal cuttings with root primordia were encapsulated with sodium alginate (medium viscosity, Sigma A-2033) solution (3.5% (w/v), mixed with 1.25% charcoal and different concentration of fungicide (thiophanate methyl, sigma) to enhance the viability percentage of synthetic seed (Table 1). Encapsulation was followed by complexation with 1.1% (w/v) CaCl2 enriched with artificial endosperm composed of ½ MS (macro and micro) medium for 35 min. After complexation, the alginate capsules were washed twice for 15 min, each time with autoclaved rinse solution consisting of the artificial endosperm. This experiment was conducted with five treatments, each comprising three replications and 10 synthetic seeds per replication.
The optimum level of TM fungicide was evaluated for better conversion and viability of synthetic seeds. Alginate media was provided with different concentrations of TM and prepared synthetic seeds were stored for 15 days. The best resulting dose of TM was further used to evaluate the viability percentage of synthetic seeds at 4°C for different time intervals of 0, 15, 30, 45, 60, and 75 days after encapsulation. Seeds in the control treatment were sown directly on germination media without giving any storage after encapsulation. After storage at different time intervals, synthetic seeds were sown on MS medium supplemented with 100 mg/L myo-inositol, 0.5 mg/L nicotinic acid, 0.5 mg/L pyridoxine, 0.1 mg/L thiamine, 1 mg/L GA3, 30 g/L sugar, and 7 g/L agar. The data were calculated for germination percentage, and growth rate was measured on a weekly basis by recording viability, number of leaves, shoot length (cm), number of nodes, and conversion ability. After four weeks, rooting of synthetic seeds in terms of rooting %, the number of roots per plantlets, root length (cm), and rooting index. The rooting index is a measure through which the effect of length and number of roots on the performance of roots can be evaluated. Longer roots with less number per synthetic seed are not desirable, whereas shorter roots with a greater number of roots perform better as well-established rooting necessary for the success of plantlets. The pH of the whole media used in this study was maintained at 5.8 and cultures were incubated at 25 ± 1°C under a 16/8 h photoperiod (2,000 lux) with white fluorescent tubes (Philips TL 40W/54).
Statistical analysis of the data was carried out using ANOVA and differences among treatment means were compared by using the least significance difference (LSD) test at a 5% probability level [17]. Pearson correlation coefficient (r) was used to study the pairwise relationship between measured traits.
Data regarding contamination revealed that there was a 92% survival rate with a vigor rate of 6.12, and only 8% of total seeds were infested. Plantlets attained an average height of 2.1 cm and 3.12 leaves in the first week, while, 4.19 cm height of shoots and 5.19 leaves was recorded in the second week. In the third week, plantlets attained a height of 6.7 cm with seven leaves on the shoot (Fig. 1).
Figure 1: Number of leaves and shoot length (cm) of potato plants sown on Murashige and Skoog medium
3.2 Evaluation of Optimum Dose of Thiophanate Methyl
Data showed significant results in terms of viability and growth rate of synthetic seeds stored at 4°C for 15 days. The viability percentage increased gradually from 60% to 80% (Table 2) in synthetic seeds from 0 mg/L (T1) to 150 mg/L (T4) respectively (Fig. 2) with increasing fungicide concentrations. A Marked decline (45%) in the viability and growth rate of potatoes due to the higher concentration of TM at 200 mg/L (T5) in encapsulated media was observed.
Figure 2: Maximum viability percentage (80%) of synthetic seeds at 150 mg/L TM (T4)
3.2.2 Shoot Number and Shoot Length (cm)
Statistical analysis showed a significant effect of different concentrations of TM incorporated in encapsulation medium on shoot number and shoot length of plantlets derived from synthetic seed. In the control treatment shoot number was 0.73 with a shoot length of 1.55 cm (Table 2). More number of shoots, i.e., 1.07 at 50 mg/L (T2) (Fig. 3a) and maximum shoot length, i.e., 4.24 cm (Fig. 3b) was recorded at 150 mg/L (T4) after which there was a marked decline (Table 2). Moreover, higher concentration (200 mg/L) of TM decreased shoot number up to 0.60 and shoot length reduced to 2.15 cm.
Figure 3: Maximum (a) shoot number (1.07) at 50 mg/L (T2) and (b) shoot length (4.24 cm) derived from synthetic seeds added with 150 mg/L of TM (T4) sown on Murashige and Skoog medium after 15 days of storage interval
3.2.3 Rooting Percentage, Root Number and Root Length (cm)
Significant results were obtained in the case of rooting percentage, number, and length of roots (Table 3). Best rooting percentage of 73.33% was recorded at 150 mg/L (T4) with a maximum root number of 4.70 and root length of 2.29 cm (Fig. 4). Declining trend was noticed at suboptimal (<150 mg/L) and supraoptimal (>150 mg/L) concentrations.
Figure 4: Maximum (a) root number (4.70) and (b) root length (2.29 cm) observed at 150 mg/L (T4)
Different storage intervals affected the viability percentage of synthetic seeds. Results showed that 83.33% (Table 4) was the maximum viability percentage that was obtained in synthetic seeds sown just after encapsulation (control treatment). Beyond 45 days (S3) storage, there was a significant decline in viability percentage (63.33%), and synthetic seeds at 60 and 75 days (S4 and S5) of storage interval showed poor viability percentage of 59.33% and 43.03%, respectively (Table 4).
Potato plants develop a single shoot on each node as it has no axil to develop a side shoot. Results in Table 4 illustrate the maximum outcome regarding the shoot number of 1.10 after 45 days (S3) (Fig. 5a), while a minimum number of shoots (0.57) was obtained at 75 days (S5) (Fig. 5b).
Figure 5: Number of shoots regenerated from synthetic seeds sown on Murashige and Skoog medium (a) maximum (1.10) at 45 days of storage interval (S3) (b) minimum (0.57) at 75 days of storage interval (S5)
ANOVA showed a significant effect of storage intervals on shoot length at p < 0.05 (Table 4). The controlled treatment (0 days) gave maximum shoot length, i.e., 10.58 cm (Fig. 6a), and with increasing storage intervals, shoot length decreased markedly. A declining trend was observed with increasing the storage interval from 15 to 75 days. The storage interval of 75 days (S5) resulted in reduced plant height (3.29 cm) (Fig. 6b) which showed that increased storage intervals have a negative effect on the shoot length.
Figure 6: Shoot length regenerated from synthetic seeds sown on Murashige and Skoog medium (a) maximum (10.58 cm) at 0 days (S0) (b) minimum at (3.28 cm) 75 days of storage interval (S5)
3.3.4 Number of Leaves and Nodes
The number of leaves and nodes is the same because each node produces a leaf. Significant results were obtained in terms of the number of leaves and nodes per synthetic seed as affected by storage interval. Marked differences were observed between the control (0 days) and other storage intervals (15, 30, 45, 60, and 75 days) in terms of producing the number of leaves and nodes. The maximum number of leaves and nodes were recorded in controlled treatment, i.e., 8.07 (Table 4) (Fig. 7a). There was a gradual decrease in the number of leaves and nodes up to different storage intervals. Therefore, shoot length affects the number of leaves and nodes; smaller shoots give a smaller number of leaves and nodes (Fig. 7b), while larger shoots give a larger number of leaves and nodes.
Figure 7: Number of leaves and nodes regenerated from synthetic seeds sown on Murashige and Skoog medium (a) maximum (8.07) at 0 days (S0) (b) minimum (3.09) at 75 days (S5) of storage interval
The rooting percentage of plantlets derived from synthetic seed was maximum in the control treatment (0 days), i.e., 86.67% (Table 5). The results of different storage intervals (0, 15, 30, 45, 60, and 75 days) differed significantly from each other. It was observed that the rooting percentage of plantlets derived from synthetic seeds decreased with an increase in storage intervals. A maximum rooting level of 76.6% was obtained in synthetic seeds sown after 15 days (S1) of storage interval. In comparison, a minimum rooting level (54%) was observed in synthetic seeds kept for 75 days (S5) of storage interval. There was a decreasing trend in the case of rooting percentage up to 45 days (S5), and after that there were highly poor results.
3.4.2 Number of Roots and Root Length (cm)
Synthetic seeds stored for 15 days (S2) produced a maximum number of roots (8.00) (Fig. 8a) followed by a controlled treatment (0 days) that has shown non-significant difference in terms of producing the number of roots (7.12). Prolonged storage interval decreased the number of roots (Fig. 8b), and a substantial difference was observed with each increase in the number of days. Whereas maximum root length was recorded in the control treatment (0 days), reaching 7.95 cm (Fig. 9a) while 75 days (S5) produced minimum root length (2.59 cm) (Fig. 9b), there was a rapid decline in results obtained as with increased storage interval root length significantly dropped (Table 5). Root length significantly decreased with an increase in storage interval as 15 days of storage interval produced a root length of 7.51 cm followed by indicative less root length of 7.00 cm in 30 days of storage. With the increase in storage interval from 30 to 45 days, root length reduced to 6.47 cm, and when the synthetic seed was stored for 60 days, the root length decreased even more and reached up to 5.10 cm. Decreased root length with the increase in storage interval could be attributed to the poor response of synthetic seed for long-term storage.
Figure 8: Number of roots regenerated from synthetic seeds sown on Murashige and Skoog medium (a) maximum at 15 days (S2) of cold storage (b) minimum at 75 days (S5) of cold storage
Figure 9: Root length (cm) of plantlets regenerated from synthetic seeds sown on Murashige and Skoog medium (a) maximum (7.95 cm) at 0 days (S0) (b) minimum (2.59 cm) at 75 days (S5) of cold storage
Maximum rooting index obtained in S1 (15 days), i.e., 60.08 (Table 6) while S5 (75 days) gave a minimum rooting index (of 8.96) (Table 6). Results indicated that synthetic seed placed at 15 days of storage produced good roots whereas rooting index per plantlets decreased adversely at 75 days of storage interval.
Scatterplot was used to build a relationship between the number of roots and shoots under different storage intervals and fungicides treatments. A positive relationship was observed among the number of roots and shoots in response to different storage intervals (Fig. 10). The regression equation obtained was y = 3.8299x + 2.4732 with R2 of 0.80. A Negative relationship was observed between the number of roots and shoots under different fungicides treatments. The equation obtained was y= −1.0194x + 4.1221 with R2 = 0.30 (Fig. 11). Furthermore, Pearson correlation analysis was used to elaborate relationship among different study variables in response to different storage intervals and fungicides treatments (Tables 6 and 7).
Figure 10: Relationship between the number of shoots with roots under different storage intervals
Figure 11: Relationship of the number of shoots with roots under different fungicides treatments
Fungicides such as PPM, carbendazine, and TM are being used to control the contamination of synthetic seeds [18]. After a meticulous study on fungicides, TM was found to be effective and therefore used in this study [19]. TM dose evaluated for better growth and viability of synthetic seed after this research was 150 mg/L. However, our findings are optimum for potatoes, and may not be applicable to other crops; thus, there is a need for further investigation to find out the interaction of in vitro plant tissue of other species with different levels of TM. Yugar et al. [20] reported that higher concentrations reduced viability while lower concentrations of fungicide along with charcoal increased the viability of the synthetic seed. Tomlin [21] showed that micronuclei could be induced by TM but they did not reveal any centromeric signal, revealing clastogenic action (disruption or breakage, as of chromosome) of TM. Moreover, it was observed that cellular proliferation was delayed, and the frequency of apoptotic cells was increased as a function of the concentration of TM as cellular proliferation delayed viability percentage. Higher concentration of fungicides is considered metabolic inhibitors because they inhibit electron transport chain (etc.), enzymes, nucleic acid, proteins, and sterol synthesis, therefore, decreasing the viability of synthetic seeds [22]. The poor performance of synthetic seeds with a higher concentration of TM might be due to the variability in fungicide protective action to the persistence of active ingredients in the plant tissues [23]. Ganapathi et al. [24] concluded that the fungicide carbendazine proved better in controlling the contamination of synthetic seeds from microorganisms. Similarly, Micheli et al. [25] reported that the addition of PPM in the encapsulation matrix enhances the conversion of synthetic seeds in apple rootstock. Moreover, it was observed that PPM reduced the sprouting and conversion of synthetic seeds in citrus, and it also suppressed in vitro shoot regeneration and proliferation of other plants [26]. Fungicides are supposed to induce morphogenetic and organogenetic responses in encapsulated cutting [27].
In this study, the reduction in shoot number and shoot length was due to the higher concentration of fungicides, as they hinder nucleic acid and protein synthesis by inhibiting DNA and RNA synthesis (nuclear division) and disrupting cell division and cellular metabolism. Moreover, it slower down, etc., along the cytochromes by reducing mitochondrial respiration and blocking the cytochrome bc1 complex. Cell wall production is affected by sterol inhibition due to fungicide (dimethomorph) application leading towards poor cell division and hence results in less number and stunted shoots [22]. TM is a systemic fungicide affecting cell division and it was observed that TM has cytotoxic and cell-transforming activities [28]. Moreover, Yugar et al. [20] suggested that the incorporation of TM to avoid contamination is an appropriate strategy to increase the number of shoots. When TM was incorporated with an appropriate quantity (150 mg/L), its influence was positive and gave maximum shoot number and length. Gardi et al. [29] found beneficial effects of adjuvants on the growth of synthetic seeds increasing shoot length and number. Since the main hindrance in synthetic seed technology was the unipolar (only shoot primordia) nature of cuttings taken for encapsulation which was resolved afterward by root induction treatments with suitable auxins [30]. Rooting of synthetic seed also depends on the morphology of the plant part taken for encapsulation. As shoot length increases, auxin concentration inside the plant increase and more ethylene is produced, resulting in maximum root length and a greater number of roots. Ethylene enhances IAA transport in cells of the epidermis and central cylinder of plant tissues which arbitrate its basipetal and acropetal movements [31]. Inhibited root growth might be related to oxygen deficiency in the gel matrix and the release of detrimental metabolites [18].
Storage of synthetic seed is employed for the conservation and exchange of germplasm for longer periods of time and for long-distance transportation. The optimum storage period is desirable in synthetic seeds, without loss of their viability significantly, so, basic purpose of this study was to find out the optimum period of storage with a high level of viability to store synthetic seeds efficiently after preparation up to storage. Although there was a declining trend in terms of viability percentage and maintained at a reasonable level, i.e., up to 45 days, and this could be attributed to the accretion of certain endogenous chemicals accumulated during cold storage, but the viability was not lost completely [32].
In our study, better results up to certain period (45 days) could be linked with the accumulation of polyamines in the cells of encapsulated cuttings. Previously, it was observed that 15 days of cold storage increased endogenous spermidine content, which plays an important role in cell protection [33]. Furthermore, it was reported by Shen et al. [34] that polyamines are induced by cold storage, having a further role in membrane protection and cellular function. The accumulation of polyamines is one of the typical responses of plant resistance to cold storage [35]. Polyamines have a considerable influence on the growth and differentiation of plant cells and tissues [36]. They stabilize the cellular membrane by binding with negatively charged cellular components such as nucleic acid, proteins, and phospholipids which help in germination by promoting cell division. Manipulation of polyamines level in the cuttings inhibits the rise of protease activity, or it can be concluded that the integrity of the membrane is preserved by preventing degradation from cytoplasmic or vascular proteases [37]. Singh et al. [38] reported a reduction in survival rates with increased storage time which suggested that conditions during storage could reduce the viability and vigor of encapsulated propagules. Along with storage intervals, certain co-factors (inclusion of nutrients in alginate matrix, storage conditions, and type of encapsulated cuttings) can also be highlighted in terms of affecting the viability of synthetic seed, as all of these factors work in association with each other. It was assumed that any rate of decline in viability percentage observed among encapsulated cuttings stored at low temperatures may have resulted because of inhibited respiration of plant tissues due to alginate cover. The performance of synthetic seed is supposed to be affected by restricted oxygen diffusion, increased ethylene production, and the hindrance of coating material for shoot and radical emergence [39].
According to Ballester et al. [32], one of the most important factors that can influence the success of cold storage (4°C) is the physiological state of explants at the time of being placed in cold storage. Storage effects increased viability in the case of woody species like in olives [40] or Cedrela odorata [41], but our results were different from these reports, as the plant used in this research was herbaceous. The reason for this contradiction could be that the plant was sensitive to cold storage. However, our results can be in line with the results acquired in other solanaceous plants like tomato, as Porter [42] reported that storage of encapsulated cuttings failed to produce viable plants. Furthermore, West et al. [43] observed that the viability percentage of synthetic seed decreased over time as a result of nutrient depletion within the synthetic seed. Protrusion of the cutting from the surface of the gel matrix due to incomplete coverage of cutting by the sodium alginate in a few synthetic seeds is the most common problem of less viability level [43]. As the cuttings come under the effect of cold storage, they are dehydrated and viability decreases. Detrimental effects include desiccation, susceptibility to shearing forces, early emergence, and necrosis [44]. RNA degradation leads to a lack of signal in cell, resulting in tissue deterioration and reduction of biological activity during storage. Porter [42] observed that storage of synseeds for 15 and 30 days resulted in a significantly lower survival and conversion rates.
Encapsulated cuttings remained responsive for cold storage for up to 45 days, after which there was a significant decline in terms of viability percentage. Similarly, Naik et al. [30] concluded that after 30 days of storage at 4°C, there was a decrease in viability, whereas 60 days of storage led to a complete loss in the viability of synthetic seeds. This might be due to reduced respiration as a result of alginate coating, because in storage, encapsulated cuttings need to perform their metabolic activities, and termination in this due to any factor would cause a poor viability of shriveled or shrunken cuttings. To avoid this problem, charcoal was added in encapsulated media in this research, as many researchers opine that charcoal increases porosity and, ultimately, aeration. Whereas addition of charcoal in encapsulated media increases its conversion ratio and synthetic seeds start sprouting even within the storage interval. For instance, Kumar et al. [39] reported enhanced germination and conversion to a maximum extent by adding charcoal to the alginate matrix, which increased the diffusion of gases, nutrients, and respiration of tissues. West et al. [43] stated that storage interval has no negative effect on the conversion of encapsulated bud and hence, the number of shoot development. The shoot number was unaffected by the storage condition because all nodes produce one shoot each, regardless of the storage time. The development of more, or a smaller number of shoots depends on the morphology of the encapsulated cutting. Storage intervals have no effect on the number of shoots, but possibilities could be withdrawn for the development of more number of shoots when encapsulated media was provided with growth hormone [45].
The inclusion of energy-giving substances like sucrose could not be neglected in affecting the performance of explants as encapsulation media was provided with sucrose because it has its role along with storage interval. The addition of sucrose might be logical for the development of a smaller number of shoots, as sucrose gives osmotic stress to the plant. Mamiya et al. [19] reported that an increase in osmotic stress by the addition of sucrose inhibited shoot growth. Nieves et al. [46] studied that sucrose has two main roles under in vitro conditions, that is, a carbon source and an osmotic agent. A high level of osmotic stress has been associated with cellular plasmolysis and a high level of endogenous abscisic acid (ABA) [46].
Moreover, Nieves et al. [46] also found that osmotic stress has a role in ABA production and synthesis of proline, other amino acids, and sugars or protein. Depletion of nutrients occurs from artificial endosperm due to leakage into the external medium during storage interval, whereas nutrients have a marked role in the development of the number of shoots. Moreover, it was observed by Tsvetkov et al. [47] that storage efficiencies of encapsulated micro cuttings could be improved by the addition of nutritive ingredients during storage, as during long-term storage, encapsulated cuttings require a continuous supply of mineral nutrients to store their energy reserves for the cell developmental process on sowing after storage. In our studies, since encapsulated cuttings were subjected to cold storage at 4°C, perhaps low temperature ceased the growth activities of the encapsulated cutting [48]. Hence, the most important and evident factor in reducing the shoot length of plantlets after different storage intervals could be the temperature at which synthetic seeds were placed. Previous results revealed that a temperature of 20°C is better for preservation as reported by Maruyama et al. [41] and Nassar [48]. Low temperature of 5–15°C is not suitable for synthetic seed as it loses viability, leading to poor shoot length [49].
Many crops are cold sensitive and hence lead to the failure of the shoots like in the tomato crop observed by Porter [42]. Therefore, the most probable reason for reduced shoot length during long-term storage could be the storage temperature, which was 4°C, and it might cause physical dysfunction in synthetic seeds at this temperature [48]. Cold sensitivity is the premeiotic DNA synthesis or G2-early leptotene, chiasmata are formed, an event during cold storage predetermines whether the chiasmata were formed or not [50]. Conversion of starch to sugar after exposure to the low temperature for longer period may cause the plant to undergo the phenomenon of low-temperature sweetening (LTS), causing reduction in shoot length [51,52]. Moreover, Son et al. [53] reported that starch degrading activities by starch degrading enzymes, including α-amylase, iso-amylase, β-amylase, α-glucosidase, and phosphorylase could be noticed after exposure to the cold storage. With the increase in storage interval, all reserves in cells are used in respiration, so there is a lack of energy reserves which might be the factor for a decrease in the number of leaves and nodes. Ding et al. [54] stated that with increasing storage temperature and storage interval, carbohydrates declined rapidly. Hence it can be recognized as a key declining factor for a smaller number of leaves and nodes. Certain physiological changes occur within the cells of the encapsulated cutting at low temperatures that reroutes towards the development of a smaller number of leaves and shoots. At a low-temperature, direct binding of the polyamines to the membranes, prevents lipid peroxidation and proteolytic attack and slows down ethylene synthesis through inhibition of 1-aminocyclopropane-l-carboxylic acid (ACC) synthase by restricting the accumulation of ACC-synthase transcripts leading towards improper metabolic activities [55].
Starch degradation during long-term storage at low temperatures could be highlighted for the reduction in the number of leaves and nodes. As it was reported by Sowokinos et al. [56] and Soon et al. [57] that starch inside the cells starts mortifying by starch-degrading enzymes (α-amylase, isoamylase, β-amylase, α-glucosidase, and phosphorylase) directed towards less starch accumulation and hence less biomass production for developing a greater number of leaves and nodes. Restricted metabolic activities during storage could also be outlined for this reduction in the number of leaves and nodes. During cold storage, enzyme induction and the massive transfer of reaction substrates and products in and out of subcellular organelles could be reasoned for control of metabolic responses inside the cells [58]. Degradation of RNA had occurred during storage, leading towards reduced biological activities and disrupting the cell cycle, hence, less photosynthetic activities. Low yields of RNA inside the tissues of encapsulated cuttings are liable to necrosis [42]. Long-term cold storage accumulates unidentified chemical, causing local disturbance in the cambium connection, which may result from growth suspension [53]. All of these can be focused on less number of leaves after long-term storage. Long-term storage could be made successful by the application of carbon source, such as sugar provided in endosperm solution focused. Earlier, it was observed by Agarwal et al. [59] that carbon sources play an important role in various biological functions. It acts as a growth-promoting factor and induces secondary embryogenesis by providing osmotic stress but inhibits the chlorophyll content, and hence, reduces photosynthesis. Less photosynthetic activity hence produces less number of leaves and nodes after long-term storage at low temperature. These results suggest that nutrient loss from beads with increasing storage interval might be the cause for poor root development. This supposition agrees with the findings of West et al. [43], who stated that an increase in storage time resulted in the exhaustion of nutrients from the alginate matrix that decreases regeneration quality.
The most plausible reason for the good rooting percentage in the control treatment was that synthetic seeds were sown just after preparation without any storage treatment, as demonstrated by Porter [42] in tomato crops. The effect of long-term storage can be improved by the addition of charcoal and fungicide (TM) to obtain maximum roots. The darkening effect of media enhances rooting; considering this key factor, the encapsulated media was provided with charcoal which, in collaboration with other constituents, increase the porosity of the alginate matrix that provides more aeration, and also provides the dark effect for root growth [60]. The beneficial effect of activated charcoal on plantlets regenerated from synthetic seed may also be attributable to their ability to absorb unwanted exudes such as 5-hydroxymenthylfurfural (a toxic breakdown product of sucrose formed during autoclaving) and other harmful phenolic oxidation products [41]. The oxidation process is essential and helpful for the rooting process. Besides this Kumar et al. [39] found that the inclusion of activated charcoal in the synthetic endosperm increased the diffusion of gases, nutrients, and respiration which enhanced the germination and conversion of synthetic seeds to the maximum extent.
Previous studies on synthetic seeds, in general, showed that cold storage favored rooting as better root length might be due to its effect on alterations in the biosynthesis, transport, and catabolism of IAA [61]. These reports are consistent with our findings and imply up to a certain extent, i.e., 45 days, after which discrepancies from these early reports were found in our results. The possible reason for this diversion in results could be the sensitivity of the encapsulated cuttings for cold storage because different crops perform differently for the storage interval and storage condition. Results in this study are consistent with the findings of Porter [42] on tomato crop for its performance after storage interval. These results support our results in this regard that both potato and tomato are closely related crops belonging to the same family (Solanaceae) having very close genera therefore the same effect could be obtained in both after different storage intervals. Hence, it is concluded that the effect of storage interval is specie specific, and our findings could not match with the results of previous studies obtained in woody species [62].
Storage intervals are supposed to affect the rooting percentage of synthetic seeds as Machii et al. [63] elucidated that 30 days of storage interval at 4°C gave better root development in the synthetic seed of mulberry, but potato remained less responsive for storage intervals because with the increase in the number of days rooting percentage frequency was lowered. For better response and to obtain success in synthetic seeds, certain treatments worked out like along with storage interval and condition, pre-encapsulation treatment of cuttings with IBA was practiced, which gave favorable results; however, the frequency of root development although remained at a lower level in synthetic seeds stored for a longer time period (60 and 75 days) even after pretreatment. Because of this IBA treatment remains successful for a certain time limit, i.e., for 45 days, after which the rooting percentage dropped down but did not lose completely. Haq et al. [64] stated that IBA increased cambial growth at the base of micro cuttings resulting in the differentiation of root primordial and ultimately leading towards maximum rooting percentage. Results obtained in this study are in line with the work of West et al. [43] who reported that as storage time increases root length of plantlets derived from synthetic seed decreased in a linear fashion. Maximum root length in control treatment could be correlated with IBA applied for root induction. Once root primordia develop in cuttings after which, remarkable metabolic activities of tissues start leading toward root growth. In these activities particularly peroxidase (PER) enzyme in the cells catalyze the lignification in the cell wall leading to cell elongation, an evident process during rooting [65]. Furthermore, this can also be mentioned here that the addition of nutrients (sugar) in artificial endosperm solution used for coating the cuttings also positively affected the root length as it serves as an external source of energy used for root development and elongation.
The significant reduction in root length and the number of roots can be associated with the loss of nutrients from the encapsulation media by seeping them into the external substratum. Husen et al. [66] stated that sugars are utilized during root growth, which is indicated by the loss of carbohydrates from the rooting zone of cuttings and, therefore, exogenous application of nutrients was considered a must for further increase in root length. Moreover, this was documented by Gardi et al. [29] that supplemental nutrients are required for encapsulated cuttings because of the reduced photosynthesis and increased respiration. Aeschabacher et al. [67] found that the formation of adventitious roots is a high energy-requiring process involving cell division causing predetermined cells to switch from their morphogenetic path to act as mother cells for the root primordia development. Encapsulated cuttings are the living bodies detached from plants undergoing metabolic activities in cells without any stop and hence lead towards loss of storage carbohydrates after some time. But the elongation process cannot be completed without the availability of energy in the form of storage reserves, therefore, decreased root length. An increase in the level of putrescine in cells in response to polyamines could be reasoned for less root development [68]. The reason for the reduction in root length with an increase in the number of days could be attributed to the loss of viability of encapsulated cuttings as storage interval increases. Moreover, Haissig [69] articulated that root primordial initiation endorsed the carbohydrate reserves in the micro cuttings, which are the principal source of energy for its formation. Lack of reserves and loss of viability of the encapsulated cuttings might be the driving factor for the decrease in root length.
Cell division and cell enlargement are the indicative features during root development and growth and are supposed to trigger tissue sensitivity for root formation and elongation. However, cell division and cell elongation are both high energy-requiring processes, as reported by Thrope [70]. Similarly, Custodio et al. [71] also reported that root formation and growth is a high energy-demanding process. Encapsulated cuttings excised from the plant have no contact with the plant, which is a source of energy, so for long-term storage, exogenous energy is needed to retain its condition. As it was obvious from the previous works that, root formation, and elongation is an energy-requiring process, while encapsulated cuttings are deprived of sufficient energy [72–76]. So, the addition of nutrients is required with the passage of time; nutrients deplete from the alginate matrix, hence, there is less root elongation. Media used in this study was not provided with any plant growth regulator except sucrose, but previous studies revealed that sucrose induces osmotic stress and has no effect on root growth. Hence it can be concluded that the unavailability of the energy-providing compound during the storage interval leads to poor root growth and less root length.
This study provided evidence that contamination of synthetic seeds could be controlled by using fungicides such as PPM, carbendazine, and TM. The recommended optimum level of TM for better growth and viability of synthetic seeds is 150 mg/L. Hence it is recommended that Sodium alginate (3.5%) + charcoal (1.25%) + fungicide (thiophanate methyl, 150 mg/L) should be used to get higher viability percentage, more number of shoots and maximum shoot length as well as other study traits. Scatterplot showed a positive relationship among the number of roots and shoots in response to different storage intervals while negative under different fungicides treatments. Hence optimum dose of fungicides should be used to get a better outcome for synthetic potato seed production.
Acknowledgement: This research is part of the thesis work of the first author, who is thankful to the Department of Horticulture Tissue Culture Laboratory, PMAS-Arid Agriculture University, Rawalpindi, Pakistan, for providing the resources and facilities to carry out this research.
Funding Statement: The authors received no specific funding for this study.
Author Contributions: The authors confirm their contribution to the paper as follows: study conception and design: T.K. and T.A.; data collection: T.K.; analysis and interpretation of results: T.K. and M.A.; draft manuscript preparation: T.K., T.A., M.A.K., M.H., M.A., and S.W. All authors reviewed the results and approved the final version of the manuscript.
Availability of Data and Materials: The datasets generated during and/or analyzed during the current study are available from the corresponding author on reasonable request.
Conflicts of Interest: The authors declare that they have no conflicts of interest to report regarding the present study.
References
1. FAO (2004). Low cost options for tissue culture technology in developing countries. Proceedings of a Technical Meeting, Joint FAO/IAEA Division of Nuclear Techniques in Food and Agriculture, Vienna, Austria. [Google Scholar]
2. Singh, J., Kaur, L., Moughen, P. J. (2012). Importance of chemistry technology and nutrition in potato processing. Food Chemistry, 133(4), 1091. https://doi.org/10.1016/j.foodchem.2012.02.128 [Google Scholar] [CrossRef]
3. Arun, K. B., Chandran, J., Dhanya, R., Krishna, P., Jayamurthy, P. et al. (2015). A comparative evaluation of antioxidants and antidiabetic potential of peel from young and matured potato. Food Bioscience, 9(4), 36–46. https://doi.org/10.1016/j.fbio.2014.10.003 [Google Scholar] [CrossRef]
4. Chung, I. M., Kim, J. K., Jin, Y. I., Oh, Y. T., Prabakaran, M. et al. (2016). Discriminative study of potato (Solanum tuberosum L.) cultivation region by measuring the stable isotope ratios of bioelements. Food Chemistry, 212, 48–57. https://doi.org/10.1016/j.foodchem.2016.05.161 [Google Scholar] [PubMed] [CrossRef]
5. Struik, P. C., Wiersema, S. G. (1999). Seed potato technology. Wageningen: Wageningen Pers. [Google Scholar]
6. Badoni, A., Chauhan, J. S. (2010). Conventional vis-a-vis biotechnological methods of propagation in potato: A review. Stem Cell, 1(1), 1–6. [Google Scholar]
7. Muthoni, J., Kabira, J. (2014). Multiplication of seed potatoes in a conventional potato breeding programme: A case of Kenya’s national potato programme. Australian Journal of Crop Science, 8(8), 1195–1199. [Google Scholar]
8. Khurana, S. M. P., Minhas, J. S., Pandey, S. K. (2003). The potato: Production and utilization in subtropics. New Delhi, India: Mehta Publishers. [Google Scholar]
9. Naik, P. S., Buckseth, T. (2018). Recent advances in virus elimination and tissue culture for quality potato seed production. In: Gosal, S., Wani, S. (Eds.Biotechnologies of crop improvement, vol. 1, pp. 31–158. Cham: Springer. https://doi.org/10.1007/978-3-319-78283-6_4 [Google Scholar] [CrossRef]
10. Altaf, H., Iqbal Ahmed, Q., Hummera, N., Ikram, U. (2012). Plant tissue culture: Current status and opportunities. In: Annarita, L., Laura, M. R. R. (Eds.Recent advances in plant in vitro culture. Rijeka: IntechOpen. https://doi.org/10.5772/50568 [Google Scholar] [CrossRef]
11. Espinosa-Leal, C. A., Puente-Garza, C. A., García-Lara, S. (2018). In vitro plant tissue culture: Means for production of biological active compounds. Planta, 248(1), 1–18. https://doi.org/10.1007/s00425-018-2910-1 [Google Scholar] [PubMed] [CrossRef]
12. Rubio, L., Galipienso, L., Ferriol, I. (2020). Detection of plant viruses and disease management: Relevance of genetic diversity and evolution. Frontiers in Plant Science, 11, 320. https://doi.org/10.3389/fpls.2020.01092 [Google Scholar] [PubMed] [CrossRef]
13. Ahmed, M., Fatima, Z., Iqbal, P., Kalsoom, T., Abbasi, K. S. et al. (2020). Potato modeling. In: Ahmed, M. (Ed.Systems modeling, pp. 83–401. Singapore: Springer Singapore. https://doi.org/10.1007/978-981-15-4728-7_14 [Google Scholar] [CrossRef]
14. Saiprasad, G. V. S. (2007). Artificial seeds and their applications. American Journal of Plant Sciences, 6(5), 39–47. https://doi.org/10.1007/BF02839082 [Google Scholar] [CrossRef]
15. Nyende, A. B., Schittenhelm, S., Mix-Wagner, G., Greef, J. M. (2004). Production storability, and regeneration of shoot tips of potato (Solanum tuberosum) encapsulated in calcium alginate hollow beads. In Vitro Cellular & Developmental Biology–Plant, 540–544. https://doi.org/10.1079/IVP2003442 [Google Scholar] [CrossRef]
16. Joonseol, L., Minam, C., Byeongchoon, J., Youngsup, A., Hagsin, K. et al. (2007). Establishment of mass propagation system of virus free sweet potato plants and conservation. Korean Journal of Crop Science, 52(2), 220–227. [Google Scholar]
17. Steel, R., Torrie, J. (1980). Principles and procedures of statistics, 2nd edition New York: McGraw-Hill Book Co. [Google Scholar]
18. Antonietta, G. M., Ahmed, H. I., Maurizio, M., Alvaro, S. (2007). Preliminary research on conversion of encapsulated somatic embryo of Citrus reticulate Blanco, cv. Mandarino Tardivo di Ciaculli. Plant Cell, Tissue and Organ Culture, 88(1), 117–120. https://doi.org/10.1007/s11240-006-9185-0 [Google Scholar] [CrossRef]
19. Mamiya, K., Sakamoto, Y. (2001). Effect of sugar concentration and strength of basal medium on conversion of somatic embryos in Asparagus officinalis L. Scientia Horticulturae, 84(1–2), 15–26. https://doi.org/10.1016/S0304-4238(99)00098-9 [Google Scholar] [CrossRef]
20. Yugar, E. W. S., Vesco, L. L. D., Steinmacher, D. A., Stolf, E. C., Guerra, M. P. (2009). Microshoot encapsulation and plant conservation of Musa sp. cv. Grand naine. Ciencia Rural Santa Maria, 39(4), 998–1004. https://doi.org/10.1590/S0103-84782009005000024 [Google Scholar] [CrossRef]
21. Tomlin, C. D. S. (1994). The pesticide manual world compendium, 10th editionSurrey, UK: The British Crop Protec. Council. [Google Scholar]
22. Matheron, M. (2001). Modes of action of plant disease management chemistries. 11th Annual Desert Vegetable Crop Workshop. https://cals.arizona.edu/crop/diseases/papers/dischemistry.html [Google Scholar]
23. Ferreira, E. M., Alfenas, A. C., Maffia, L. A., Mafia, R. G., Mounteerc, A. H. (2008). Effectiveness of systemic fungicides in the control of Quambalaria eucalypti and their effects on production of eucalypt mini-cuttings for rooting. Crop Protection, 27(2), 161–170. https://doi.org/10.1016/j.cropro.2007.05.001 [Google Scholar] [CrossRef]
24. Ganapathi, T. R., Srinivas, L., Suprasanna, P., Bapat, V. A. (2001). Regeneration of plants from alginate-encapsulated somatic embryos of banana cv. Rasthali (Musa SPP. AAB Group). In vitro Cellular & Developmental Biology–Plant, 37, 178–181. https://doi.org/10.1007/s11627-001-0031-0 [Google Scholar] [CrossRef]
25. Micheli, M., Standardi, A. (2002). Preliminary studies on the synthetic seed encapsulation technologies of vitro-derived olive explants. Acta Horticulturae, 586, 911–914. https://doi.org/10.17660/ActaHortic.2002.586.199 [Google Scholar] [CrossRef]
26. George, M. W., Tripepi, R. R. (1999). Plant Preservtive Mixture (PPM) can reduce shoot regeneration from leaf explants of selected plants. HortScience, 34(3), 455E–455. https://doi.org/10.21273/HORTSCI.34.3.455E [Google Scholar] [CrossRef]
27. Ruzic, D., Vujovic, T., Milenkovic, S., Cerovic, R., Miletic, R. (2008). The influence of Imidazole fungicides on multiplication in vitro of dwarf pear rootstock. Australian Journal of Crop Science, 1, 63–68. [Google Scholar]
28. Perocco, P., Ciello, C. D., Mazullo, M., Rocchi, P., Ferreri, A. M. et al. (1997). Cytotoxic and cell transforming activities of the fungicide methyl thiophanate on BALB/C 3T3 cells in vitro. Mutation Research/Genetic Toxicology and Environmental Mutagenesis, 394(1), 29–35. https://doi.org/10.1016/S1383-5718(97)00120-4 [Google Scholar] [PubMed] [CrossRef]
29. Gardi, T., Piccioni, E., Standardi, A. (1999). Effect of bead nutrient composition on regrowth of stored in vitro-derived encapsulated microcuttings of different woody species. Journal of Microencapsulation, 16, 13–25. https://doi.org/10.1080/026520499289275 [Google Scholar] [PubMed] [CrossRef]
30. Naik, S. K., Chand, P. K. (2006). Nutrient-alginate encapsulation of in vitro nodal segments of Pomegranate (Punica granatum L.) for germplasm distribution and exchange. Scientia Horticulturae, 108(3), 247–252. https://doi.org/10.1016/j.scienta.2006.01.030 [Google Scholar] [CrossRef]
31. Negi, S., Ivanchenko, M. G., Muday, G. K. (2008). Ethylene regulates lateral root formation and auxin transport in Arabidopsis thaliana. The Plant Journal, 55, 175–187. https://doi.org/10.1111/j.1365-313X.2008.03495.x [Google Scholar] [PubMed] [CrossRef]
32. Ballester, A., Janeiro, L. V., Vieitez, A. M. (1997). Cold storage of shoot cultures and alginate encapsulation of shoot tips of Camellia japonica L. and Camellia reticulata Lindley. Scientia Horticulturae, 71(1–2), 67–78. https://doi.org/10.1016/S0304-4238(97)00074-5 [Google Scholar] [CrossRef]
33. Jouve, L., Jacques, D., Douglas, G. C., Hoffmann, L., Hausman, J. F. (2007). Biochemical characterization of early and late bud flushing in common ash (Fraxinus excelsior L.). Plant Science, 172(5), 962–969. https://doi.org/10.1016/j.plantsci.2007.02.008 [Google Scholar] [CrossRef]
34. Shen, W., Kazuyoshi, N., Tachibana, S. (2000). Involvement of polyamines in the chilling tolerance of cucumber cultivar. Plant Physiology, 124(1), 431–439. https://doi.org/10.1104/pp.124.1.431 [Google Scholar] [PubMed] [CrossRef]
35. He, L., Nada, K., Tachibana, S. (2002). Effect of spd pretreatment through the roots on growth and photosynthesis of chilled cucumber plants (Cucumus sativus L.). Journal of the Japanese Society for Horticultural Science, 71, 490–498. [Google Scholar]
36. Jeon, M. G., Jo, H., Han, T. J. (1998). Effect of polyamines on adventitious root formation from tobacco (Nicotina tobacum) leaf segment. Journal of Plant Biology, 41(1), 31–36. https://doi.org/10.1007/BF03030470 [Google Scholar] [CrossRef]
37. Besford, R. T., Richardson, C. M., Campos, J. L., Tiburcio, A. F. (1993). Effects of polyamines on stabilization of molecular complexes in thylakoid membranes of osmotically stressed oat leaves. Planta, 189(2), 201–206. https://doi.org/10.1007/BF00195077 [Google Scholar] [CrossRef]
38. Singh, A. K., Varshney, R., Sharma, M., Agarwal, S. S., Bansal, K. C. (2005). Regeneration of plants from alginate-encapsulated shoot tips of Withania somnifera (L.) Dunal, a medicinally important plant species. Journal of Plant Physiology, 163(2), 220–223. https://doi.org/10.1016/j.jplph.2005.06.013 [Google Scholar] [PubMed] [CrossRef]
39. Kumar, A. M. B., Vakeswaran, V., Krishnasamy, V. (2005). Enhancement of synthetic seed conversion to seedling in hybrid rice. Plant Cell, Tissue and Organ Culture, 81(1), 97–100. https://doi.org/10.1007/s11240-004-2448-8 [Google Scholar] [CrossRef]
40. Micheli, M., Hafiz, I. A., Standardi, A. (2007). Encapsulation of in vitro-derived explants of olive (Olea europaea L. cv. Moraiolo) II. Effect of storage on capsule and derived shoots performance. Scientia Horticulturae, 113(3), 286–292. https://doi.org/10.1016/j.scienta.2007.04.001 [Google Scholar] [CrossRef]
41. Maruyama, E., Kinoshita, I., Ishii, K., Shigenaga, H., Ohba, K. et al. (1997). Alginate-encapsulation technology for the propagation of the tropical forest trees: Cedrela odorata L., Guazama crinita Mart., Jacaranda mimosaefolia D. Don. Silvae Genetica, 46, 17–23. [Google Scholar]
42. Porter, J. (2008). Analysis of tomato synthetic seeds for the development of an optimized encapsulation system (Graduate Theses, Dissertations, and Problem Reports). West Virginia University, Morgantown,https://researchrepository.wvu.edu/etd/2613 [Google Scholar]
43. West, T. P., Ravindra, M. B., Preece, J. E. (2006). Encapsulation, cold storage, and growth of Hibiscus moscheutos nodal segments. Plant Cell, Tissue and Organ Culture, 87(3), 223–231. https://doi.org/10.1007/s11240-006-9155-6 [Google Scholar] [CrossRef]
44. Patel, A. V., Pusch, I., Mix-Wagner, G., Vorlop, K. D. (2000). A novel encapsulation technique for the production of artificial seeds. Plant Cell Reports, 19(9), 868–874. https://doi.org/10.1007/s002990000223 [Google Scholar] [PubMed] [CrossRef]
45. Rout, G. R., Das, G., Samantaray, S., Das, P. (2001). Micropropagation of Plumbago zeylanica L. by encapsulated nodal explants. The Journal of Horticultural Scince and Biotechnology, 76(1), 24–29. https://doi.org/10.1080/14620316.2001.11511321 [Google Scholar] [CrossRef]
46. Nieves, N., Martinez, M. E., Castillo, R., Blanco, A. M., Olmedo, J. L. G. (2001). Effect of abscisic acid and jasmonic acid on partial desiccation of encapsulated somatic embryos of sugarcane. Plant Cell, Tissue and Organ Culture, 65(1), 15–21. https://doi.org/10.1023/A:1010699532641 [Google Scholar] [CrossRef]
47. Tsvetkov, I., Jouve, L., Hausman, J. F. (2006). Effect of alginate matrix composition on regrowth of in vitro-derived encapsulated apical microcuttings of hybrid aspen. Biologia Plantarum, 50(4), 722–724. https://doi.org/10.1007/s10535-006-0115-0 [Google Scholar] [CrossRef]
48. Nassar, A. H. (2003). Slow growth storage of encapsulated germplasm of Coffea arabica L. International Journal of Agriculture and Biology, 4, 517–520. [Google Scholar]
49. Tyagi, R. K., Goswami, R., Sanayaima, R., Singh, R., Tandon, R. et al. (2009). Micropropagation and slow growth conservation of cardamom (Elettaria cardamomum Maton). In Vitro Cellular & Developmental Biology-Plant, 45(6), 721–729. https://doi.org/10.1007/s11627-009-9234-6 [Google Scholar] [CrossRef]
50. Church, K., Wimber, D. E. (2004). Meiosis in Ornithogalum virens (lilliaceae). II. Univalent production by preprophase cold treatment. Experimental Cell Research, 64(1), 119–124. https://doi.org/10.1016/0014-4827(71)90200-X [Google Scholar] [PubMed] [CrossRef]
51. Rees, T., Dixon, W. L., Pollock, C. J., Franks, F. (1981). Low temperature sweetening of higher plants. In: Recent Advances in the biochemistry of fruits and vegetables, vol. 35, pp. 41–61. New York: Academic Press. [Google Scholar]
52. O’donoghue, E. P., Yada, R. Y., Marangoni, A. G. (1995). Low temperature sweetening in potato tubers: The role of the amyloplast membrane. Journal of Plant Physiology, 145(3), 335–341. https://doi.org/10.1016/S0176-1617(11)81899-7 [Google Scholar] [CrossRef]
53. Son, S. H., Chun, Y. W., Hall, R. B. (1991). Cold storage of in vitro of hybrid poplar shoots (Populus alba L. x P. gandidenata Michx.). Plant Cell, Tissue and Organ Culture, 27(2), 161–168. https://doi.org/10.1007/BF00041285 [Google Scholar] [CrossRef]
54. Ding, C. K., Chachin, K., Hamauzi, Y., Ueda, Y. (1998). Effect of storage temperature and physiology and quality of loquot fruit. Postharvest Biology and Technology, 14, 300–315. [Google Scholar]
55. Lee, T. M., Lur, H. S., Chu, C. (1995). Abscisic acid and put accumulation in chilling tolerant rice cultivars. Crop Science, 35(2), 502–508. https://doi.org/10.2135/cropsci1995.0011183X003500020037x [Google Scholar] [CrossRef]
56. Sowokinos, J. R., Varns, J. L. (1992). Induction of sucrose synthase in potato tissue culture: Effect of carbon source and metabolic regulators on sink strength. Journal of Plant Physiology, 139(6), 672–679. https://doi.org/10.1016/S0176-1617(11)81709-8 [Google Scholar] [CrossRef]
57. Soon, K. H., Heung, J. J., Hwa, C. K., Hee, J. Y., Mil, L. B. et al. (1997). Change of starch and sugar contents and activity of sucrose synthase during microtuberization in potato. Journal of Korean Society of Horticulture Science, 38, 211–215. [Google Scholar]
58. Bonnier, F. J. M., Keller, J., Tuyl, J. M. V. (1992). Conductivity and potassium leakage as indicators for viability of vegetative materials of lily, onion and tulip. Acta Horticulturae, 325(325), 643–647. https://doi.org/10.17660/ActaHortic.1992.325.91 [Google Scholar] [CrossRef]
59. Agarwal, S., Kanwar, K., Sharma, D. R. (2004). Factors affecting secondary somatic embryogenesis and embryo maturation in Morus alba L. Scientia Horticulturae, 102(3), 359–368. https://doi.org/10.1016/j.scienta.2004.04.002 [Google Scholar] [CrossRef]
60. Thomas, T. D. (2008). The role of activated charcoal in plant tissue culture. Biotechnology Advances, 29(6), 618–631. https://doi.org/10.1016/j.biotechadv.2008.08.003 [Google Scholar] [PubMed] [CrossRef]
61. Garrido, G., Arnao, M. B., Acosta, M., Bravo, J. S. (2003). Polar transport of indole 3-acetic acid in relation to rooting in carnation cuttings: Influence of cold storage duration and cultivar. Biologia Plantarum, 47(4), 481–485. https://doi.org/10.1023/B:BIOP.0000041050.41513.da [Google Scholar] [CrossRef]
62. Pattnaik, S. P., Chand, K. (2000). Morphogenic response of the alginate-encapsulated axillary buds from in vitro shoot cultures of six mulberries. Plant Cell, Tissue and Organ Culture, 60(3), 177–185. https://doi.org/10.1023/A:1006424626433 [Google Scholar] [CrossRef]
63. Machii, H., Yamanouchi, H. (1993). Growth of mulberry synthetic seeds on vermiculite sand and soil media. The Journal of Sericultural Science of Japan, 62, 85–87. [Google Scholar]
64. Haq, I., Ahmad, T., Hafiz, I. A., Abbasi, N. A. (2009). Influence of microcutting sizes and IBA concenteration on In vitro rooting of olive cv. ‘Dolce agogia’. Pakistan Journal of Botany, 41(3), 1213–1222. [Google Scholar]
65. Sato, Y., Sugiyama, M., Gorecki, R. J., Fukuda, H., Komamine, A. (1993). Interrelationship between lignin deposition and the activities of peroxidase isoenzymes in differentiating tracheary elements of Zinnia. Planta, 189(4), 584–589. https://doi.org/10.1007/BF00198223 [Google Scholar] [CrossRef]
66. Husen, A., Pal, M. (2007). Metabolic changes during adventitious root primordium development in Tectona grandis Linn. f. (teak) cuttings as affected by the age of donor plants and auxins (IBA and NAA) treatment. New Forests, 33(3), 309–323. https://doi.org/10.1007/s11056-006-9030-7 [Google Scholar] [CrossRef]
67. Aeschbacher, R. A., Schiefelbein, J. W., Benfey, P. N. (1994). The genetic and molecular basis of root development. Annual Review of Plant Physiology and Plant Molecular Biology, 45(1), 25–45. https://doi.org/10.1146/annurev.pp.45.060194.000325 [Google Scholar] [CrossRef]
68. Baraldi, R., Bertazza, G., Bregoli, A. M., Fasolo, F., Rotondi, A. et al. (1995). Auxins and polyamines in relation to differential in vitro root induction on microcuttings of two pear cultivars. Journal of Plant Growth Regulator, 14(1), 49–59. https://doi.org/10.1007/BF00212646 [Google Scholar] [CrossRef]
69. Haissig, B. E. (1982). Activity of some glycolytic and pentose phosphate pathway enzymes during the development of adventitious roots. Physiologia Plantarum, 55(3), 261–272. https://doi.org/10.1111/j.1399-3054.1982.tb00290.x [Google Scholar] [CrossRef]
70. Thrope, T. A. (1982). Carbohydrate utilization and metabolism. In: Bonga, J. M., Durzan, D. J. (Eds.Tissue culture in foretsry, vol. 25, pp. 325–368. Dordreeht, Netherlands, Wageningen: Martinus Nijhoff Publi. [Google Scholar]
71. Custodio, L., Loucao, M. A., Romano, A. (2004). Influence of sugar on in vitro rooting and acclimatization of crob tree. Biologia Plantarum, 48(3), 469–506. https://doi.org/10.1023/B:BIOP.0000041107.23191.8c [Google Scholar] [CrossRef]
72. Rihan, H. Z., Kareem, F., El-Mahrouk, M. E., Fuller, M. P. (2017). Artificial seeds (principle, aspects and applications). Agronomy, 7(4), 71. https://doi.org/10.3390/agronomy7040071 [Google Scholar] [CrossRef]
73. Sarkar, D., Naik, P. S. (1998). Synseed in potato: An investigation using nutrient-encapsulated in vitro nodal segments. Scientia Horticulturae, 73(2–3), 179–184. https://doi.org/10.1016/S0304-4238(97)00146-5 [Google Scholar] [CrossRef]
74. Standardi, A., Piccioni, E. (1998). Recent perspectives on synthetic seed technology using nonembryogenic in vitro-derived explants. International Journal of Plant Sciences, 159(6), 968–978. https://doi.org/10.1086/314087 [Google Scholar] [CrossRef]
75. Vinterhalter, D., Vinterhalter, B., Calovic, M. (1997). The relationship between sucrose and cytokinins in the regulation of growth and branching in potato cv. Desiree shoot culture. Acta Horticulturae, 462, 319–323. https://doi.org/10.17660/ActaHortic.1997.462.46 [Google Scholar] [CrossRef]
76. Hasnat, R., Akhtar, N., Ahmad, T., Hafiz, I. A. (2007). Induction and regeneration of hypocotyls derived calli in hot chilli (Capsicum frutescens L.) varieties. Pakistan Journal of Botany, 39(5), 1787–1795. [Google Scholar]
Cite This Article
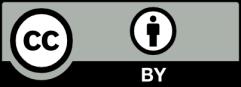
This work is licensed under a Creative Commons Attribution 4.0 International License , which permits unrestricted use, distribution, and reproduction in any medium, provided the original work is properly cited.