Open Access
ARTICLE
Effects of Manganese on the Antioxidant System and Related Gene Expression Levels in the “Hong Yang” Kiwifruit Seedlings
1 Key Laboratory of Plant Resource Conservation and Germplasm Innovation in Mountainous Region (Ministry of Education), Institute of Agro-Bioengineering, College of Life Sciences, Guizhou University, Guiyang, 550025, China
2 Institute of Mountain Resource, Guizhou Academy of Sciences, Guiyang, 550001, China
* Corresponding Authors: Weijie Li. Email: ; Xiaopeng Wen. Email:
(This article belongs to the Special Issue: New Approaches to Mitigate Abiotic and Biotic Stresses for Improving Crop Productivity and Quality)
Phyton-International Journal of Experimental Botany 2023, 92(8), 2399-2412. https://doi.org/10.32604/phyton.2023.029891
Received 14 March 2023; Accepted 27 April 2023; Issue published 25 June 2023
Abstract
To explore how manganese affects the antioxidant system and the expression levels of related genes of “Hong yang” seedlings, the leaves of its tissue cultured seedlings were taken as test materials, and single factor treatment was performed by changing the manganese chloride (MnCl2.4H2O) solution concentration when spraying the leaves. The expression levels of Mn-SOD, POD64 and POD27 genes in leaves were quantitatively analyzed by real-time quantitative PCR (qRT-PCR) at different determination times. Meanwhile, the contents of malondialdehyde (MDA), hydrogen peroxide (H2O2), the activities of antioxidant enzymes, including catalase (CAT), peroxidase (POD), and superoxide dismutase (SOD). The results showed that the SOD, CAT, POD, ascorbate peroxidase (APX), and reduced glutathione (GSH) activities in leaves were the highest at 12 h post-treatment with 50 μM MnCl2·4H2O. Furthermore, the contents of MDA and H2O2 in leaves also peaked when the concentration of H2O2 is 50 μM, which is the minimum value. Additionally at 50 μM Mn2+, the Mn-SOD and POD27 expression was up-regulated as compared to the control, which promoted the expression of their respective enzyme activities. However, POD64 expression increased with the increasing Mn2+ concentration. Therefore, 50 μM is the optimal concentration of Mn when exogenously applied on “Hong yang”, which improve the antioxidant enzyme activity and regulate the plant’s physiological and biochemical functions.
Keywords
Although manganese (Mn) is one of the essential trace metal elements for plants [1], it is needed in small quantities [2]. Mn deficiency in plants leads to low efficiency of their antioxidant system and chlorophyll degradation [3], with Mn being irreplaceable in plant photosynthetic oxygen release, maintaining normal organellar structure, and activating enzyme activities.
“Hong yang” is a unique red flesh kiwi variety in China, with a large neatly shaped fruit that was tender, delicious, and has a small coefficient of variation [4]. Although its market demand increasing, its production is plagued by some problems, including weak growth, concave fruit top, and easy-to-bruise fruit peel [5]. In order to solve the related problems, people mostly through the increase of different organic fertilizers, while the increase of fertilizer before and after the kiwifruit leaf nutrition and fruit quality of the corresponding determination and comparative analysis, to screen out the best treatment method to improve the kiwifruit leaf nutrition and fruit quality [6,7].
Another reason is that copper (Cu), zinc (Zn), manganese (Mn), nickel (Ni), and iron (Fe) are essential metal elements, and they can serve as co-factors of enzymes that are involved in important regulatory processes of many organisms [8]. One study [9] found that the Fe-application on soil and foliage during drought stress can increase the plant’s enzymatic antioxidant activity. This test shows that antioxidant levels of essential metals can raise enzyme activity in plants. At the same time, related studies on grapes [10] and peaches [11] have found that exogenously applying the trace element Mn on their roots can promote fruit growth, nutrient balance, and improve fruit quality. Therefore, studying the effect of Mn on the physiological and biochemical functions of plants is important. Currently, existing studies on the effects of Mn on the physiological and biochemical functions of plants have mainly focused on manganese tolerant plants, including soybean [12], paper mulberry [13], Masson’s pine [14], etc. Furthermore, very few reports have studied the effects of Mn on the antioxidant system of kiwi seedlings. To explore the effects of different Mn concentrations on the antioxidant system of kiwi seedlings, it is important to select the appropriate concentration.
In this study, the “Hong yang” seedlings were taken as the study material. With water taken as the control, Mn concentration gradients were set as different treatments, followed by evaluating the antioxidant system related gene expression in “Hong yang” leaves under different treatments. Furthermore, the determination time was also modulated to verify the sustained effect of the treatment. Thus, the optimal treatment concentration formula and spraying time of antioxidant enzymes in the leaves of “Hong yang” seedlings under the action of exogenous Mn were selected. Therefore, this study can provide a theoretical basis for the rational and effective application of Mn fertilizer, or the use of exogenous substances Mn to improve the activity of antioxidant enzymes in plants, regulate the physiological and biochemical functions of plants, optimize their fruit quality, and enhance their stress resistance.
The shoots, stems and leaves of 5-year-old “Hong yang” kiwifruit were selected for tissue culture, and the “Hong yang” seedlings obtained by tissue culture were taken as the experimental material. The explants were collected from the kiwifruit production-study-research Base (E:104°57’58.48"E; N:26°24’ 55.04"N; 1,100 m above the sea level), in the Shuicheng District, Guizhou Province, China. These were then cultured by the kiwifruit team of Institute of Mountain Resources, Guizhou Academy of Sciences, Guizhou Province, China.
In the experiment, the “Hong yang” seedlings with the same growth potential were selected and moved to the canopy in the field, where the potted matrix had the perlite: vermiculite ratio was 2:1. When the seedlings grew to have six leaves of one heart, relevant treatment was started. During the 21 days of the experiment, MnCl2.4H2O solution was sprayed on the leaf surface of tissue culture seedlings at 9 AM or 4 PM on three sunny days. Field temperature is 25°C ± 2°C and humidity is 80%. Each spraying interval should be at least three days. Five treatments were set up in the experiment, and the treatment concentration gradient was designed and improved according to a previous experimental design [15]: 5, 10, 50, and 100 μM. The water treatment was taken as the control. The amount of each spray was 10 ml until the leaves were fully wetted by hand-held mist sprayer [16]. Treated leaves were sampled and measurements were taken at 6, 12, 24, and 48 h post spraying, with the preserved samples being immediately frozen at −80°C with liquid nitrogen until further use.
2.3 Measuring Items and Methods
2.3.1 Determination of Physiological and Biochemical Indexes
The activities of superoxide dismutase (SOD), peroxidase (POD), catalase (CAT), and ascorbate peroxidase (APX), along with reduced glutathione (GSH), hydrogen peroxide (H2O2) and malondialdehyde (MDA) contents were determined in the leaves to study the effects of Mn2+ on the antioxidant system of leaves of “Hong yang” tissue culture seedlings. POD activity was determined by the guaiacol oxidation method [17]. CAT activity was determined using visible light spectrophotometry [18]. The SOD activity was determined by the nitrogen blue tetrazole (NBT) photoreduction method [19]. The APX activity was determined by determining the oxidation rate of ascorbic acid [20]. The GSH content was determined using 2-nitrobenzoic acid (DTNB) [21]. The MDA content was determined by the thiobarbituric acid (TBA) method [22]. The H2O2 content was determined by the titanium salt-based spectrophotometry method [23].
2.3.2 Determination of Relative Gene Expression in Leaves of “Hong Yang” Seedlings Treated with MnCl2.4H2O
The relative expression of the genes was determined using real-time quantitative PCR (qRT-PCR). The reverse transcription product cDNA synthesized from the total RNA of the “Hong yang” seedling leaves was used as the template for qRT-PCR. According to the conserved region sequences of POD27 and POD64 genes of the Mn-SOD and POD gene family members in the kiwifruit genome database, combined with the transcriptome sequencing results of “Hong yang” Kiwifruit, gene-specific PCR primers were designed accordingly (see Table 1), which were then synthesized by Shanghai Weiqing Biotechnology Co., Ltd. (China). Using cDNA as a template and polyubiquitin (UBQ) as the internal reference gene, qRT-PCR was performed.
Microsoft Excel 2010 software was used to process the data, while SPSS16.0 software was used for variance analysis and correlation analysis, with the significance level set at 0.05. The Origin 2018 software was used for plotting.
3.1 Effects of Foliar Spraying MnCl2.4H2O on the Antioxidant System of “Hong Yang” Seedlings
3.1.1 Effect of Foliar Spraying MnCl2.4H2O on the SOD Activity of “Hong Yang” Seedlings
SOD is a widely existing metalloenzyme in organisms. It can catalyze the dismutation of superoxide anions and is vital in the biological antioxidant system. As shown in Table 2, as compared with the control treatment (water spraying), spraying MnCl2.4H2O solution on the leaf can improve the SOD activity of “Hong yang” seedlings. Under the same measurement time, with the increase in the MnCl2.4H2O concentration, the SOD activity of leaves of “Hong yang” seedlings first increased and then decreased. Under 50 μM MnCl2.4H2O, the SOD activity was significantly higher than that of other concentrations, especially, 12 h post treatment, the SOD activity was the highest, with the difference being the most significant. Furthermore, at the same treatment concentration and different determination times, the SOD activity also showed a trend of first increasing and then decreasing, with the SOD activity at 12 h being the highest. In conclusion, the SOD activity of “Hong yang” leaves can be improved by using 50 μM MnCl2.4H2O, with it being the most significant at 12 h post treatment.
3.1.2 Effect of Foliar Spraying MnCl2.4H2O on POD Activity of “Hong Yang” Seedlings
POD catalyzes diverse oxidative reactions involving H2O2, and is closely related to various physiological and biochemical processes, including respiration, photosynthesis, and auxin oxidation. It is vital in the cellular redox metabolism. As shown in Table 3, as compared with the control treatment, foliar spraying with MnCl2.4H2O improved the POD activity of “Hong yang” seedlings. POD activity at 50 μM MnCl2.4H2O was significantly higher than those of the control group and 5 μM treatment groups, with the most significant difference observed at 12 h. However, when MnCl2.4H2O was (≤50 μM), the POD activity in the leaves first increased, and then decreased with the increasing determination time, but it only decreased at 100 μM. With the increasing concentration, the POD activity of the leaves of “Hong yang” showed a trend of first increasing and then decreasing during the same determination time, with its peak being observed at 50 μM at 12 h post treatment. Therefore, MnCl2.4H2O at 50 μM was the most beneficial for improving the POD activity of the “Hong yang” leaves.
3.1.3 Effects of Foliar Spraying MnCl2.4H2O on the CAT Activity of “Hong Yang” Seedlings
CAT assists SOD in removing superoxide free radicals. In plants, the vital coordination between SOD, CAT, and POD maintains the cellular redox homeostasis. Table 4 shows that the CAT activity in the “Hong yang” leaves first increased and then decreased with the increasing MnCl2.4H2O treatment concentration at the same determination time. At 50 μM, the CAT activity of leaves was the highest among all concentrations (p < 0.05). Under different determination times, the CAT activity showed an “N” type trend under control treatment, with the maximum being 4.391 U·g−1 at 24 h post treatment. At 5 μM MnCl2.4H2O, the CAT activity first decreased and then increased, with the minimum value of 3.121 U·g−1 being reached at 12 h post treatment. Although the opposite trend was seen at 10 or 50 μM, the maximum CAT activities were 7.158 and 11.213 U·g−1, respectively, at 12 h post spraying. However, for 100 μM, the CAT activity decreased with the increasing time. Thus, the CAT activity of leaves was significantly affected by the MnCl2.4H2O treatment. Therefore, spraying MnCl2.4H2O at 50 μM can promote and improve the CAT activity, with the effect being best at 12 h after spraying.
3.1.4 Effects of Foliar Spraying of MnCl2.4H2O on the APX Activity of “Hong Yang” Seedlings
APX is a specific peroxidase with ascorbic acid as the electron donor, which directly affects the ascorbic acid content in plants. As one of the most important antioxidant enzymes involved in the metabolism of reactive oxygen species (ROS) in plants, it can remove the damaging H2O2 that accumulated and protect chloroplasts and other cellular components. As can be seen from Table 5, at the same measurement time, with the increasing MnCl2.4H2O concentration, the APX activity in the “Hong yang” leaves first increased and then decreased. The same trend was also seen for the same concentration and different determination times. At 50 μM, the APX activity showed the maximum value, and was significantly higher than the control group (p < 0.05). The APX activity in the leaves also increased first and then decreased when the same concentration of MnCl2.4H2O was sprayed under different. When the treatment concentration was ≤10 μM, the APX activity was the maximum at 24 h post treatment, which when compared with other treatment times, the increase was not significant (p < 0.05). Although when the treatment concentration was (>10 μmol·L−1), the APX activity peaked at 12 h post treatment, which was not significant as compared with other treatment times. Therefore, the APX activity in leaves was significantly affected by MnCl2.4H2O treatment, which significantly differed from the control group. Therefore, spraying 50 μM MnCl2.4H2O can improve the APX activity, with the effect being the best at 12 h post spraying.
3.1.5 Effect of Foliar Spraying of MnCl2.4H2O on the GSH Content of “Hong Yang” Seedlings
GSH is an important antioxidant participating in the ASA-GSH cycle that is vital to abiotic stress tolerance. It helps maintain the plant immune system, with its antioxidative and integrated detoxification effects. Table 6 shows that, at the same determination time, with the increasing MnCl2.4H2O concentration, the GSH content in “Hong yang” first increased and then decreased. At 50 μM, the GSH content was the highest, which significantly differed from the control group. However, under the same concentration at different determination times, although it showed a trend of first increasing and then decreasing, it tended to stabilize and was not significantly different. Therefore, the GSH content of leaves was significantly affected by the MnCl2.4H2O, which was improved by spraying at a concentration of 50 μM, and reached the highest (62.22 µg·g−1) at 12 h after spraying.
3.1.6 Effects of Foliar Spraying of MnCl2.4H2O on the MDA and H2O2 Contents of “Hong Yang” Seedlings
MDA is the end product of membrane lipid peroxidation, which inhibits the cell protective enzymes. Since the MDA content can reflect the stress-induced cellular stability, increased plant MDA content indicates increased plant cell membrane damage. As can be seen from Table 7, within the same determination time, the MDA content in “Hong yang” showed a trend of first decreasing and then increasing with the increasing MnCl2.4H2O treatment concentration, with the minimum observed at 50 μM. Meanwhile, as compared with the control group, the MDA content in the “Hong yang” leaves had significantly decreased by 16.4%, 13.3%, 19.2%, and 24.89%. Under the same treatment concentration of MnCl2.4H2O and different determination times, the MDA content in leaves also showed a trend of first decreasing and then increasing. It can be seen that the MDA content in the leaves is affected by the concentration of MnCl2.4H2O. Foliar spraying of 50 μM MnCl2.4H2O can significantly reduce the MDA content, with the best effect (5.312 nmol.g−1) seen at 12 h post treatment.
H2O2 is the most common reactive oxygen molecule in living organisms and is the hub of mutual transformation of reactive oxygen species. It is mainly generated as a byproduct by SOD and xanthine oxidase (XOD), which is then degraded by CAT and POD. It can not only directly or indirectly oxidize nucleic acid, protein, and other biological macromolecules, but also damage the cell membrane, thereby accelerating cellular aging and disintegration. As can be seen from Table 8, H2O2 content in the leaves of the “Hong yang” seedlings first decreased and then increased with increasing treatment concentration during the same determination time. At 50 μM MnCl2.4H2O, the H2O2 content in leaves was 98.02%, 96.63%, 97.47% and 97.54% of that in control group, and significantly decreased (p < 0.05). Under the same treatment concentration and different determination times, the H2O2 content of leaves also showed a trend of first decreasing and then increasing. When the treatment concentration was ≤10 μM, the minimum H2O2 content was at 24 h post treatment, with the difference not being significant as compared to other determination times. However, when the concentration was >10 μM, the H2O2 content was the minimum at 12 h post treatment, which did not significantly differ from other determination times. Therefore, at the same treatment concentration and the increasing determination times, the H2O2 content was unaffected, as its enzymatic decomposition remained similar irrespective of the increasing determination time post MnCl2.4H2O treatment. Therefore, when the spraying time of MnCl2.4H2O is 12 h, the minimum H2O2 content was 72.167 μmol.g−1 when the spraying concentration was 50 μM.
3.2 Effects of Foliar Spraying of MnCl2.4H2O on the Expression of Antioxidant System-Related Genes in “Hong Yang” Seedlings
3.2.1 3.2.1 Effects of Foliar Spraying of MnCl2.4H2O on Relative Expression Level of Mn-SOD in “Hong Yang” Seedlings
Mn-SOD mainly exists in prokaryotic cells and a few plant cells, where most are located in their mitochondria. Besides chloroplasts, plant mitochondria are the second site producing ROS, with Mn-SOD located in them being vital in clearing ROS. As can be seen from Fig. 1, with the increase in the Mn2+ treatment concentration, the Mn-SOD expression level first increased and then decreased under different determination times. However, when the Mn2+ was >50 μM), the Mn-SOD expression showed a decreasing trend under different determination times. Therefore, with the increasing Mn2+ concentration, the Mn-SOD expression was significantly up-regulated under the same determination time, as compared to the control group. However, when the Mn2+ concentration was too high, it had greater toxicity to the leaves of “Hong yang” seedlings.
Figure 1: Effects of different treatment concentrations and determination time on the relative expression of Mn-SOD in “Hong yang” leaves. Data were expressed as the mean ± SD of three replicates. Values designated over the bars in different letter are significant differences at p < 0.05
At the same concentration of Mn2+, the Mn-SOD expression in the leaves also showed a trend of first increasing and then decreasing with the increasing determination time, while peaking at 12 h post treatment, which was higher than other treatments. Therefore, at 50 μM MnCl2.4H2O and 12 h post treatment, the Mn-SOD expression was the highest. But when the determination time was >12 h, the promoting effect of Mn2+ on the antioxidant system of “Hong yang” seedling leaves would decline, and the effect would be gradually weakened with the extension of time.
3.2.2 Effects of Foliar Spraying with MnCl2.4H2O on Relative Expression Levels of POD27 and POD64 in “Hong Yang” Seedlings
POD27 and POD64 are mainly involved in hydrogen peroxide decomposition, auxin metabolism, lignin synthesis, and stress response in intercellular spaces and vacuoles. Fig. 2 shows that with the increasing Mn2+ concentration, the POD27 expression in leaves first increased and then decreased when the determination time was ≤24 h. However, when Mn2+ concentration ≥50 μM, the expression level of the POD27 expression in leaves showed a decreasing trend at all determination times. Moreover, when the determination time was (>24 h), it showed a downward trend even with the increasing Mn2+ concentration. Therefore, the POD27 expression was the highest when the determination treatment time was 12 h and the Mn2+ concentration was 50 μM.
Figure 2: Effects of different treatment concentrations and determination time on the relative expression of POD27 in “Hong yang” leaves. Data were expressed as mean ± SD of three replicates. Values designated over the bars in different letter are significant differences at p < 0.05
With the increasing Mn2+ concentration, the POD64 expression showed an “M” shaped trend. At the same Mn2+ concentration, it first increased and then decreased with the increasing determination time. However, at 12 h and 50 μM, the POD64 expression level was the highest (see Fig. 3).
Figure 3: Effects of different treatment concentrations and determination time on the relative expression of POD64 in “Hong yang” leaves. Data were expressed as mean ± SD of three replicates. Values designated over the bars in different letter are significant differences at p < 0.05
Plants have a powerful enzymatic antioxidant system, including SOD, POD, and CAT, which coordinate with each other and play an important defense role [24]. They can effectively remove excess ROS, reduce the associated toxicity, and maintain the metabolic balance in plants [25]. They also provide abiotic stress resistance. SOD is an important antioxidant enzyme in plants, and the higher the activity of SOD, the stronger the resistance of plants. The results showed that the activities of SOD, CAT, POD, and APX in the leaves of “Hong yang” seedlings first increased and then decreased with the increasing Mn2+ concentration, which indicated that excess MnCl2.4H2O would cause toxicity, thereby reducing the activities of SOD, POD, CAT, and APX and the GSH content in the “Hong yang” seedlings. The contents of MDA and H2O2 decreased first and then increased with the increase of Mn2+ concentration. One reason is that the SOD protection system is not activated at low Mn concentration [26]. The other is that with the increase of Mn concentration, SOD activity gradually increases, and the maximum value appears at the concentration of 50 μM. The results of this study were consistent with previous results [27], which indicated that the rate of cell oxidation could be effectively reduced when suitable Mn concentration was sprayed on kiwifruit seedlings [28]. However, when MnCl2.4H2O is sprayed with high concentration, lipid composition in membrane and membrane structure will be changed, and the damage of membrane lipid will be aggravated, which is consistent with the results of previous studies [29,30,31]. When different concentrations of MnCl2.4H2O were sprayed on the leaf surface of “Hong yang” seedlings, the expressions of antioxidant system-related genes like Mn-SOD and POD27 first increased and then decreased with the increasing Mn2+ concentration. The reason is that Mn is a cofactor of SOD [32]. Under appropriate concentration, Mn can not only stimulate the activity of plant growth-related enzymes, but also promote plant growth and development, improve the activities of POD and CAT, and produce certain resistance to various abiotic stresses affecting plant growth, playing an important role in defense. At the same time, with higher antioxidant enzyme activity and antioxidant gene expression, lower plasma membrane permeability [33], which plays a crucial role in plant metabolism [34].
In the experiment, it was also found that the activities of SOD, POD, GSH, APX and CAT contents of “Hong yang” seedlings increased first and then decreased with the passing of time, and the maximum value appeared at 12 h, but the overall difference was not significant. The expression level of antioxidant system related gene POD64 changed significantly with the passage of Mn2+ determination time, indicating that Mn would accumulate continuously in kiwifruit seedlings over time, resulting in increased accumulation of ROS in vivo, leading to impaired enzyme activity [35]. It is further verified that when the concentration of Mn in plants is higher than the optimal content in cells, it will have a destructive effect on plants and reduce the vitality of plant cells [36]. It can also cause manganese poisoning [37], which reduces plant quality and, in severe cases, leads to the death of affected plants [38].
Currently, there are very few studies on the effects of Mn treatment on the antioxidant system of plants and their temporal response. This study can provide a theoretical basis for later studies on the effects of trace metal elements on the antioxidant system of plants at different times. However, there are still some shortcomings. Whether these antioxidant activity changes of kiwifruit post Mn2+ treatment can improve the fruit quality, improve plant stress resistance and prevent the invasion of pathogens needs to be studied further.
In this study, different MnCl2.4H2O concentrations were sprayed on the leaf surface of “Hong yang” seedlings. The results showed that although low concentration of MnCl2.4H2O could improve the activities of SOD, POD, and CAT in the leaves, high concentrations could also cause certain damage to the “Hong yang” seedlings. Furthermore, the activities of SOD, POD, and CAT were increased for determination times up to 12 h post spraying, with the expressions of Mn-SOD, POD27, and POD64 related genes in the leaves also being promoted. Therefore, when the exogenous Mn acts on “Hong yang” kiwifruit, the optimal treatment concentration formula of antioxidant enzyme in leaves is 50 μM, which can improve the antioxidant enzyme activity and regulate the physiological and biochemical functions of the plant itself.
Acknowledgement: The authors would like to express their gratitude to EditSprings (https://www.editsprings.cn) for the expert linguistic services provided.
Funding Statement: This work was jointly supported by the following grants: Science and Technology Support Plan of Guizhou Province: Breeding Research and Demonstration of All-Red Bud Transformation of “GH-1” Clone of “Hong Yang” Kiwifruit (Guizhou Family Combination Support [2021] General 234), Innovation Capacity Construction Project of Scientific Research Institutions in Guizhou Province: Technology R&D and Service Capacity Construction of Fine Fruit (Kiwifruit, Passion Fruit) Industry in Guizhou Province [2019]4004, the National Key Research and Development Program “Quality and Efficiency Improvement Technology Integration and Demonstration of Advantageous Characteristic Industries in Guizhou Karst Mountain Area (2021YFD1100300)” Post-Subsidy Fund.
Author Contributions: Weijie Li and Liangliang Li conceived and designed the experiment. Chongpei Zheng and Zhencheng Han collected the test data; Chongpei Zheng analyzed and interpreted the test data. Chongpei Zheng wrote the manuscript. Weijie Li, Xiaopeng Wen, and Liangliang Li guided the article writing. All the authors reviewed the findings and approved the final version of the manuscript.
Conflicts of Interest: The authors declare that they have no conflicts of interest to report regarding the present study.
References
1. Li, M., Zhu, Q., Hu, C. W., Chen, L., Liu, Z. L. et al. (2007). Cobalt and manganese stress in the microalga Pavlova viridis (PrymnesiophyceaeEffects on lipid peroxidation and antioxidant enzymes. Journal of Environmental Sciences, 19(11), 1330–1335. https://doi.org/10.1016/S1001-0742(07)60217-4 [Google Scholar] [PubMed] [CrossRef]
2. Ragab, G., Saad-Allah, K. (2021). Seed priming with greenly synthesized sulfur nanoparticles enhances antioxidative defense machinery and restricts oxidative injury under manganese stress in Helianthus annuus (L.) seedlings. Journal of Plant Growth Regulation, 40, 1894–1902. https://doi.org/10.1007/s00344-020-10240-y [Google Scholar] [CrossRef]
3. Rodrigues, W. S., Pereira, Y. C., de Souza, A. L. M., Batista, B. L., Lobato, A. et al. (2020). Alleviation of oxidative stress induced by 24-epibrassinolide in soybean plants exposed to different manganese supplies: UpRegulation of antioxidant enzymes and maintenance of photosynthetic pigments. Journal of Plant Growth Regulation, 9(4), 1425–1440. https://doi.org/10.1007/s00344-020-10091-7 [Google Scholar] [CrossRef]
4. Long, Q. J., Wu, Y. J., Xie, M. (2010). Tissue culture and rapid micropropagation from leaves and stems of kiwifruit. Acta Agricultural Zhejiang Ensis, 22(4), 429–432. https://kns.cnki.net/kcms/detail/detail.aspx?FileName=ZJNB201004005&DbName=CJFQ2010 [Google Scholar]
5. Li, M. Z., Qiu, L. N., Wang, L. H., Zheng, X. Q., Liao, M. A. (2011). Inheritance trend of main characters in F1 progenies of Hong Yang kiwifruit variety. Journal of Fruit Science, 28(1), 51–54. https://kns.cnki.net/kcms/detail/detail.aspx?FileName=GSKK201101011&DbName=CJFQ2011 [Google Scholar]
6. Pan, L. S., Ren, C. G., Yang, R., Su, W. W., Wu, J. W. et al. (2021). Effects of adding organic fertilizer and bacterial fertilizer on fruit quality of kiwifruit. Non-Wood Forest, 39(2), 140–147. https://kns.cnki.net/kcms/detail/detail.aspx?FileName=JLYJ202102017&DbName=CJFQ2021 [Google Scholar]
7. Wu, D., Wan, J. G., Ren, C. G. (2022). Effects of different organic fertilizers on leaf nutrition and fruit quality of “Hong yang”. Kiwifruit, Agriculture and Technology, 42(24), 12–15. https://kns.cnki.net/kcms/detail/detail.aspx?FileName=NYYS202224003&DbName=CJFQ2022 [Google Scholar]
8. Chaffai, R., Koyama, H. (2011). Heavy metal tolerance in Arabidopsis thaliana. Advances in Botanical Research, 60, 1–49. https://doi.org/10.1016/B978-0-12-385851-1.00001-9 [Google Scholar] [CrossRef]
9. Fathi Amirkhiz, K., Amini Dehaghi, M., Heshmati, S. (2015). Antioxidant enzymes and physiological responses of safflower (Carthamus tinctorius L.) to iron application, under water deficit condition. Notulae Scientia Biologicae, 7(2), 203–209. https://doi.org/10.15835/nsb729456 [Google Scholar] [CrossRef]
10. Chen, H. Z., Deng, X. X., Yang, J., Zhang, Z. W. (2020). Effects of leaf application of manganese on fruit quality of ‘Cabernet Sauvignon’ grape during ripening process before and after color turning stage. Northern Horticulture, 2020(1), 34–41. https://kns.cnki.net/kcms/detail/detail.aspx?FileName=BFYY202001007&DbName=CJFQ2020 [Google Scholar]
11. Feng, L., Wang, Y. N., Yu, J. Z. (2008). Effects of manganese and calcium treatments on storage quality of peaches. Journal of Shanxi Agricultural University (Natural Science Edition), 2008(3), 357–361. https://kns.cnki.net/kcms/detail/detail.aspx?FileName=SXNY200803036&DbName=CJFQ2008 [Google Scholar]
12. Mahjoubi, Y., Rzigui, T., Ben Massoud, M., Kharbech, O., Loussaief, N. et al. (2020). Leaf Gas exchange of bean (Phaseolus vulgaris L.) seedlings subjected to manganese stress. Russian Journal of Plant Physiology, 67(1), 168–174. https://doi.org/10.1134/S1021443720010100 [Google Scholar] [CrossRef]
13. Huang, H. M., Zhao, Y. L., Xu, Z. G., Zhang, W. T., Jiang, K. K. (2019). Physiological responses of Broussonetia papyrifera to manganese stress, a candidate plant for phytoremediation. Ecotoxicology and Environmental Safety, 181, 18–25. https://doi.org/10.1016/j.ecoenv.2019.05.063 [Google Scholar] [PubMed] [CrossRef]
14. Bai, Y., Zhou, Y., Gong, J. (2021). Physiological mechanisms of the tolerance response to manganese stress exhibited by Pinus massoniana, a candidate plant for the phytoremediation of Mn-contaminated soil. Environmental Science and Pollution Research, 28(33), 45422–45433. https://doi.org/10.1007/s11356-021-13912-8 [Google Scholar] [PubMed] [CrossRef]
15. Mi, Y. Fa, Huo, Y. J., Cui, R. H. (2015). Effect of hypoxia stress and level of Mn2+ on anti-oxidative system of seeding in two resistant kiwifruit species. Science and Technology Review, 33(22), 83–88. https://kns.cnki.net/kcms/detail/detail.aspx?FileName=KJDB201522025&DbName=CJFQ2015 [Google Scholar]
16. Ali, M., Liu, M. M., Wang, Z. E., Li, S. E., Jiang, T. J. et al. (2019). Pre-harvest spraying of oxalic acid improves postharvest quality associated with increase in ascorbic acid and regulation of ethanol fermentation in kiwifruit cv. Bruno during storage. Journal of Integrative Agriculture, 18(11), 2514–2520. https://doi.org/10.1016/S2095-3119(19)62791-7 [Google Scholar] [CrossRef]
17. Koduri, R. S., Tien, M. (1995). Oxidation ofguaiacol by lignin peroxidase: Role of veratryl alcohol. Journal of Biological Chemistry, 270(38), 22254–22258. https://doi.org/10.1074/jbc.270.38.22254 [Google Scholar] [PubMed] [CrossRef]
18. Uchida, A., Jagendorf, A. T., Hibino, T., Takabe, T., Takabe, T. (1994). Effects of hydrogen peroxide and nitric oxide on both salt and heat stress tolerance in rice. Plant Science, 163(3), 515–523. https://doi.org/10.1016/S0168-9452(02)00159-0 [Google Scholar] [CrossRef]
19. Shen, W. B., Xu, L., Ye, M. B., Zhang, R. X. (1996). The suitable condition for determining SOD activity by bitro blue tetrazolium (NBT) photoreduction method. Journal of Nanjing Agricultural University, 19(2), 101–102. https://kns.cnki.net/kcms/detail/detail.aspx?FileName=NJNY199602022&DbName=CJFQ1996 [Google Scholar]
20. Kavita, S., Sareeta, N. (2012). Heat exposure alters the expression of SOD, POD, APX and CAT isozymes and mitigates low cadmium toxicity in seedlings of sensitive and tolerant rice cultivars. Plant Physiology and Biochemistry, 57(2), 106–113. https://doi.org/10.1016/j.plaphy.2012.05.007 [Google Scholar] [PubMed] [CrossRef]
21. Chen, J., Yang, L., Gu, J., Bai, X., Ren, Y. et al. (2015). MAN3 gene regulates cadmium tolerance through the glutathione-dependent pathway in Arabidopsis thaliana. New Phytologist, 205(2), 570–582. https://doi.org/10.1111/nph.13101 [Google Scholar] [PubMed] [CrossRef]
22. Zhao, S. J., Xu, C. C., Zou, Q., Meng, Q. W. (1994). Improvements of method for measurement of malondialdehvde in plant tis-sues. Plant Physiology Journal, 30(3), 207–210. https://kns.cnki.net/kcms/detail/detail.aspx?FileName=ZWSL199403017&DbName=CJFQ1994 [Google Scholar]
23. Rodriguez, C., Mayo, J. C., Sainz, R. M., Antolín, I., Herrera, F. et al. (2004). Regulation of antioxidant enzymes: A significant role for melatonin. Journal of Pineal Research, 36(1), 1–9. https://doi.org/10.1046/j.1600-079x.2003.00092.x [Google Scholar] [PubMed] [CrossRef]
24. Jung, W. J., Jin, Y. L., Kim, Y. C., Kim, K. Y., Park, R. D. et al. (2004). Inoculation of Paenibacillus illinoisensis alleviates root mortality, activates of lignification-related enzymes, and induction of the isozymes in pepper plants infected by Phytophthora capsici. Biological Control, 30(3), 645–652. https://doi.org/10.1016/j.biocontrol.2004.03.006 [Google Scholar] [CrossRef]
25. Gill, S. S., Tuteja, N. (2010). Reactive oxygen species and antioxidant machinery in abiotic stress tolerance in crop plants. Plant Physiology and Biochemistry, 48(12), 909–930. https://doi.org/10.1016/j.plaphy.2010.08.016 [Google Scholar] [PubMed] [CrossRef]
26. Liang, Y. H., Teng, W. C. (2021). Effect of manganese stress on growth and physiological indexes of castanopsis hystrix young seedling. Guangxi Forestry Science, 50(6), 685–689. https://kns.cnki.net/kcms/detail/detail.aspx?FileName=GXLK202106011&DbName=CJFQ2021 [Google Scholar]
27. Gebauer, J., El-Siddig, K., Salih, A. A., Ebert, G. (2004). Tamarindus indica L. seedlings are moderately salt tolerant when exposed to NaCl-induced salinity. Scientia Horticulturae, 103(1), 1–8. https://doi.org/10.1016/j.scienta.2004.04.022 [Google Scholar] [CrossRef]
28. Yildirim, E., Ekinci, M., Turan, M., Ors, S., Dursun, A. (2023). Physiological, morphological and biochemical responses of exogenous hydrogen sulfide in salt-stressed tomato seedlings. Sustainability, 15(2), 1098. https://doi.org/10.3390/su15021098 [Google Scholar] [CrossRef]
29. Qi, D. Z., Liang, M. X., Jiang, F. D., Li, J. Z., Qiao, X. Q. et al. (2020). Effects of exogenous manganese (Mn) on mineral elements, polyamines and antioxidants in apple rootstock malus robusta rehd. Phyton-International Journal of Experimental Botany, 89(4), 943–955. https://doi.org/10.32604/phyton.2020.013801 [Google Scholar] [CrossRef]
30. Yasuor, H., Firer, M., Beit-Yannai, E. (2015). Protective structures and manganese amendments effects on antioxidant activity in pepper fruit. Scientia Horticulturae, 185, 211–218. https://doi.org/10.1016/j.scienta.2015.01.034 [Google Scholar] [CrossRef]
31. Dong, S. X., Yang, H. Y., Wu, W. L., Li, W. L., Lv, L. F. (2020). Physiological and morphological responses of blueberry to manganese stress in soil. Brazilian Journal of Botany, 43, 419–427. https://doi.org/10.1007/s40415-020-00625-4 [Google Scholar] [CrossRef]
32. Canton, G. C., Bertolazi, A. A., Cogo, A. J. D., Frederico, J. E., Juliana, M. et al. (2016). Biochemical and ecophysiological responses to manganese stress by ectomycorrhizal fungus Pisolithus tinctorius and in association with Eucalyptus grandis. Mycorrhiza, 26(5), 475–487. https://doi.org/10.1007/s00572-016-0686-3 [Google Scholar] [PubMed] [CrossRef]
33. Gao, H. S., Huang, L. Z., Gong, Z. J., Wang, X. T., Qiao, X. Q. et al. (2022). Exogenous melatonin application improves resistance to high manganese stress through regulating reactive oxygen species scavenging and ion homeostasis in tobacco. Plant Growth Regulation, 98, 219–233. https://doi.org/10.1007/s10725-022-00857-2 [Google Scholar] [CrossRef]
34. Sieprawska, A., Skórka, M., Bednarska-Kozakiewicz, E., Niedojadło, K., Janiak, A. et al. (2021). Significance of selenium supplementation in root-shoot reactions under manganese stress in wheat seedlings–biochemical and cytological studies. Plant and Soil, 468, 389–410. https://doi.org/10.1007/s11104-021-05138-x [Google Scholar] [CrossRef]
35. Baxter, A., Mittler, R., Suzuki, N. (2014). ROS as key players in plant stress signalling. Journal of Experimental Botany, 65(5), 1229–1240. https://doi.org/10.1093/jxb/ert375 [Google Scholar] [PubMed] [CrossRef]
36. Hussain, A., Nazir, F., Fariduddin, Q. (2019). 24-epibrassinolide and spermidine alleviate Mn stress via the modulation of root morphology, stomatal behavior, photosynthetic attributes and antioxidant defense in Brassica juncea. Physiology and Molecular Biology of Plants, 25(4), 905–919. https://doi.org/10.1007/s12298-019-00672-6 [Google Scholar] [PubMed] [CrossRef]
37. Przybysz, A., Gawroński, S. W., Gawrońska, H., Małecka-Przybysz, M., Pietrzyk, S. et al. (2012). Effect of manganese on yield and quality of hydroponically grown lettuce. Journal of Elementology, 22. https://doi.org/10.5601/jelem.2016.21.1.1127 [Google Scholar] [CrossRef]
38. Xie, Q. Q., Li, Z. J., Yang, L. M., Lv, J., Jobe, T. O. et al. (2015). A newly identified passive hyperaccumulator Eucalyptus grandis × E. urophylla under manganese stress. PLoS One, 10(9), e0136606. https://doi.org/10.1371/journal.pone.0136606 [Google Scholar] [PubMed] [CrossRef]
Cite This Article
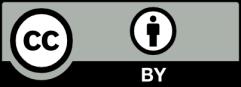
This work is licensed under a Creative Commons Attribution 4.0 International License , which permits unrestricted use, distribution, and reproduction in any medium, provided the original work is properly cited.