Open Access
REVIEW
Elicitation-Based Modulation of Shelf Life in Fruits: Physiological and Molecular Insights
1 Plant Physiology and Molecular Biology Research Unit, Department of Botany, University of Kalyani, Kalyani, 741235, India
2 Laboratory of Plant Stress Responses, Faculty of Agriculture, Kagawa University, Kagawa, 761-0795, Japan
3 Department of Agronomy, Faculty of Agriculture, Sher-E-Bangla Agricultural University, Dhaka, 1207, Bangladesh
* Corresponding Authors: Malay Kumar Adak. Email: ; Mirza Hasanuzzaman. Email:
(This article belongs to the Special Issue: Phytohormones in Improving Crop Production and Stress Tolerance)
Phyton-International Journal of Experimental Botany 2023, 92(8), 2283-2300. https://doi.org/10.32604/phyton.2023.028178
Received 03 January 2023; Accepted 11 April 2023; Issue published 25 June 2023
Abstract
The process of ripening involves physiological and biochemical events that become a concern during postharvest storage. We have documented different approaches for the preservation and maintenance of fruit quality during the postharvest period that are biocompatible and fully safe for consumption. Chemical residues that sustain sensory characteristics, such as color, flavor, aroma, and texture, are considered. In fruit ripening, both physical and chemical elicitors are described that regulate ethylene biosynthesis or its signaling for gene expression. The key regulatory enzymes, such as ACC synthase and ACC oxidase, for ethylene biosynthesis, are important for both climacteric and non-climacteric fruits. Anti-oxidizing genes that retain sensory characteristics are concerns in this respect. Chemical elicitors, including chitosan, polyamine, phenolics, lipopolysaccharide, silver derivatives, and nanocomposites, are described. Gas pressure, light wavelengths, relative humidity, cooling, and other environmental factors are important for improved postharvest storage. These elicitors maintain redox status by inhibiting the generation of reactive oxygen species (ROS) or their lysis. Growth regulators, including abscisic acid, auxin, brassinosteroids, jasmonic acid, and salicylic acid, are important for the regulation of ripening. Mechanical injuries, ionic imbalances, temperature variations, and tissue dehydration can occur irrespective of ripening categories. The use of synthetic physiochemically active compounds is discussed in terms of physiological, metabolic, cellular, and molecular functions. Ethylene-induced autocatalytic processes, antioxidant cascades, epigenetic regulation, and homeodomain gene expression are discussed. Sugar–acid metabolism, dissolution of the cell wall, and direct or indirect production of secondary metabolites related to postharvest storage are mentioned regarding chilling storage. Elicitors and agrochemicals that trigger plant defense to increase secondary metabolite production are discussed for reducing fruit senescence during postharvest storage.Keywords
Postharvest storage has been consistently associated with horticultural practices aimed at maintaining adequate nutritional values and sensory qualities. Harvested fruits may continue to be metabolically active, with processes that may include the activation of some senescence-inducing genes. Senescence following the drying or decaying of fruits can be suppressed by chemicals to maintain postharvest quality [1]. Postharvest management through optimized chemical treatments would be effective in regulating the physiological and metabolic processes of ripening during storage [2]. The regulation of temperature or thermal sensitivity, CO2 sensitization, non-hazardous irradiation, bio-compatible coatings, hormonal supplementation, induction by anti-oxidizing chemicals, and exposure to ozone could minimize fruit losses in both quality and quantity [3]. Nitric oxide and other elicitor chemicals have the potential to control 1-aminocyclopropane-1-carboxylic acid synthase (ACS) and 1-aminocyclopropane-1-carboxylic acid oxidase (ACO) and control ethylene signaling (ETR1) [4]. In ripening physiology, sulfur residues are targeted in sulfur-containing amino acids like methionine to maintain a reducing redox status. In grape vines, fruit coat photosynthesis was retained by sulfur dioxide fumigation, which induced the expression of some antioxidant genes [5]. The Food and Agricultural Organization (FAO) has estimated that 31.21% of fruits and fruit products are either wasted or undergo decomposition [6]. Fresh fruits may be used directly by consumers, but both natural fruit senescence and microbial spoilage could be reduced by postharvest treatments, such as irradiation, coating, moisture stabilization, and reduction of low-aroma volatile compounds [7].
Postharvest fruit losses can also be mediated by dehydration, measured by the relative water content (RWC), osmotic potential, and sensory characteristics, including fruit coat rigidity and texture [8]. Non-climacteric fruits (strawberries, grapes, citrus, etc.) undergo rapid water losses during postharvest storage and therefore experience water deficit stress that develops even in the initial days of storage. Therefore, abscisic acid bioaccumulation and metabolism could be factors that arise in response to many elicitors, including light intensity and temperature, to moderate fruit shelf life. Other conventional practices, such as treatments with gases like nitric oxide, sulfur dioxide, and ozone, are important for improving cold storage for moisture, sustenance of fruit coat texture, etc. Conversely, ozone can induce hydrolysis by regulating S-adenosyl-l-methionine decarboxylase (SAM-DC). Moreover, gaseous ozone may serve to preserve fruit quality by reducing microbial contamination [9].
Studies on fruit pre-cooling have shown that its effects are dependent on the physiological status of ripening fruits. Contrarily, chilling injury during cold storage is related to suppression of anti-oxidation and osmotic relations, while fungal infection is related to sulfur treatment. Observations on cucumber fruits have implicated hydrogen sulfide in the alleviation of chilling injury and extended shelf life [10]. By retarding ethylene production, the loss of fruit wall integrity related to senescence is also retarded, decay severity is reduced, fruit respiration is decelerated, and other cellular and biochemical changes target fruit preservation. Therefore, techniques for triggering ethylene retardation and retaining fruit wall integrity to delay the postharvest period must be updated in the existing literature in terms of the metabolic, cellular, and molecular events triggered by these practices. Notwithstanding the achievements in postharvest science, many issues remain unresolved for reducing postharvest losses in fruit sciences. Elicitors may be the most effective tools for improving the generation of essential secondary metabolites, vitamins, and antioxidants. Improvement of nutrient qualities by the use of diverse elicitors (either chemical or physical), agrochemicals, herbicides, plant products, coating materials, and volatile or gaseous residues remains a promising strategy [11]. Nevertheless, for the control of biotic losses during postharvest storage, the use of elicitors may induce or promote defense mechanisms and improve the phenolic content of fruit pulp [12]. By providing this background view, this review article may serve as a source of fundamental insights into elicitors for the genetic control of fruit ripening processes during postharvest storage.
2 Elicitors in the Ripening Biology of Fruits
Elicitation primarily induces or influences any physical, chemical, or physiochemical phenomenon. In plant biology, elicitation materials are generally compounds that are highly bio-compatible through tissue systems, physiologically and biochemically by the regulators, and finally, capacity to induce gene expression [13]. Elicitors have diverse roles in plant growth and development, starting from seed germination, organ development, energy harnessing process, the flow of metabolic energy, senescence, and finally abscission of plant organs [14]. Importantly, elicitation can act as environmental signals through the plant systems, and its consequent gene expression manifested into cellular, physiological, and biochemical processes [15]. Fruit ripening is physiologically the penultimate plant developmental stage, mostly catabolic processes predominant over metabolic activities. Fruit physiology during the ripening period is important for postharvest storage and commercialization as well as marketability. Ripening fruits through postharvest storage requires extensive techniques where different approaches through physical and chemical elicitations are important. Among the physical agents, light, temperature, gaseous exchange, physical compactness, etc., are important that hinder the possibility of ripening and subsequent increase in shelf life [16]. On the other hand, chemical elicitors are mostly chemicals, biocompatible and non-toxic in nature, easily able in translocation, either inert or easily metabolized. Mostly phenolics and their derivatives are secondary metabolites with diverse chemical configurations that retard fruit ripening. Phenols are generally projected with a benzene ring with one or more hydroxyl groups as a common ligand. Phenols are classified in different groups: anthocyanin, flavonoids, flavones, flavanols, flavanone and non-flavonoids (hydrolyzed/non-hydrolyzed), tannins, benzoic acid, cinnamic acids, their hydroxylated derivatives [17]. The importance of phenolic content in fruit by elicitation is mostly due to increasing the quality characters of fruits as well as regulating the pathways of ripening processes. The most important compounds, flavonoids, and stilbenes are produced from the bifurcation of this pathway [18]. The biomarker enzyme chalcone synthase is another focus of importance which introduces a polyphenol ring with two carbon residues. Those chemicals are often categorized as either organic (methyl jasmonate, chitosan, benzothiadiazole) or inorganic (oxalic acid, calcium chloride) [19]. However, the extent of efficacy of those compounds is variable rather than inconsistence in nature as secondary metabolites like phenolics vary with their qualitative and quantitative modes.
3 Polyphenolics Are Major Constituents of Indigenous Elicitors
Polyphenolics are important compounds widely found in different taxa of the plant kingdom. The implication of polyphenolics is mostly regarded as antioxidants against oxidative stress due to biotic and abiotic factors. Some of the polyphenolic residues, for instance, phytoalexins are exclusively induced under abiotic stress factors, and act as stress alleviation. These might be including senescence, abscission, and also ripening for a growing fruit [19]. Some of the metabolites are quite abundant in inducing fruit ripening phenomenon and are required for organoleptic and qualitative characteristics of the ripening for both climacteric and non-climacteric types of fruit. Likewise, anthocyanin would be a vital biomarker for a variety of fruit’s qualitative characteristics [20]. A few polyphenolics like flavan-3-ols are involved in pungencies, bitterness of a few compounds in ripening fruits [21]. Phenolics as elicitors are mostly required to increase the shelf life of the fruits, particularly, during postharvest storage. The hydroxyl (–OH) groups with the unpaired electron of the phenyl nucleus are required to oxidize the free radicals. These reactions are often accelerated when free radicals are lysed by the metals due to their capacity to chelate metallic ions [22]. Polyphenolics also inhibit pro-oxidant enzymes in the generation of reactive oxygen species (ROS) [23]. Characteristically, elicitors like polyphenolics also induce specific genes which develop other antioxidants, and those block transfer of electrons to molecular oxygen through components of electron carriers in mitochondria [24].
4 Effects of Polyphenolics in Fruit Ripening
It has been reported that polyphenolics improve fruit characteristics like pigmentation, aroma and other qualitative characteristics in the early ripening fruits [25]. They also help in preventing the decaying or degradation of any biotic compounds related to ripening. For example, epicatechin was found to prevent the degradation of lipoxygenase enzyme and prevent the rotting of fruits due to fungal infection [26]. It was also observed that ethylene-induced fruit ripening was directly related to polyphenol accumulation [26]. Moreover, the autocatalytic production of ethylene is also induced by the application of polyphenolics in climacteric fruits. Fruits with variable concentrations of phenolics are required for different antioxidation efficiency, which is required to sustain the freshness of fruit pulp. This has also been reported in grapes where the polymerization of some pro-cyanides was unchanged or delayed the ripening progress by exogenous application of phenolics [27]. Therefore, the dynamics of fruit ripening would be associated with polyphenol accumulation as the turnover of fruit color (from green to yellow to red) through biosynthesis of pigments like chlorophyll, carotenoids, and oxygenated derivatives. Fruit polyphenolics are also used in cosmetics and nutraceuticals compounds [28]. Fruit color being the primary indicator of its maturity, quality and freshness serving an important parameter in the accumulation of different pigments through the maturity stages. Fruit color, with its diverse pigment accumulation are also genetically controlled with biosynthetic genes. Therefore, the turnover of pigments through ripening stages may be the criteria of the fruit for optimal postharvest storage. Apart from the light-harvesting mechanism with photosynthetic machinery as well as photoinhibition, phenolics like anthocyanin acts as UV filter that protects the plants from high light energy, particularly, at low temperatures [29]. Therefore, anthocyanin can also be an ameliorative compound to enhance chilling tolerance in fruits at cold storage. Additionally, this reduces photo inhibition which would be a factor for retaining the greenness of fresh fruits through photosynthetic activities in fruit coats and might open a possibility for postharvest preservation.
5 Perception of Hormonal Impact on Postharvest Ripening
Some of the plant hormones, such as ethylene, is the major controlling network for fruit ripening as they act as the regulator of the developmental process at the cellular and molecular level. A fruit for its genetically regulated process must involve three distinct developmental stages viz., setting of fruits after pollination, developmental advancement, and ripening or senescence [30]. This is mediated by specific coordinated network of interacting gene(s) and signaling pathways. The most influential hormones are ethylene, abscisic acid and gibberellin are most apprehensive to improve texture, flavor and aroma of fruits [31]. Ethylene has a major role in the development and maturity of fruits with ethylene perception on cell membrane following its translocation and gene expression in nucleus [32]. In tomatoes those genes are initially described as LeETR1, R2, R3, R4, R5, R6 [33]. These are the receptors encoded by NR and LeETR4 genes that functions as negative regulators of ethylene paths in fruits through functional compensation belonging to multigenic family. The identification of another new number, LeETR7 has been added with polygenetic analysis through validation of the similarity index with other receptors. In another mutant characterized with a replacement of pro-residue by leucine on the N-terminal binding sites shows impaired or anomalous ripening in tomato [33]. This is confirmed by the identification of LeETR3 and LeETR4 as the major receptor expressed at the ripening stages, Still, LeETR7 also plays a ripening related expression and thereby happens to be the third most abundantly expressed protein during ripening [34].
6 Stress Hormones: Abscisic Acid-Related Gene Expression in Postharvest Ripening
Abscisic acid being an undoubted stress hormone and it also induces a collective operational cascade where ABA, stress and ripening (ASR) in a coordinated manner of gene expression. These were first identified with ASR gene in Lycopersicon c-DNA library under dehydration with ABA induction. In ripening fruits also ASR genes are simultaneously expressed under ABA interferences as also happens in plant species under water stress [35]. ASR genes have been involved in ripening specific developmental stages are reported in many climacteric and non-climacteric fruits. The analysis of expression in various tissues of tomato reveals abundant ASR1 transcripts accumulation in leaves and fruit pericarp but ASR2 only in roots [17]. In Pinus, another form of ASR (lp3) was expressed in roots, however, under dehydration stress and that also remains overexpressed in fruit ripening. In fact, ripening-induced dehydration that also activates ASR genes from a small gene family with stretches of exons interrupted by one intron. In more details when fruits undergo senescence, desiccation induces some LEA (late embryogenesis abundant) proteins that functions as cell wall stabilizer for fruit coat [17]. At molecular characterization these classes resemble highly hydrophilic but intrinsically unstructured mostly by glycine residues those also impart flexible conformation of fruit coat favorable for delayed ripening.
ABA in fruit ripening involves two rate-limiting gene for biosynthesis: 9-cis-epoxycarotenoid dioxygenase (NCED) and 1-aminocyclopropane-1-carboxylic acid synthase (ACCS) that also regulates for ethylene biosynthesis. The relationship between ABA and ethylene during ripening is rather complex [36]. Initially, there rise the expression of NCED1 gene occurred with high ABA content. This was followed by LeACS4, and LeACO1 genes which induce the climacteric rise of respiration and ethylene concentration. This expression was also coordinated with the activity of ACC oxidase (ACO) through the accumulation of ethylene and reached a peak at the intermediate stages of ripening. This would possibly confer that NCED triggers ABA biosynthesis at the initial of ripening and also switches on the minimum level of ethylene through ACCS activity.
7 Role of Elicitors in Secondary Metabolic Dynamics through Ripening
The ripening is sensitive to different physicochemical inputs such as temperature (high and low) and radiation (solar, ultraviolet, gamma-radiation) [37]. For example, the grapes containing stilbene are induced by UV light and sometimes accumulation becomes higher than untreated [38]. UV-C irradiated fruits can overcome several fold increased sugar alcohols compared to non-UV treatment. Under post harvested fruits UV also induces a higher level of trans-resveratrol and other phenolic residues in berries [39]. Flavonoid biosynthesis is also under up-regulation with different physical treatments like cold stress. Carbon dioxide sensitization can also induce secondary metabolites such as anthocyanin in fruits. Several chemical elicitors are mostly involved in the preservation of the fruit quality through different sensory characteristics like odor, hydration, softness, etc. which helps in maintaining the postharvest preservation [40]. Some of these important elicitors are chitosan, methyl-cyclopropen, benzothiadiazol and agro-chemicals. Some of these compounds can elicit the jasmonic acids and salicylic acid pathway to reduce the fungal infestation [41]. Fruits treated with these chemicals act as elicitors in signaling of fruit defense cascade, hypersensitive reactions. Some compounds like derivatives of phenolics, flavonoids, other macromolecules such as lignin, and special glycoproteins are increased with benzothiadiazol compounds on fruits [42]. Following treatments with elicitors fruits also regulate ethylene biosynthetic pathways through autocatalytic mode. A number of chemicals often downregulate genes for ACC synthesis or ACC oxidation and/or to lysis ethylene [43]. In most of the cases the biosynthetic inhibitors and structural analogues are quite efficient in ethylene metabolism to regulate the ripening phenomenon in horticultural fruits [44].
8 Types of Elicitors Used in Ripening
A number of chemicals and physical elicitors are effective in increasing the quality and quantity of ripening substances (Fig. 1). In the grapes UV radiation caused several folds higher of specific phenolics as compared to other secondary metabolites [45]. UV radiation increases content of resveratrol in apple and other volatile phenolics in berry fruits under postharvest storage [46]. Under chilling stress, the gene activation for flavonoid biosynthesis with overexpression of phenyl alanine ammonia lyase (PAL) is the result. Similarly, CO2 enrichment induces the pigments for anthocyanin and its aglycoside derivatives like pro-anthocyanidine in grapes [47]. A number of chemical elicitors like hydrophilic chitosan, methyl cyclopropane, benzothiadiazole (BTH) and other agro-chemicals can be used as substituents for salicylic and jasmonic acid. Mostly these chemicals are engaged with triggering of postharvest pathological degradation of fruits. The resistant substances are induced by postharvest thiadiazole compounds which induce number of secondary metabolites including phenolics and lignin, hydroxy-proline, glycoproteins etc. in orchard crops like mango [48]. Postharvest management could be done by reducing the oxidative burst of many fruits. The generated ROS can be down regulated by foliar application of few hairpin proteins as found in tomato [49]. Chitosan coated materials could be used as successful elicitors to inhibit the wilting during postharvest storage of horticultural fruits. Besides, naturally occurring senescence, postharvest storage could be affected by pathogenic invasion. The mentioned chemical compounds not only function as bactericidal or bacteriostatic but also induce the chemical defense as mostly illustrated with phenolic residues. As for example benzothiadiazole, carbothioic acids and its methyl esters can be supplemented with methyl jasmonate to induce systemic acquired resistance during postharvest storage. This mixture can induce PAL of postharvest treatment of orchard fruits. There are several biosynthetic routes by which secondary metabolites could be induced in normal metabolic flux [50]. The rate limiting enzymes are glucose-6-phosphate dehydrogenase (GPDH), tyrosine ammonia lyase, dihydro-flavanol reduetase, 4-coumarate ligase etc. In banana, polyphenol oxidase and class 3 peroxidases are required for increased biosynthesis of phenolics. In addition, pre-harvest treatment of berry fruits are induced to accumulate quercetin, kaempferol for disease resistance but has to compromise with the quality characters [51]. The accumulation of para-coumaric acid and gallic acids in leaves under treatment with BTH can translocate into fruits and improved anthocyanin biosynthesis. Therefore, both for senescence inducing ripening as well as fungal infection could be managed by BTH to delay the ripening of fruits under postharvest storage.
Figure 1: A general scheme for commonly available elicitors in application for modulation of fruit(s) ripening
9 Molecular Bases of Elicitations in Signaling
In most cases, elicitations in ripening fruits are counted by the accumulation of plant secondary metabolites, those are regulated at the biosynthesis, cellular transport, turnover, and degradation [52]. During ripening, phenolic residues like flavonoids can be regulated by different elicitors by various mechanisms. The application of chitosan, salicylate, inorganic substances like potassium nitrate (KNO3) could stimulate the de-novo synthesis of quercetin, anthocyanin. Chitin could also enhance the anthraquinone biosynthesis, which could check the fungal infestation of solanaceous fruits through increased permeability of cell membrane. Different elicitors and their modalities in ripening regulation for the delayed shelf life of fruits are summarized in Table 1.
The current knowledge of metabolites on elicitation in ripening is exclusively based on the induction of secondary metabolites. However, few primary metabolic paths are also important for chemical elicitation also. As for example, jasmonates and its methyl derivatives are marked on changes of central metabolic pools of phosphoenol pyruvic acid, one carbon residues like formate, glyceraldehydes-3-phosphate like unit residues of energy-yielding metabolism. Fruit tissues both on-shoot and off-shoot senescence could be prevented through few antioxidative enzymes with fungal elicitors. Likewise, glutathione (GSH) induction by glutathione S-transferase (GST) which are required in conjugations of electrophilic residues to reduced glutathione (GSH) is important in this context [53]. During ripening, a number of phenyl-propanoid residues may be transported into vacuoles through catalyzing GSH conjugation residues. Therefore, GSH biosynthesis would be a good approach for enhancing fruit quality during ripening.
Ripening or senescence becoming a self-stressed condition for the fruits. The increase in cell permeability, maintenance of osmotic potential, cellular turgidity and other water relation attributes must be included to modulate by chemical or physical elicitations in the reduction of fruit senescence. In addition, the possibility of cell death by autolytic procedures could also be reversed by such elicitors where leakage of cell membrane could be prevented. Thereby, solute loss by fruit coat could find an ameliorative effect by such treatments.
Regulation of hydrolysis or turnover of secondary metabolites is important in fruit ripening. Sometimes, this often exceeds the induction of biosynthesis of secondary metabolites by elicitors. For example, constitutive or inducible alkaloids are often regulated by elicitors in fruits. Phytoalexin and other polyphenolics are sometimes degraded by UV, and those are regulated with overexpression by elicitor treatment [54]. Many composite combinations of originally synthesized and constitutive residues also have been effective for chemical elicitation. Moreover, several secondary metabolites act specifically as signal molecules in different pathways of the ripening process [55]. The compatibilities of different elicitors in the regulation of delayed ripening are based on a few common paths to follow like osmotic adjustment, wall hydrolysis, and redox homeostasis (Fig. 2).
Figure 2: Compatibilities of different chemicals and biological elicitors with molecular mechanism of delayed ripening phenomena
10 Genetics of Ripening with the Elicitation of Ethylene Metabolism
Ethylene metabolism is the central dogma for ripening physiology that has the most convincing impact for various processes such as color changes and softening of fruit coat [38]; tissue dehydration [56]; turnover of protein and pigments solubilization of complex carbohydrates into sugars [34]; sugar-organic acid interconversion [57]. Ethylene metabolism is based on the complexity of transcriptional networks and their regulation through polygenetic formulas that may advance the downstream processes in different phases of ripening. Apart from its role in climacteric ripening, ethylene regulates non-climacteric ripening mostly through signal transduction pathways with chemical elicitation (Fig. 3). The pathways by which ethylene induces ripening-related genes may interconnect various signaling cascades are still to be fully elucidated.
Figure 3: Physiological, metabolic and cellular modalities in general for regulation of ripening of fruit(s) under elicitation
In plants, DNA methylation is the basis of reversible epigenetic modification. However, conserved by the introduction of a –CH3 residue into cytosine ring. De novo DNA methylation, active DNA de-methylation and passive DNA de-methylation are the most common in plant systems with reference to fruit ripening development [58]. A number of enzymatic pathways with the participation of DNA methyl-transferase, chromomethylase and RNA dependent direct methylation paths are important in developmental biology of fruits [59]. In tomato, this has been more clarified with DNA methylomes as well as in some non-climacteric berries as also found some hypo-methylation sites on ripening. The loss of DNA methylation in such fruits follows the weakening of RNA-directed DNA methylation pathways rather than an induced expression of DNA demethylase genes. The use of DNA methylation inhibitors promotes the fast ripening of strawberries. Thus, irrespective of modalities of ripening categories for both climacteric and non-climacteric fruits, a flux of metabolites gathers more information to support autocatalytic ethylene production. Although ethylene is the major key to regulating ripening, but the non-climacteric ones do not essentially respond to ethylene metabolism but induce senescence-inducing genes. In specific elicitors-mediated ripening like salicylic acid a significant accumulation of ABA can corroborate the induction of antioxidant defense. Therefore, the genetics of ripening is yet to be differentiated in relation to ethylene translocation and signaling alone or any other growth residues. For non-climacteric ones, most possible and established fact for ethylene involvement is the differential responses to ethylene for diversity of ethylene response factors (ERFs). ERFs are the multi-genic largest families of MAD box transcription factors. It is already known that ethylene signaling is the most important on fruit maturation through senescence either on-shoot or off-shoot [60]. The identification of ripening related ERFs acting on upstream or in conjugation with ethylene induction has made it more complex. ERF well may cover “RIPENING INHIBITOR” (RIN), NON-RIPENING (NOR), TOMATO AGAMOUS-LIKE 1 (TAG L1), HOMEO-DOMAIN-LEUCINE ZIPPER HOMEO BOX PROTEIN (LeHB-1), MADs box S1 (MADS1), APETALA2a are the most probable players in ripening signaling as well as ethylene biosynthetic pathways [61].
Among all transcription factors, two distinct genes are important. First, ACS and, second, ACO which are required for conversion of S-adenosine methionine into ACC following ethylene, respectively [14]. Ethylene is perceived on the cell membrane by a receptor protein called Ethylene Triple Response (ETR). There are specific other signaling components like RN1 is a copper cofactor for the binding of the ethylene on the membrane. There are other proteins also involved in such binding. Another factor glucocorticoid receptor (GR) acts as receptor for ETR-RN1 complex for downstream mediation of signaling output. There is another protein which may bind to these receptors complex and later degrades the complex. The receptor complex with ethylene on membrane is negatively regulated when ethylene is presented by another CTR1. CTR1, in fact down regulates ethylene responses by the inactivation of ethylene insensitive 2 (EIN2) which behaves a transcription factors. In more details, the transcription factor EIN3 as well as ethylene insensitive 3- like-1 (EIL1) is degraded by another ethylene insensitive 3 binding F-Box [62]. In absence of EIL1 factor of ethylene responsive genes is down-regulated when ethylene binds to the receptors, the receptors undergo inactive following switching off CTR1 phosphorylation. Active EIL thus becomes essential for expression of target genes of ERF mediated transcription factors. ERF may bind with other ethylene response elements in developmental plant biology and modulates the ethylene regulated genes in their target promoter.
In the last decade, significant efforts and progress have been possible through different biotechnological as well as genetic engineering to develop transformed fruits. Besides, the quality and quantity of fruits, emphasis has been given on the fruit quality, and shelf-life. Therefore, the ripening with prolonged shelf life of fruits by applying chemical or physical elicitors would be the research focus [63]. In regard to slow ripening, the maintenance of flavor, aroma, texture and overall freshness of fruit are most important. In most cases, ripening of tomato was more advantageous for such biotechnological events with RNAi like approaches for easy transformation capacity [64].
From the biochemical point of view, good ripening depends on an increase in respiration rate with a sharp accumulation of ethylene. Therefore, control of ethylene biosynthesis or its sensitivity could be the most important index of fruits than postharvest storage. Different physicochemical conditions in the maintenance of those ripening-specific stress are widely studied. For instance, the stimulation of cellular, and environmental condition mimic storage capacity and storage conditions for the retention of fruits quality and extended shelf life. In ripening fruits, chlorophyll undergoes degradation, the green color may be shifted into red pigmentation in tomatoes as when lycopene is biosynthesized. Therefore, the inhibition of lycopene synthesis is another aspect for genetically engineering fruits in support to postharvest storage [65]. The thinning and softening of the fruit pulp by cell wall hydrolyzing enzymes like pectin methyl esterase, poly-galacturonase and methyl esterase are target points to downregulate the activity. Elicitors in terms of enzyme inhibitor compounds could check activity directly or indirectly through antisense technology. For revised orientation of ripening specific proteins or produce non-functional transcripts may be the target [66]. Ethylene production is also targeted to the hydrolysis of starch to sugar and increases the generation of varying secondary metabolites, including aroma, flavor, smell, and other sensory characteristics. It can be reversed by such antisense technology, particularly, for genes of ethylene metabolism: ACC synthase and ACC oxidase. There are few reports for genetically important postharvest storage in tomatoes where pTOM5 encodes the phytoene synthase through carotenoid biosynthesis pathway for red coloration of tomato, would be the concern for biotechnological improvement of fruits. Other genes like pTOM6 and pTOM and ACC synthase are the targets for modification of tomato. Another enzyme endo-polygalacturonase which is responsible of Flavr Savr tomato pauses of softening of fruits due to degradation of cell wall bound pectin by the enzyme from the tomato. Antisense mRNA for polygalacturonase has been isolated, cloned into plants for development of pTOM6 and for these cases the transgenic plants through cDNA manipulation have been best results. The biotechnologically improvement of Flavr Savr tomato was developed in US which is recommended as good as normal tomato. The advantages of these materials have a long storage, almost double the time as compared to wild tomato without any cold storage. For the ethylene metabolism, both ethylene biosynthesis genes like ACC-1 as well as ethylene perception gene ETR1, ETR2 were targeted in antisense strategies for delay ripening [67]. In other modalities a bacterial gene ACC deaminase was cloned in tomato which remove NH2 group from ACC in reduction of substrate availability for ethylene biosynthesis. By this technique, ethylene exhibited above 90% in transgenic plants, which physiologically delays ripening within 45 days [68].
11 Mechanism of Physical Elicitation in Ripening Behavior
The useful secondary metabolites produced by plants are the inductive responses to stress. For example, medicinal or aromatic plants from their wild habitat when transferred to cultivated conditions under optimum growing condition, the influence of stressors are induced to produce a large number of secondary metabolites [68]. Many bioactive compounds are induced under different abiotic stressors are effective in regulation of biological residues. Likewise, fruit ripening with its alteration of metabolites is thought to be induced by different physical elicitors on the development and distribution of secondary metabolites. In fruit ripening, a number of physical elicitors such as light and gaseous substances are also important. Therefore, light becomes a signaling component of fruits which retards pigment accumulation in ripening monitoring phytochromes and undoubtedly modulates through different domains of light. Light is known to retard the senescence both on leaves and chloroplast. Therefore, changes in pigmentation and its rate through the ripening period could be modulated in different ways. Notably, the quality and quantity of light through regulated devices at specific wavelengths have been most important. For example, light emitting diode (LED) with variable wavelengths can change fruit metabolomics in relation to senescence [57]. This is more important with light spectrum added with blue wavelength on fruit wall, metabolomes, particularly, at the pericarp during ripening [69]. Green tomato fruits when exposed to variable light conditions in comparison to dark, exhibited a significant amount of phytochrome-regulated lycopene biosynthesis, however, independent of ethylene production. It is also noted that the effect of color shade in net format can also induce leaf photosynthetic parameters, which in downstream, contribute translocation of metabolites for tomato fruit quality [70]. The analysis of transcripts for phytochrome A is overexpressed by several folds during ripening. The softening of pericarp tissues revealed that the ratio of red to far red is the most important to increase the inhibitory compounds for ripening from immature green to red ripe condition. A brief pulse of red wavelength on postharvest storage, green fruits could be induced by lycopene accumulation in tomatoes [71].
12 Exogenous Application of Elicitors Act Differentially for Fruit Maturity
For physical elicitors, the temperature more towards chilling is most effective in fruits, but their effects are variable for climacteric and non-climacteric fruits [72]. For climacteric fruits, control of temperature would be the key ethylene-mediated phenomenon of ripening, where ACC synthase, ACC oxidase play vital roles. The conversion of ACC to ethylene are temperature dependent. This correlates with downregulation of sensory characters like-volatilization of organic residues, changes fruit coat, softness of fruit pulp. Therefore, both ACC synthase and ACC oxidase would be targets under the control of temperature-mediated delay ripening. Light sensitivity is important in fruit ripening phenomena particularly changes of fruit like- tomato, banana, etc., where the application wavelength near ultraviolet (UV-C) facilitated the biosynthesis of lycopene genes (LCYE, LCYB) for terpenoid pathway(s). The rate-limiting enzymes like hydroxy methyl glutaryl CoA synthase (HMG-CoA synthase) are the target to regulate overproduction of lycopene by blue, UV, and X-ray [73]. Therefore, lycopene biosynthesis becomes the index of delayed ripening in fruit by changes of light intensity. Mostly, γ and X-ray are used to suppress the secondary metabolites of fruit greenness to other colors on fruit coat vis-a-vis the prolonged shelf life [74]. In another way, the pulsed light beams are also effective. The hydrolysis of cell wall residues, particularly, glucan, xylan and other hetero-polysaccharides are controlled with down regulation of cell wall bound hydrolysis by hydrolases and esterases [75]. For postharvest storage, other factors like bacterial and fungal infection causes the biotic loss. It was found that effective application of ultrasound (250 kw for 9.8 min) in fruits reduce the biotic loss for sensory characters [76].
The application of chemical residues has been studied in details in changes of senescence and decay in different fruits. In most of the cases, the dehydration stress is controlled by oxidative redox of fruit tissues and effective with chemicals for delayed ripening. Likewise, inorganic compounds: calcium salt (CaCl2), reduced sulfur are most fruitful for regulation of osmotic potential of fruits under postharvest condition. The maintenance of viability of fruit coat tissues through growth respiration is moderated by a reduced oxidative potential of tissue where hydrogen sulfide, sodium sulfide-like compounds are effective. These treatments are more effective in the regulation of anti-oxidation Thus, exogenously applied growth residues like auxin, gibberellic acid are potential in fruit maturation where an inhibitor of GA biosynthesis was found effective. Application of GA biosynthesis inhibitor, when reduced the activity of α-amylase, declined the accumulation of reducing sugar and thereby regulates sugar-acid interconversion [77]. This is also found with some auxin derivatives where, the activity of chlorophyllase, peroxidase exists with the correlation of softness in fruit coat [78]. Essential oil with specific mono and di-terpenoid residues when mix with ether also exhibited antioxidant defense through the increased pool of reduced carbon supplements as respiratory fuel. The oxidative breakage of macromolecules like nucleic acid, protein, dextran is escaped in oxidation with the application of polyamines. The latter makes a shield over the backbones of the cell organelles with ROS inversion [79]. Biocompatible coating materials like gluten, methylcellulose is found potential both for pre and postharvest preservation of fruits that requires for retention of hydration, increase porosity, bypass of fermentative reactions, and thereby as a whole increase shelf life [80].
13 Conclusion and Perspectives
The activity of different elicitors may be physical or chemical and has differential responses in ripening-mediated phenomena. The application of elicitors is also varied with the exposure period, duration, chemical or physical nature of elicitors, the state of fruit, storage condition, etc. Most of cases, the variability of elicitors-mediated responses is manifested with up/downregulation of specific pathways contributing to ripening and senescence of fruits under postharvest storage. The physiochemical treatments of fruits on postharvest storage, irrespective of climacteric and non-climacteric ones, depend on ethylene metabolism or ethylene-induced genes related to cell wall hydrolysis and redox regulation. Not a single character relating ripening phenomenon but a multiplex of different biochemical events are involved in retaining the quality characters supporting the viabilities of fruit tissues. Elicitors on hormonal regulation of different types are concerned with two major domains; first, signaling the ethylene over the membrane, and second one, is the elicitation of antioxidant defense systems. Physical elicitors, mostly the stimulation of edaphic factors is important in terms of light, temperature, CO2 exposure, etc. Fruit responds variably with qualitative and quantitative ripening-specific indices directly or indirectly interfered by chemical and physical elicitation. Thus, sensitization with those is based on physiological parameters related to the acquisition of cell wall material, particularly, cellulosic and other complex polysaccharides in relation to the hydrophilicity of ripening fruits. Other elicitors like relative humidity and temperature have better accessibility to modulate the specific genes on ripening for softness, aroma production, and others. As a whole, fruit ripening being a multi-cascade phenomenon is eventually sensitive to various elicitation, particularly, with regards to its regulation and delayed ripening to focus on postharvest preservation and other perspectives.
Acknowledgement: We thank Md. Rakib Hossain Raihan and Farzana Nowroz for their critical proof check of the manuscript.
Funding Statement: This work received no external funding.
Author Contributions: Conceptualization, M.K.A., M.F. and M.H.; writing—original draft preparation, A.K., A.D., S.P., A.G., M.K.A.; writing—review and editing, M.F. and M.H.; visualization, M.K.A. and M.H.; supervision, M.K.A. All authors have read and agreed to the published version of the manuscript.
Availability of Data and Materials: All related information is available in this manuscript.
Conflicts of Interest: The authors declare that they have no conflicts of interest to report regarding the present study.
References
1. Panghal, A., Yadav, D. N., Khatkar, B. S., Sharma, H., Kumar, V. et al. (2018). Postharvest malpractices in fresh fruits and vegetables: Food safety and health issues in India. Nutrition and Food Science, 48, 561–578. [Google Scholar]
2. Zhang, Y., Huber, D. J., Hu, M., Jiang, G., Gao, Z. et al. (2018). Delay of postharvest browning in litchi fruit by melatonin via the enhancing of antioxidative processes and oxidation repair. Journal of Agricultural and Food Chemistry, 66, 7475–7484. [Google Scholar] [PubMed]
3. Mayookha, V. P., Pandiselvam, R., Kothakota, A., Ishwarya, S. P., Khanashyam, A. S. et al. (2022). Ozone and cold plasma: Emerging oxidation technologies for inactivation of enzymes in fruits, vegetables, and fruit juices. Food Control, 144, 109399. [Google Scholar]
4. Steffens, C. A., Santana, G. R. O., do Amarante, C. V. T., Antonovviski, J. L., Miqueloto, T. et al. (2022). Treatment with nitric oxide in controlled atmosphere storage to preserve the quality of ‘Laetitia’ plums. LWT, 158, 113033. [Google Scholar]
5. Considine, M. J., Foyer, C. H. (2015). Metabolic responses to sulfur dioxide in grapevine (Vitis vinifera L.Photosynthetic tissues and berries. Frontiers in Plant Science, 6, 60. [Google Scholar] [PubMed]
6. Hussein, Z., Fawole, O. A., Opara, U. L. (2020). Harvest and postharvest factors affecting bruise damage of fresh fruits. Horticultural Plant Journal, 6(1), 1–13. [Google Scholar]
7. Munhuweyi, K., Mpai, S., Sivakumar, D. (2020). Extension of avocado fruit postharvest quality using non-chemical treatments. Agronomy, 10(2), 212. [Google Scholar]
8. Di Stasio, E., Rouphael, Y., Raimondi, G., El-Nakhel, C., de Pascale, S. (2018). Postharvest performance of cut rose cv. lovely red as affected by osmoprotectant and antitraspirant compounds. Advances in Horticultural Science, 32, 311–318. [Google Scholar]
9. Minas, I. S., Vicente, A. R., Dhanapal, A. P., Manganaris, G. A., Goulas, V. et al. (2014). Ozone-induced kiwifruit ripening delay is mediated by ethylene biosynthesis inhibition and cell wall dismantling regulation. Plant Science, 229, 76–85. [Google Scholar] [PubMed]
10. Wang, J., Zhao, Y., Ma, Z., Zheng, Y., Jin, P. (2022). Hydrogen sulfide treatment alleviates chilling injury in cucumber fruit by regulating antioxidant capacity, energy metabolism and proline metabolism. Foods, 11(18), 2749. [Google Scholar] [PubMed]
11. Dhuldhaj, U. P., Singh, R., Singh, V. K. (2022). Pesticide contamination in agro-ecosystems: Toxicity, impacts, and bio-based management strategies. Environmental Science and Pollution Research, 30, 9243–9270. [Google Scholar] [PubMed]
12. Portu, J., López, R., Baroja, E., Santamaría, P., Garde-Cerdán, T. (2016). Improvement of grape and wine phenolic content by foliar application to grapevine of three different elicitors: Methyl jasmonate, chitosan, and yeast extract. Food Chemistry, 201, 213–221. [Google Scholar] [PubMed]
13. Manjunatha, G., Lokesh, V., Neelwarne, B. (2010). Nitric oxide in fruit ripening: Trends and opportunities. Biotechnology Advances, 28, 489–499. [Google Scholar] [PubMed]
14. Houben, M., de Poel, B. V., (2019). 1-aminocyclopropane-1-carboxylic acid oxidase (ACOThe enzyme that makes the plant hormone ethylene. Frontiers in Plant Science, 10, 695. [Google Scholar] [PubMed]
15. Cheema, A., Padmanabhan, P., Amer, A., Parry, M., Lim, L. et al. (2018). Postharvest hexanal vapor treatment delays ripening and enhances shelf life of greenhouse grown sweet bell pepper (Capsicum annum L.). Postharvest Biology and Technology, 136, 80–89. [Google Scholar]
16. Leneveu-Jenvrin, C., Charles, F., Barba, F. J., Remize, F. (2020). Role of biological control agents and physical treatments in maintaining the quality of fresh and minimally-processed fruit and vegetables. Critical Reviews in Food Science and Nutrition, 60(17), 2837–2855. [Google Scholar] [PubMed]
17. Duarte-Sierra, A., Tiznado-Hernández, M. E., Jha, D. K., Janmeja, N., Arul, J. (2020). Abiotic stress hormesis: An approach to maintain quality, extend storability, and enhance phytochemicals on fresh produce during postharvest. Comprehensive Reviews in Food Science and Food Safety, 19(6), 3659–3682. https://doi.org/10.1111/1541-4337.12628 [Google Scholar] [PubMed] [CrossRef]
18. El-Sharkawy, I., Sherif, S., Qubbaj, T., Sullivan, A. J., Jayasankar, S. (2016). Stimulated auxin levels enhance plum fruit ripening, but limit shelf-life characteristics. Postharvest Biology and Technology, 112, 215–223. https://doi.org/10.1016/j.postharvbio.2015.09.012 [Google Scholar] [CrossRef]
19. Pakkish, Z., Ghorbani, B., Najafzadeh, R. (2019). Fruit quality and shelf life improvement of grape cv. Rish Baba using Brassinosteroid during cold storage. Journal of Food Measurement and Characterization, 13(2), 967–975. [Google Scholar]
20. Terry, L. A., Joyce, D. C. (2004). Elicitors of induced disease resistance in postharvest horticultural crops: A brief review. Postharvest Biology and Technology, 32(1), 1–13. [Google Scholar]
21. Bose, S. K., Howlader, P., Wang, W., Yin, H. (2021). Oligosaccharide is a promising natural preservative for improving postharvest preservation of fruit: A review. Food Chemistry, 341(377), 128178. [Google Scholar] [PubMed]
22. Kalia, A., Kaur, M., Shami, A., Jawandha, S. K., Alghuthaymi, M. A. et al. (2021). Nettle-leaf extract derived ZnO/CuO nanoparticle-biopolymer-based antioxidant and antimicrobial nanocomposite packaging films and their impact on extending the postharvest shelf life of guava fruit. Biomolecules, 11(2), 224. [Google Scholar] [PubMed]
23. Munhuweyi, K., Mpai, S., Sivakumar, D. (2020). Extension of avocado fruit postharvest quality using non-chemical treatments. Agronomy, 10(2), 212. [Google Scholar]
24. Darré, M., Vicente, A. R., Cisneros-Zevallos, L., Artés-Hernández, F. (2022). Postharvest ultraviolet radiation in fruit and vegetables: Applications and factors modulating its efficacy on bioactive compounds and microbial growth. Foods, 11(5), 653. [Google Scholar]
25. Li, Z., Yao, L., Yang, Y., Li, A. (2006). Transgenic approach to improve quality traits of melon fruit. Scientia Horticulturae, 108(3), 268–277. [Google Scholar]
26. Xu, R., Wang, L., Li, K., Cao, J., Zhao, Z. (2022). Integrative transcriptomic and metabolomic alterations unravel the effect of melatonin on mitigating postharvest chilling injury upon plum (cv.Friar) fruit. Postharvest Biology and Technology, 186, 111819. [Google Scholar]
27. Obianom, C., Romanazzi, G., Sivakumar, D. (2019). Effects of chitosan treatment on avocado postharvest diseases and expression of phenylalanine ammonia-lyase, chitinase and lipoxygenase genes. Postharvest Biology and Technology, 147, 214–221. [Google Scholar]
28. Geransayeh, M., Sepahvand, S., Abdossic, V., Zarrinniad, V. (2015). Effect of methyl jasmonate treatment on decay, postharvest life and quality of Strawberry (Fragaria ananassa L. cv. Gaviota) fruit. International Journal of Current Science and Research, 15, 123–131. [Google Scholar]
29. Sharif, R., Mujtaba, M., Ur Rahman, M., Shalmani, A., Ahmad, H. et al. (2018). The multifunctional role of chitosan in horticultural crops: A review. Molecules, 23, 872. [Google Scholar] [PubMed]
30. Chandrasekaran, M., Boopathi, T., Paramasivan, M. (2021). A status-quo review on CRISPR-Cas9 gene editing applications in tomato. International Journal of Biological Macromolecules, 190, 120–129. [Google Scholar] [PubMed]
31. Lattanzio, V. (2003). Bioactive polyphenols: Their role in quality and storability of fruit and vegetables. Journal of Applied Botany, 77, 128–146. [Google Scholar]
32. Aizat, W. M., Ahmad-Hashim, F. H., Jaafar, S. N. S. (2019). Valorization of mangosteen, “The Queen of Fruits,” and new advances in postharvest and in food and engineering applications: A review. Journal of Advanced Research, 20, 61–70. [Google Scholar] [PubMed]
33. Ghorbani, B., Pakkish, Z., Najafzadeh, R. (2017). Shelf life improvement of grape (Vitis vinifera L. cv. Rish Baba) using nitric oxide (NO) during chilling damage. International Journal of Food Properties, 20, 1094–2912. [Google Scholar]
34. Reyes-Díaz, M., Lobos, T., Cardemil, L., Nunes-Nesi, A., Retamales, J. et al. (2016). Methyl jasmonate: An alternative for improving the quality and health properties of fresh fruits. Molecules, 21, 567. [Google Scholar]
35. Hamdi, K., Brini, F., Kharrat, N., Masmoudi, K., Yakoubi, I. (2020). Abscisic acid, stress, and ripening (TtASR1) gene as a functional marker for salt tolerance in durum wheat. BioMed Research International, 2020, 2314–6133. [Google Scholar]
36. Kou, X., Zhou, J., Wu, C. E., Yang, S., Liu, Y. et al. (2021). The interplay between ABA/ethylene and NAC TFs in tomato fruit ripening: A review. Plant Molecular Biology, 106, 223–238. [Google Scholar] [PubMed]
37. Lu, L., Ji, L., Ma, Q., Yang, M., Li, S. et al. (2019). Depression of fungal polygalacturonase activity in Solanum lycopersicum contributes to antagonistic yeast-mediated fruit immunity to botrytis. Journal of Agricultural and Food Chemistry, 67, 3293–3304. [Google Scholar] [PubMed]
38. Posé, S., García-Gago, J. A., Santiago-Doménech, N., Pliego-Alfaro, F., Quesada, M. A. et al. (2011). Strawberry fruit softening: Role of cell wall disassembly and its manipulation in transgenic plants. Genes, Genomes and Genomics, 5, 40–48. [Google Scholar]
39. Kyriacou, M. C., Rouphael, Y. (2018). Towards a new definition of quality for fresh fruits and vegetables. Scientia Horticulturae, 234, 463–469. [Google Scholar]
40. Sultan, M., Hafez, O. M., Saleh, M. A., Youssef, A. M. (2021). Smart edible coating films based on chitosan and beeswax-pollen grains for the postharvest preservation of Le Conte pear. RSC Advances, 11, 9572–9585. [Google Scholar] [PubMed]
41. Zhang, H., Mahunu, G. K., Castoria, R., Apaliya, M. T., Yang, Q. (2017). Augmentation of biocontrol agents with physical methods against postharvest diseases of fruits and vegetables. Trends in Food Science and Technology, 69, 36–45. [Google Scholar]
42. Mutha, R. E., Tatiya, A. U., Surana, S. J. (2021). Flavonoids as natural phenolic compounds and their role in therapeutics: An overview. Future Journal of Pharmaceutical Sciences, 7, 1–13. [Google Scholar]
43. Ippolito, A. (2008). Storage and shelf life of fresh fruits, vegetables and cut flowers. Microbial Biotechnology in Horticulture, 2, 259. [Google Scholar]
44. Wei, W. E., Zhongqi, F. A., Jianye, C. H., Kuang, J., Wangjin, L. U. et al. (2017). A banana PHD-type transcription factor MaPHD1 represses a cell wall-degradation gene MaXTH6 during fruit ripening. Horticultural Plant Journal, 3, 190–198. [Google Scholar]
45. Centeno, D. C., Osorio, S., Nunes-Nesi, A., Bertolo, A. L., Carneiro, R. T. et al. (2011). Malate plays a crucial role in starch metabolism, ripening, and soluble solid content of tomato fruit and affects postharvest softening. The Plant Cell, 23, 162–184. [Google Scholar] [PubMed]
46. Ghosh, M., Singh, A. K. (2022). Potential of engineered nanostructured biopolymer based coatings for perishable fruits with Coronavirus safety perspectives. Progress in Organic Coatings, 163(9), 106632. [Google Scholar] [PubMed]
47. Ankolekar, C., Sarkar, D., Greene, D., Shetty, K. (2021). Using biological elicitation to improve type 2 diabetes targeted food quality of stored apple. Frontiers in Sustainable Food Systems, 5, 709384. [Google Scholar]
48. Mahajan, P. V., Caleb, O. J., Gil, M. I., Izumi, H., Colelli, G. et al. (2017). Quality and safety of fresh horticultural commodities: Recent advances and future perspectives. Food Packaging and Shelf Life, 14(5), 2–11. [Google Scholar]
49. Shewfelt, R. L., Del, R. B. A. (2000). The role of lipid peroxidation in storage disorders of fresh fruits and vegetables. HortScience, 35(4), 575–579. [Google Scholar]
50. Zhang, W., Jiang, W. (2019). UV treatment improved the quality of postharvest fruits and vegetables by inducing resistance. Trends in Food Science and Technology, 92(3), 71–80. [Google Scholar]
51. Archana, T. J., Gogoi, R., Kaur, C., Varghese, E., Sharma, R. R. et al. (2021). Bacterial volatile mediated suppression of postharvest anthracnose and quality enhancement in mango. Postharvest Biology and Technology, 177, 111525. [Google Scholar]
52. Xu, M., Shen, C., Zhu, Q., Xu, Y., Xue, C. et al. (2022). Comparative metabolomic and transcriptomic analyses revealed the differential accumulation of secondary metabolites during the ripening process of acerola cherry (Malpighia emarginata) fruit. Journal of the Science of Food and Agriculture, 102, 1488–1497. [Google Scholar] [PubMed]
53. Gao, J., Chen, B., Lin, H., Liu, Y., Wei, Y. et al. (2020). Identification and characterization of the glutathione S-transferase (GST) family in radish reveals a likely role in anthocyanin biosynthesis and heavy metal stress tolerance. Gene, 743, 144484. [Google Scholar] [PubMed]
54. Dos, S., Nascimento, L. B., Brunetti, C., Agati, G., Lo, I. C. et al. (2020). Short-term pre-harvest UV-B supplement enhances the polyphenol content and antioxidant capacity of Ocimum basilicum leaves during storage. Plants, 9, 797. [Google Scholar]
55. Redenbaugh, K., Hiatt, W., Martineau, B., Emlay, D. (1994). Regulatory assessment of the FLAVR SAVR tomato. Trends in Food Science and Technology, 5, 105–110. [Google Scholar]
56. Samuolienė, G., Brazaitytė, A., Vaštakaitė, V. (2017). Light emitting diodes for agriculture, pp. 149–190. Singapore: Springer. [Google Scholar]
57. Gao, J., Zhang, Y., Li, Z., Liu, M. (2020). Role of ethylene response factors (ERFs) in fruit ripening. Food Quality and Safety, 4(1), 15–20. [Google Scholar]
58. Erdmann, R. M., Picard, C. L. (2020). RNA-directed DNA methylation. PLoS Genetics, 16(10), 1009034. [Google Scholar]
59. Agarwal, G., Choudhary, D., Singh, V. P., Arora, A. (2012). Role of ethylene receptors during senescence and ripening in horticultural crops. Plant Signaling and Behavior, 7(7), 827–846. [Google Scholar] [PubMed]
60. Martel, C., Vrebalov, J., Tafelmeyer, P., Giovannoni, J. J. (2011). The tomato MADS-box transcription factor RIPENING INHIBITOR interacts with promoters involved in numerous ripening processes in a COLORLESS NONRIPENING-dependent manner. Plant Physiology, 157(3), 1568–1579. [Google Scholar] [PubMed]
61. Dolgikh, V. A., Pukhovaya, E. M., Zemlyanskaya, E. V. (2019). Shaping ethylene response: The role of EIN3/EIL1 transcription factors. Frontiers in Plant Science, 10, 1030. [Google Scholar] [PubMed]
62. Duarte-Sierra, A., Tiznado-Hernández, M. E., Jha, D. K., Janmeja, N., Arul, J. (2020). Abiotic stress hormesis: An approach to maintain quality, extend storability, and enhance phytochemicals on fresh produce during postharvest. Comprehensive Reviews in Food Science and Food Safety, 19, 3659–3682. [Google Scholar] [PubMed]
63. Gerszberg, A., Hnatuszko-Konka, K., Kowalczyk, T., Kononowicz, A. K. (2015). Tomato (Solanum lycopersicum L.) in the service of biotechnology. Plant Cell, Tissue and Organ Culture, 120, 881–902. [Google Scholar]
64. Ohnmar, W. T., Srilaong, V., Lay, K. K., Poomputsa, K., Kanlayanarat, S. (2006). Biochemical and physiological changes during chlorophyll degradation in lime (Citrus aurantifolia Swingle cv. ‘Paan’). Journal of Horticultural Science and Biotechnology, 81, 471–477. [Google Scholar]
65. Ghosh, A., Saha, I., Debnath, S. C., Hasanuzzaman, M., Adak, M. K. (2021). Chitosan and putrescine modulate reactive oxygen species metabolism and physiological responses during chili fruit ripening. Plant Physiology and Biochemistry, 163, 55–67. [Google Scholar] [PubMed]
66. Yang, X., Song, J., Campbell-Palmer, L., Fillmore, S., Zhang, Z. (2013). Effect of ethylene and 1-MCP on expression of genes involved in ethylene biosynthesis and perception during ripening of apple fruit. Postharvest Biology and Technology, 78, 55–66. [Google Scholar]
67. Wang, N., Chen, H., Nonaka, S., Sato-Izawa, K., Kusano, M. et al. (2018). Ethylene biosynthesis controlled by NON-RIPENING: A regulatory conflict between wounding and ripening. Plant Physiology and Biochemistry, 132, 720–726. [Google Scholar] [PubMed]
68. Famiani, F., Farinelli, D., Palliotti, A., Moscatello, S., Battistelli, A. et al. (2014). Is stored malate the quantitatively most important substrate utilised by respiration and ethanolic fermentation in grape berry pericarp during ripening? Plant Physiology and Biochemistry, 76, 52–57. [Google Scholar] [PubMed]
69. Lopez, D., Carazo, N., Rodrigo, M. C., Garcia, J. (2006). Coloured shade nets effects on tomato crops quality. Acta Horticulturae, 747, 121–124. [Google Scholar]
70. Pataro, G., Sinik, M., Capitoli, M. M., Donsì, G., Ferrari, G. et al. (2015). The influence of post-harvest UV-C and pulsed light treatments on quality and antioxidant properties of tomato fruits during storage. Innovative Food Science & Emerging Technologies, 30(1), 103–111. [Google Scholar]
71. Alba, R., Cordonnier-Pratt, M. M., Pratt, L. H. (2000). Fruit localized phytochromes regulate lycopene accumulation independently of ethylene production in tomato. Plant Physiology, 123(1), 363–370. [Google Scholar] [PubMed]
72. Farcuh, M., Tajima, H., Lerno, L. A., Blumwald, E. (2022). Changes in ethylene and sugar metabolism regulate flavonoid composition in climacteric and non-climacteric plums during postharvest storage. Food Chemistry: Molecular Sciences, 4, 100075. [Google Scholar] [PubMed]
73. Liu, B., Xin, Q., Zhang, M., Chen, J., Lu, Q. et al. (2022). Research progress on mango post-harvest ripening physiology and the regulatory technologies. Foods, 12, 173. [Google Scholar] [PubMed]
74. Cordero, C., Squara, S., Caratti, A., Alladio, E., Vincenti, M. et al. (2022). Unlocking the future of comprehensive two-dimensional chromatography in food-omics by artificial intelligence algorithms. Atti del XXIX Congresso della Divisione di Chimica Analitica della Società Chimica Italiana, Messina, Italy. [Google Scholar]
75. Lu, H., Yadav, V., Zhong, M., Bilal, M., Taherzadeh, M. J. et al. (2022). Bioengineered microbial platforms for biomass-derived biofuel production—a review. Chemosphere, 288, 132528. [Google Scholar] [PubMed]
76. Cao, S., Hu, Z., Pang, B. (2010). Optimization of postharvest ultrasonic treatment of strawberry fruit. Postharvest Biology and Technology, 55, 150–153. [Google Scholar]
77. Leonova, T., Popova, V., Tsarev, A., Henning, C., Antonova, K. et al. (2020). Does protein glycation impact on the drought-related changes in metabolism and nutritional properties of mature pea (Pisum sativum L.) seeds. International Journal of Molecular Sciences, 21, 567. [Google Scholar] [PubMed]
78. Helaly, M. N., El-Hoseiny, H. M., Elsheery, N. I., Kalaji, H. M., de Los Santos-Villalobos, S. et al. (2022). 5-Aminolevulinic acid and 24-epibrassinolide improve the drought stress resilience and productivity of banana plants. Plants, 11(6), 743. [Google Scholar] [PubMed]
79. Li, W., Lei, X., Feng, H., Li, B., Kong, J. (2022). Layer-by-layer cell encapsulation for drug delivery: The history, technique basis, and applications. Pharmaceutics, 14(2), 297. [Google Scholar] [PubMed]
80. Pirsa, S., Sani, I. K., Mirtalebi, S. S. (2022). Nano-biocomposite based color sensors: Investigation of structure, function, and applications in intelligent food packaging. Food Packaging and Shelf Life, 31(1), 100789. [Google Scholar]
Cite This Article
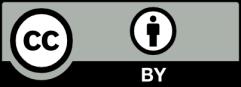
This work is licensed under a Creative Commons Attribution 4.0 International License , which permits unrestricted use, distribution, and reproduction in any medium, provided the original work is properly cited.