Open Access
ARTICLE
Antibacterial Activity and Mechanisms of Ethanol Extracts from Scutellaria baicalensis Georgi and Magnolia officinalis against Phytophthora nicotianae
College of Agronomy, Hunan Agricultural University, Changsha, 410128, China
* Corresponding Author: Qiang Li. Email:
Phyton-International Journal of Experimental Botany 2023, 92(7), 1987-1999. https://doi.org/10.32604/phyton.2023.027318
Received 24 October 2022; Accepted 26 January 2023; Issue published 29 May 2023
Abstract
Phytophthora nicotianae causes substantial economic losses in most countries where tobacco is produced. At present, the control of P. nicotianae mainly depends on chemical methods, with considerable environmental and health issues. We investigated the effects of ethanol extracts from Scutellaria baicalensis Georgi (SBG) and Magnolia officinalis (MO). On mycelial growth, sporangium formation, and zoospore release of P. nicotianae. Both extracts inhibited the growth of P. nicotianae, with mycelial growth inhibition rates of 88.92% and 93.92%, respectively, at 40 mg/mL, and EC50 values of 5.39 and 5.74 mg/mL, respectively. The underlying mechanisms were the inhibition of sporangium formation, the reduction of zoospore number, and the destruction of the mycelium structure. At an SBG extract concentration of 16.17 mg/mL, the inhibition rates for sporangia and zoospores were 98.66% and 99.39%, respectively. At an MO extract concentration of 2.87 mg/mL, the production of sporangia and zoospores was completely inhibited. The hyphae treated with the two plant extracts showed different degrees of deformation and damage. Hyphae treated with SBG extract showed adhesion and local swelling, whereas treatment with MO extract resulted in broken hyphae. Mixture of the extracts resulted in a good synergistic effect.Keywords
Pathogens within the oomycete genus Phytophthora are among the most destructive plant pathogens globally, causing disease and significant losses in important agricultural and forestry crops [1,2]. The genus Phytophthora consists of nearly 200 described species, such as P. infestans, P. sojae, and P. nicotianae. Of these, P. infestans is the causal agent of potato late blight, which is still regarded as one of the most devastating plant pathogens and continues to cause approximately 50 billion USD in lost revenue annually [3–5]. Phytophthora sojae is responsible for estimated annual yield losses of 200 million USD in the United States and 1–2 billion USD worldwide [6,7].
Phytophthora nicotianae damages all cultivated tobacco varieties, including cured, sun-cured, burley, and aromatic tobacco, is highly destructive, and can infect tobacco at different growth periods [8,9]. The main primary infection sources of P. nicotianae are fungus-carrying soil, manure, and irrigation water [9,10]. The sporangium and zoospores produced on diseased plants can also lead to secondary infection by rain or irrigation water [11]. Under natural conditions, viable zoospores are released from the sporangium, and disease occurrence is positively correlated with the sporangium number [12].
Currently, most of the commonly used methods to prevent tobacco black shank disease in agriculture are chemical control methods, applying fungicides such as metalaxyl, propamocarb, and dimethomorph [13]. Although chemical control methods are rapid, efficient, economical, and convenient, they result in the production of large amounts of residues that pollute the environment, making pathogens drug-resistant and threatening non-target organisms [14]. Consequently, there are considerable impacts on the yield and quality of tobacco and the prevention and treatment of black shank.
The search for high-efficiency, low-residue, and safe options to control tobacco black shank disease has therefore become a research hotspot. Studies have shown that plant extracts and botanical fungicides can potentially prevent this disease. For example, ragweed extract has a good inhibitory effect on the hyphae of Aspergillus flavus at 100 μg/mL [15]. The volatile oil extracted from the leaves of 22 species, including Eupatorium adenophorum, can significantly inhibit the activities of Erwinia herbicola and Pseudomonas putida, which cause fruit and vegetable rot [16]. Eucalyptus globulus essential oil has a good inhibitory effect on A. flavus and A. parasiticus [17].
Scutellaria baicalensis Georgi (SBG) and Magnolia officinalis (MO) are traditional herbal drug widely used in China, Japan and South Korea [18]. The principal active substances of SBG include baicalein, baicalin, wogonin and wogonoside, is generally applied to treat respiratory tract infections, pneumonia, control tumors and inhibit cardiovascular [19,20]; it also has a broad-spectrum antifungal activity [21]. MO is the main component of Chinese medicine formulae Banxia Houpo decoction, and Japanese formula Saibokuto and so on, which is widely used in clinic to improve abdominal distension, abdominal pain, asthma, depression and other symptoms. The main chemical components of MO are magnolol, honokiol, 4-methoxyhonokiol, which have attracted much attention due to their excellent activity [22].
However, the inhibitory effects of the extracts of SBG and MO on P. nicotianae are still largely unclear. This study investigates the effects of SBG and MO extracts on mycelium growth, sporangia, and zoospore release of P. nicotianae. The results provide a theoretical reference for the development and research of tobacco black shank disease plant-derived pesticides.
The P. nicotianae used in this experiment was obtained from the ‘Key Laboratory of Crop Stress Biology in Arid Areas’ led by Dr. Shan Weixing at the Northwest Agriculture and Forestry University in China.
The plant material consisted of epidermis and root bark of MO and root of SBG, purchased from Hunan Provincial Hospital of Traditional Chinese Medicine. For extraction, plant powder (10 g) was added into 100 mL ethanol (95%), and extraction was performed at 25°C via ultrasonication for 30 min. Subsequently, the mixture was filtered three times with medium-speed quantitative filter paper (Φ15 cm), and the obtained filtrate was concentrated in a vacuum rotary evaporator to obtain a paste, which was fixed at a concentration of l g/mL and stored at 4°C.
2.3 In Vitro Antifungal Experiment
The mycelial growth rate method was used to determine the inhibitory effects of the plant extracts and their compounds on the growth of tobacco black shank mycelium [23]. The final prepared plant extract solutions had five concentrations (40, 20, 10, 5, 1 mg/mL) and a volume of 20 mL. Under aseptic conditions, sterilized Oatmeal Agar (OA) medium was cooled to about 50°C, and the plant extracts were thoroughly mixed with the medium. For the control (CK), the same amount of ethanol was added to the petri dish (the final concentration of ethanol in the petri dish was 3.8%). A sterilized punch with diameter of 0.5 cm was used to beat the fungus-containing medium block on the edge of the cultivated fungal colony, which was inoculated to the prepared medicated medium separately. After culturing in the dark in a 26°C incubator for 5 days, the diameter of the colony was measured by the cross method. All treatments and controls were replicated four times. The percentage of inhibition was calculated according to Eq. (1).
where 0.5 is the diameter of the fungus-containing medium block. Where D and d represent the diameter of the control and treated colonies, respectively. In the toxicity calculation method, the percentage of growth inhibition is converted into a probability value by checking the conversion table of the biometric probability values. The toxicity to establish the regression equation is calculated as y = a + bx, and the EC50 (median effect concentration) is determined. Here, y represents the probability value of the colony growth inhibition rate, and x represents the logarithm of the different mass concentrations of the extract.
2.4 In Vitro Sporangium Germination and Zoospore Release Test
According to the method proposed by Mulugeta et al. [24], in vitro experiments were performed to determine the inhibitory effects of the two plant extracts on sporangium germination and zoospore release. Under aseptic conditions, the extract was uniformly mixed with sterilized 10% V8 culture solution. Finally, a medium containing extract at five concentrations (1/2EC50, 1EC50, 2EC50, and 3EC50) and a control (CK) with sterile water were prepared. The experiment was done in duplicate.
We transferred 10 pieces of fresh P. nicotianae mycelium (approximately 2 mm × 2 mm in size) to the prepared 10% V8 fluid extract medium (including control) and cultured them in a 26°C incubator in the dark for 3 days. Subsequently, the mycelium was removed and washed with sterile water. After this, 20 mL of sterile water was added to the petri dish for suspension, followed by 5 drops of filtered and sterilized soil extract; the mixture was cultured in a 26°C incubator. The water was changed every 12 h, adding new soil extract, and after 48 h, the number of sporangia was determined under a 10 × 20 optical microscope; colonies were randomly checked in three fields. The stimulated mycelium that produced a large number of sporangia was placed in a refrigerator at 4°C for 30 min and subsequently incubated at 26°C for 30 min until the zoospores were released. A micro-sampler was used to absorb 4 µL of zoospore suspension onto a clean glass slide and draw out short, thin strips. Zoospore number was determined under a 10 × 10 optical microscope at four replications. The zoospore concentration of the suspension (zoospores/mL) was calculated according to Eq. (2).
2.5 Changes in Mycelium Morphology under the Electron Microscope
Two plant extracts with a concentration of EC50 were used to cultivate OA as described above. The same amount of sterile water (CK1) and 95% ethanol (CK2) were used for the control. After culturing in an incubator at 26°C for 5 days, the hyphae were cut from the edge of the fungal colony and observed under an electron microscope to obtain the morphology and structure.
2.6 Evaluation of the Antifungal Effects of the Two Extracts in Vitro
The plant extract solutions were mixed at five volume ratios (1:9, 3:7, 5:5, 7:3, and 9:1) to obtain the formulations, with medium concentrations of 10, 7.5, 5, 2.5, and 1 mg/mL. The same amount of 95% ethanol as that in the control was used. The antifungal activity was determined by the mycelial growth rate method. According to Yang et al. [25], the synergistic ratio (SR) was applied to determine the antifungal activity of the different complex combinations. An SR < 0.5 was antagonistic, SR = 0.5–1.5 indicated an additive effect, and an SR > l.5 was synergistic.
The inhibition percentage of each compound to P. nicotianae was calculated according to Eq. (1). The value of EC50 was calculated by the toxicity regression equation, and the theoretical EC50 was calculated according to Eq. (3).
where y represents the theoretical EC50, a and b represent the proportions of the two components in the mixture, and A and B represent the EC50 values of the SBG and MO extracts.
The synergistic ratio (SR) was calculated according to Eq. (4).
where y and y1 represent the theoretical and the measured EC50, respectively.
The data were analyzed using the Origin 2018 software. Significance was tested using the Least Significant Difference (LSD) test with 95% and 99% confidence levels in SPSS 23.0.
4.1 Inhibitory Effects of Plant Extracts on P. nicotianae
As shown in Fig. 1, the ethanol extracts of the two plants showed a significant dose-dependent inhibition of P. nicotianae. At concentrations of 40, 20, and 10 mg/mL, the inhibition rates of the MO extract on mycelial growth were 92.93%, 86.04%, and 64.41%, respectively, which were significantly higher than the corresponding values of the SBG extract (88.29%, 78.83%, and 50.45%, respectively). At concentrations of 5 and 1 mg/mL, the inhibition rates of MO extract on mycelial growth were 31.53% and 13.29%, respectively, which were lower than the corresponding inhibition rates of the SBG extract (35.81% and 28.60%, respectively). This shows that the inhibitory effect of MO extract on the growth of P. nicotianae hyphae at high concentrations is better than that of SBG extract, but it has a stronger dose dependence. At the same time, the inhibitory effect of the ethanol extracts of the two plants on the growth of P. nicotianae hyphae at each concentration was considerably higher than that of the ethanol treatment at the same concentration, indicating that the ethanol in the extract solution does not play a dominant role.
Figure 1: Inhibition effects of the two plant ethanol extracts on P. nicotianae
Note: Different capital letters above the histograms represent extreme significance among treatments (p < 0.01); Different lower-case letters above the histograms represent significance among treatments (LSD determination) (p < 0.05).
As shown in Table 1, the virulence regression equation was calculated according to the inhibition rates of the five treatment concentrations of the extract against P. nicotianae, and the EC50 was calculated. The virulence correlation of each extract to P. nicotianae was relatively good, with coefficient of determination values above 0.8, indicating that the regression equation has a high degree of fit. Based on the EC50 value, the overall inhibitory effect of SBG extract on P. nicotianae is better than that of MO extract.
4.2 Effects of Ethanol Extracts from Two Plants on Sporangium Production and the Release of Zoospores of P. nicotianae
As shown in Fig. 2A, the two plant extracts at 1/2 EC50, EC50, 2 EC50, and 3 EC50 had highly significant inhibitory effects on sporangium production and zoospore release of P. nicotianae. Regarding the sporangium production of a single hypha, the MO extract completely inhibited sporangium production at a concentration of EC50 (5.74 mg/mL) or above. The inhibitory effect of the SBG extract was slightly weaker, and at concentrations above EC50 (5.39 mg/mL), it also significantly inhibited sporangium production, although the inhibition rates did not reach a significant level. However, when the concentration was 1/2 EC50 (2.87 mg/mL), the inhibitory rate of MO extract on sporangium production reached 98.18%, which is significantly higher than the 74.66% obtained with the SBG extract at 1/2 EC50 (2.70 mg/mL).
Figure 2: (A) Effects of the two plant extracts on sporangium production of P. nicotianae, (B) Effects of the two plant extracts on zoospore release of P. nicotianae
Note: Different capital letters above the histograms represent extreme significance among treatments (p < 0.01); Different lower-case letters above the histograms represent significance among treatments (LSD determination) (p < 0.05).
As seen in Fig. 2B, regarding the release of zoospores, the inhibition rate of the MO extract at each concentration reached 100%. Inhibition was significantly higher at 2 EC50 (11.48 mg/mL) compared to that of the SBG extract at 2 EC50 (10.78 mg/mL), which was 97.58%, and its concentration at 1/2 EC50 (2.87 mg/mL) and (2.87 mg/mL) is extremely significantly higher than the inhibition rate of SBG extract at 1/2 EC50 (2.70 mg/mL) and (5.39 mg/mL) at 82.73% and 92.42%, respectively.
4.3 Effects of Plant Extracts on Mycelial Morphology of P. nicotianae
As shown in Fig. 3, the hyphae of the blank control and ethanol treatment showed a normal morphology; they were slender and uniform, the surface was smooth, and there was no diaphragm. After treatment with the two plant extracts, the hyphae showed obvious differences in morphology, indicating that it was not the ethanal that caused the morphological changes. The hyphae treated with plant extracts were observed under a scanning electron microscope. Compared with the blank control, the following morphological abnormalities were found: hyphae treated with SBG extract and observed under a ×1,000 electron microscope showed obvious adhesion (Fig. 3C); under ×5,000 magnification, hyphae appeared swollen and partially broken (Fig. 3D). Hyphae treated with MO extract showed ruptured walls and were broken (Figs. 3G and 3H).
Figure 3: Hyphae of P. nicotianae under a scanning electron microscope
Note: (A) (×5,000) CK1; (B) (×1,000) CK1; (C) (×5,000) CK2; (D) (×1,000) CK2; (E) (×5,000), SBG extract at a concentration of EC50; (F) (×1,000), SBG extract at a concentration of EC50; (G) (×5,000), MO extract at a concentration of EC50; (H) (×1,000), MO extract at a concentration of EC50.
4.4 In Vitro Antifungal Effect of Ethanol Extracts
As shown in Fig. 4, compared to using one of the plant extracts alone, the combination of SBG and MO extracts showed a higher antifungal effect. At 10 mg/mL, the inhibitory rates reached 82.07%, 83.54%, 80.17%, 79.75%, and 76.16%, respectively. According to the EC50 and SR values under each compound volume ratio in Table 2, when the compound volume ratio was 3:7 (v:v), the actual EC50 was the smallest, with 2.63 mg/mL, and the SR SR value is the largest, with 2.137. This shows that this volume ratio is the most suitable one for the combination of the two plant extracts. At compound ratios of 1:9 (v:v) and 7:3 (v:v), the SR values were 1.828 and 1.523, respectively, both greater than 1.5. This leads us to infer that the two plant extracts have a synergistic effect at this compounding volume ratio. In addition, when the compound volume ratios were 5:5 (v:v) and 9:1 (v:v), the SR values were 1.373 and 1.391, also greater than 0.5, indicating an additive effect. According to Fig. 5, with an increasing volume ratio of the complex, the compound inhibitory effect first increased and then decreased gradually, stabilizing at a volume ratio of 3:7 (v:v).
Figure 4: Inhibition rate effects of compound SBG and MO extracts on P. nicotianae at different volume ratios
Note: Different lower-case letters above the histograms represent significance among treatments (LSD determination) (p < 0.05).
Figure 5: Synergistic trend of the combination of SBG and MO extracts at different volume ratios. SBG = Scutellaria baicalensis Georgi, MO = Magnolia officinalis
Although chemical pesticides can well control the occurrence of fungal diseases, they have the disadvantages of high residues, environmental pollution, drug resistance induction, and phytotoxicity to non-target organisms [7]. It is therefore crucial to find environmentally friendly approaches.
In recent years, numerous scientists have investigated the fungicidal activity of plant extracts. Park et al. [26] reported that 27 plants, including Acorus calamus L., Frankincense, Artemisia vulgaris L., Saussurea lappa Clarke, and Cnidium officinale Makion, have inhibitory effects on six fungal species that damage gramineous plants. Maswada et al. [27] found that Cyperus mitis, Asparagus L., and Stipagrostis Nees plant methanol extracts had inhibitory effects on Alternaria solani (Ell.et Mart.) Jones et Grout., Aspergillus niger, and Rhizopus stolonifer. Yosef et al. [28] reported that the water, ethanol, and petroleum ether extracts of 12 species of Momordica charantia showed different antifungal activities against melon Fusarium wilt, potato anthracnose, and early blight. Dai et al. [29] found that ethanol extracts of Psoralen and Sophora flavescens can inhibit the growth of P. nicotianae in vitro by affecting the mycelial structure. According to Liu [30], the methanol extract of Eupatorium adenophorum leaves has a high antifungal activity against oomycete pathogens, with sesquiterpene being the main active compound. These studies have provided a theoretical basis for the use of plant extracts to control fungal diseases, paving the way for the application of these extracts in the prevention and treatment of fungal diseases.
The results of this study showed that the ethanol extracts of SBG and MO showed different levels of inhibitory effects on the growth of P. nicotianae: at higher concentrations (40, 20, and 20 mg/mL), the MO extract had a higher inhibitory effect on mycelial growth. However, at lower concentrations (5 and 1 mg/mL), the SBG extract showed a stronger inhibitory effect on mycelial growth. For both plant extracts, the effects were dose-dependent. We also observed significant inhibitory effects on sporangium production and zoospore release of P. nicotianae, which is inconsistent with the effects on hyphal growth at lower concentrations. We assume that at lower concentrations, the SBG extract exerts a higher inhibitory effect on active mitosis, biofilm synthesis, and signal transduction activities of the mycelium in the growth stage than the MO extract, which is in agreement with the findings of Kim [31]. In contrast, the MO extract seems to have a better inhibitory effect on the more active respiration during the spore germination stage.
Numerous studies have shown that the plant secondary metabolites in plant extracts have good inhibitory effects on fungi, but the inhibition mechanism is not the same as that for pathogenic microorganisms. Pandima et al. [32] found that the eugenol in cloves can inhibit the activity of pathogens by destroying their plasma membrane. Its values of minimum inhibitory concentration and minimum bactericidal concentration are 125 and 250 mg/L, respectively. Lin et al. [33] reported nine plant secondary metabolites in Notopterygium incisum that can inhibit the germination and formation of spores of apple fruit fungi to inhibit pathogen activity, with MIC values of 8 to 250 mg/L. Vuko et al. [34] found that plant secondary metabolites can also control the occurrence of diseases by activating plant defenses. According to Mehdi et al. [35], Azadirachta indica A. Juss. Leaves and seeds can alter the cytoplasm of Aspergillus parasiticus mycelium, thereby affecting the activity of the pathogen.
In the present study, the scanning electron microscope results also revealed the antifungal mechanism of the two plant extracts. The morphology of the mycelium of P. nicotianae after treatment with plant extracts showed obvious differences. In the blank control and ethanol treatment, the hyphae were normal, slender, and uniform, with a smoot surface without septa. However, after treatment with SBG extract, the hyphae showed adhesion, local swelling, and damage. Hyphae treated with the MO extract had ruptured and broken mycelial walls, indicating that MO extract inhibits fungal growth by destroying the hyphae, with a lethal effect on P. nicotianae. In contrast, SBG extract inhibits fungal growth through local expansion and adhesion of the mycelium, with a less pronounced lethal effect. This is in agreement with previous findings [21]. The combination of the two plant extracts showed superior results, which is consistent with the findings of previous studies [36,37]. This can be explained by the diversity of antifungal components and the different antifungal mechanisms in the two plant extracts [37].
Although we studied the in vitro inhibitory effects of SBG and MO extracts on P. nicotianae, the antifungal components and mechanisms of the two plant extracts are still largely unclear. Further inoculation with live plants should be carried out, and in vivo tests are needed to fully support the application of the two plant extracts in the prevention and control of fungal diseases.
Ethanol extracts of both SBG and MO can effectively control P. nicotianae. The inhibition rates of SBG and MO extracts on mycelial growth were 88.92% and 93.92%, respectively, with EC50 values of 5.39 and 5.74 mg/mL, respectively. For both extracts, the underlying mechanisms of action were inhibition of sporangium formation, reduction in the number of zoospores, destruction of the mycelium structure, and mycelium growth inhibition. However, to support these findings and to facilitate the large-scale application of these extracts in the prevention and control of fungal diseases, further in vivo studies are needed.
Funding Statement: This research was funded by financial grants from the Education Department of Hunan Province (SCX1840 and CX20190515).
Author Contributions: The authors confirm their contribution to the paper as follows: study conception and design: X. Gao, Q. Li; data collection: Z. Q. Shen; draft manuscript preparation: J. L. Xu, M. Fan. All authors reviewed the results and approved the finalversion of the manuscript.
Conflicts of Interest: The authors declare that they have no conflicts of interest to report regarding the present study.
References
1. El-Hasan, A., Ngatia, G., Link, T. I., Voegele, R. T. (2022). Isolation, identification, and biocontrol potential of root fungal endophytes associated with solanaceous plants against potato late blight (Phytophthora infestans). Plants, 11(12), 1605. https://doi.org/10.3390/plants11121605 [Google Scholar] [PubMed] [CrossRef]
2. Midgley, K. A., van den Berg, N., Swart, V. (2022). Unraveling plant cell death during Phytophthora infection. Microorganisms, 10(6), 1139. https://doi.org/10.3390/microorganisms10061139 [Google Scholar] [PubMed] [CrossRef]
3. Zhou, Y., Yang, K., Yan, Q., Wang, X., Cheng, M. et al. (2021). Targeting of anti-microbial proteins to the hyphal surface amplifies protection of crop plants against Phytophthora pathogens. Molecular Plant, 14(8), 1391–1403. https://doi.org/10.1016/j.molp.2021.05.007 [Google Scholar] [PubMed] [CrossRef]
4. Leesutthiphonchai, W., Vu, A. L., Ah-Fong, A. M. V., Judelson, H. S. (2018). How does Phytophthora infestans evade control efforts? Modern insight into the late blight disease. Phytopathology, 108(8), 916–924. https://doi.org/10.1094/PHYTO-04-18-0130-IA [Google Scholar] [PubMed] [CrossRef]
5. Wu, J., Xue, Z., Miao, J., Zhang, F., Gao, X. et al. (2020). Sensitivity of different developmental stages and resistance risk assessment of Phytophthora capsici to fluopicolide in China. Frontiers in Microbiology, 11, 185. https://doi.org/10.3389/fmicb.2020.00185 [Google Scholar] [PubMed] [CrossRef]
6. Wang, W., Chen, L., Fengler, K., Bolar, J., Llaca, V. et al. (2021). A giant NLR gene confers broad-spectrum resistance to Phytophthora sojae in soybean. Nature Communications, 12(1), 1–8. https://doi.org/10.1038/s41467-021-26554-8 [Google Scholar] [PubMed] [CrossRef]
7. Zhang, C., Cui, T., Zhang, F., Xue, Z., Miao, J. et al. (2020). Identification of differentially activated pathways in Phytophthora sojae at the mycelial, cyst, and oospore stages by TMT-based quantitative proteomics analysis. Journal of Proteomics, 221, 103776. https://doi.org/10.1016/j.jprot.2020.103776 [Google Scholar] [PubMed] [CrossRef]
8. Wang, W. J., Zhang, J. H., Cao, Y., Yang, X. Y., Wang, F. L. et al. (2022). NtbHLH49, a jasmonate-regulated transcription factor, negatively regulates tobacco responses to Phytophthora nicotianae. Frontiers in Plant Science, 13, 1073856. https://doi.org/10.3389/fpls.2022.1073856 [Google Scholar] [PubMed] [CrossRef]
9. van Breda, D. H. (1896). De Bibitziekte in de Del-itabak veroorzaakt door Phytophthora nicotianae. Slands Plentuim, 1896(15), 1–107. [Google Scholar]
10. Ma, S. G., Gao, Z. M., Chen, J. (2003). Research progress of tobacco black tibia bacteria (I). Tobacco Science and Technology, 2003(4), 35–42. [Google Scholar]
11. Duniway, J. M. (1983). Role of physical factors in the development of Phytophthora diseases//Erwin DC, Bartnicki GS, Tsao PH. Phytophthora: It biology taxonomy ecology and pathology. AGRIS, 1983, 175–188. [Google Scholar]
12. Yu, Q., Yang, S. Y., Wang, H., Li, Z. L. (2017). Effect of boron on mycelial growth, sporangiogenesis and zoosporogenesis of Phytophthora nicotianae and the possible inhibitory mechanisms. European Journal of Plant Pathology, 149(4), 945–952. [Google Scholar]
13. Ren, X. F., Qi, W. Z., Cheng, X. K., Liu, Z. J., Ji, X. X. et al. (2018). Effect of fluorobacteria propamocarb on tobacco black tibia and its effect on the growth of tobacco strains. Acta Tabacaria Sinica, 24(4), 129–134. [Google Scholar]
14. Lucía da, C. C., Virginia, F. P., Andrea, P. (2013). Application of plant derived compounds to control fungal spoilage and mycotoxin production in foods. International Journal of Food Microbiology, 166(1), 1–14. https://doi.org/10.1016/j.ijfoodmicro.2013.05.026 [Google Scholar] [PubMed] [CrossRef]
15. Kumar, R., Mishra, A. K., Dubey, N. K., Tripathi, Y. B. (2007). Evaluation of chenopodium ambrosioides oil as a potential source of antifungal, antiaflatoxigenic and antioxidant activity. International Journal of Food Microbiology, 115(2), 159–164. https://doi.org/10.1016/j.ijfoodmicro.2006.10.017 [Google Scholar] [PubMed] [CrossRef]
16. Abhay, K. P., Manindra, M., Pooja, S., Uma, T. P., Tripathi, N. N. (2014). Chemical composition, antifungal and antioxidant activity of essential oil of Eupatorium adenophorum Spreng. from Eastern Uttar Pradesh, India. Food Bioscience, 7, 80–87. https://doi.org/10.1016/j.fbio.2014.06.001 [Google Scholar] [CrossRef]
17. Georgia, R. V., Gustavo, S. D. A., Marisa, A. B. R. D., Maria, H. D. M., José, O. B. et al. (2008). Activity of essential oil and its major compound, 1,8-cineole, from Eucalyptus globulus Labill., against the storage fungi Aspergillus flavus link and Aspergillus parasiticus speare. Journal of Stored Products Research, 45(2), 108–111. https://doi.org/10.1016/j.jspr.2008.10.006 [Google Scholar] [CrossRef]
18. Raison-Peyron, N., Césaire, A., Du-Thanh, A., Dereure, O. (2015). Allergic contact dermatitis caused by Magnolia officinalis bark extract in a facial anti-ageing cream. Contact Dermatitis, 72(6), 416–417. https://doi.org/10.1111/cod.12372 [Google Scholar] [PubMed] [CrossRef]
19. Nilausen, T. D., Johansen, J. D., Thyssen, J. P. (2016). Allergic contact dermatitis of the face caused by Magnolia officinalis bark extract. Contact Dermatitis, 75(6), 385–387. https://doi.org/10.1111/cod.12655 [Google Scholar] [PubMed] [CrossRef]
20. Liao, H. F., Ye, J., Gao, L. L., Liu, Y. L. (2021). The main bioactive compounds of Scutellaria baicalensis Georgi. for alleviation of inflammatory cytokines: A comprehensive review. Biomedicine and Pharmacotherapy, 133, 110917. https://doi.org/10.1016/j.biopha.2020.110917 [Google Scholar] [PubMed] [CrossRef]
21. Mélanie, P., Pierre, D. (2017). Biological activity and toxicity of the Chinese herb Magnolia officinalis Rehder & E. Wilson (Houpo) and its constituents. Journal of Zhejiang University-SCIENCE B, 18(3), 194–214. https://doi.org/10.1631/jzus.B1600299. [Google Scholar] [PubMed] [CrossRef]
22. Lin, N., Hou, Y. Y., Jiang, M., Bai, G. (2021). The rich pharmacological activities of Magnolia officinalis and secondary effects based on significant intestinal contributions. Journal of Ethnopharmacology, 281, 114524. https://doi.org/10.1016/j.jep.2021.114524 [Google Scholar] [PubMed] [CrossRef]
23. Svecova, E., Proietti, S., Caruso, S., Colla, G., Crino, P. (2013). Antifungal activity of Vitex agnus-castus extract against Pythium ultimum in tomato. Crop Protection, 43, 223–230. https://doi.org/10.1016/j.cropro.2012.10.008 [Google Scholar] [CrossRef]
24. Mulugeta, T., Abreha, K., Tekie, H., Mulatu, B., Yesuf, M. et al. (2019). Phosphite protects against potato and tomato late blight in tropical climates and has varying toxicity depending on the Phytophthora infestans isolate. Crop Protection, 121, 139–146. https://doi.org/10.1016/j.cropro.2019.03.019 [Google Scholar] [CrossRef]
25. Yang, X. P., Jiang, X. D., Chen, J. J., Zhang, S. S. (2013). Control of postharvest grey mould decay of nectarine by tea polyphenol combined with tea saponin. Letters in Applied Microbiology, 57(6), 502–509. https://doi.org/10.1111/lam.12139 [Google Scholar] [PubMed] [CrossRef]
26. Park, I. K., Kim, J., Lee, Y. S., Shin, S. C. (2008). In vivo fungicidal activity of medicinal plant extracts against six phytopathogenic fungi. International Journal of Pest Management, 54(1), 63–68. https://doi.org/10.1080/09670870701549665 [Google Scholar] [CrossRef]
27. Maswada, H. F., Abdallah, S. A. (2013). In vitro antifungal activity of three geophytic plant extracts against three post-harvest pathogenic fungi. Pakistan Journal of Biological Sciences, 16(23), 1698–1705. https://doi.org/10.3923/pjbs.2013.1698.1705 [Google Scholar] [CrossRef]
28. Yosef, B., Adi, J. L., Ekaterina, G., Carmela, H., Uzi, S. et al. (2010). Variation in antifungal activity in extracts from Momordica plants. Israel Journal of Plant Sciences, 58(1), 1–7. https://doi.org/10.1560/IJPS.58.1.1 [Google Scholar] [CrossRef]
29. Dai, M. L., Xie, Y., Yan, C. B., Liu, J. H., Zhang, Y. P. et al. (2020). Inhibition of ethanol extracts of Psoralea corylifolia and Sophora flavescens on Phytophthora nicotianae and their compound effect. International Journal of Agriculture and Biology, 23(1), 75–81. https://doi.org/10.17957/IJAB/15.1260 [Google Scholar] [CrossRef]
30. Liu, X. M. (2016). Study on antibacterial activity, mechanism of action and hydrolysis law of sesquiterpenoids in Eupatorium Adenophorum study on antibacterial activity, mechanism and hydrolysis of peniterpenes (Ph.D. Thesis). Chinese Academy of Agricultural Sciences, China. [Google Scholar]
31. Kim, Y. (2013). Antibiofilm activity of scutellaria baicalensis through the inhibition of synthesis of the cell wall (1,3)-;-D-Glucan polymer. Korean Journal of Microbiology and Biotechnology Letters, 41(1), 88–95. [Google Scholar]
32. Pandima, D. K., Arif Nisha, S., Sakthivel, R., Karutha Pandian, S. (2010). Eugenol (an essential oil of clove) acts as an antifungal agent against Salmonella typhi by disrupting the cellular membrane. Journal of Ethnopharmacology, 130(1), 107–115. https://doi.org/10.1016/j.jep.2010.04.025 [Google Scholar] [PubMed] [CrossRef]
33. Lin, X., Zhou, Y. M., Zhang, X. F., Du, F. Y. (2018). Notopterygium incisum extract and associated secondary metabolites inhibit apple fruit fungal pathogens. Pesticide Biochemistry and Physiology, 150, 59–65. https://doi.org/10.1016/j.pestbp.2018.07.001 [Google Scholar] [PubMed] [CrossRef]
34. Vuko, E., Rusak, G., Dunkic, V., Kremer, D., Kosalec, I. et al. (2019). Inhibition of satellite RNA associated cucumber mosaic virus infection by essential oil of Micromeria croatica (Pers.) Schott. Molecules, 24(7), 1342–1354. https://doi.org/10.3390/molecules24071342 [Google Scholar] [PubMed] [CrossRef]
35. Mehdi, R. A., Abdolamir, A., Taki, T., Masoomeh, S. G., Mehdi, G. (2005). Morphological alterations in toxigenic Aspergillus parasiticus exposed to neem (Azadirachta indica) leaf and seed aqueous extracts. Mycopathologia, 159(4), 565–570. https://doi.org/10.1007/s11046-005-4332-4 [Google Scholar] [PubMed] [CrossRef]
36. Li, Q., Dai, M. L., Xie, Y., Yan, C. B., Liu, J. H. et al. (2020). Inhibition of ethanol extracts of Psoralea corylifolia and Sophora flavescens on Phytophthora nicotianae and their compound effect. International Journal of Agriculture and Biology, 23, 75–81. https://doi.org/10.17957/IJAB/15.1260 [Google Scholar] [CrossRef]
37. Wang, R., Wang, S., Pan, W., Li, Q., Xia, Z. et al. (2018). Strategy of tobacco plant against black shank and tobacco mosaic virus infection via induction of PR-1, PR-4 and PR-5 proteins assisted by medicinal plant extracts. Physiological and Molecular Plant Pathology, 101(2), 127–145. https://doi.org/10.1016/j.pmpp.2017.07.002 [Google Scholar] [CrossRef]
Cite This Article
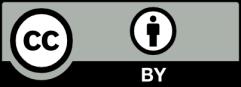
This work is licensed under a Creative Commons Attribution 4.0 International License , which permits unrestricted use, distribution, and reproduction in any medium, provided the original work is properly cited.