Open Access
ARTICLE
Repellent and Insecticidal Activity of Naphthoquinones from the Heartwood of Tectona grandis on Incisitermes marginipennis (Latreille)
1
Facultad de Ingeniería en Tecnología de la Madera, Universidad Michoacana de San Nicolás de Hidalgo, Morelia, México
2
Escuela Nacional de Estudios Superiores, Unidad Morelia, Morelia, México
3
Instituto de Investigaciones Químico-Biológicas, Universidad Michoacana de San Nicolás de Hidalgo, Morelia, México
* Corresponding Author: Crisanto Velázquez-Becerra. Email:
(This article belongs to the Special Issue: Plant Bioactive Compounds-Chemotaxonomic Significance, Antioxidant Properties, Potential Application as Food Ingredients as well as Therapeutics, and the Analytical Technology in Their Efficient Discovery)
Phyton-International Journal of Experimental Botany 2023, 92(7), 2165-2181. https://doi.org/10.32604/phyton.2023.026954
Received 11 October 2022; Accepted 27 February 2023; Issue published 29 May 2023
Abstract
Wood is a lignocellulosic material that, because of its organic nature, is a source of nutrients for bacteria, fungi, and insects. Incisitermes marginipennis (Latreille) is an interesting dry wood-boring termite. Because it is an extremely destructive pest, difficult to control due to its cryptic lifestyle, and for its role in the biogeochemical cycle of carbon and other elements; it is a fundamental organism. The use of less toxic and eco-friendly substances is important for protecting domestic, cultural, and historical heritage made of wood or lignocellulosic materials. The aim of this study was to evaluate the biological activity of various naphthoquinones extracted from the heartwood of T. grandis on I. marginipennis. First, heartwood flour was used for Soxhlet-type extractions to isolate and characterize the heartwood compounds by thin-layer chromatography. To identify the heartwood compounds, nuclear magnetic resonance and gas chromatography coupled to a mass spectrometer were used. The information obtained was supported using the Interactive Spectroscopy software. The identified heartwood compounds were lapachol, tectoquinone, deoxylapachol, dehydro-α-lapachone, tectol and tecomaquinone-I. Using nymphal termites of I. marginipennis as a biological model, the isolated and identified molecules were under three different tests (repellent, insecticide and protective) and their biological activity was individually evaluated. The results showed that the molecules obtained have a repellent-protective effect against I. marginipennis, although not in all cases, these molecules have high percentages of mortality and decreased the weight loss of the treated material exposed to I. marginipennis.Keywords
Nomenclature
LC50 | Lethal concentration 50 |
Tecomaq-I | Tecomaquinone-I |
D-α-L | Dehydro-α-lapachone |
Termites have invaded almost all natural habitats. Now, understanding its ecological impacts is just beginning. They are fundamental in the biogeochemistry of carbon cycling from cellulosic sources, such as wood and wooden articles obtained from a fast-growing tree or pioneer species with low-density wood. In particular, the wood of legumes is the most vulnerable to termites. However, the wood of slow-growing climax tree species is susceptible to termite delay. Termites are social organisms with diverse social and feeding behaviour, e.g., subterranean termites whose population is very large and shows rapid spread. In contrast, other termite species form colonies with few individuals in extreme niches [1]. However, all of them affect economically important agricultural and forestry crops.
The termite family Kalotermitidae comprises nearly five hundred species worldwide. Until 2019, one hundred fifty-eight termite species were described in American Continent [2]. Kalotermitids generally have their life cycle within the dry or wet wood, where they nest, and feed on cellulose. They boring the wood and building galleries affecting their aesthetic and physical-mechanic properties. Kalotermitids are sometimes collectively referred to as “drywood” termites. Among the New World Kalotermitidae, some species prefer a dry habitat (Incisitermes, Marginitermes, Pterotermes, and Tauritermes), but others prefer a humid habitat, like Cryptotermes brevis (Walker), or Incisitermes minor (Hagen) and Marginitermes hubbardi (Banks), capable of living in a dry or humid environment, infesting structural wood [2,3].
Incisitermes marginipennis (Latreille) has a cryptic lifestyle. They make tunnels in the wood in search of food. Extrem wood biodeterioration is internal, silent, and not surface. It is not visible. Due to its destructive power of wood assets and its fundamental ecological role in the biogeochemical cycle of C, O, N, H, P and S. It is necessary to implement a method strict with special pesticides to their control. The less toxic substances for mammals and ecology are of great value to protect any element of wood or cellulose from the attack of termites. Also, to reduce the frequency of maintenance, conservation and care processes [4,5].
A few termites can cause big damage to the low density and durability of drywood from Pinus spp. or Abies spp. Conventional controls of drywood termites use chemicals applied by different methods. In service wood, halogen fumigants such as sulfuryl fluoride, methyl bromide, and methyl bromide/carbon dioxide mixture are pumped into the area. While in stored wood are applied chlorpyrifos, bifenthrin, imidacloprid, endosulfan and lindane [6]. Obtaining new molecules with alternative application methods for pest control is a challenge to overcome. Both its presence and its derivatives must last a short time in the environment without causing toxicity or contamination. Consequently, safe plant products are being tested worldwide as a substitute for pest control agents, e.g., termite-repellent compounds. I. marginipennis is a drywood termite considered a pest that competes for cellulosic products utilized by humans [7,8].
Historically, control of insect pests with plant derivatives was successful. In a time of minimal production of food and other items that the population required. However, its use was abandoned due to the effectiveness of conventional synthetic pesticides used in the mass production of food and other necessary items for the growing population. Now that we know that the cost is greater than the benefit of using it, e.g., environmental contamination and the appearance of resistant pests. There is a renewed interest in today’s society for botanical pesticides to control pests of crops of economic interest. Plant secondary metabolites with a deleterious effect on pest organisms, such as biocides, repellents, anti-feeders, oviposition inhibitors, and confounding factors, have been described [9,10]. However, few of the compounds pure, in mixtures, or crude plant extracts have passed field tests. The control of biodeterioration and biodegradation of wood is no exception. The biomass of certain plants possesses insecticidal activity that can be exploited for controlling termites [9]. We also know the metabolome of a small number of land plants. So, we can discover plants with toxic, repulsive, antifeedant, confounding, or oviposition-inhibiting metabolites. Plants for this purpose are regional, abundant, and low-cost. With the hope of obtaining a formulation based on molecules or components of vegetable origin for the control of biodeterioration and biodegradation of wood [10,11].
Besides, according to several studies, the Tectona grandis L.f. wood is “very durable” to rot caused by fungi and termites (ASTM standards). It has good machining properties, it is a commercial wood due to its excellent workability, durability, aesthetic characteristics and high dimensional stability. The heartwood is characterized by a golden brown colour with an irregular distribution of black veins along the rings. It is a friendly species with acceptable reproduction and rapid development in commercial plantations [12]. The aim of this study was to evaluate the effect of various naphthoquinones obtained from the heartwood of T. grandis, which have repellent and/or insecticidal biological activity on I. marginipennis termites.
2.1 Obtaining Heartwood Powder from Tectona grandis
The wood was obtained from a commercial forestry plantation, located at 19°12′10.4″N 101°52′22.1″W/19.202874, −101.872814. In 14-year-old trees, two consecutive transversal cuts were made at the height of 1.5 m from the base of the trunk to obtain log samples, and then they were unbarked. The sapwood and heartwood were carefully separated from each other. The heartwood was broken into 5 cm × 1 cm splinters with a hammer and chisel. The splinters were pulverized with a mill (MICRON-K2OF). The powdered wood was sieved with TYLER equipment (model RX-29), through 40 mesh (425 microns). The heartwood powder was placed in a desiccator until use.
Raw extract from heartwood T. grandis was obtained with the Soxhlet extraction technique. Heartwood powder sample (400 g) was placed in an extraction thimble holder (Whatman, GE Healthcare) and the maceration/percolation was for 6 h with chloroform as solvent [13]. Then, the chloroform was removed from the raw extract by a rotary evaporator (BUCHI brand). The extract protected from light was stored at 4°C until use. The yield index was obtained through the fresh weight of the wood and its difference with the flour obtained.
2.3 Characterization of Naphthoquinone Compounds from Tectona grandis
Using the powdered wood obtained and following the method by Gupta et al. [14], the extraction of naphthoquinone compounds was carried out. The flour was tested through reflux extraction in Soxhlet equipment for 6 h, using chloroform as a solvent in a ratio of 1:12.5 (weight/volume). Subsequently, 200 mL of a 2 N Na2CO3 solution (in a 1:1 ratio with the chloroform solution) were used to separate the acid and neutral fraction, verifying its nature with the help of a potentiometer device (PH130). The neutral fraction (aqueous) was subjected to a reaction with 6 N HCl (20 mL) to obtain lapachol (C15H14O3). The acid phase was fractionated by column chromatography on Silica Gel G/UV254 (deactivated with 10% acetic acid), obtaining up to 4 fractions (identifying them as A, B, C and D).
Fraction A was subjected to crystallization with methanol (10 mL) to obtain tecomaquinone-I (C30H24O4); Fraction B was separated by preparative Thin Layer Chromatography (TLC) with benzene (250 mL) as mobile phase, to obtain tectoquinone (C15H10O2) and deoxylapachol (C15H14O2). Fraction C was crystallized with chloroform (20 mL) until dehydro-α-lapachone (C15H12O3) was obtained. Fraction D was again separated using TLC with benzene and ethyl acetate (250 mL) as mobile phase, in a 1:1 ratio until obtaining tectol (C30H26O4). Finally, the compounds obtained were stored refrigerated at 4°C (LG Mod. GF22BGSK) in amber containers [14,15]. Subsequently, for the identification of the compounds obtained, they were analyzed by nuclear magnetic resonance (1H (NMR 1H)) in a Varian Mercury plus Spectrometer equipment at 200 MHz, and Gas Chromatography (Agilent Technology model 6890 plus), coupled to a mass spectrometer (Mod 5973N). The information obtained in the equipment was corroborated using the Interactive Spectroscopy software (Advanced Chemistry Development, Inc., Toronto, Canada, 2022).
2.4 Adaptation Conditions to Incisitermes marginipennis
The termite colony (I. marginipennis) was collected from dry wood of Pinus sp. in Morelia, Michoacán, Mexico (19°42′10″N, 101°11′32″W). The taxonomic classification of termites was carried out using the morphological characteristics of the soldier termite and reproductive wings [16]. Subsequently, growth conditions were adjusted for the colony adaptation and reproduction, using a piece of pine wood (dry) (45 cm length × 15 cm width) placed on a filter paper base (Whatman No. 1) inside a plastic container (50 cm× 30 cm× 20 cm) under controlled environmental conditions at a temperature of 28°C and darkness for 90 days. During this period, once a week, the surface of the wood was sprinkled with 20 mL of distilled water. Subsequently, the nymphal termites were recovered from the wood piece used in the following tests [5].
2.5 Concentration-Response Effect with Cypermethrin on the Termite Incisitermes marginipennis
Using a Petri dish system (in triplicate) with 5 cm diameter cellulose discs (Whatman No. 1) and distilled water as a vehicle [17], 100 µL of the solution containing the following concentrations of cypermethrin (GOWAN CIPERMETRINA 200) were added to the center of the disc; zero (Control), 0.015, 0.03, 0.06, 0.12, 0.24, 0.48 mg/mL. Next, 10 nymphal termites of I. marginipennis were carefully placed per Petri dish and transferred to a chamber with dark conditions and a temperature of 28°C. After 12 and 24 h, the weight loss of the cellulose disk and the percentage of mortality for each concentration were quantified.
With the values obtained from the test, the lethal concentration 50 (LC50) was calculated using the linear regression method, 100% survival for the lowest concentrations and 0% survival for the highest concentrations. LC50 was determined through linear regression of the R2 line equation, and from the calculation of the Pearson correlation coefficient [18].
2.6 Insecticide Effect of Naphthoquinones on the Termite Incisitermes marginipennis
Following the methodology described by Ganapaty et al. [19], the extracted and isolated compounds were used to determine the repellent activity on I. marginipennis, using cellulose discs with a diameter of 5 cm (Whatman No. 1) in glass Petri dishes, adding 100 µL of the solution containing each of the extracted compounds to the center of the disk (using ethanol as a vehicle at a concentration of 0.2 mg/mL). Thus, obtaining the following treatments: (1) distilled water (100 µL), (2) chloroform (100 µL), (3) ethanol (100 µL), (4) deoxylapachol*, (5) tecomaquinone-I*, (6) tectoquinone*, (7) Lapachol*, (8) dehydro-α-lapachone*, and (9) tectol*. The assay was tested in triplicate (*indicates the concentration tested in each treatment, 0.2 mg/mL).
For one hour and under laminar flow hood conditions, the Petri dishes of all treatments were kept open at room temperature, allowing the evaporation of the different solvents. Subsequently, 10 nymphal termites of I. marginipennis were placed per plate and transferred to a dark chamber with a controlled temperature of 28°C. Periodically (9, 18, and 27 days), the weight loss of the cellulose disc and mortality percentage for each treatment (1 to 9) were quantified.
2.7 Repellent Effect of Naphthoquinones on the Termite Incisitermes marginipennis
According to the methodology described by Ganapaty et al. [19], the naphthoquinone compounds obtained were tested to evaluate the deterrent effect on I. marginipennis, which consisted of using a Petri dish system; two cellulose (Whatman No. 1) discs of 2.5 cm diameter were placed at opposite poles. For each plate assay, 50 µL of ethanol was added to one disk, and 50 µL of each compound obtained (deoxylapachol, tectoquinone, lapachol, dehydro-α-lapachone, tecomaquinone-I and tectol) was added to the other disk, using ethanol as vehicle at a concentration of 0.2 mg/mL [7,20].
For one hour and under laminar flow conditions, the plates of all the treatments were kept open at room temperature, allowing the evaporation of the different solvents. Subsequently, 10 termite nymphs of I. marginipennis were placed per Petri dish (tested in triplicate) and transferred to a chamber in darkness with controlled temperature (28°C). After 24 h, the percentage of mortality in termites and the number of individuals found in contact with the cellulose disc added with any of the tested naphthoquinone compounds were quantified.
2.8 Wood Protection Effect of Naphthoquinones on the Termite Incisitermes marginipennis
Using wood from Pinus sp., the methodology established for the AWPA test (American Wood Protection Association) [21] was followed with some modifications, where the wood pieces were first conditioned for use: they were cut with dimensions of 25 mm × 25 mm × 25 mm, then they were placed in a drying chamber (70°C) until constant weight (Initial weight). Subsequently, 1 mL of ethanol was added to each piece, which contained each of the naphthoquinones at a concentration of 0.2 mg/mL, thus achieving the following treatments; (1) distilled water (1 mL), (2) chloroform (1 mL), (3) ethanol (1 mL), (4) deoxylapachol*, (5) tecomaquinone-I*, (6) tectoquinone*, (7) lapachol*, (8) dehydro-α-lapachone*, and (9) tectol* (*indicates the concentration tested in each treatment, 0.2 mg/mL). Twenty pieces of wood per treatment were placed in a plastic container with a cylindrical shape (10 cm × 25 cm), then 30 nymphal termites (I. marginipennis) were carefully placed per container and transferred to an incubator under dark conditions at 28°C for 27 days. Subsequently, according to Bakar et al. [22] the weight loss was calculated for each piece, and the mortality percentage of the termites in the treatments (1 to 9) was quantified.
The significance of the experimental data was obtained with the software STATISTICA v10.0 (TIBCO Statistica, Palo Alto, CA) using Two-Way ANOVA and Tukey test (p < 0.05).
3.1 Naphthoquinones Characterization
Through the fractions obtained (A, B, C and D), and with the use of thin-layer chromatography (TLC) it was possible to separate the various naphthoquinones. Fig. 1a shows the spectrum corresponding to lapachol, exposing the displacement of the aliphatic groups found between 1 and 2 ppm which corresponds to double bonds and a methylene group at 2.6 ppm. The keto groups found at 3.3 ppm showed the hydroxyl group, alkenes are observed at 5.2 ppm and between 7.8 and 8.3 ppm is the corresponding aromatic ring. Fig. 1b shows the spectrum of tectoquinone, indicating the displacement of the aliphatic groups found between 0.5 and 2.5 ppm, the alkene groups are observed at 5.1 ppm and between 7.25 and 8.34 ppm, and the aromatic ring is observed. Fig. 1c shows the spectrum of tecomaquinone-I, where the displacement of the aliphatic groups is found between 15 and 36 ppm, alkene groups at 40 ppm, the aromatic ring at 76 ppm and groups belonging to acids at 126 to 138 ppm. In Fig. 1d (deoxylapachol), the displacement of the aliphatic groups is observed, the double bonds and alkynes are between 0.85 and 2.7 (CH, CH2), at 5.2 ppm are the alkenes and at the end of the spectrum (7.28 to 8.28) the aromatic ring. In Fig. 1e, the spectrum corresponding to dehydro-α-lapachone is shown, where the displacement of the aliphatic groups can be observed, the double bonds and alkynes are between 21.8 and 32.1 ppm (CH, CH2), from 76.7 to 77.3 ppm are the alkene groups and from 72 to 84 ppm is the aromatic ring, and between 123.2 to 145.2 ppm the acid groups. Fig. 1f shows the spectrum of tectol where the aliphatic groups are observed, the double bonds were found at 0.3 and 2.3 ppm (CH, CH2), at 2.1 ppm the ketones were observed, at 2.5 ppm the hydroxyl group, at 4.8 and 5.5 ppm the alkenes and at the end of the spectrum at 7.25 to 8.35 ppm the aromatic ring.
Figure 1: Spectra corresponding to lapachol (a), tectoquinone (b), tecomaquinone-I (c), deoxylapachol (d), dehydro-α-lapachone (e), and tectol (f)
3.2 Evaluation of the Toxic Effect of Cypermethrin in Termites
Cypermethrin is widely used in termite pest control and, in this work, it was used as a reference compound and concentration. Under the Petri dish system with cellulose discs, the weight loss of the cellulose material was not statistically significant between treatments (Table 1a). However, the mortality percentages showed a concentration-dependent dose effect, since, at low concentrations (0.015 and 0.03 mg/mL) the termites exposed to the cypermethrin compound did not show a decrease in their population (mortality), however, increasing the concentration (0.06, up to 0.48 mg/mL) also increases the percentage of deaths in individuals (mortality), reaching up to 100% in deaths after 12 h* of exposure to the compound (Table 1b). For its part, the calculation of the lethal concentration 50 showed that 0.196 mg/mL is required to reach 50% of mortality in the population. And based on the value obtained, it was decided to use the concentration of 0.2 mg/mL for the following tests with the naphthoquinones obtained (toxic-repellent effect, direct choice, and preservative effect on wood).
3.3 Toxic-Repellent Effect of Naphthoquinones on Incisitermes marginipennis
Under the Petri dish system with cellulose discs, it was found that using a concentration of 0.2 mg/mL of deoxylapachol, tecomaquinone-I, tectoquinone, lapachol, dehydro-α-lapachone or tectol, the statistical analysis revealed a significant difference on the weight loss in the cellulose discs, compared to the control treatments (water, chloroform, ethanol) (Table 2a), showing the repellent effect exerted on the termites because of the extracted naphthoquinones.
The mortality percentage indicated an increase through the exposure time, the treatments deoxylapachol and tectoquinone were found to reach mortality values of up to 90 percent in just 9 days, and 100 percent after 28 days (Table 2b), showing that the water, chloroform and ethanol treatments, the damage on the cellulose discs by the termite I. marginipennis was evident, the rest of the treatments showed no apparent damage.
3.4 Direct Choice Assay of Naphthoquinones on Incisitermes marginipennis
Table 3 shows the results of termites placed outside the disks, after one hour of exposure the migration of the insects was quantified, finding that the disks impregnated with 0.2 mg/mL of deoxylapachol, tectoquinone, lapachol, dehydro-α-lapachone, tecomaquinone-I or tectol, exhibited a low presence of termites (“In contact” column), with percentages between 3 (tectoquinone) and 27 percent (dehydro-α-lapachone and tectol). Most of the termite population tested were found on the cellulose discs impregnated with ethanol or distributed in the Petri dish (“without contact” column), with values between 57 (lapachol) and 97 percent (tectoquinone). Also, it was observed that the percentage of mortality in termites because of exposure reached high values, 87, 90, and 100 percent in the lapachol, dehydro-α-lapachone and tecomaquinone-I treatments, respectively (“Mortality” column).
3.5 Preservative Effect on Low Durability of Wood
In the Pinus sp. wood pieces, tested and preserved with the various naphthoquinone compounds, a protective effect was found against the damage caused by I. marginipennis, that is, the wood pieces treated with any of the naphthoquinone compounds did not show significant weight loss upon exposure to termites. On the other hand, the following treatments: water, chloroform, and ethanol presented average values of weight loss of 318, 155, and 158 mg, respectively (Table 4a). Also, it was observed that the only treatments where statistically significant mortality was observed (after 28 days of exposure) were deoxylapachol, tecomaquinone-I, lapachol and tectoquinone (100, 100, 90, and 60 percent, respectively) (Table 4b). Fig. 2 shows the wood pieces used for the treatments, where the damage caused (red arrow) by I. marginipennis (water, chloroform, ethanol, and tecomaquinone-I) is observed in some of them.
Figure 2: Damage on the wood pieces treated with naphthoquinones (red arrows)
The pathogens pose serious health challenges to mankind, the importance of naphthoquinones have an interesting biotechnological value, and the ability of different naphthoquinones to inhibit and/or eradicate pathogens has been investigated [23]. di Marco et al. [24], reported that capacity of different naphthoquinones to inhibit Yersinia enterocolitica strains WAP 314/CLC001, inhibition of biofilm formation and cell viability, quorum sensing inhibition, and oxidative stress generation.
The naphthoquinones have achieved a distinct position to defeat microbial pathogens (Enterococcus faecium, Staphylococcus aureus, Klebsiella pneumoniae, Acinetobacter baumannii, Pseudomonas aeruginosa, and Enterobacter spp.). In bacteria, may exhibit their function in the following ways (a) plasmid curing, (b) inhibiting efflux pumps, (c) generating reactive oxygen species, and (d) the inhibition of topoisomerase activity [25].
Deoxylapachol might be an interesting natural product as an antifungal compound. A transgenic Aspergillus niger cell line proved to be a tool for confirming antifungal activity and for learning more about its function [26]. Deoxylapachol has anti-wood rot activity, it can inhibit the brown rot fungi Gloeophyllum sepiarium CBS 353.74 and G. trabeum CBS 318.50 and the white rot fungi Merulius tremellosus CBS 280.73 and Phlebia brevispora CBS 509.92. It also can induce stress in fungal cells [27].
Cypermethrin is a broad-spectrum insecticide with a neurotoxic activity that is widely used, for pest control against termites and other insects, used in agriculture, forestry, and the urban environment, however, despite its proven effectiveness, a replacement is necessary that promises effectiveness with less environmental damage, in addition, that the pests do not acquire a chemical resistance to the compound. Because this insecticide produces alterations in ionic transport (Na+) and extends the duration of the nervous stimulus [28], cypermethrin can affect other non-target organisms, such as fish, amphibians, arthropods, and even birds and mammals [28–32]. For example, some lethal concentration values (LC50) reported in fish (Poecilia reticulata) are 0.0094 mg/mL [33] and 0.00599 mg/mL in Oreochromis niloticus [30], for amphibians (Rana nigromaculata) [29]. In this work, the results of mortality percentage revealed a concentration-dependent lethal effect, between 0.015 and 0.03 mg/mL, the exposed individuals did not register deaths and when increasing the concentration to 0.06, 0.12, 0.24, and 0.48 mg/mL, the percentage of mortality also increased, being 0.48 mg/mL where 100% of the deaths were achieved. The biological model used in this work showed that 0.196 mg/mL is necessary to reach the LC50, exposing that high concentrations are required to exert a toxic effect on the termite, which can have a negative impact on the environment, since a compound with a broad spectrum and perpetuity, can affect other organisms.
Considering the above, in a repellency test using the naphthoquinone compounds obtained, it was found that the termites, after 27 days of exposure, exhibited an interesting effect; however, not all the tested compounds reached high mortality termites (Table 2b), it was also found that the treatment with tectol showed a mortality percentage similar to the control treatment (chloroform). Despite the low percentages of mortality in termites with some treatments, it stands out that all of these exerted a protective effect on the cellulose disc. Regarding the compound that showed the highest performance (42 mg of deoxylapachol), it is among those with the greatest protective effect, which according to some authors, in T. grandis, tectoquinone, lapachol and deoxylapachol are the main responsible for providing pest resistance on all against fungi and insects [34]. Deoxylapachol has been tested on an important diversity of fungal pathogenic organisms, such as Trametes versicolor and Fomitopsis palustris [35], but the effect that the molecule has on drywood termites has not been documented. The antibacterial activity of various antibiotics such as anthracycline, daunomycin, or doxorubicin [36], is associated with the structure of hydroxyquinone. Equivalent active sites are also present for tetracycline and myxopyronine antibiotics.
For its part, the effect that naphthoquinones exert on insects is known, where roots of plants such as Diospyros kaki have insecticidal activity against larvae of Aedes aegypti, Culex pipiens pallens and Ochlerotatus togoi, identifying 5-hydroxy-2-methyl-1,4-naphthoquinone as a bioactive component [37]. Also, menadione (2-methyl-1,4-naphthoquinone) has been documented to exert a toxic effect on the subterranean termite Coptotermes formosanus Shiraki (trial with menadione-treated sand from 6 to 600 ppm), probably due to interference with mitochondrial oxidative phosphorylation, obtaining mortality values ≥90% after seven days and minimal or no food consumption. Showing that menadione affects the behavior related to survival and feeding capacity, also influencing their behavior to generate tunnels in the sand [38].
The cellular target of naphthoquinones (insecticide) is unknown and is a pharmacological challenge, as it may depend on the type of organism or the chemical nature of the molecule (naphthoquinones, anthraquinones, etc.). However, it is known that atovaquone, an analogue of ubiquinone, acts at the cellular level by interfering with the electron transport chain of the mitochondrial system, a phenomenon observed in Plasmodium species, inhibiting the synthesis of nucleic acids and ATP [39]. Because naphthoquinones induce oxidative stress in cells, forming species such as O2 and/or H2O2, the process of cell apoptosis is favoured by an increase in them. For its part, the quinone is reduced to form dianion, this reaction can be catalyzed by several enzymes such as flavones, NADPH, cytochrome P450 reductase, NADPH cytochrome b5 reductase, or NADPH ubiquinone oxidoreductase. Once the reduced species or dianion is formed, it can reduce the molecular oxygen to the superoxide radical, which in the presence of the enzyme superoxide dismutase can be transformed into H2O2 [40]. The superoxide radical, through the Fenton reaction, generates HO• inside the cell, where H2O2 can also promote the oxidation of some biomolecules such as lipids, giving rise to the formation of free radicals from their fatty acids and proteins, modifying their primary structure and functionality, contributing to cell death.
The natural durability of wood is understood as the resistance that some species present against environmental deterioration, and is attributed to the presence of extractive compounds [41], and in this sense, for low-durability wood, a wide variety of organic and inorganic compounds are used in the preservation industry to control termites and other xylophagous organisms, using physical (Barriers toxic and nontoxic, also treatments employing heat, electricity, freezing, or microwave), chemical (copper/chromium/arsenical salts; copper/azoles/organics; coppers/azoles/organics/boron; cypermethrin; pentachlorophenol; arsenic/copper/ammonia; chromium/zinc/chlorine) and biological methods (bacterial toxins, mycotoxins, essentials oils and more) [42,43]. The present work is shown as the only one where naphthoquinone compounds have been tested on low-durability wood, finding that wood preserved with deoxylapachol, tecomaquinone-I, and lapachol showed mortality percentages of ≥90 in drywood termites. There are still doubts to be resolved, but molecular coupling studies will help us understand how the interaction between the structural components of the lignocellulosic material with the tested molecules takes place [44]. It is also important to remark that, in the wood preservation industry, one of the main problems faced by new proposals and substances is their perpetuity and useful life in the material, results showed that some quinones are stable above 215°C, while others are not, e.g., 1,2-naphthoquinone decomposes above 100°C [45], characteristics that increase the possibilities of being used for biological control of termites and other organisms involved in the deterioration of lignocellulosic materials.
The molecules obtained from the heartwood of Tectona grandis showed a repellent-protective effect against Incisitermes marginipennis, although these molecules did not show high percentages of mortality in all the treatments. However, they presented a statistically significant decrease in the weight loss of the treated material.
Acknowledgement: To the National Council of Science and Technology (CONACYT), the Coordination of Scientific Research of our university (UMSNH), and Dr. Ramón Santillán Galván (IMSS).
Funding Statement: This study was supported by the Coordination of Scientific Research, UMSNH (Project 2022).
Author Contributions: Study conception and design: Crisanto Velázquez-Becerra and Rafael Torres-Martínez; data collection and analysis: Gu Yen Ambriz-Ortiz; manuscript preparation: Mauro Manuel Martínez-Pacheco. All authors reviewed the results and approved the final version of the manuscript.
Conflicts of Interest: The authors declare that they have no conflicts of interest to report regarding the present study.
References
1. Evans, T. A. (2021). Predicting ecological impacts of invasive termites. Current Opinion in Insect Science, 46, 88–94. https://doi.org/10.1016/j.cois.2021.03.003 [Google Scholar] [PubMed] [CrossRef]
2. Scheffrahn, R. H. (2019). Expanded New World distributions of genera in the termite family Kalotermitidae. Sociobiology, 66(1), 136–153. https://doi.org/10.13102/sociobiology.v66i1.3492 [Google Scholar] [CrossRef]
3. Scheffrahn, R. H., Crowe, W. (2011). Ship-borne termite (Isoptera) border interceptions in Australia and onboard infestations in Florida, 1986–2009. Florida Entomologist, 94(1), 57–63. https://doi.org/10.1653/024.094.0108 [Google Scholar] [CrossRef]
4. Meusemann, K., Korb, J., Schughart, M., Staubach, F. (2020). No evidence for single-copy immune-gene specific signals of selection in termites. Frontiers in Ecology and Evolution, 8(26), 1–16. https://doi.org/10.3389/fevo.2020.00026 [Google Scholar] [CrossRef]
5. Ramírez-López, C. B., Beltrán-Sánchez, R., Hernández-Izquierdo, A., Salvadorhernández, J. L., Salcedo-Pérez, E. et al. (2021). Antifeedant activity of Caesalpinia coriaria essential oil against Incisitermes marginipennis (Latreille). Phyton-International Journal of Experimental Botany, 90(3), 907–920. https://doi.org/10.32604/phyton.2021.013775 [Google Scholar] [CrossRef]
6. Sindhu, S. S., Rakshiya, Y. S., Verma, M. K. (2011). Biological control of termites by antagonistic soil microorganisms. In: Bioaugmentation, biostimulation and biocontrol, pp. 261–309. Berlin, Heidelberg: Springer. https://doi.org/10.1007/978-3-642-19769-7_12 [Google Scholar] [CrossRef]
7. Raya-González, D., Ron-Echeverría, O. A., Flores-García, A., Macias-Rodríguez, L. I., Martínez-Pacheco, M. M. (2013). Dissuasive effect of an aqueous extract from Enterolobium cyclocarpum (Jacq) Griseb on the drywood termite Incisitermes marginipennis (Isoptera: Kalotermitidae) (Latreille). Emirates Journal of Food and Agriculture, 25(7), 524–530. https://doi.org/10.9755/ejfa.v25i7.15987 [Google Scholar] [CrossRef]
8. Ramírez-López, C. B., García-Sánchez, E., Martínez-Muñoz, R. E. M. M., Del Río, R. E., Martínez-Pacheco, M. M. (2016). Chemical composition of the essential oil from Ageratina jocotepecana and its repellent effect on Drywood termite Incisitermes marginipennis. Boletín Latinoamericano y del Caribe de Plantas Medicinales y Aromáticas, 15(1), 53–60. [Google Scholar]
9. Kaur, P. J., Satya, S., Pant, K. K., Naik, S. N. (2016). Eco-friendly preservation of bamboo species: Traditional to modern techniques. BioResources, 11(4), 10604–10624. [Google Scholar]
10. Mishra, P., Tripathi, A., Dikshit, A., Pandey, A. (2020). Insecticides derived from natural products: Diversity and potential applications. In: Natural bioactive products in sustainable agriculture, pp. 83–99. Singapore: Springer. https://doi.org/10.1007/978-981-15-3024-1_6 [Google Scholar] [CrossRef]
11. Mantzoukas, S., Eliopoulos, P. A. (2020). Endophytic entomopathogenic fungi: A valuable biological control tool against plant pests. Applied Sciences, 10(1), 360. https://doi.org/10.3390/app10010360 [Google Scholar] [CrossRef]
12. Moya, R., Bond, B., Quesada, H. (2014). A review of heartwood properties of Tectona grandis trees from fast-growth plantations. Wood Science and Technology, 48(2), 411–433. https://doi.org/10.1007/s00226-014-0618-3 [Google Scholar] [CrossRef]
13. Lourenço, A., Neiva, D. M., Gominho, J., Marques, A. V., Pereira, H. (2015). Characterization of lignin in heartwood, sapwood and bark from Tectona grandis using Py–GC–MS/FID. Wood Science and Technology, 49(1), 159–175. https://doi.org/10.1007/s00226-014-0684-6 [Google Scholar] [CrossRef]
14. Gupta, P. K., Singh, P. (2004). Note: A naphthoquinone derivative from Tectona grandis (LINN.). Journal of Asian Natural Products Research, 6(3), 237–240. https://doi.org/10.1080/10286020310001653192 [Google Scholar] [PubMed] [CrossRef]
15. Vyas, P., Wadhwani, B. D., Khandelwal, P., Araya, H., Fujimoto, Y. (2022). Tectonaquinones A, B and C: Three new naphthoquinone derivatives from the heartwood of Tectona grandis. Natural Product Research, 36(7), 1707–1715. https://doi.org/10.1080/14786419.2020.1810035 [Google Scholar] [PubMed] [CrossRef]
16. Rust, M. K., Su, N. Y. (2012). Managing social insects of urban importance. Annual Review of Entomology, 57(1), 355–375. https://doi.org/10.1146/annurev-ento-120710-100634 [Google Scholar] [PubMed] [CrossRef]
17. Davis, H. E., Meconcelli, S., Radek, R., McMahon, D. P. (2018). Termites shape their collective behavioural response based on stage of infection. Scientific Reports, 8(1), 1–10. https://doi.org/10.1038/s41598-018-32721-7 [Google Scholar] [PubMed] [CrossRef]
18. Silva, M. S., Broglio, S. M. F., Trindade, R. C. P., Ferrreira, E. S., Gomes, I. B. et al. (2015). Toxicity and application of neem in fall armyworm. Comunicata Scientiae, 6(3), 359–364. https://doi.org/10.14295/cs.v6i3.808 [Google Scholar] [CrossRef]
19. Ganapaty, S., Thomas, P. S., Fotso, S., Laatsch, H. (2004). Antitermitic quinones from Diospyros sylvatica. Phytochemistry, 65(9), 1265–1271. https://doi.org/10.1016/j.phytochem.2004.03.011 [Google Scholar] [PubMed] [CrossRef]
20. Manzoor, F., Pervez, M., Adeyemi, M. M. H., Malik, S. A. (2011). Effects of three plant extracts on the repellency, toxicity and tunneling of subterranean termite Heterotermes Indicola (Wasmann). Journal of Applied Environmental and Biological Sciences, 1(7), 107–114. [Google Scholar]
21. Sarker, P. K. (2006). Standard test methods for wood preservatives by laboratory agar-block and soil-block test, pp. 37–56. Bangladesh: Khulna University. [Google Scholar]
22. Bakar, E. S., Hao, J., Ashaari, Z., Yong, A. C. C. (2013). Durability of phenolic-resin-treated oil palm wood against subterranean termites a white-rot fungus. International Biodeterioration and Biodegradation, 85, 126–130. https://doi.org/10.1016/j.ibiod.2013.04.019 [Google Scholar] [CrossRef]
23. Hussain, H., Krohn, K., Ahmad, V. U., Miana, G. A., Green, I. R. (2007). Lapachol: An overview. Arkivoc, 2(1), 145–171. [Google Scholar]
24. di Marco, N. I., Páez, P. L., Lucero-Estrada, C. S., Pungitore, C. R. (2021). Naphthoquinones inhibit formation and viability of Yersinia enterocolitica biofilm. World Journal of Microbiology and Biotechnology, 37(2), 1–13. [Google Scholar]
25. Mone, N. S., Bhagwat, S. A., Sharma, D., Chaskar, M., Patil, R. H. et al. (2021). Naphthoquinones and their derivatives: Emerging trends in combating microbial pathogens. Coatings, 11(4), 434. [Google Scholar]
26. Sumthong, P., Damveld, R. A., Choi, Y. H., Arentshorst, M., Ram, A. F. et al. (2006). Activity of quinones from teak (Tectona grandis) on fungal cell wall stress. Planta Medica, 72(10), 943–944. https://doi.org/10.1055/s-2006-946676 [Google Scholar] [PubMed] [CrossRef]
27. Sumthong, P., Romero-González, R. R., Verpoorte, R. (2008). Identification of anti-wood rot compounds in teak (Tectona grandis Lf) sawdust extract. Journal of Wood Chemistry and Technology, 28(4), 247–260. https://doi.org/10.1080/02773810802452592 [Google Scholar] [CrossRef]
28. Yilmaz, S. N., Çömelekoğlu, Ü., Coşkun, B., Balli, E., Özge, A. (2008). Effects of cypermethrin on isolated frog sciatic nerve: An ultrastructural study. Turkish Journal of Medical Sciences, 38(2), 121–125. https://journals.tubitak.gov.tr/medical/vol38/iss2/5 [Google Scholar]
29. Xu, P., Huang, L. (2017). Effects of α-cypermethrin enantiomers on the growth, biochemical parameters and bioaccumulation in Rana nigromaculata tadpoles of the anuran amphibians. Ecotoxicology and Environmental Safety, 139, 431–438. https://doi.org/10.1016/j.ecoenv.2017.02.015 [Google Scholar] [PubMed] [CrossRef]
30. Sarikaya, R. (2009). Investigation of acute toxicity of alpha-cypermethrin on adult Nile tilapia (Oreochromis niloticus L.). Turkish Journal of Fisheries and Aquatic Sciences, 9(1), 85–89. [Google Scholar]
31. Agostini, M. G., Roesler, I., Bonetto, C., Ronco, A. E., Bilenca, D. (2020). Pesticides in the real world: The consequences of GMO-based intensive agriculture on native amphibians. Biological Conservation, 241(5), 108355. https://doi.org/10.1016/j.biocon.2019.108355 [Google Scholar] [CrossRef]
32. Biga, L. M., Blaustein, A. R. (2013). Variations in lethal and sublethal effects of cypermethrin among aquatic stages and species of anuran amphibians. Environmental Toxicology and Chemistry, 32(12), 2855–2860. https://doi.org/10.1002/etc.2379 [Google Scholar] [PubMed] [CrossRef]
33. Yılmaz, M., Gül, A., Erbaşlı, K. (2004). Acute toxicity of alpha-cypermethrin to guppy (Poecilia reticulata, Pallas, 1859). Chemosphere, 56(4), 381–385. https://doi.org/10.1016/j.chemosphere.2004.02.034 [Google Scholar] [PubMed] [CrossRef]
34. Anoop, K., Varun, T. K., Ajeesh, A. K., Aravind, A., Praveen, P. et al. (2020). Screening of potential inhibitors for COVID-19 main protease from phytoconstituents of Tectona grandis Linn: Application of molecular modeling studies. Research Square, 1–28. https://doi.org/10.21203/rs.3.rs-21617/v1 [Google Scholar] [CrossRef]
35. Ismayati, M., Zulfiana, D., Tarmadi, D., Lestari, A. S., Krishanti, N. P. R. A. et al. (2019). Biological control of wood destroying organism using plant extracts collected from Mt. Merapi National Park, Indonesia. Biosaintifika, 11(3), 360–368. https://doi.org/10.15294/biosaintifika.v11i3.20102 [Google Scholar] [CrossRef]
36. Whitener, R., Mosley, R. J., Wower, J., Byrne, M. E. (2021). Nucleic acid biohybrid nanocarriers with high-therapeutic payload and controllable extended release of daunomycin for cancer therapy. Journal of Biomedical Materials Research Part A, 109(7), 1256–1265. https://doi.org/10.1002/jbm.a.37119 [Google Scholar] [PubMed] [CrossRef]
37. Kim, M. G., Lee, H. S. (2016). Insecticidal toxicities of naphthoquinone and its structural derivatives. Applied Biological Chemistry, 59(1), 3–8. https://doi.org/10.1007/s13765-015-0115-x [Google Scholar] [CrossRef]
38. Ngo, K., Castillo, P., Laine, R. A., Sun, Q. (2021). Effects of menadione on survival, feeding, and tunneling activity of the formosan subterranean termite. Insects, 12(12), 1109. https://doi.org/10.3390/insects12121109 [Google Scholar] [PubMed] [CrossRef]
39. Egwu, C. O., Tsamesidis, I., Pério, P., Augereau, J. M., Benoit-Vical, F. et al. (2021). Superoxide: A major role in the mechanism of action of essential antimalarial drugs. Free Radical Biology and Medicine, 167(13), 271–275. https://doi.org/10.1016/j.freeradbiomed.2021.03.001 [Google Scholar] [PubMed] [CrossRef]
40. Bolton, J. L., Dunlap, T. (2017). Formation and biological targets of quinones: Cytotoxic versus cytoprotective effects. Chemical Research in Toxicology, 30(1), 13–37. https://doi.org/10.1021/acs.chemrestox.6b00256 [Google Scholar] [PubMed] [CrossRef]
41. Perrot, T., Salzet, G., Amusant, N., Beauchene, J., Gerardin, P. et al. (2020). A reverse chemical ecology approach to explore wood natural durability. Microbial Biotechnology, 13(5), 1673–1677. https://doi.org/10.1111/1751-7915.13540 [Google Scholar] [PubMed] [CrossRef]
42. Mishra, P., Verma, M., Jha, S., Tripathi, A., Pandey, A. et al. (2021). Biological approaches of termite management: A review. Current Botany, 12, 121–131. [Google Scholar]
43. Carcamo-Ibarra, E., Martinez-Pacheco, M., Munro-Rojas, A., Ambriz-Parra, J., Velazquez-Becerra, C. (2022). Antifungal activity of crude extracts of Tectona grandis Lf against wood decay fungi. Phyton-International Journal of Experimental Botany, 91(8), 1795–1808. https://doi.org/10.32604/phyton.2022.020578 [Google Scholar] [CrossRef]
44. Fan, J., Fu, A., Zhang, L. (2019). Progress in molecular docking. Quantitative Biology, 7(2), 83–89. https://doi.org/10.1007/s40484-019-0172-y [Google Scholar] [CrossRef]
45. Sousa, E. T., da Silva, M. M., de Andrade, S. J., Cardoso, M. P., Silva, L. A. et al. (2012). Evaluation of thermal stability of quinones by thermal analysis techniques. Thermochimica Acta, 529, 1–5. https://doi.org/10.1016/j.tca.2011.11.012 [Google Scholar] [CrossRef]
Cite This Article
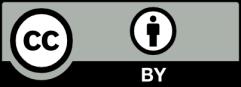
This work is licensed under a Creative Commons Attribution 4.0 International License , which permits unrestricted use, distribution, and reproduction in any medium, provided the original work is properly cited.