Open Access
ARTICLE
Micropropagation of Daylily (Hemerocallis fulva) from Crown-Tip Explants and Assessment of Somaclonal Variation of in Vitro-Propagated Plants Using SCoT Markers
1
Genetics Department, Faculty of Agriculture, Zagazig University, Zagazig, 44511, Egypt
2
Department of Agricultural Biotechnology, College of Agriculture and Food Sciences, King Faisal University, Al-Ahsa, 31982,
Saudi Arabia
3
Department of Biology, College of Science, University of Jeddah, Jeddah, 21959, Saudi Arabia
4
Department of Biology, College of Science, Princess Nourah bint Abdulrahman University, Riyadh, 11671, Saudi Arabia
5
Department of Plant Production, (Genetic Branch), Faculty of Environmental and Agricultural Sciences, Arish University, El-Arish,
45511, Egypt
* Corresponding Authors: Jameel M. Al-Khayri. Email: ; Abdallah A. Hassanin. Email:
Phyton-International Journal of Experimental Botany 2023, 92(7), 2183-2196. https://doi.org/10.32604/phyton.2023.028537
Received 23 December 2022; Accepted 06 March 2023; Issue published 29 May 2023
Abstract
Determination of the somaclonal variation of in vitro-propagated plants is crucial to determine the appropriate micropropagation protocol and growth regulators for commercial scale multiplication. In this research, nine multiplication media (MM) augmented with different concentrations of 6-benzyl adenine (BA), Kinetin (Kin), and Thidiazuron (TDZ), Three rooting media (RM) supplemented with three levels of α-naphthalene acetic acid (NAA) and three types of soil mixtures (v/v); Coco peat/Vermiculite/Sand (CVS), Peat moss/Perlite/Sand (PPS) and Peat moss/Perlite (PP) were used in the micropropagation protocol of daylily plants. MM2 showed the maximum shoot length and the number of leaves, while MM9 showed the maximum number of shoots. The RM1 showed the maximum root length and the number of roots. During acclimatization, CVS, PPS, and PP soil mixture showed similar performance except the CVS mixture showed lower performance regarding plant height and diameter. The genetic fidelity of micropropagated plants was evaluated using Start Codon Targeted (SCoT) Markers. Six SCoT primers amplified 51 scorable bands with an approximate range from 146 bp to 1598 bp size. Thirty one out of 51 loci were presented in the mother plants. 40 loci were polymorphic, 11 were monomorphic and 7 were unique. The amplification patterns of the micropropagated plants demonstrated genetic integrity to the mother plant ranging from 84.32 to 47.06 and somaclonal variations ranging from 52.94 with 5 mg/l BA pathway to 15.68 with 1mg/l TDZ pathway, thus demonstrating that the homogeneity and the variation of the micropropagated plants affected by the type and the quantity of the plant growth regulator used during multiplication subcultures. This research can be successfully used for other ornamental and medicinal plants’ bulk multiplication, germplasm conservation, and future genetic improvement.Keywords
Daylily (Hemerocallis fulva) is a profitable plant, which is increasingly popular, mainly due to its uses in the landscape [1], beautiful flowers [2], and medicinal and pharmaceutical uses [3,4]. Like many other economically valuable crops, the daylily is investigated for reliable in vitro mass production. The utilization of in vitro micropropagation techniques is critical in the case of daylilies due to the plant’s most economically valuable feature, wherefore, this requires accurate multiplication of the parental phenotype, which frequently affects customer choice. In vitro propagation is thus one of the best ways to consistently meet customers’ expectations on a large scale and promptly. Despite significant advances in tissue micropropagation procedures [2], Daylilies present a significant challenge for micropropagation, particularly for plant regeneration. Unfortunately, there is a false perception that daylily is a simple model for in vitro plant organogenesis. Various types of explants including shoot tips, fragments of flower buds, and other parts of the inflorescence were used for in vitro propagation of daylily [5]. Several studies reported that the use of flower petals or scapes as explants has been basically the only method for successful in vitro regeneration of daylilies [6–8]. The use of TDZ in combination with BAP, 6-furfurylaminopurine (kinetin), and 2iP in the medium significantly accelerated shoot regeneration and increased the multiplication efficiency [9]. Occasionally reported techniques that are usually unreliable or challenging to reproduce are frequently used to support this idea. As a result, attempts to enhance the crop using recent techniques like genetic modification and/or CRISPR/Cas9 editing [10–13] have been hampered. The daylily plant naturally makes a few extra divisions during each growing season. At such a low reproduction rate, it can take up to 20 years for an excellent cultivar to transition to the mass market [14]. It is encouraging to see efforts made to enhance traditional in vivo methods for better daylily propagation [15,16], and the use of cytokinin and/or auxin has proven to be the most effective strategy [15,17]. The current investigation for micropropagation of daylily applied a direct pathway protocol for daylily micropropagation without passing the callus induction pathway. The improvement of the daylily plant will be substantially accelerated by the use of in vitro methods for entire plant regeneration, somaclonal diversity produced from tissue culture, and the insertion of novel traits via gene transfer. The assessment of genetic fidelity is crucial to investigate the genetic variation of micropropagated plants compared to the mother plant, Plant growth regulators, explant type, and different subcultures during micropropagation could all contribute to the somaclonal variation which negatively affects the uniformity of regenerated plants [18,19]. An effective approach to evaluate the genetic variations is the SCoT molecular marker that enables screening of the total plant genome [20–22]. SCoT marker is a dominant and reproducible marker that is based on the short conserved sequence in plant genes flanking the ATG start codon using a single 18-mer primer in the PCR assay and high annealing temperature [23]. Furthermore, genetic homogeneity research needs to be conducted on micropropagated plants, primarily in areas with higher industrial applicability. In the current work, daylily micropropagation responses to various types and concentrations of plant growth regulators were investigated. This study aimed to generate a simple, cost-effective, and highly effective daylily micropropagation procedure using the crown-tip explants for the large-scale propagation of daylily and to assess the genetic fidelity of the micropropagated plants using SCoT-PCR-based markers.
2.1 Explant Preparation and Sterilization
The daylily (Hemerocallis fulva) mother plant was obtained from the Egyptian German Company for agricultural production. The crown-tip explants (1–2 cm length) were used for daylily in vitro micropropagation as it takes the direct organogenesis pathway. The prepared crown-tip explants were washed for one hour under running water, surface sterilized for 30 min with 60% commercial Clorox solution (5.25% NaOC1), and then washed three times with sterile distilled water. Under sterile circumstances, the explants (one explants/treatment) were cultured individually in a screwed jar containing 50 ml of Murashig and Skoog (MS) multiplication media [24].
2.2 Media Preparation and Culture Conditions
Daylily plants were in vitro propagated using MS media with vitamins containing 30 g/l sucrose, 7 g/l agar, and various combinations of plant growth regulators (PGRs). The current study evaluated two types of nutrient media (multiplication media and rooting media). The multiplication media included nine different media compositions, i.e., three levels of BA (1, 3, and 5 mg/l), three levels of TDZ (1, 3, and 5 mg/l), and three levels of Kin (1, 3, and 5 mg/l) (Table 1). The individual shoots were transferred from multiplication media to rooting media augmented with different levels of NAA for root formation. The rooting media included three levels of NAA (0.5, 1, and 1.5 mg/l) (Table 1) supplemented with activated charcoal (AC). The pH of the medium was adjusted to 5.7 ± 0.1 and then the medium was autoclaved at 121°C for 20 min. Cultures were shifted to fresh media every three weeks for four subcultures during the multiplication stage and one subculture during the rooting stage after one month. All treatments were incubated at photoperiod (8 h darkness/16 h light) using a light intensity of 3000 lux at 27°C. The crown tip explants of the daylily mother plant were first prepared and cultured on MS medium without growth regulators as control and MS media augmented with BA, Kin, and TDZ. Three levels (1, 3, and 5 mg/l) of each hormone were used to compare the various concentrations on the multiplication performance, somaclonal variation, and genetic fidelity of regenerated daylily plants. The well-developed shoots were transferred to three different MS rooting media supplemented with NAA (0.5, 1, and 1.5 mg/l). The plantlets were moved to small plastic pots in the hardening stage.
All individual plantlets were moved from the rooting medium, washed using tap water to remove the adherent media, and then cultured in pots containing one of three distinct soil mixtures; Coco peat/Vermiculite/Sand (1:1:1), Peat moss/Perlite/Sand (1:1:1) and Peat moss/Perlite (1:1). The pots were covered with plastic bags to maintain the relative humidity. Half-strength MS solution was used to water the plants every alternate day for three weeks, followed by adapting the plants in the greenhouse.
2.4 Data Observations and Collections
During the multiplication stage, the shoot length, the number of leaves, and the number of shoots were measured at the end of each subculture. At the end of the fourth week of the rooting stage, the survival rate (%), root number, and root length were recorded. In the same context, after two months of hardening, the plant height, the number of leaves, and plant diameter were recorded.
2.5 DNA Extraction and PCR (SCoT) Amplification
The young daylily leaves were used to extract the genomic DNA from nine regenerated plants of daylily in addition to the mother plant using the CTAB method with some modifications [25]. The quantity and quality of the purified DNA were evaluated by NanoDrop 2000 (Thermo Scientific™, Waltham, MA, USA). A reaction mixture of 25 μl containing 25 ng template DNA, 0.2 μM dNTPs, 1.5 µM of each primer, 1.5 mM MgCl2, 2 μL 5 × buffer, and 1 unit of Taq polymerase (Promega) was used to conduct the SCoT-PCR-based reactions. On a thermal cycler (applied biosystems), PCR reaction was conducted using the following program: 94°C for 5 min for the initial denaturation followed by 30 cycles at 94°C for 1 min, 1 min at 50°C for primer annealing (Table 2), and 2 min for elongation at SCoT-PCR-based banding profiles were visualized by 1% agarose gel including 1 µg/mL ethidium bromide in 0.5 × TBE buffer (50 V, 90 min). The final step involved using the gel documentation system to take pictures of the PCR products resulting in the presence of 100 bp and 1 kbp DNA ladders as a molecular size reference (WVP, USA).
In all experiments, the data were presented as mean ± standard error. IBM SPSS software was used to evaluate the results statistically [26], Moreover, the Fisher LSD test was used to evaluate the mean differences at a probability level of p ≤ 0.05. For SCoT analysis, the generated bands were scored by the gel documentation program as (1) if present and (0) if absent in the gel. The identical bands of molecular weight were regarded as one locus. The number of bands, polymorphic bands, and the average number of bands per primer was recorded. To detect the genetic fidelity, the banding profiles of regenerated plants were compared with the mother plant profile, and the variation rate (%) was calculated by dividing the number of varied loci by the total scored loci.
The various multiplication media showed distinct variations in the multiplication ability of the daylily explants. The explants that exhibited the highest shoot length were those placed on MS medium augmented with 3 mg/l (BA) followed by 5 mg/l (Kin) and 3 mg/l (TDZ) (Figs. 1A and 1B). The highest number of leaves (5.6) was observed on MS medium augmented with BA (3 mg/l) followed by Kin (3 mg/l) (Figs. 1A and 1C). The highest shoot number (4.5) was recorded on MS medium augmented with TDZ (5 mg/l) and TDZ (3 mg/l) (Figs. 1A and 1D).
Figure 1: (A) Preparation and multiplication stages of daylily. (B–D) Effects of various BA concentrations, Kin and TDZ growth regulators on shoot length, number of leaves and number of shoots, respectively
Note: The presented data are the average mean ± SE of ten plants. Charts with the same letters are not statistically different at p > 0.05.
The well-developed shoots were transferred to three different MS rooting. After two weeks of culture on rooting media, the cultured shoots triggered root development. The number of roots and the root length varied significantly among the three levels of NAA. The number of roots/shoot (5.56) and the root length (7.3) were recorded on RM1 (0.5 mg/l NAA) followed by RM2 (1.0 mg/l NAA) and (1.5 mg/l NAA) (Figs. 2A and 2B).
Figure 2: (A) Rooting stage of daylily. (B) Effects of different concentrations of NAA growth regulator on the number of roots and root length
Note: The presented data are the average mean ± SE of ten plant charts with the same letters that are not statistically different at p > 0.05.
The well-rooted plantlets were moved to small plastic pots and covered with a polyethylene cover with perforations for acclimation for two months at a greenhouse (Fig. 3A). They were then moved to pots with three different soil mixtures; Coco peat/Vermiculite/Sand (CVS), Peat moss/Perlite/Sand (PPS), and Peat moss/Perlite (PP). The survival rate of the hardened plants was 93%, 92%, and 90% in CVS, PPS, and PP, respectively. The hardening efficiency varied significantly among the three types of soils regarding the plant height, the number of leaves, and the plant diameter. There were no significant differences between PPS and PP soils in all recorded parameters. A significant difference was observed among CVS and each of the PPS and PP soils, while no significant differences were recorded among them regarding the number of leaves (Fig. 3B).
Figure 3: (A) Hardening stage of daylily. (B) Effects of three different soil compositions on plant height, number of leaves and plant diameter of acclimatized daylily plants
Note: The presented data are the average mean ± SE of ten plants; charts with the same letters are not statistically different at p > 0.05.
3.4 Genetic Fidelity Assessment
SCoT-PCR-based molecular marker was used to assess the genetic fidelity of the micropropagated plants compared with the mother plant. The six SCoT primers amplified a total of 51 scorable bands with the approximate range from 146 to 1598 bp size (Table 3; Fig. 4) with an average of 8.5 amplified loci per each SCoT primer. Thirty-one out of the 51 loci were present in the mother plants. There were 40 polymorphic loci, 11 monomorphic loci, and seven unique loci (Table 3). SCoT2 and SCoT3 primers showed the highest polymorphism (100%), while SCoT5 showed the lowest polymorphism (62.5%), with an average of 77.63% per primer. Somaclonal variations resulting in regenerated plants were calculated based on the banding profiles compared to the mother plant. Maximum somaclonal variation (52.94%) occurred in plant one resulting from the 5 mg/l BA pathway, while the minimum somaclonal variation (15.68%) occurred in plant 9 resulting from the 3 mg/l BA pathway (Fig. 5). Conversely, Maximum genetic fidelity (84.32%) occurred in plant 9, resulting from the 3 mg/l BA pathway, while the minimum genetic fidelity (47.06%) occurred in plant 1, resulting from the 5 mg/l BA pathway (Fig. 5).
Figure 4: SCoT markers-based genetic fidelity analysis of Hemerocallis fulva in vitro-propagated plants amplified with six primers. Lane M: 100 bp marker with all primers except SCoT 6. The marker was 100 bp plus, Lanes 2–10: PCR amplification of in vitro-regenerated plants (plant 1–plant 9) using different growth regulators during the multiplication stage (5 mg/l BA, 5 mg/l TDZ, 3 mg/l Kin, 3 mg/l TDZ, 1 mg/l TDZ, 1 mg/l BA, 5 mg/l Kin, 1 mg/l Kin, and 3 mg/l BA, respectively). Lane 11: DNA PCR amplification of mother plant
Figure 5: Somaclonal variation percentage of plant 1–plant 9 with the mother plant propagated using 5 mg/l BA, 5 mg/l TDZ, 3 mg/l Kin, 3 mg/l TDZ, 1 mg/l TDZ, 1 mg/l BA, 5 mg/l Kin, 1 mg/l Kin, and 3 mg/l BA, respectively during multiplication stage
The current investigation has successfully discussed the somaclonal variation and genetic fidelity of regenerated plants compared to the mother plant, in addition to the potential use of crown-tip as a successful explant on different MS media compositions for commercial micropropagation of daylily plants. The determination of the ideal concentration of the growth regulator is the main reason for the success of the multiplication and rooting stages. During the multiplication stage, BA (3 mg/l) was the best cytokinin concentration producing the highest shoot length and the number of leaves, while the TDZ (3 mg/l) promoted the number of shoots. These results indicated that moderate concentrations of cytokinins were the best choice to micropropagate daylily. Hence, the TDZ (3 mg/l) was the most favorable cytokinin type and concentration for daylily multiplication. Previous tissue culture studies using TDZ have frequently produced responses across plant species (Neolamarkia Cadamba, Portulaca pilosa L.) that are quite successful and exceed those using various other growth regulators [27–30]. One of the most effective cytokinin-like agents for encouraging cell division in tissue culture is TDZ [31,32], in addition to being more effective than BA, resulting in a greater number of multiple shoots per plant [33]. Generally, TDZ is used at lower concentrations in plant tissue culture [34], the low concentrations have reported to be effective in shoot organogenesis [35]. However, in this study, the 5 mg/L has proven to be the best concentration to produce the highest number of shoots. Therefore, more studies are encouraged to determine the related threshold concentration. The importance of cytokinin is not limited to daylily micropropagation as its application has significantly promoted in vitro multiplication [36,37]. In the rooting stage, the number of roots and root length were better at the low NAA concentration (0.5 mg/l) than at the higher NAA concentrations indicating that daylily tissues may contain a high quantity of auxins. Kannan et al. [38] used a low concentration of IBA (0.5 mg/l) and obtained comparable results in Solanum nigrum. The fine structure, stability, and ease of transfer qualify the IBA for the high frequency of in vitro rooting [39]. CVS soil was the best mixture regarding plant height, the number of leaves, and plant diameter, in addition to achieving the best survival rate (93%).
For the established micropropagation methodology to be used on a commercial scale, genetic fidelity analysis of the micropropagated plantlets must be verified to determine the appropriate conservation programs. Screening of genetic homogeneity among micropropagated plants is preferable to reconfirm genetic variations [40]. The SCoT-PCR-based molecular marker is a simple, quick, trustworthy, and efficient approach for determining the genetic integrity of in vitro regenerated plantlets [41,42]. The genetic fidelity of the regenerated plantlets was evaluated after two months of hardening. The results of molecular profiling proved that the plants produced by 5 mg/l BA during the multiplication stage showed the highest somaclonal variation (52.94%) and the lowest genetic fidelity (47.06%); this is unfavorable in the case of producing identical plants but maybe favorable in the micropropagation of daylily as an ornamental plant to expand the variation base. The plants produced by 3 mg/l BA during the multiplication stage showed the lowest somaclonal variation (15.68%) and the highest genetic fidelity (84.32%). These results concluded that the 3 mg/l BA was not only the best treatment that produced the highest shoot length and the highest number of leaves but also showed the lowest somaclonal variation and the highest genetic fidelity. The SCoT molecular marker was used to conduct similar genetic fidelity studies on in vitro regenerants Pisum sativum [43], Helicteres isora [44], and Cicer arietinum [45]. Also, the fidelity among regenerated plants using ISSR-PCR-based was reported in Eleusine coracana [46] and Morus spp [47]. Consistency, several studies assessed the genetic fidelity in in vitro regenerants was reported in other plant species as well, such as Prunus dulcis [48], Phoenix dactylifera [49], Jatropha curcas [50], Brassica oleracea [51], and Rhodiola imbricate [52].
According to the current study’s findings, the mother plant and the micropropagated plants had slightly different genetic make-ups. The variation introduced during clonal propagation varied depending on the kind and quantity of growth regulators utilized during the multiplication stage.
The current study may support the genetic improvement of daylily plants as ornamental and medicinal plants by producing new somaclonal variants through a tissue culture approach. In the same context, genetic improvement may be conducted through traditional breeding [53], mutation breeding [54], using genetic engineering [55], genome editing [10,11], molecular markers and phylogeny [56,57], and in silico analysis [58].
In vitro propagation is the most effective approach for daylily regeneration. Among the nine multiplication media used, the MM2 showed the maximum shoot length and the number of leaves, and the MM9 showed the maximum number of shoots. The RM1 showed the maximum root length and number of roots among the three rooting media used. During acclimatization CVS, PPS, and PP soil mixture showed similar performance with one exception; the CVS mixture showed lower performance regarding plant height and diameter. Variations in growth regulator type and concentration are responsible for variation in regeneration frequencies. The results revealed by SCoT markers showed a range of somaclonal variations among the micropropagated plants ranging from 15.68% to 52.94% and genetic fidelity ranging from 47.06% to 84.32%. This may shed light on the importance of determining the type and the quantity of the growth regulator used, during multiplication subcultures and avoid using high concentrations of BA to avoid the high rate of somaclonal variation.
Acknowledgement: The authors extend their appreciation for the Deanship of Scientific Research, Vice Presidency for Graduate Studies and Scientific Research, King Faisal University, Saudi Arabia [Project No. GRANT3039] and the Princess Nourah bint Abdulrahman University Researchers Supporting Project Number (PNURSP2023R318), Princess Nourah bint Abdulrahman University, Riyadh, Saudi Arabia for funding this research project. Also, the authors extend their appreciation forZagazig University, Egypt for providing facilities to conduct this research.
Funding Statement: This work was supported by the Deanship of Scientific Research, Vice Presidency for Graduate Studies and Scientific Research, King Faisal University, Saudi Arabia [Project No. GRANT3039] and the Princess Nourah bint Abdulrahman University Researchers Supporting Project Number (PNURSP2023R318), Princess Nourah bint Abdulrahman University, Riyadh, Saudi Arabia.
Author Contributions: Conceptualization, S.S.S., A.A.M. and A.A.H.; methodology, E.E.S., S.S.S., A.A.M. and A.A.H.; software, S.M.A., F.A.S., D.A.E and A.S.J.; validation, S.S.S., A.A.H.; formal analysis, S.M.A., F.A.S., D.A.E and A.S.J.; investigation, E.E.S., S.S.S., A.A.M. and A.A.H.; resources, E.E.S., S.S.S., A.A.M. and A.A.H.; data curation, E.E.S., S.S.S., A.A.M. and A.A.H.; writing original draft preparation, E.E.S., S.S.S., J.M.A.E, and A.A.H.; writing review and editing, S.S.S., J.M.A.E, and A.A.H.; supervision, S.S.S., A.A.M. and A.A.H.; funding acquisition, J.M.A.E., F.A.S., A.S.J.
Conflicts of Interest: The authors declare that they have no conflicts of interest to report regarding the present study.
References
1. Blythe, E. K., Pounders, C., Anderson, M., Watts, E., Watts, B. (2015). Survey of 575 daylily cultivars for severity of daylily rust in a Southern Mississippi landscape. HortTechnology, 25(4), 551–564. [Google Scholar]
2. Matand, K., Shoemake, M., Li, C. (2020). High frequency in vitro regeneration of adventitious shoots in daylilies (Hemerocallis sp.) stem tissue using thidiazuron. BMC Plant Biology, 20(1), 1–10. [Google Scholar]
3. Du, B., Tang, X., Liu, F., Zhang, C., Zhao, G. et al. (2014). Antidepressant-like effects of the hydroalcoholic extracts of Hemerocallis Citrina and its potential active components. BMC Complementary and Alternative Medicine, 14(1), 1–11. [Google Scholar]
4. Farcas, A. D., Mot, A. C., Zagrean-Tuza, C., Ticolea, M., Sevastre, B. et al. (2019). Remarkable rutin-rich Hypericum capitatum extract exhibits anti-inflammatory effects on turpentine oil-induced inflammation in rats. BMC Complementary and Alternative Medicine, 19(1), 1–13. [Google Scholar]
5. Gabryszewska, E., Wojtania, A. (2005). Metody rozmnażania liliowca (Hemerocallis spp.) in vitro. Zeszyty Problemowe Postępów Nauk Rolniczych, 504(2), 575–583. [Google Scholar]
6. Chen, J., Hall, D. E., de Luca, V. (2005). Effects of the growth retardant paclobutrazol on large-scale micropropagation of daylily (Hemerocallis spp.). In Vitro Cellular & Developmental Biology-Plant, 41, 58–62. [Google Scholar]
7. Krikorian, A. (1995). Regeneration of plants from protoplasts of Hemerocallis (Daylily). Plant Protoplasts and Genetic Engineering VI, 34, 70–78. [Google Scholar]
8. Meyer, M. (1976). Propagation of daylilies by tissue culture1. HortScience, 11(5), 485–487. [Google Scholar]
9. Podwyszyńska, M., Orlikowska, T., Trojak-Goluch, A., Wojtania, A. (2022). Application and improvement of in vitro culture systems for commercial production of ornamental, fruit, and industrial plants in poland. Acta Societatis Botanicorum Poloniae, 91, 914. [Google Scholar]
10. Abdelnour, S. A., Xie, L., Hassanin, A. A., Zuo, E., Lu, Y. (2021). The potential of CRISPR/Cas9 gene editing as a treatment strategy for inherited diseases. Frontiers in Cell and Developmental Biology, 9, 699597. [Google Scholar] [PubMed]
11. Raza, S. H. A., Hassanin, A. A., Pant, S. D., Bing, S., Sitohy, M. Z. et al. (2021). Potentials, prospects and applications of genome editing technologies in livestock production. Saudi Journal of Biological Sciences, 29(4), 1928–1935. [Google Scholar] [PubMed]
12. Kanyand, M., Meordrick, S., Chenxin, L. (2021). In vitro daylily (Hemerocallis species) bract multiple shoot induction. African Journal of Biotechnology, 20(2), 43–50. [Google Scholar]
13. Yi, R., Costabile, L., Cousins, A., DiMario, R., Voytas, D. et al. (2019). 2019 in vitro biology meeting, June 8–12, Tampa, Florida. In Vitro Cellular & Developmental Biology-Plant, 55, 476–501. [Google Scholar]
14. Pounders, C., Garton, S. (1996). High frequency adventitious regeneration in daylily tissue culture stimulated by thidiazuron (TDZ). Southern Nursery Association Proceeding, 41, 243–246. [Google Scholar]
15. Dunwell, W. C. (1996). Hemerocallis (Daylily) propagation. Proceedings of the Combined Proceedings-International Plant Propagators Society, vol. 46, pp. 590–594. Univ Washington-Int Plant Propagation Soc. [Google Scholar]
16. Leclerc, M., Caldwell, C. D., Lada, R. R., Norrie, J. (2006). Effect of plant growth regulators on propagule formation in Hemerocallis spp. and Hosta spp. HortScience, 41(3), 651–653. [Google Scholar]
17. Amling, J., Keever, G., Kessler Jr.,J., Eakes, D. (2007). Benzyladenine (BA) promotes ramet formation in Hemerocallis. Journal of Environmental Horticulture, 25(1), 9–12. [Google Scholar]
18. Lakshmanan, V., Reddampalli Venkataramareddy, S., Neelwarne, B. (2007). Molecular analysis of genetic stability in long-term micropropagated shoots of banana using RAPD and ISSR markers. Electronic Journal of Biotechnology, 10(1), 106–113. [Google Scholar]
19. Bairu, M. W., Aremu, A. O., van Staden, J. (2011). Somaclonal variation in plants: Causes and detection methods. Plant Growth Regulation, 63(2), 147–173. [Google Scholar]
20. Thakur, J., Dwivedi, M. D., Sourabh, P., Uniyal, P. L., Pandey, A. K. (2016). Genetic homogeneity revealed using SCoT, ISSR and RAPD markers in micropropagated Pittosporum eriocarpum Royle—an endemic and endangered medicinal plant. PLoS One, 11(7), e0159050. [Google Scholar] [PubMed]
21. Collard, B., Mackill, D. (2009). Conserved DNA-derived polymorphism (CDDPA simple and novel method for generating DNA markers in plants. Plant Molecular Biology Reporter, 27(4), 558–562. [Google Scholar]
22. Chirumamilla, P., Gopu, C., Jogam, P., Taduri, S. (2021). Highly efficient rapid micropropagation and assessment of genetic fidelity of regenerants by ISSR and SCoT markers of Solanum khasianum Clarke. Plant Cell, Tissue and Organ Culture (PCTOC), 144(2), 397–407. [Google Scholar]
23. Collard, B. C. Y., Mackill, D. J. (2009). Start codon targeted (SCoT) polymorphism: A simple, novel DNA marker technique for generating gene-targeted markers in plants. Plant Molecular Biology Reporter, 27(1), 86–93. [Google Scholar]
24. Murashige, T., Skoog, F. (1962). A revised medium for rapid growth and bio assays with tobacco tissue cultures. Physiologia Plantarum, 15(3), 473–497. [Google Scholar]
25. Doyle, J. (1991). DNA protocols for plants. In: Molecular techniques in taxonomy, vol. 57, pp. 283–293. Berlin, Heidelberg: Springer. [Google Scholar]
26. Norušis, M. J. (1993). SPSS for Windows, release 6.0. Prentice Hall (Higher Education Division, Pearson EducationHoboken, NJ, USA. https://www.ibm.com/products/spss-statistics [Google Scholar]
27. Huang, H., Wei, Y., Zhai, Y., Ouyang, K., Chen, X. et al. (2020). High frequency regeneration of plants via callus-mediated organogenesis from cotyledon and hypocotyl cultures in a multipurpose tropical tree (Neolamarkia Cadamba). Scientific Reports, 10(1), 1–10. [Google Scholar]
28. Chen, S., Xiong, Y., Yu, X., Pang, J., Zhang, T. et al. (2020). Adventitious shoot organogenesis from leaf explants of Portulaca pilosa L. Scientific Reports, 10(1), 1–8. [Google Scholar]
29. Guo, B., Abbasi, B. H., Zeb, A., Xu, L., Wei, Y. (2011). Thidiazuron: A multi-dimensional plant growth regulator. African Journal of Biotechnology, 10(45), 8984–9000. [Google Scholar]
30. Gaba, V. P. (2005). Plant growth regulators in plant tissue culture and development. In: Plant development and biotechnology, pp. 87–99. Boca Raton, FL: CRC Press. [Google Scholar]
31. Huetteman, C. A., Preece, J. E. (1993). Thidiazuron: A potent cytokinin for woody plant tissue culture. Plant Cell, Tissue and Organ Culture, 33(2), 105–119. [Google Scholar]
32. Gaspar, T., Kevers, C., Penel, C., Greppin, H., Reid, D. M. et al. (1996). Plant hormones and plant growth regulators in plant tissue culture. In Vitro Cellular & Developmental Biology-Plant, 32(4), 272–289. [Google Scholar]
33. Corredoira, E., Ballester, A., Vieitez, A. (2008). Thidiazuron-induced high-frequency plant regeneration from leaf explants of Paulownia tomentosa mature trees. Plant Cell, Tissue and Organ Culture, 95(2), 197–208. [Google Scholar]
34. Govindaraj, S. (2018). Thidiazuron: A potent phytohormone for in vitro regeneration. In: Thidiazuron: From urea derivative to plant growth regulator, pp. 393–418. [Google Scholar]
35. Giridhar, P., Vaddadi, S., Matam, P., Shreelakshmi, S. (2018). TDZ induced diverse in vitro responses in some economically important plants. Thidiazuron: From Urea Derivative to Plant Growth Regulator, 12(8), 329–341. [Google Scholar]
36. Matand, K., Wu, N., Conley, S., Acquaah, G. (2013). A more improved protocol for in vitro shoot organogenesis in daylily (Hemerocallis sp.). African Journal of Biotechnology, 12(8), 329–341. [Google Scholar]
37. Abdelghaffar, A. M., Soliman, S. S., Ismail, T. A., Alzohairy, A. M., Latef, A. A. H. A. et al. (2023). In vitro propagation of three date palm (Phoenix dactylifera L.) varieties using immature female inflorescences. Plants, 12(3), 644. [Google Scholar] [PubMed]
38. Kannan, T., Nagarajan, S., Kulothungan, S. (2006). Micropropagation of Solanum nigrum L.-a medicinal herb. Plant Archives, 6(1), 97–99. [Google Scholar]
39. Ahn, Y. J., Vang, L., McKeon, T. A., Chen, G. Q. (2007). High-frequency plant regeneration through adventitious shoot formation in castor (Ricinus communis L.). In Vitro Cellular & Developmental Biology-Plant, 43(1), 9–15. [Google Scholar]
40. Rohela, G. K., Jogam, P., Bylla, P., Reuben, C. (2019). Indirect regeneration and assessment of genetic fidelity of acclimated plantlets by SCoT, ISSR, and RAPD markers in Rauwolfia tetraphylla L.: An endangered medicinal plant. BioMed Research International, 2019, 3698742. [Google Scholar] [PubMed]
41. Savita, Bhagat, A., Pati, P. K., Virk, G. S., Nagpal, A. (2012). An efficient micropropagation protocol for Citrus jambhiri Lush. and assessment of clonal fidelity employing anatomical studies and RAPD markers. In Vitro Cellular & Developmental Biology-Plant, 48(5), 512–520. [Google Scholar]
42. Al-Khayri, J. M., Mahdy, E. M. B., Taha, H. S. A., Eldomiaty, A. S., Abd-Elfattah, M. A. et al. (2022). Genetic and morphological diversity assessment of five kalanchoe genotypes by SCoT, ISSR and RAPD-PCR markers. Plants, 11(13), 1722. [Google Scholar] [PubMed]
43. Ajithan, C., Vasudevan, V., Sathish, D., Sathish, S., Krishnan, V. et al. (2019). The influential role of polyamines on the in vitro regeneration of pea (Pisum sativum L.) and genetic fidelity assessment by SCoT and RAPD markers. Plant Cell, Tissue and Organ Culture (PCTOC), 139(3), 547–561. [Google Scholar]
44. Muthukumar, M., Muthukrishnan, S., Kumar, T. S., Rao, M. V. (2020). Direct regeneration, microshoot recovery and assessment of genetic fidelity in Helicteres isora L., a medicinally important tree. Biocatalysis and Agricultural Biotechnology, 23, 101415. [Google Scholar]
45. Sadhu, S., Jogam, P., Thampu, R. K., Abbagani, S., Penna, S. et al. (2020). High efficiency plant regeneration and genetic fidelity of regenerants by SCoT and ISSR markers in chickpea (Cicer arietinum L.). Plant Cell, Tissue and Organ Culture (PCTOC), 141(3), 465–477. [Google Scholar]
46. Atul Babu, G., Vinoth, A., Ravindhran, R. (2018). Direct shoot regeneration and genetic fidelity analysis in finger millet using ISSR markers. Plant Cell, Tissue and Organ Culture (PCTOC), 132(1), 157–164. [Google Scholar]
47. Rohela, G. K., Jogam, P., Shabnam, A. A., Shukla, P., Abbagani, S. et al. (2018). In vitro regeneration and assessment of genetic fidelity of acclimated plantlets by using ISSR markers in PPR-1 (Morus sp.An economically important plant. Scientia Horticulturae, 241, 313–321. [Google Scholar]
48. Martins, M., Sarmento, D., Oliveira, M. (2004). Genetic stability of micropropagated almond plantlets, as assessed by RAPD and ISSR markers. Plant Cell Reports, 23(7), 492–496. [Google Scholar] [PubMed]
49. Saker, M., Adawy, S., Mohamed, A., El-Itriby, H. (2006). Monitoring of cultivar identity in tissue culture-derived date palms using RAPD and AFLP analysis. Biologia Plantarum, 50, 198–204. [Google Scholar]
50. Sharma, U., Agrawal, V. (2018). In vitro shoot regeneration and enhanced synthesis of plumbagin in root callus of Plumbago zeylanica L.—An important medicinal herb. In Vitro Cellular & Developmental Biology-Plant, 54, 423–435. [Google Scholar]
51. Srivastava, S., Krishna, R., Sinha, R. P., Singh, M. (2017). TDZ-induced plant regeneration in Brassica oleracea L. var. botrytis: Effect of antioxidative enzyme activity and genetic stability in regenerated plantlets. In Vitro Cellular & Developmental Biology-Plant, 53, 598–605. [Google Scholar]
52. Bhardwaj, A. K., Singh, B., Kaur, K., Roshan, P., Sharma, A. et al. (2018). In vitro propagation, clonal fidelity and phytochemical analysis of Rhodiola imbricata Edgew: A rare trans-Himalayan medicinal plant. Plant Cell, Tissue and Organ Culture (PCTOC), 135, 499–513. [Google Scholar]
53. Hassanin, A. A., Saad, A. M., Bardisi, E. A., Salama, A., Sitohy, M. Z. (2020). Transfer of anthocyanin accumulating Delila and Rosea1 genes from the transgenic tomato micro-tom cultivar to moneymaker cultivar by conventional breeding. Journal of Agricultural and Food Chemistry, 68(39), 10741–10749. [Google Scholar] [PubMed]
54. Ghareeb, Y. E., Soliman, S. S., Ismail, T. A., Hassan, M. A., Abdelkader, M. A. et al. (2022). Improvement of german chamomile (Matricaria recutita L.) for mechanical harvesting, high flower yield and essential oil content using physical and chemical mutagenesis. Plants, 11(21), 2940. [Google Scholar] [PubMed]
55. Hassanin, A. A., Soliman, S. S. A., Ismail, T. A., Amin, M. K. A. (2017). The role of SLMYB gene in tomato fruit development. Zagazig Journal of Agricultural Research, 44(3), 969–988. [Google Scholar]
56. Hassanin, A., Osman, A., Atallah, O., El-Saadony, M., Abdelnour, S. et al. (2022). Phylogenetic comparative analysis: Chemical and biological features of caseins (alpha-S-1, alpha-S-2, beta-and kappa-) in domestic dairy animals. Frontiers in Veterinary Science, 9, 952319. [Google Scholar] [PubMed]
57. Essa, S. M., Wafa, H. A., Mahgoub, E. S. I., Hassanin, A. A., Al-Khayri, J. M. et al. (2023). Assessment of eight faba bean (Vicia faba L.) cultivars for drought stress tolerance through molecular, morphological, and physiochemical parameters. Sustainability, 15(4), 3291. [Google Scholar]
58. AL-Khayri, J. M., Abdel latef, A. A. H., Taha, H. S. A., Eldomiaty, A. S., Abd-elfattah, M. A. et al. (2022). In silico profiling of proline biosynthesis and degradation related genes during fruit development of tomato. SABRAO Journal of Breeding and Genetics, 54(3), 549–564. [Google Scholar]
Cite This Article
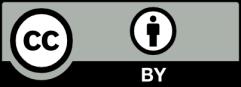
This work is licensed under a Creative Commons Attribution 4.0 International License , which permits unrestricted use, distribution, and reproduction in any medium, provided the original work is properly cited.