Open Access
ARTICLE
Pollination Biology of the Endangered Herbal Medicines Dendrobium chrysotoxum (Orchidaceae)
1 Medicinal Plant Research Center, Dehong Tropical Agriculture Research Institute of Yunnan, Ruili, 678600, China
2 Human Resources Management Office, The People’s Hospital of Xiangyun, Xiangyun, 672100, China
* Corresponding Authors: Shanshan Yin. Email: ; Zesheng Li. Email:
(This article belongs to the Special Issue: Plant–Environment Interactions)
Phyton-International Journal of Experimental Botany 2023, 92(7), 1975-1986. https://doi.org/10.32604/phyton.2023.028232
Received 06 December 2022; Accepted 27 February 2023; Issue published 29 May 2023
Abstract
Pollination biology studies of the endangered herbal medicines Dendrobium chrysotoxum were conducted in natural pollination conditions using flower observation, pollinator observation and artificial pollination experiments. Populations of D. chrysotoxum with fragrance and nectar were pollinated by Ctenoplectra davidi Valhalla (Hymenoptera: Apidae) species. The floral structure of D. chrysotoxum adapted precisely to its pollinators. Flowers had a low capsule setting (0.17%) under natural conditions. However, compared to open pollination, artificial pollination experiments showed a significant increase in capsule setting, and D. chrysotoxum was cross-compatible and self-compatible, but there was pollinator limitation also. This study will provide important information for the preservation of this endangered species.Keywords
One of the biggest genera in the Orchidaceae family is Dendrobium, which has more than 1200 species [1]. While 11 species of Dendrobium were recorded and have been used as medical plants for a long time [2], the majority of species in this genus have great decorative, medicinal, and commercial benefits and are prized by botanists and plant enthusiasts [3]. From the stems of many Dendrobium species, certain secondary metabolites have been identified. These compounds exhibit a variety of biological effects, including antimalarial, anticancer, hepatoprotective, and immunomodulatory activities [4–6].
Dendrobium was an endangered species due to its poor germination rate, sluggish development, habitat degradation, and excessive human exploitation for large profits [7]. One of the critically endangered Dendrobium species with great ornamental value is D. chrysotoxum. It grows between 520 and 1620 msl on tree trunks in the open forests of the North Eastern Himalayas, and is also found in China’s Southwest Yunnan Province [8]. These species were classified in the Chinese Pharmacopoeia as well as being utilized in traditional herbal treatments in China and India [9,10]. We investigated the pollination biology of D. chrysotoxum previously in Tongbiguan Nature Reserve. Unfortunately, the number of capsules setting produced by D. chrysotoxum in a wild was quite low, and the species had a very limited population with severely fragmented habitats. It is commonly known that the crucial stages in the plant reproductive process are pollination and breeding. Research on D. chrysotoxum’s pollination and breeding is quite challenging since it frequently grows on towering tree trunks. Studies on D. chrysotoxum’s pollination are problematic since it is rarely visited by pollinators and lives in locations that are difficult to access. As a result, pollen deposition is hardly observed. Since all research must be done in the wild, obtaining these data is quite challenging. The floral morphology and pollination biology of D. chrysotoxum must be thoroughly studied, crucial prerequisites for conservation. Up to now, pollination and reproduction of D. chrysotoxum have never been investigated or directly observed. The reproduction of D. chrysotoxum was first described in this paper based on field observations and pollination tests, which may serve as a foundation for the restoration and management of D. chrysotoxum’s conservation.
The study was carried out in the Dendrobium germplasm resource garden in Ruili City, Yunnan Province, China (97°51′4.79″–97°51′14.64″ E, 24°01′9.42″–24°01′14.00″ N; 770–779 msl). The Dendrobium germplasm resource garden was situated on the Mengxiu Mountain, which has a high temperature tropical monsoon climate in Tongbiguan Nature Reserve. Since 2002, D. chrysotoxum was mostly propagated using plant division, cuttage, and tissue culture, and then it was planted on the stem of trees by imitating wild conditions. Tissue culture uses seeds, the product of sexual reproduction of D. chrysotoxum, as explants to obtain many individuals. More importantly, different individuals are labeled.
2.2 Floral Phenology and Morphology
From March to May 2020, D. chrysotoxum’s floral phenology was monitored to note flowering period’s beginning, peak, and finish. On 40 inflorescences in the bud stage (40 plants), 100 unopened flowers were marked randomly and then examined. When a flower’s dorsal sepal parted from an extended labellum, it was considered to be open. A flower was considered closed when the labellum wilted and crumpled. From the opening of the first bloom until the collapse and withering of the last flower, the flowering time of each individual flower and the flowering duration of each population were observed.
The following parameters were measured with vernier calipers (0.01 mm) to examine the floral morphology of 30 fresh flowers on 30 additional unopened inflorescences (30 plants). These measurements included the length and width of the petal, the labellum, the central sepal, the lateral sepal, the height of the flower passage, the width of the flower passage [11,12] and the depth of the flower passage (the distance between the spur mouth and the middle of the stigmatic surface).
In order to determine if the flowers produced nectar, 30 flowers from 10 individual D. chrysotoxum plants were randomly chosen in late April of 2020, and their spurs were examined using a stereoscopic microscope (Leica M165 C, Germany).
2.3 Flower Visitors and Their Visiting Behavior
SONY digital cameras (Sony ILCE-7M2, Thailand) were utilized to capture visitors in the research locations throughout D. chrysotoxum’s flowering periods in 2019 and 2020, as well as to note their behavior. The observation times every day were from 6:00 until 18:00. By observing the disappearance of pollinia in the mornings, the nightly visitors were assessed. Behavior and species of insect visitors were observed and recorded by camera, with the record of daily weather conditions.
The ethyl acetate fumes from ethyl acetate were used to catch and kill the insects that were observed visiting D. chrysotoxum in a jar. Insect specimens were identified by X. Gao and R. Zhang of Yunnan Agriculture University. Voucher specimens are deposited at the Dehong Tropical Agriculture Research Institute of Yunnan. On the caught insects, the following dimensions were recorded: the height of the insect’s thorax, the width of the insect body, and the length between the midpoint of the thorax and the endpoint of the proboscis.
3000 flowers of D. chrysotoxum that were planted on the stems of trees in 2019 and 2020 made up the sampling. Each flower was marked and labeled. We checked that all labelled flowers contained pollinia at the column apex but no pollinia deposited in the stigmatic cavity. Success of natural pollination conditions was estimated as capsules setting. In 2019 and 2020, artificial pollination treatments comprised untouched flowers as direct autogamy, self-pollination, and cross-pollination. This sampling included 400 flowers on 40 inflorescences (40 plants). The labellum of each flower expanded and opened before any insect activity happened on the first day for all selected flowers in each treatment. Flowers were chosen at random and divided into three treatments. (1) Direct autogamy. Flowers were bagged in white paper bags before anthesis till senescence. (2) Self-pollination. A second flower on the same plant provided the stigma with whole pollinia. (3) Cross-pollination. Each stigma received whole pollinia from another plant’s flower. At the end of the flowering period, the ovary’s swelling was noticed, and the number of capsule setting was counted. At 24, 48, 72, and 96 h following pollination, pollen tube growth was observed. The observed samples were fixed, softened, and stained [13], then a rotary microtome (Leica CM3050S, Germany) was used to cut sample into longitudinal slices (90 μm), and fluorescence microscopy (Leica DM5000 B, Germany) was used to observe the sample.
Comparison of the lengths of dorsal sepals, petals, and labellums of D. chrysotoxum was assessed using the Duncan test while comparing the morphological traits of D. chrysotoxum flowers and the pollinator was carried out using the independent sample T test by SPSS version 19.
3.1 Floral Phenology and Morphology
Flowering of D. chrysotoxum began in early March, peaked in mid-April, and finished in mid-May. An individual flower had a life span of about 12 days under natural conditions. Fertilized flowers would wither in approximately 3 days after pollination. The inflorescence of D. chrysotoxum was a raceme with 10 to 20 flowers (Fig. 1A). Furthermore, the flowers of D. chrysotoxum often emitted a mild fragrant scent when exposed to direct sunlight or high temperatures during the day. The flowers of D. chrysotoxum were both zygomorphic and hermaphroditic (Figs. 1C, 1F and 1G). The sepals were golden and oval. The petals were yellow and ovate. The labellums were large oval, yellow, and had a sorrel-colored core with trichomes (Fig. 1B). The mean length and width of lateral sepals, central sepal, petals and labellum were 21.13 mm (SD = 1.2, N = 30) and 9.91 mm (SD = 0.6, N = 30), 21.30 mm (SD = 1.3, N = 30) and 10.18 mm (SD = 0.5, N = 30), 24.99 mm (SD = 1.2, N = 30) and 15.49 mm (SD = 0.8, N = 30), and 25.90 mm (SD = 1.1, N = 30) and 28.97 mm (SD = 1.9, N = 30), respectively. A short tissue connected the top of the yellow anther cap to the gynostemium (Figs. 1I–1K). The pollinia were ceraceous and yellow under the anther cap (Fig. 1L). The flowers of D. chrysotoxum possessed a single nectar gland at the bottom of the spur (Figs. 1D, 1E and 1H). Base on the fact that nectar secretions were found on every single one of the 30 flowers analyzed, the spur dissection experiment results revealed that D. chrysotoxum generates nectar.
Figure 1: The inflorescence and flower of D. chrysotoxum. (A) Inflorescence of D. chrysotoxum. (B) Flower. (C) Floral architecture of D. chrysotoxum. (D) An enclosing nectar gland space compressed by protrusion (arrowheads) of labellum. (E) The entry of nectar stealers was blocked protrusion of labellum. (F) Gynostemium. (G) Stigma. (H) Nectar gland. (I) ‘Tipping-like’ anther cap. (J) The front of anther cap. (K) The back of anther cap. (L) Pollinia. Scale bars = 5 mm (A–C), 500 µm (D to L)
3.2 Flower Visitors and Their Visiting Behavior
We discovered that hymenopterans were the most abundant insect visitors based on the observed visitations. It was also noted that members of the Coleoptera and Diptera visited the flowers of D. chrysotoxum, but they did not engage in typical pollinator behavior; rather, the majority of them only simply landed or rested on the flowers. Although the thrip Frankliniella occidentalis and the ant Camponotus japonicus couldn’t get nectar and could not carry pollinia due to their small bodies, occasionally nectar-seeking behavior was observed. In the garden, we counted 29 species of visiting insects, although the majority were not actual pollinators (Table 1). Only one species of the visiting insects was observed to pollinate, depositing pollinia and removing them from the scutum of Ctenoplectra davidi Valhalla (Hymenoptera: Apidae) (Figs. 2A and 2B). The pollinators of D. chrysotoxum were exceptionally rare, hardly observable in the garden. On sunny or warm days, C. davidi usually appeared. C. davidi visited the flowers most frequently between the hours of 13:00 and 15:00 one day in April. They frequently flew quickly and landed on the flower of D. chrysotoxum. Then C. davidi crawled toward the sorrel center of the labellum with trichomes and probed into the spur cavity. The C. davidi was prevented from entering by the labellum’s protrusion. They held labellum with their fore and middle legs and inserted their mouthparts deeply into the spur to find and suck nectar at the base of the passage with bitingsucking mouthparts. By pushing back of the labellum with a flexible joint, a retreating C. davidi of appropriate size obtained smearing of stigmatic liquid on its scutum, its scutellum sticked anther cap and pushed back the anther cap, coupling with the anther cap falling (Fig. 2C). ‘Tipping-like’ anther cap delivered the pollinia precisely to the scutum of C. davidi. After C. davidi has left the flower, the pollinia could be positioned on the scutum in the middle of its thorax. If a C. davidi carrying pollinia on its scutum entered a second flower of D. chrysotoxum that lacked pollinia on its receptive stigma. Then C. davidi leaved the flower, pollinia was placed on the stigma precisely by pushing back the labellum (Fig. 3).
Figure 2: Visiting behavior and morphology of pollinators. (A) C. davidi is carrying pollinia on its scutum. (B) The pollinia positioned on the scutum of C. davidi. (C) Scutellum and scutum of C. davidi. Scale bars = 5 mm (A and B), 1 mm (C)
Figure 3: The pollination process of D. chrysotoxum
3.3 Floral Architecture and Morphological Adaptations
Some more Dendrobium species were blooming at the same time as D. chrysotoxum, such as D. thyrsiflorum, D. fimbriatum, and D. nobile. Their gynostemia were intact, and the anther cap had not been pushed away. So, their pollinia were not lost and never removed by C. davidi. As a result, it was certain that the pollen carried by C. davidi originated from D. chrysotoxum. This finding agreed with C. davidi being the pollinator of D. chrysotoxum. Therefore, we could surmise from the observation that C. davidi was the only pollinator of D. chrysotoxum. A comparison of the pollinator and D. chrysotoxum flower sizes provided evidence to support this hypothesis (Table 2). The mean height of C. davidi thorax was 2.46 mm (SD = 0.12, N = 11), the mean width of C. davidi body was 3.03 mm (SD = 0.14, N = 11), the mean length between the midpoint of the thorax and the endpoint of the proboscis was 4.54 mm (SD = 0.23, N = 11). Then the flower passage, which had a mean height of 2.75 mm (SD = 0.24, N = 30), a mean width of 3.44 mm (SD = 0.26, N = 30), a mean depth of 4.53 mm (SD = 0.26, N = 30), fitted the pollinator needs perfectly. The chances that C. davidi would remove pollinia and ensure that the pollinia were transferred to another flower were increased by these morphological changes to the flowers. These results indicated that the floral structure of D. chrysotoxum was well adapted to its pollinators. When C. davidi was probing the spur cavity, the curved gynostemium of the flower exactly fitted the scutum and scutellum between the mesothorax and the abdomen of C. davidi. The protrusion of labellum made the C. davidi arch its body, and thus the hind edge of the mesothorax rose up and reached a position towarded the anther cap and stigma when the C. davidi lowered its head to probe deep into the spur cavity (Figs. 4A–4C). The pollinia changed their direction passively and pointed towards the stigma as C. davidi left and entered the flower, and pollination was completed.
Figure 4: Floral architecture and morphological adaptations. (A) Longitudinal sections of labellum and gynostemium. (B) Reached a position towards the anther cap. (C) ‘Tipping-like’ anther cap. Scale bars = 1 mm (A–C)
Only 0.17% of capsules setting were naturally pollinated in 2019, which was quite low. No capsule setting of D. chrysotoxum was formed under natural pollination conditions in 2020. No capsule setting of D. chrysotoxum was formed by direct autogamy in 2019 and 2020. The capsules setting of flowers treated by self-pollination was 17.75%, 5.50% in 2019 and 2020, respectively, while the capsules setting of flowers treated by cross-pollination was 85.00% and 99.25% (Table 3). The data showed very low capsules setting at natural pollination conditions, especially there was no capsule setting in the absence of pollinators. Whereas artificial pollination was known to yield high capsules setting rates, both when the flowers were treated by self-pollination or cross-pollination, proving that they were cross-compatible and partially self-compatible. During pollen tube growth of self-pollinated individuals, the pollen tube grew very slowly and was short (Figs. 5A–5D), black spots were present after 48 h of self-pollination (Fig. 5B), and the zone of black spots became larger until the pollen tube grew into the ovary (Fig. 5D). This might be due to the degradation of callose in the pollen tube which cannot make the pollen tube fluorescent, leading to the partly Self-incompatibility phenotype. Inversely, the pollen tube bundle of cross-pollinated individuals grew faster, reaching the ovaries in 72 h or 96 h after cross-pollination (Figs. 5E–5H).
Figure 5: Growth of pollen tubes after self-pollination and cross-pollination of D. chrysotoxum. (A) 24 h after self-pollination. (B) 48 h after self-pollination. (C) 72 h after self-pollination. (D) 96 h after self-pollination. (E) 24 h after cross-pollination. (F) 48 h after cross-pollination. (G) 72 h after cross-pollination. (H) 96 h after cross-pollination. Scale bars = 500 µm (A to H)
The large, yellow flowers of D. chrysotoxum were presumed to have an attraction of the C. davidi in the distance, as well as the fragrant scent might have played a significant part in their attraction. Plants attracted pollinators through floral displays, fragrance, and nectar, all of which could increase the frequency of pollinator visits to flowers [14]. There were 10–20 flowers in each of the inflorescences that a D. chrysotoxum plant might generate (Fig. 1A). D. chrysotoxum used a large-scale display as part of its pollination strategy, expanding the inflorescence by adding flowers, which would increase the attraction units of more pollen donors and receivers.
This mechanism of pollination was special. Effective orchid conservation required an understanding of the D. chrysotoxum pollination mechanism. Only C. davidi was found to be a pollinator in the Dendrobium germplasm resource garden out of the 29 species of visiting insects that were present in the D. chrysotoxum (Table 1). About 67% of Orchidaceae plants had just one pollinator species [15]. Although the majority of Dendrobium species were self-compatible, pollinators were necessary to transport pollen. The exception was D. wangliangii, which was spontaneously self-pollinated through the growing pollinium slid down the stigma [16]. A single species of C. davidi appeared to be the only pollinator of D. chrysotoxum flowers in the Dendrobium germplasm resource garden. The measuring results demonstrated that the mean height and width of the D. chrysotoxum entry were marginally greater than the thorax dimensions of the C. davidi, permitting either removal or deposition of pollinia (Table 2). As a result, the functional architecture of D. chrysotoxum flowers fitted the physical characteristics of the species of pollinators. Usually, significant floral variety in many big Cape genera was likely explained by adaptive changes in pollination systems [17]. Therefore, pollination evidence implies that adaptation frequently contributed to floral diversity [18].
The majority of orchid species had low capsule setting [19]. The rates of capsules setting in D. chrysotoxum flowers treated with self-pollination within two years were 17.75% in 2019 and 0.00% in 2020 (Table 3). The environment in various years might have played a role in this difference. In natural conditions, the capsule setting (0.17%) was incredibly low. However, compared to natural pollination, artificial pollination experiments revealed a significantly higher setting of capsules, indicating that D. chrysotoxum was self-compatible. While direct autogamy was not found, pollinators seemed to be crucial for capsules setting under capsules setting. It has been widely reported that orchids exhibit self-compatibility, which was probably the first step towards mechanical self-pollination and was also thought to be an adaptation for poor pollinators [20–22].
Many studies mainly focused on the effect of floral structural traits on pollinators, such as flower size and display [23]. So far, fewer studies paid attention to the connection of the mechanical fit between flowers and pollinators. Both pollinators and nectar-seeking insects were prevented from sucking by the labellum’s projection (Fig. 1D). In order to obtain nectar from the flowers, pollinators must hold the labellum and insert their mouthparts into the nectar gland (Fig. 3). However, not all pollinators could discover the nectar gland and successfully suck nectar, and those who are not paid will not return to the flowers. Nectar was not lost in twenty-one Asian Dendrobium species [24]. Research has revealed that pollination rates were higher in rewarding species [25]. Despite the fact that D. chrysotoxum might produce nectar and provide rewards, capsule setting was still very low, which might be due to the pollinator limitation or the fact that some pollinators were prevented from obtaining nectar. Pollinator limitation was frequently one of the possible reasons of the low level of fruit setting by open pollination [26]. According to the high capsule setting by artificial pollination but low capsule setting under natural conditions for D. chrysotoxum, there was a pollinator limitation under natural conditions. According to Stpiczyska, a large display attracted more pollinators and increases reproductive success [27]. This limitation had not been entirely removed by numerous members of D. chrysotoxum plants attracted more pollinator species. However, several kinds of research found no connection between the number of flowers and the probability of setting fruit [28]. Nevertheless, it should be mentioned that the study location was in the Dendrobium germplasm resource garden, where the pollinator limitation would be worse. These findings implied a significant pollinator limitation in the D. chrysotoxum population under study.
The survival of D. chrysotoxum might be seriously threatened by the disruption of the interactions among plants, pollinators, and food sources. The study of pollinators was crucial for reintroducing D. chrysotoxum to its original habitat. Plant-pollinator interactions should not be overlooked when deciding on protection measures for endangered plants in order to ensure their success in sexual reproduction [29]. Additionally, artificial pollination would be beneficial for increasing the capsule setting and improving reproductive success, thus enhancing D. chrysotoxum’s recovery.
Funding Statement: This work was funded by the Basic Research Priorities Program of Yunnan Province-the Applied Basic Research Programs of Science and Technology Department for Young Scholars (No. 2019FD005), Technological Innovation Talents Cultivation Project of Yunnan Province (No. 202205AD160043), and Technological Innovation Talents Cultivation Project of Dehong City (No. 2021RC007).
Conflicts of Interest: The authors declare that they have no conflicts of interest to report regarding the present study.
References
1. Adams, P. B. (2011). Systematics of Dendrobiinae (Orchidaceaewith special reference to Australian taxa. Botanical Journal of the Linnean Society, 166(2), 105–126. [Google Scholar]
2. Hossain, M. M. (2011). Therapeutic orchids: Traditional uses and recent advances—An overview. Fitoterapia, 82(2), 102–140. [Google Scholar] [PubMed]
3. Pridgeon, A. M., Cribb, P. J., Chase, M. W., Rasmussen, F. N. (2014). Genera orchidacearum volume 6: Epidendroideae (part 3). UK: Oxford University Press. [Google Scholar]
4. Liu, X. F., Zhu, J., Ge, S. Y., Xia, L. J., Yang, H. Y. (2011). Orally administered Dendrobium officinale and its polysaccharides enhance immune functions in BALB/c mice. Natural Product Communications, 6(6), 867–870. [Google Scholar] [PubMed]
5. Chanvorachote, P., Kowitdamrong, A., Ruanghirun, T., Sritularak, B., Mungmee, C. (2013). Anti-metastatic activities of bibenzyls from Dendrobium pulchellum. Natural Product Communications, 8(1), 115–118. [Google Scholar] [PubMed]
6. Sukphan, P., Sritularak, B., Mekboonsonglarp, W., Lipipun, V., Likhitwitayawuid, K. (2014). Chemical constituents of Dendrobium venustum and their antimalarial and anti-herpetic properties. Natural Product Communications, 9(6), 825–827. [Google Scholar] [PubMed]
7. Luo, K., Li, Z. S., Bai, Y. B., Jiang, Y., Yao, Z. J. (2021). Diversity and protection of Dendrobium. Heilongjiang Agricultural Sciences, 326(8), 85–89. [Google Scholar]
8. Chen, X. Q., Luo, Y. B., Wood, J. J. (2009). Flora of China. China: Science Press Beijing, Missouri Botanical Garden Press. [Google Scholar]
9. National Pharmacopoeia Commission (2020). People’s Republic of China pharmacopoeia. China: Chinese Medicine Science and Technology Press. [Google Scholar]
10. Shailajan, S., Kumaria, S., Gurjar, D., Joshi, M., Paul, P. (2015). Variation in the marker content of five different Dendrobium species: Comparative evaluation using validated HPTLC technique. Journal of Applied Pharmaceutical Science, 5(10), 32–38. [Google Scholar]
11. Brodmann, J., Twele, R., Francke, W., Luo, Y. B., Song, X. Q. (2009). Orchid mimics honey bee alarm pheromone in order to attract hornets for pollination. Current Biology, 19(16), 1368–1372. [Google Scholar] [PubMed]
12. Li, P., Luo, Y., Bernhardt, P., Kou, Y., Perner, H. (2008). Pollination of Cypripedium plectrochilum (Orchidaceae) by Lasioglossum spp. (HalictidaeThe roles of generalist attractants versus restrictive floral architecture. Plant Biology, 10(2), 220–230. [Google Scholar] [PubMed]
13. Niu, S. C., Huang, J., Xu, Q., Li, P. X., Yang, H. J. (2018). Morphological type identification of self-incompatibility in Dendrobium and its phylogenetic evolution pattern. International Journal of Molecular Sciences, 19(9), 2595. [Google Scholar] [PubMed]
14. Faast, R., Farrington, L., Facelli, J. M., Austin, A. D. (2009). Bees and white spiders: Unravelling the pollination syndrome of Caladenia rigida (Orchidaceae). Australian Journal of Botany, 57(4), 315–325. [Google Scholar]
15. Tremblay, R. L. (1992). Trends in the pollination ecology of the Orchidaceae: Evolution and systematics. Canadian Journal of Botany, 70(3), 642–650. [Google Scholar]
16. Wang, Q., Shao, S., Su, Y., Hu, X., Shen, Y. (2019). A novel case of autogamy and cleistogamy in Dendrobium wangliangii: A rare orchid distributed in the dry-hot valley. Ecology and Evolution, 9(22), 12906–12914. [Google Scholar] [PubMed]
17. Johnson, S. D. (1996). Pollination, adaptation and speciation models in the Cape flora of South Africa. Taxon, 45(1), 59–66. [Google Scholar]
18. Harder, L. D., Johnson, S. D. (2009). Darwin’s beautiful contrivances: Evolutionary and functional evidence for floral adaptation. New Phytologist, 183(3), 530–545. [Google Scholar] [PubMed]
19. Tremblay, R. L., Ackerman, J. D., Zimmerman, J. K., Calvo, R. N. (2005). Variation in sexual reproduction in orchids and its evolutionary consequences: A spasmodic journey to diversification. Biological Journal of the Linnean Society, 84(1), 1–54. [Google Scholar]
20. Sipes, S. D., Tepedino, V. J. (1995). Reproductive biology of the rare orchid, Spiranthes diluvialis: Breeding system, pollination, and implications for conservation. Conservation Biology, 9(4), 929–938. [Google Scholar]
21. Clayton, S., Aizen, M. A. (1996). Effects of pollinia removal and insertion on flower longevity in Chloraea alpina (Orchidaceae). Evolutionary Ecology, 10(6), 653–660. [Google Scholar]
22. Galetto, L., Bernardello, G., Rivera, G. L. (1997). Nectar, nectaries, flower visitors, and breeding system in five terrestrial Orchidaceae from central Argentina. Journal of Plant Research, 110(4), 393–403. [Google Scholar]
23. Parachnowitsch, A. L., Kessler, A. (2010). Pollinators exert natural selection on flower size and floral display in Penstemon digitalis. New Phytologist, 188(2), 393–402. [Google Scholar] [PubMed]
24. Jia, L. B., Huang, S. Q. (2022). An examination of nectar production in 34 species of Dendrobium indicates that deceptive pollination in the orchids is not popular. Journal of Systematics and Evolution, 60(6), 1371–1377. [Google Scholar]
25. Scopece, G., Cozzolino, S., Johnson, S. D., Schiestl, F. P. (2010). Pollination efficiency and the evolution of specialized deceptive pollination systems. The American Naturalist, 175(1), 98–105. [Google Scholar] [PubMed]
26. Matsui, K., Ushimaru, T., Fujita, N. (2001). Pollinator limitaton in a deceptive orchid, Pogonia japonica, on a floating peat mat. Plant Species Biology, 16(3), 231–235. [Google Scholar]
27. Stpiczyńska, M. (2003). Floral longevity and nectar secretion of Platanthera chlorantha (Custer) Rchb. (Orchidaceae). Annals of Botany, 92(2), 191–197. [Google Scholar] [PubMed]
28. Sabat, A. M., Ackerman, J. D. (1996). Fruit set in a deceptive orchid: The effect of flowering phenology, display size, and local floral abundance. American Journal of Botany, 83(9), 1181–1186. [Google Scholar]
29. Ashworth, L., Aguilar, R., Galetto, L., Aizen, M. A. (2004). Why do pollination generalist and specialist plant species show similar reproductive susceptibility to habitat fragmentation? Journal of Ecology, 92(4), 717–719. https://doi.org/10.1111/j.0022-0477.2004.00910.x [Google Scholar] [CrossRef]
Cite This Article
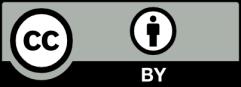
This work is licensed under a Creative Commons Attribution 4.0 International License , which permits unrestricted use, distribution, and reproduction in any medium, provided the original work is properly cited.