Open Access
ARTICLE
Effects of Different Nitrogen and Phosphorus Synergistic Fertilizer on Enzymes and Genes Related to Nitrogen Metabolism in Wheat
Dryland-Technology Key Laboratory of Shandong Province, College of Agronomy, Qingdao Agricultural University, Qingdao, China
* Corresponding Author: Yan Shi. Email:
(This article belongs to the Special Issue: Comprehensive Effects of Biochar or other Additives after Applied to Agricultural and Forest Soils)
Phyton-International Journal of Experimental Botany 2023, 92(7), 2151-2164. https://doi.org/10.32604/phyton.2023.027930
Received 22 November 2022; Accepted 09 March 2023; Issue published 29 May 2023
Abstract
In recent years, in order to improve nutrient use efficiency, especially nitrogen use efficiency, fertilizer value-added technology has been developed rapidly. However, the mechanism of the effect of synergistic fertilizer on plant nitrogen utilization is not clear. A study was, therefore, conducted to explore the activities and gene expression of key enzymes for nitrogen assimilation and the gene expression of nitrogen transporters in wheat after the application of synergistic fertilizer. Soil column experiment was set up in Qingdao Agricultural University experimental base from October 2018 to June 2019. Maleic acid and itaconic acid were copolymerized with acrylic acid as cross-linking monomer to make a fluid gel, which was sprayed on the fertilizer surface to make nitrogen and phosphorus synergistic fertilizer. A total of 6 treatments was set according to different nitrogen and phosphorus fertilizer ratios: (1) 100% common nitrogen fertilizer+100% common phosphate fertilizer (2) 70% nitrogen synergistic fertilizer+100% phosphorus synergistic fertilizer; (3) 100% nitrogen synergistic fertilizer+70% phosphorus synergistic fertilizer; (4) 100% nitrogen synergistic fertilizer+100% phosphorus synergistic fertilizer; (5) 70% nitrogen synergistic fertilizer+70% phosphorus synergistic fertilizer; (6) 100% commercial nitrogen synergistic fertilizer+100% commercial phosphorus synergistic fertilizer. The results are as follows: (1) the enzyme activities of wheat plants under synergistic fertilizer condition were higher than those under ordinary fertilizer, except under the treatment that nitrogen and phosphorus synergistic fertilizer were both reduced; (2) the expression level of the genes under the treatment “100% nitrogen synergistic fertilizer+100% phosphorus synergistic fertilizer” was significantly higher than those in other treatments. Combined with the higher performance of nitrogen concentration in various parts of the plant under the condition of applying synergistic fertilizer, this study indicated that the application of synergistic fertilizer can improve the nitrogen metabolism of the plant by increasing the nitrogen level in the rhizosphere soil, inducing the expression of nitrogen transporter genes and key assimilation enzymes genes.
Keywords
Wheat (Triticum aestivum L.) is one of the major staple grain crops, and fertilization plays an important role in increasing its yield. However, in recent years, the yield seems to be stable [1], the increase in the crop growth rate and yield have not matched the increase in the rate of fertilizer application [2]. It seems that the agronomic production model of increasing yield by increasing fertilizer input has become unsustainable, and the application of fertilizer is accompanied by huge energy and environmental costs [3–5]. To overcome this problem, polymer coating can be used on ordinary fertilizer to improve its efficiency and reduce the release rate of nutrients in the soil [6]. Slow-release technology is the latest highly developed method of nutrition feeding plants. So far, various slow-release fertilizers with different materials, preparation methods and mechanisms of action have been developed [7]. The combination of superabsorbent resin and slow-release fertilizer can improve soil water-holding and nutrient retention capacity [8]. Acrylic acid is the most widely used monomer in the preparation of super absorbent hydrogel, so it’s commonly used in slow-release fertilizers [8–10]. Itaconic acid produced by biological fermentation is one of 12 bio-based platform chemicals because of its special properties such as renewability, degradability and economic advantages [11]. Zhao et al. [12] combined itaconic acid, urea and acrylic acid in methanol to form oligomers, which could be quickly cross-linked by methanol evaporation to obtain a new high-performance water and nutrient retaining fertilizer, effectively reducing the loss of water and nutrients.
Nitrogen is an important factor related to yield in the plant metabolic system. The absorption and utilization of nitrogen to plants is a complex process, including nitrogen absorption, reduction, assimilation, transport and reuse, which is affected by various factors [13]. In general, the plant-soil nitrogen cycle can be broken down into two major sections: environmental (how the plant accesses nitrogen) and genetic (how the plant uses it once it is assimilated) [14]. Previous studies have shown that slow-release fertilizer can effectively improve fertilizer utilization and enhance crop yield [15]. Slow-release fertilizer can regulate N release rate to meet N demand of crops during the whole growth period. This property leads to a high N harvest index of N fertilizer and a high N uptake of plants [16,17]. Study of Xing et al. [18] showed that the combination of slow-release N fertilizer and moderate N fertilizer application rate have significant effects on improving the N uptake, N translocation, and N translocation efficiency. However, the effects of slow-release fertilizer on plant nitrogen metabolism are not well studied. Phosphorus is also an essential mineral nutrient element for plants [19]. Coating granular fertilizers with substances to slow the initial dissolution is one of the effective methods attempted to extend P release through a growing season and increase user efficiency [20]. Tyliszczak et al. [21] studied the release of phosphorus in the slow-release fertilizer and found that it was related to the water absorption kinetics of the slow-release fertilizer. According to the research of Chuan et al. [22], the mean yield response of wheat in China to nitrogen and phosphorus were 1.7 and 1.0 t/ha, respectively, nitrogen was the nutrient most limiting yield, followed by phosphorus. Therefore, in this study, synergistic fertilizers were developed based on ordinary fertilizer, thereby extending the release time and improving the use efficiency of the fertilizers and the effects of synergistic fertilizer on nitrogen metabolism was studied in depth. Maleic acid and itaconic acid were copolymerized to make a fluid gel, which was sprayed on the nitrogen and phosphorus fertilizer surface to make nitrogen synergistic and phosphorus synergistic fertilizer. Different nitrogen and phosphorus fertilizer ratios were set, combined with the nitrogen concentration level of each part of the plant, the effects of different nitrogen and phosphorus synergist fertilizer ratios on nitrogen metabolism related enzymes (nitrogen assimilation related enzyme activity) and genes (nitrogen transporter genes and assimilation enzyme genes expression) of wheat were studied to provide basis for its application.
Plants of winter wheat cultivar YanNong 999 were grown in soil column.
Common nitrogen fertilizer (CNF, urea, 46% N), common phosphate fertilizer (CPF, superphosphate, 12% P2O5), common potassium fertilizer (CKF, potassium sulfate, 50% K2O), commercial nitrogen synergistic fertilizer (CNSF, slow-release urea, 46% N), and commercial phosphate synergistic fertilizer (CPSF, slow-release calcium superphosphate, 12% P2O5) were used as purchased.
Itaconic acid, maleic acid, potassium persulfate and acrylic acid were purchased from Aladdin Biochemical Technology Co., Ltd. (Shanghai, China) to make a synergistic agent.
According to the method of Chen et al. [23], the purchased urea and superphosphate were prepared to form nitrogen synergistic fertilizer (NSF) and phosphorus synergistic fertilizer (PSF) fertilizer with the synergistic agent, respectively.
The experiment was conducted under field conditions at the experimental base of Qingdao Agricultural University (37°48′10″N, 119°54′33″E), Shandong Province, China, from October 2018 to June 2019. The climate type of the experiment site is Monsoon Climate of Medium Latitudes. During the wheat growth period, the precipitation is about 300 mm, the sunshine duration is 1400~2000 h, and the accumulated temperature above 0°C is 2000°C~2200°C, which is suitable for the growth of wheat.
2.3 Preparation of Soil Column
PVC pipes (15 cm in diameter, 160 cm in height) were placed after the soil was dug out according to its layers. The excavated soil was then evenly mixed and transferred into the pipes from deep to shallow, with stones, wood blocks and other sundries screening out.
The basic fertility characteristics of the topsoil are given in Table 1.
A randomized complete block design was adopted. Six treatments were set up, with 10 replicates per treatment and 4 plants per column. The respective treatments were:
1. Control (CK): 100% CNF+100% CPF;
2. Treatment 1 (T1): 70% NSF+100% PSF;
3. Treatment 2 (T2): 100% NSF+70% PSF;
4. Treatment 3 (T3): 100% NSF+100% PSF;
5. Treatment 4 (T4): 70% NSF+70% PSF;
6. Treatment 5 (T5): 100% CNSF+100% CPSF.
Potassium fertilizer was applied to all treatments, and all fertilizers were applied evenly into 5–35 cm soil layers before sowing as base fertilizer, no dressing was conducted. The specific fertilization amount was determined according to the basic soil fertility. The nitrogen, phosphorus and potassium contents of the fertilizers in each treatment were shown in Table 2.
Wheat was sown on October 11, 2018, blooming on May 07, 2019, and harvested on June 17, 2019. Seven seeds were sown in each column, and the seedlings were thinned after the emergence to ensure that four plants were obtained in per column, and the sowing depth was 5 cm. No irrigation was conducted during wheat growth. Chemical agents were sprayed before jointing of wheat to control diseases, pests and weeds.
Within 30 days after wheat flowering, 9 aboveground plants of wheat were randomly selected every 10 days from each treatment, separated into stems, leaves and ears, dried at 75°C after inactivating the enzyme at 105°C and then crashed for nitrogen concentration determination.
Another 9 intact plants were taken from each treatment at the same time. The flag leaf and roots (washed with running water) were respectively removed and placed in liquid nitrogen for the determination of enzyme activity and gene expression.
2.6.1 Plant Nitrogen Concentration
Kjeldahl method was used to determine the total nitrogen concentration of each part [24].
2.6.2 Enzyme Activity Determination
Nitrate reductase (NR) and glutamine synthetase (GS) activities were determined according to the method of Balotf et al. [25]. One unit of NR was defined as the amount of enzyme required for production of 1 µmol nitrite per hour. The NR activity was expressed as NR units per gram of fresh weight. One unit of GS was the amount of enzyme catalysing the formation of 1 µmol of glutamylmonohydroxamate per minute. GS activity expressed as GS units per milligram of fresh weight.
2.6.3 RNA Extraction and Real-Time PCR
Total RNA was extracted using RNA-plus buffer (Takara Bio, Japan). The concentration of purified total RNA was determined using a Nano-drop (ND) 1000 spectrophotometer. Agarose gel electrophoresis was used to determine the integrity of total RNA before the synthesis of the first-strand cDNA. The Evo M-MLV RT Kit with gDNA Clean for qPCR (Accurate Bio, China) was used to remove residual DNA and synthetic cDNA.
The determined gene name and corresponding primer sequence was shown in Table 3. SYBR Green Premix Pro Taq HS qPCR Kit (Accurate Bio, China) was used for fluorescent quantitative PCR reaction. A total of 40 cycles was performed, and the cycle parameters were: 95°C, 30 s; 95°C, 10 s; 60°C, 30 s. The relative gene expression was calculated by 2−ΔΔCT (CT: the number of cycles required when the fluorescence intensity reaches the threshold) method [26], with the ADP-RF gene as the internal reference. The determination included three biological repetitions and three technical repetitions.
Data processing and chart drawing were performed in Excel 2019 and Sigmaplot14.0 (SYSTAT Software Company, California, USA), the statistical analysis and the significance test of difference were calculated by IBM SPSS Statistics. Two-way analysis of variance (ANOVA) was performed. At the probability level of 0.05, the mean values of different treatments were compared using the least significant difference (LSD). And the marked letter method was used to indicate differences between treatments, with different letters representing significant differences.
3.1 Effects of Different Nitrogen and Phosphorus Synergistic Fertilizer on Total Nitrogen Concentration of Wheat Plants
The results of variance analysis showed that post-flowering time and different nitrogen fertilizer treatments had significant effects on the total nitrogen concentration of wheat stems, leaves and ears, but their interaction was not significant. It can be seen from Fig. 1 that within 0–30 days after anthesis, with the advance of growth process, the total nitrogen concentration of wheat stems and leaves showed a trend of slow to rapidly decline, while the total nitrogen concentration of wheat ears gradually increased. The nitrogen concentration in leaves and ears of wheat expressed as T3 > T2 > T5 and T1 > CK > T4 (p < 0.05), and in stems it expressed as T1, T2, T3 and T5 > CK and T4 (p < 0.05). The nitrogen concentration finally in leaves, stems and ears of wheat under T3 treatment was 11.17%, 12.35% and 7.28% higher than those under CK, respectively. The nitrogen concentrations finally in leaves of wheat under T2, T5 and T1 treatment were 5.79%, 5.54% and 1.65% higher than those under CK, respectively. As for nitrogen concentration of stem, these ratios were 8.67%, 6.18% and 3.92%. And for nitrogen concentration of the ear, these ratios were 5.88%, 2.10% and 0.38%, respectively. From 0 to 20 days after anthesis, nitrogen concentration in leaves and ears of CK was significantly higher than that of T4, but the difference was not significant in stems. The nitrogen concentration finally in leaves and ears of wheat under T4 treatment was 1.99% and 0.11% lower than those under CK.
Figure 1: Effects of different nitrogen and phosphorus synergistic fertilizer on total nitrogen concentration
3.2 Effects of Different Nitrogen and Phosphorus Synergistic Fertilizer on the Expression of Nitrogen Transporter Genes in Wheat Roots
As shown in Fig. 2, the expression of TaNRT1.1 under T3 treatment was up-regulated with the development process, while it in other treatments had no significant change in the dimension of time. According to the significance among the six treatments, the expression of TaNRT1.1 was basically expressed as T3 > T2/T5 > T1/T4/CK. The expression of TaNRT2.1 under T3 and T5 treatments had no significant change with the development process, while it was up-regulated in other treatments. For the order among treatments, T3 was significantly higher than T2, followed by T1 and T5. There was no significant difference between CK and T1, but in the early stage T5 was significantly higher than CK, and for most of the time there was no significant difference between T4 and CK. Compared with TaNRT1.1, TaNRT2.1 showed little difference among treatments. The expression of TaNRT2.3 was basically up-regulated with the development process under each treatment and it also varied considerably among treatments (basically expressed as T3 > T2 > T1 > CK/T5 > T4). And as for the expression of TaAMT, it had no significant change with the development process under T2 treatment, while it was up-regulated in other treatments. The order it under the six treatments was T3 > T5 > CK/T1/T2 > T4. It can be seen that the expression level of these four genes in T3 treatment was significantly higher than that in other treatments in the same period. Within 0–30 days after anthesis, the expression of TaNRT1.1 in T3 was up-regulated by 0.63 times; the expression of TaNRT2.1 in CK, T1, T2 and T3 was up-regulated by 2.60, 2.57, 0.60 and 2.00 times; the expression of TaNRT2.3 in CK, T1, T2, T3, T4 and T5 was up-regulated by 3.70, 1.48, 0.54, 0.74, 2.08 and 2.97 times, respectively; and the expression of TaAMT in CK, T1, T3, T4 and T5 was up-regulated by 0.45, 0.40, 0.35, 3.79 and 0.25 times.
Figure 2: Effects of different nitrogen and phosphorus synergistic fertilizer on the expression of nitrogen transporter genes in wheat roots. Means denoted by different letters above the standard deviation bars of the same position indicate significant differences according to LSD test (p < 0.05). Lowercase letters represent the differences between different treatments in the same period, and capital letters represent the differences in different periods of the same treatment
3.3 Effects of Different Nitrogen and Phosphorus Synergistic Fertilizer on Nitrate Reductase (NR) and Glutamine Synthase (GS) Enzyme
It can be seen from Fig. 3 that NR activity in flag leaves and roots of wheat showed a downward trend after anthesis. In all treatments, NR activity in flag leaves basically expressed as T3 > T2 > T5 > T1 > CK > T4. Except that there was no significant difference between T2 and T3 at 7 days after flowering, NR activity in flag leaves under T3 was significantly higher than under T2. In roots, NR activity basically expressed as T3 > T2 > T5 > T1/CK > T4. During the whole flowering period, NR activity in leaves of CK, T1, T2, T3, T4 and T5 treatment decreased by 86.36%, 84.14%, 76.50%, 71.91%, 87.44% and 79.64%, respectively, and in roots decreased by 57.71%, 55.02%, 47.58%, 48.99%, 58.37% and 51.20%, respectively. Both in leaves and roots, the NR activity under T3 treatment and T2 treatment remains at a high level, and the activity decreased more slowly, then in T5 treatment. The decreasing rate of NR activity in leaves was higher than that in roots, and the difference among treatments was smaller in roots.
Figure 3: Effect of different nitrogen and phosphorus synergistic fertilizer on NR activity. Means denoted by different letters above the standard deviation bars of the same position indicate significant differences according to LSD test (p < 0.05). Lowercase letters represent the differences between different treatments in the same period, and capital letters represent the differences in different periods of the same treatment
As for GS activity, it can be seen from Fig. 4 that after anthesis, GS activity of flag leaves in wheat showed a trend of rapid decline, then a steady decline, but showed a steady decline trend in roots. GS activity in flag leaves under different treatments expressed as T3 > T2 > T5 > T1/CK > T4, and in roots expressed as T3 > T2 > T5 > T1 > CK/T4. Within 0–30 days after anthesis, GS activity in leaves of CK, T1, T2, T3, T4 and T5 treatment decreased by 74.37%, 72.20%, 59.72%, 60.09%, 77.20% and 64.90%, respectively, while in roots decreased by 71.66%, 69.43%, 65.20%, 59.20%, 74.03% and 68.37%. During the whole flowering period, GS activity decreased faster in leaves than in roots and there was a smaller difference among these six treatments in roots.
Figure 4: Effect of different nitrogen and phosphorus synergistic fertilizer on GS activity. Means denoted by different letters above the standard deviation bars of the same position indicate significant differences according to LSD test (p < 0.05). Lowercase letters represent the differences between different treatments in the same period, and capital letters represent the differences in different periods of the same treatment
For the nitrate reductase gene TaNR, the change of its expression (Fig. 5) was opposite to the activity of the enzyme, and it was up-regulated with the flowering time under each treatment. At the same period, in T3 treatment, its expression was higher than in other treatment, and reached the peak at 30 days after anthesis, which was up-regulated by 4 times compared with CK. According to the significance among the six treatments, the expression of TaNR was basically expressed as T3 > T2 and T5 > T1 > T4 and CK. At the early stage, it was significantly higher in T5 treatment than in T1 treatment, but there was no significant difference between T1 and T5 treatment at 20 days after anthesis, and at 30 days after anthesis, it was significantly higher in T1 treatment than in T5 treatment. Within 0–30 days after anthesis, the expression of TaNR under CK, T1, T2, T3, T4 and T5 treatment were up-regulated by 3.17, 4.27, 1.06, 3.42, 0.08 and 1.07 times, respectively. Similarly, the expression of glutamine synthase gene TaGS after anthesis (Fig. 6) was higher under T3 treatment than under other treatments, and was quite the opposite in T4 treatment. The expression of TaGS was basically expressed as T3 > CK/T1/T2/T5 > T4. Within 0–30 days after anthesis, the expression of TaGS in T1, T2 and T3 treatment was up-regulated by 0.79, 0.64 and 1.12 times, while in CK, T4 and T5 treatment, it had no significant change with the development process.
Figure 5: Effect of different nitrogen and phosphorus synergistic fertilizer on the expression of TaNR gene. Means denoted by different letters above the standard deviation bars of the same position indicate significant differences according to LSD test (p < 0.05). Lowercase letters represent the differences between different treatments in the same period, and capital letters represent the differences in different periods of the same treatment
Figure 6: Effect of different nitrogen and phosphorus synergistic fertilizer on the expression of TaGS gene. Means denoted by different letters above the error bars of the same position indicate significant differences according to LSD test (p < 0.05). Means denoted by different letters above the standard deviation bars of the same position indicate significant differences according to LSD test (p < 0.05). Lowercase letters represent the differences between different treatments in the same period, and capital letters represent the differences in different periods of the same treatment
One of the most critical stages for cereal crop production is grain filling after anthesis. In wheat, a portion of the grain nitrogen is contributed by post-flowering uptake, which contributes to grain protein deposition, and most of the rest is translocated from the vegetative parts of the plant [29]. As the growth period increased, the total nitrogen concentration of wheat stems and leaves decreased consistently, while the total nitrogen concentration of wheat ears gradually increased, which was in line with the normal growth and development. In this study, the application of synergistic fertilizer had a positive effect on the nitrogen concentration of wheat. Under the same application rate of synergistic fertilizer (T3), the concentration of nitrogen in wheat leaves, stems and ears could be significantly increased. It can still provide sufficient nutrients for the normal growth of wheat even in the case of reducing 30% amount of nitrogen synergistic fertilizer (T1) or phosphorus synergistic fertilizer (T2). As another contrast, the nitrogen concentration in wheat treated with commercial synergistic fertilizer (T5) was lower than that of synergistic fertilizer, but still higher than that of common fertilizer. This may be due to the encapsulation mechanism of the synergistic fertilizer used in the experiment. Encapsulation allows for higher urea efficiency, synchronization with plants ongoing nutrient needs [6], which means less nitrogen is lost to the environment and more is taken up and used by the plant. In addition, Chen’s study [23] of synergistic fertilizers used in this experiment have shown that the contents of total nitrogen, alkali-hydrolyzed nitrogen, available phosphorus, and available potassium in soil were higher after the treatment of synergistic fertilizers than those after the treatment of ordinary slow-release fertilizers available in the market. Ample availability of soil N during grain filling favors post-anthesis N uptake [30]. And the synergistic effects of nitrogen, phosphorus and potassium are also beneficial to the utilization of nitrogen by post-anthesis wheat plants. However, the nutrient supply was not as good as conventional fertilization when the nitrogen synergistic fertilizer and phosphorus synergistic fertilizer were reduced at the same time (T4).
The nitrogen concentration of wheat plants showed the positive effect of synergistic fertilizer on nitrogen uptake and utilization of wheat. In order to explore the specific synergistic mechanism and visualize this positive effect from nitrogen absorption, transport and metabolism process, the changes in gene expression of nitrogen transporter and assimilating enzyme in wheat were detected.
Plants mainly absorb nitrogen in the form of ammonium (NH4+) and nitrate (NO3−) from the soil. Nitrate is mainly transported through members of the NRT families of NO3− transporters. The two major nitrate transporter gene families are the low-affinity NRT1 (recently named NPF) and the high-affinity NRT2 [31]. The most extensively studied NRT gene is NRT1.1 (NPF6.3). This gene is NO3− inducible and encodes a dual affinity transporter with both high affinity system and low affinity system activity [32]. In wheat, four NPF6.3 genes homologous to the sole Arabidopsis NPF gene have been identified, and shown that they have distinct tissue specific and transcriptional responses to nitrogen supply [33]. TaNRT2.1 is mainly expressed in wheat roots and may play a major role in NO3− uptake after anthesis [34]. In recent years, an increasing number of studies have shown that overexpression of NPF and NRT2 genes can significantly improve the yield, shoot biomass or NUE of crops [35,36]. In this study, whether compared with common fertilizer or commercial synergistic fertilizer, the expression level of TaNRT1.1, TaNRT2.1 and TaNRT2.3 were higher under the condition of the same amount of nitrogen synergistic and phosphorus synergistic fertilizer, and the upward revision of TaNRT1.1 within 0–30 days after anthesis was even larger. The reason for the high expression of these genes is the continuous release of nutrients, which leads to higher nitrogen levels in the soil. However, even if synergistic fertilizers provided a sustainable nutrient supply, their effect on nutrient utilization was limited when the total nitrogen applied was relatively reduced. This can be seen in the expression of these three genes under the condition of reduced synergistic fertilization.
Similar to the NRT family, the ammonium transporter (AMT) genes are divided into AMT1 and AMT2 according to the affinity level. But due to the low concentration of NH4+ in soil, NH4+ uptake mainly uses the high affinity transport system [37]. In this study, TaAMT1.1 expression also reached the highest level under the condition of 100% nitrogen synergistic fertilizer and 100% phosphorus synergistic fertilizer. Different from NRT genes, the expression level of it under commercial fertilizer was significantly higher than that of common fertilizer, but still lower than that of nitrogen synergistic fertilizer and phosphorus synergistic fertilizer, which was consistent with the results of wheat nitrogen concentration, indicating that synergistic fertilizer studied in this experiment had a better effect on improving nitrogen utilization efficiency.
In the cytosol, nitrate reduction into nitrite by the enzyme NR, which is transferred to the chloroplast, and reduced to ammonium by nitrite reductase (NIR). As a key enzyme, nitrate reductase plays an important role in the plant nitrogen uptake and assimilation [38]. Assimilation of NH4+ into glutamine and glutamate also takes place in the plastid/chloroplast through GS/glutamate synthase (GOGAT) system of reactions [39]. This pathway is of crucial importance since the glutamine and glutamate produced are donors for the biosynthesis of the major N-containing compounds [40] and GS is an important rate-limiting enzyme in NH4+ assimilation. In this study, NR and GS activities of flag leaves and roots in wheat decreased with the growth period, which could be for two reasons: (1) the vegetative organs such as flag leaf and root gradually senescence with the advance of the growth period; (2) nitrogen was remobilized from senescing leaves to seeds at the reproductive stage [13]. This was also the reason why the decrease in NR and GS activity in leaves was higher than that in roots. Higher NR and GS activities were observed in the plants under synergistic fertilization (except simultaneous reduction of nitrogen synergistic fertilizer and phosphorus synergistic fertilizer), and the reduction of the two enzyme activities was also relatively lower. Studies have shown that plants NR and GS activities were increased with an increase in nitrogen supply [41]. Therefore, the higher activities and lower activity reductions of NR and GS under the application of synergistic fertilizer could be inferred to be due to the continuous nitrogen supply by synergistic fertilizer after anthesis, while the lower activities of NR and GS were observed when the total amount of nitrogen applied was reduced. To investigate whether the changes of NR and GS activities were closely related to differential expression of plant NR and GS genes, the expression levels of NR gene and GS gene were quantified. qPCR analysis indicated that the expression levels of TaNR and TaGS were significantly higher in wheat plants under the condition of 100% nitrogen synergistic fertilizer and 100% phosphorus synergistic fertilizer.
In this study, the fluid gel formed by the polymerization of maleic acid, itaconic acid, acrylic acid and potassium persulfate was used to coat urea and superphosphate particles to make nitrogen synergistic fertilizer and phosphorus synergistic fertilizer relatively. The results of the experiment in the current study clearly showed that the simultaneous application of nitrogen synergistic fertilizer and phosphorus synergistic fertilizer had significant effects on improving nitrogen metabolism of wheat plants, including inducing the expression of nitrogen transport genes, up-regulating the expression of TaNR and TaGS and enhancing the activity of key enzymes (NR and GS) of nitrogen assimilation, which was finally manifested by higher nitrogen concentration in the plants. Like most coated fertilizers on the market, it takes a certain amount of time for the synergistic fertilizer to be released, resulting in higher nutrient levels in the soil after anthesis, which favors wheat nitrogen metabolism. And compared with commercial synergistic fertilizer in the market, the effect of synergistic fertilizers is better. However, the effect of synergistic fertilizer cannot compensate for the lack of insufficient nutrients when the application amount of nitrogen and phosphorus were reduced simultaneously.
Acknowledgement: The authors thank the College of Agronomy, Qingdao Agricultural University for its support during the completion of this study.
Funding Statement: This work was supported by the Program of Shandong Modern Agricultural Technology and Industry System–Cultivation and Soil Fertilizer (SDAIT0107) and the Major Application Technology Innovation Projects in Shandong Province and the Key Research and Development Projects of Shandong Province.
Author Contributions: The authors confirm contribution to the paper as follows: study conception and design: Yan Shi; data collection: Yajun Li, Yihui Wang, Shuang Chen, Yu Gao; analysis and interpretation of results: Yajun Li, Yihui Wang; draft manuscript preparation: Yajun Li, Yihui Wang. All authors reviewed the results and approved the final version of the manuscript.
Conflicts of Interest: The authors declare that they have no conflicts of interest to report regarding the present study.
References
1. Fischer, R., Byerlee, D., Edmeades, G. O. (2014). Crop yields and global food security: Will yield increase continue to feed the world? Canberra: Australian Centre for International Agricultural Research. [Google Scholar]
2. Duan, J., Shao, Y., He, L., Li, X., Hou, G. et al. (2019). Optimizing nitrogen management to achieve high yield, high nitrogen efficiency and low nitrogen emission in winter wheat. Science of the Total Environment, 697, 134088. [Google Scholar] [PubMed]
3. Ma, G., Liu, W., Li, S., Zhang, P., Wang, C. et al. (2019). Determining the optimal N input to improve grain yield and quality in winter wheat with reduced apparent N loss in the North China Plain. Frontiers in Plant Science, 10, 181. [Google Scholar] [PubMed]
4. Zhang, X., Davidson, E. A., Mauzerall, D. L., Searchinger, T. D., Dumas, P. et al. (2015). Managing nitrogen for sustainable development. Nature, 528(7580), 51–59. [Google Scholar] [PubMed]
5. Gruber, N., Galloway, J. N. J. (2008). An Earth-system perspective of the global nitrogen cycle. Nature, 451(7176), 293–296. [Google Scholar] [PubMed]
6. Beig, B., Niazi, M. B. K., Jahan, Z., Hussain, A., Zia, M. H. et al. (2020). Coating materials for slow release of nitrogen from urea fertilizer: A review. Journal of Plant Nutrition, 43(10), 1510–1533. [Google Scholar]
7. Fu, J., Wang, C., Chen, X., Huang, Z., Chen, D. (2018). Classification research and types of slow controlled release fertilizers (SRFs) used–A review. Communications in Soil Science and Plant Analysis, 49(17), 2219–2230. [Google Scholar]
8. Cheng, D., Liu, Y., Yang, G., Zhang, A. (2018). Water- and fertilizer-integrated hydrogel derived from the polymerization of acrylic acid and urea as a slow-release N fertilizer and water retention in agriculture. Journal of Agricultural and Food Chemistry, 66(23), 5762–5769. [Google Scholar] [PubMed]
9. Salimi, M., Motamedi, E., Motesharezedeh, B., Hosseini, H. M., Alikhani, H. A. (2020). Starch-g-poly(acrylic acid-co-acrylamide) composites reinforced with natural char nanoparticles toward environmentally benign slow-release urea fertilizers. Journal of Environmental Chemical Engineering, 8(3), 103765. [Google Scholar]
10. Teodorescu, M., Lungu, A., Stanescu, P. O. (2008). Preparation and properties of novel slow-release NPK agrochemical formulations based on poly(acrylic acid) hydrogels and liquid fertilizers. Industrial and Engineering Chemistry Research, 48(14), 6527–6534. [Google Scholar]
11. Zhao, C., Cui, Z., Zhao, X., Zhang, J., Zhang, L. et al. (2019). Enhanced itaconic acid production in Yarrowia lipolytica via heterologous expression of a mitochondrial transporter MTT. Applied Microbiology and Biotechnology, 103(5), 2181–2192. [Google Scholar] [PubMed]
12. Zhao, C., Zhang, J., Yue, Q., Zhao, L., Ma, H. et al. (2022). Itaconic acid–urea–acrylic acid copolymer as a novel water and nutrient retaining fertilizer. Environmental Technology and Innovation, 25, 102140. [Google Scholar]
13. Masclaux-Daubresse, C., Daniel-Vedele, F., Dechorgnat, J., Chardon, F., Gaufichon, L. et al. (2010). Nitrogen uptake, assimilation and remobilization in plants: Challenges for sustainable and productive agriculture. Annals of Botany, 105(7), 1141–1157. [Google Scholar] [PubMed]
14. Islam, S., Zhang, J., Zhao, Y., She, M., Ma, W. (2021). Genetic regulation of the traits contributing to wheat nitrogen use efficiency. Plant Science, 303, 110759. [Google Scholar] [PubMed]
15. Ghafoor, I., Habib-ur-Rahman, M., Ali, M., Afzal, M., Ahmed, W. et al. (2021). Slow-release nitrogen fertilizers enhance growth, yield, NUE in wheat crop and reduce nitrogen losses under an arid environment. Environmental Science and Pollution Research, 28(32), 43528–43543. [Google Scholar] [PubMed]
16. Andrade, A. B., Guelfi, D. R., Chagas, W. F. T., Cancellier, E. L., de Souza, T. L. et al. (2021). Fertilizing maize croppings with blends of slow/controlled-release and conventional nitrogen fertilizers. Journal of Plant Nutrition and Soil Science, 184(2), 227–237. [Google Scholar]
17. Timilsena, Y. P., Adhikari, R., Casey, P., Muster, T., Gill, H. et al. (2015). Enhanced efficiency fertilisers: A review of formulation and nutrient release patterns. Journal of the Science of Food and Agriculture, 95, 1131–1142. [Google Scholar] [PubMed]
18. Xing, Y., Mi, F., Wang, X. (2022). Effects of different nitrogen fertilizer types and application rates on maize yield and nitrogen use efficiency in Loess Plateau of China. Journal of Soils and Sediments, 22, 1938–1958. [Google Scholar]
19. Raghothama, K. (2000). Phosphate transport and signaling. Current Opinion in Plant Biology, 3(3), 182–187. [Google Scholar] [PubMed]
20. Weeks Jr, J. J., Hettiarachchi, G. M. (2019). A review of the latest in phosphorus fertilizer technology: Possibilities and pragmatism. Journal of Environmental Quality, 48, 1300–1313. [Google Scholar] [PubMed]
21. Tyliszczak, B., Polaczek, J., Pielichowski, J., Pielichowski, K. (2009). Preparation and properties of biodegradable slow-release PAA superabsorbent matrixes for phosphorus fertilizers. Macromolecular Symposia, 279(1), 236–242. [Google Scholar]
22. Chuan, L., He, P., Pampolino, M. F., Johnston, A. M., Jin, J. et al. (2013). Establishing a scientific basis for fertilizer recommendations for wheat in China: Yield response and agronomic efficiency. Field Crops Research, 140, 1–8. [Google Scholar]
23. Chen, S., Wang, Y., Shi, Y. (2022). Synergistic fertilizers improved chemical properties of soil in wheat (Triticum aestivum L.) field. Communications in Soil Science and Plant Analysis, 54(1), 1–17. [Google Scholar]
24. Kjeldahl, J. (1883). Neue methode zur bestimmung des stickstoffs in organischen körpern. Zeitschrift für Analytische Chemie, 22(1), 366–382. [Google Scholar]
25. Balotf, S., Kavoosi, G., Kholdebarin, B. (2016). Nitrate reductase, nitrite reductase, glutamine synthetase, and glutamate synthase expression and activity in response to different nitrogen sources in nitrogen-starved wheat seedlings. Biotechnology and Applied Biochemistry, 63(2), 220–229. [Google Scholar] [PubMed]
26. Livak, K. J., Schmittgen, T. D. (2001). Analysis of relative gene expression data using real-time quantitative PCR and the 2−ΔΔCT method. Methods, 25(4), 402–408. [Google Scholar] [PubMed]
27. Li, Y., Li, Y., Zhang, H., Wang, M., Chen, S. (2019). Diazotrophic Paenibacillus beijingensis BJ-18 provides nitrogen for plant and promotes plant growth, nitrogen uptake and metabolism. Frontiers in Microbiology, 10, 1119. [Google Scholar] [PubMed]
28. Paolacci, A. R., Tanzarella, O. A., Porceddu, E., Ciaffi, M. (2009). Identification and validation of reference genes for quantitative RT-PCR normalization in wheat. BMC Molecular Biology, 10(1), 1–27. [Google Scholar]
29. Hirel, B., Le Gouis, J., Ney, B., Gallais, A. (2007). The challenge of improving nitrogen use efficiency in crop plants: Towards a more central role for genetic variability and quantitative genetics within integrated approaches. Journal of Experimental Botany, 58(9), 2369–2387. [Google Scholar] [PubMed]
30. Madani, A., Makarem, A., Vazin, F., Joudi, M. (2012). The impact of post-anthesis nitrogen and water availability on yield formation of winter wheat. Plant, Soil and Environment, 58, 9–14. [Google Scholar]
31. Tsay, Y. F., Chiu, C. C., Tsai, C. B., Ho, C. H., Hsu, P. K. (2007). Nitrate transporters and peptide transporters. FEBS Letters, 581(12), 2290–2300. [Google Scholar] [PubMed]
32. Parker, J. L., Newstead, S. (2014). Molecular basis of nitrate uptake by the plant nitrate transporter NRT1.1. Nature, 507(7490), 68–72. [Google Scholar] [PubMed]
33. Buchner, P., Hawkesford, M. J. (2014). Complex phylogeny and gene expression patterns of members of the NITRATE TRANSPORTER 1/PEPTIDE TRANSPORTER family (NPF) in wheat. Journal of Experimental Botany, 65(19), 5697–5710. [Google Scholar] [PubMed]
34. Taulemesse, F., Le Gouis, J., Gouache, D., Gibon, Y., Allard, V. (2015). Post-flowering nitrate uptake in wheat is controlled by N status at flowering, with a putative major role of root nitrate transporter NRT2.1. PLoS One, 10(3), e0120291. [Google Scholar] [PubMed]
35. Hu, B., Wang, W., Ou, S., Tang, J., Li, H. et al. (2015). Variation in NRT1.1B contributes to nitrate-use divergence between rice subspecies. Nature Genetics, 47(7), 834–838. [Google Scholar] [PubMed]
36. Fan, X., Tang, Z., Tan, Y., Zhang, Y., Luo, B. et al. (2016). Overexpression of a pH-sensitive nitrate transporter in rice increases crop yields. Proceedings of the National Academy of Sciences of the United States of America, 113(26), 7118–7123. [Google Scholar] [PubMed]
37. Bajgain, P., Russell, B., Mohammadi, M. (2018). Phylogenetic analyses and in-seedling expression of ammonium and nitrate transporters in wheat. Scientific Reports, 8(1), 1–13. [Google Scholar]
38. Chen, H., Huang, L. (2020). Effect of nitrogen fertilizer application rate on nitrate reductase activity in maize. Applied Ecology and Environmental Research, 18, 2879–2894. [Google Scholar]
39. Suzuki, A., Knaff, D. B. (2005). Glutamate synthase: Structural, mechanistic and regulatory properties, and role in the amino acid metabolism. Photosynthesis Research, 83(2), 191–217. [Google Scholar] [PubMed]
40. Ireland, R. J., Lea, P. J. (1999). The enzymes of glutamine, glutamate, asparagine and aspartate metabolisms. In: Singh, B. K. (Ed.Plant amino acids: Biochemistry and biotechnology, pp. 49–109. New York: Marcel Dekker. [Google Scholar]
41. Iqbal, A., Dong, Q., Wang, X., Gui, H., Zhang, H. et al. (2020). Variations in nitrogen metabolism are closely linked with nitrogen uptake and utilization efficiency in cotton genotypes under various nitrogen supplies. Plants, 9, 250. [Google Scholar] [PubMed]
Cite This Article
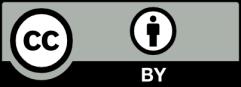
This work is licensed under a Creative Commons Attribution 4.0 International License , which permits unrestricted use, distribution, and reproduction in any medium, provided the original work is properly cited.