Open Access
ARTICLE
Insecticidal Potential of α-Pinene and β-Caryophyllene against Myzus persicae and Their Impacts on Gene Expression
1 Institute of Molecular Biology and Biotechnology (IMBB), The University of Lahore, Lahore, 54000, Pakistan
2 Institute of Pharmaceutical Sciences, University of Veterinary and Animal Sciences, Lahore, 54000, Pakistan
3 Deparment of Plant Pathology, University of Okara, Okara, 56300, Pakistan
4 Deparment of Bioinformatics, University of Okara, Okara, 56300, Pakistan
5 Department of Soil Science, The Islamia University of Bahawalpur, Bahawalpur, 63100, Pakistan
6 Department of Zoology, The Islamia University of Bahawalpur, Bahawalpur (Bahawalnagar Campus), 63100, Pakistan
7 The Steve and Cindy Rasmussen Institute for Genomic Medicine, Nationwide Children’s Hospital, Columbus, Ohio, 43205, USA
8 Department of Botany and Microbiology, College of Science, King Saud University, Riyadh, 11451, Saudi Arabia
* Corresponding Authors: Talha Ali Chohan. Email: ; Tahir Ali Chohan. Email:
Phyton-International Journal of Experimental Botany 2023, 92(7), 1943-1954. https://doi.org/10.32604/phyton.2023.026945
Received 05 October 2022; Accepted 22 February 2023; Issue published 29 May 2023
Abstract
Myzus persicae (M. persicae) is now considered a threat to agricultural crops due to economic losses. Numerous synthetic insecticides applied every year against M. persicae, are reported to be unsafe for environment, humans, and beneficial insects. Furthermore, several species of Myzus have been found to develop resistance due to over application of these insecticides. Therefore, it is required to find some novel insecticide that would be safe for the environment as well as for humans. In the current study, two major pure constituents α-pinene and β-caryophyllene were evaluated for their insecticidal potential against M. persicae using a fumigant toxicity assay. Furthermore, impact of α-pinene and β-caryophyllene on expression of five different genes, e.g., HSP 60, FPPS I, OSD, TOL and ANT responsible for reproduction, dispersion, and growth of M. persicae has also been investigated. To perform fumigant toxicity assay, five different concentrations (3.5, 4, 4.5, 5 and 6 μL L−1) of α-pinene and β-caryophyllene were prepared. Lethal concentration (LC) was calculated, and gene expression studies were executed through qRT PCR at LC30 of α-pinene and β-caryophyllene. Both constituents demonstrated excellent fumigant toxicity effects against M. persicae at all five concentrations. However, α-pinene shows significantly better results (98%) as compared to β-caryophyllene (80%) after 72 h at 6 μL L−1 of dose. The highest upregulation in expression was demonstrated at LC30 dose of α-pinene in five in three out of five genes understudy (TOL, ANT, and FPPS I). Conversely, two genes HSP 60 and OSD demonstrated downregulation at LC30 dose of β-caryophyllene. Conclusively, our results highlighted the promising insecticidal potential of both compounds α-pinene and β-caryophylleneby interfering with the reproduction and development related processes in M. persicae, allowing us to recommend the phytoconstituents under investigation as an ecofriendly alternative to synthetic insecticides.Keywords
Pests cause nearly 20%–50% of damage in agricultural crop production [1,2]. Among them, the aphid (Myzus persicae) is the most disastrous pest, categorized into more than 4300 species [3,4]. M. persicae usually causes harm in three ways; directly feeding on a plant, persisting as a vector by transmitting viral pathogen in the plant, or secreting honeydews, which helps several secondary pathogens to spread on the surface of the plant [5]. Due to different ways of damaging ability, M. persicae has become a significant crop hazard, triggering millions of dollars in losses yearly in the agricultural sector [6]. Several insecticides belonging to different classes are being utilized to control M. persicae through their multiple application on crops [7]. However extensive usage of these insecticides causes ecological contamination, pesticide remnants in food, and adverse effects on beneficial insects, organisms, and humans. Moreover, M. persicae can develop resistance against insecticides due to its high reproduction rate [8,9]. Owing to the current situation, there is a dire need to adopt eco-friendly practices to control M. persicae effectively.
Plant essential oils (EOs), a combination of bioactive volatile constituents, are regarded as one of the most important aspects of botanical insecticides due to their safety against the environment, non-target organisms, and low-level resistance [10,11]. Nowadays, essential oils and their active components are emerging as a substitute for insect control with no harmful effects on mammals and the environment [12,13]. Active constituents from the essential oil, such as Asteraceae and Foeniculum vulgare have demonstrated promising insecticidal activity against M. persicae [14,15]. Similarly, numerous studies have shown that essential oil-based secondary plant metabolites may be a worthy substitute for synthetic insecticides in controlling M. persicae [16–18].
Generally, essential oils are the amalgam of versatile chemical constituents such as terpenoids, terpenes, oxygenated terpenes, sesquiterpenes, phenylpropanoids, alcohols, esters, ketones, etc. [19]. Terpenes are plant-derivative chemicals with potent insect antifeedant and toxic properties to herbivorous insects [20]. α-pinene, a bicyclic terpene abundantly occurring in the Rosemainus officinalis, Piper nigrum or Juniperus species, and Cupressus sempervirens essential oils, have been reported to own numerous biological activities such as revolting and antifeedant activities against the Mosquito Culexpipiens, Spodoptera litura, Sitophiluszeamais, silverfish and stored grain insects [21–28]. β-caryophylleneis another naturally occurring bicyclic terpene of Psidium guajava, Cephalotaxus sinensis essential oils [29] with remarkable insecticidal potential against M. japonica, P. xylostella, and Aedes aegypti [30,31]. Considering the appreciable deterrent and insecticidal potential of both phytoconstituents α-pinene and β-caryophyllene against various insect species, the present study was designed to assess the fumigant toxicity of α-pinene and β-caryophyllene against M. persicae. Furthermore, a comparison of the effects of α-pinene and β-caryophyllene on the expression of five genes in M. persicae (HSP 60, FPPS I, OSD, TOL, and ANT) was made. The current study provides a detailed understanding of the molecular actions underlying M. persicae’s reproductive, developmental, and trauma responses to phytoconstituents under investigation. The conclusions of this study could lay the groundwork for developing original eco-friendly and decomposable insecticides derived from eatable plant essential oils.
To examine the insecticidal potential of phytochemicals under investigation against M. persicae, active constituents α-pinene and β-caryophyllene with 97% and 98% purity respectively were purchased from Innochem Science and Technology Co., Ltd. (Beijing, China).
Stock culture of M. persicae was sustained under an innocuous and insect-free environment at the insectarium of Anhui Agriculture University (China) for over four years. Cabbage plants were used to nurture the insects and then placed in an incubator at ± 26°C and 78% relative humidity in a photoperiod of 16:8 h (L:D) [32].
To assess the fumigant toxicity of compounds α-pinene and β-caryophyllene caryophyllene extracted from Rosemary (Rosemainus officinalis) and Piper cubeba essential oil. For the fumigation bioassay, a 500 ml glass container was used. In each treatment, three replication and 30 adult M. persicae were placed. Five different doses were chosen (3.5, 4.0, 4.5, 5.0, and 6.0 μL L−1). Using a microinjector, drops of each dose of α-pinene and β-caryophyllene were functional to a part of filter paper (3 × 3 cm) attached to the bottom side of the jar lid. Cabbage leaf was used to provide a suitable environment for M. persicae. Then glass containers were preserved in the culturing environments stated overhead. Those insects which were deprived of the constituent’s handling were taken as a control. All dealings and panels were executed thrice autonomously. Insect death was noted at 24, 48, and 72 h post-treatment. Abbott’s formula was applied to calculate the mortality of M. persicae [33]. Furthermore, the lethal concentrations (LC30 and LC50) were calculated using probit analysis.
2.4 Real-Time Quantitative PCR (qRT-PCR)
qRT-PCR was carried out to probe the countenance levels of five selected genes under LC30 strength of both compounds α-pinene and β-caryophyllene. After 24 h of post-treatment, at least 50 M. persicae were composed and immediately deposited in liquid nitrogen, where they were preserved at −80°C until further analysis. The constructor’s commands extracted the entire RNA from each treatment using TRIZOL reagent (Invitrogen, Carlsbad, MX, USA). The Biophotometer Plus (Eppendorf, Hamburg, Germany) was used to assess and quantify RNA integrity. To improve RNA stability, cDNA was synthesized using the Prime Script™ reagent kit (Takara, Dalian, China) according to the manufacturer’s instructions. For the quantification of OSD and gene, qRT-PCR analysis was performed using β-actin as internal control, whereas the ACE gene was used as an inner switch for quantification of ANT, HSP 60, and FPPS I. All primers for qRT-PCR analysis are enlisted in Table 1. Bio-Rad iCycler iQ Real-Time Detection System (Bio-Rad, Hercules, CA, USA) which contained 2x UltraSYBR Mixture (Progema Corporation, Beijing, China) 7.5 μL, cDNA 2 μL, each primer (10 μM) 1 μL, and of RNase-free water 3.5 μL in a final volume of 15 μL, was used for the execution of qRT-PCR. Every treatment was repeated three times for all qRT-PCR. The 2−ΔΔCT technique was used to quantify the gene expression (mean ± SD) as a relative fold change [34]. The comparative quantifiable fold appearance change was assessed using one-way ANOVA [35].
The Log-Probit model analysis was applied to mortality rate of M. persicae to determine the 30% and 50% lethal concentrations (LC30 and LC50). The data for mortality rate of M. persicae was statistically analyzed by three-way analysis of variance (ANOVA) using the Statistical Package for the Social Sciences (SPSS) version 16.0. The Tukey HSD analysis was applied at 5% probability of Type I error (α) to separate the means of the obtained data using ANOVA. The mean values were compared through alphabetical letters using Statistix 8.1 and standard error were calculated through software MS Excel version 16.0.
Two major phytoconstituents’ (α-pinene and β-caryophyllene) have demonstrated tremendous promise in pest management and may have an ecologically acceptable alternative to conventional insecticides. In the present study, the pure phytoconstituents (α-pinene and β-caryophyllene) in five ascending concentrations (3.5 to 6.0 μL L−1 of air) were used to investigate the insecticidal potential against M. persicae.
3.1 Fumigant Toxicities of α-Pinene and β-Caryophyllene
We performed a fumigant bioassay to investigate the toxicity of α-pinene and β-caryophyllene against the horticultural pest M. persicae. Five different doses (3.5, 4, 4.5, 5, and 6 μL L−1) were finalized for both constituents. The LC30 and LC50 values of mortality rate after 24, 48, and 72 h of α-pinene and β-caryophyllene application were calculated through probit analysis (Table 2). After 24 h LC30 and LC50 α-pinene and β-caryophyllene were 4.2, 5.2, and 5.5, 6.8 μL L−1 of air. The constituent’s fumigant control proved effective against M. persicae. Both constituents performed well; however, the response of α-pinene was superior to that of β-caryophyllene. The highest mortality rates of 98% and 74.80% after 72 h were observed by exposing to the highest concentration of α-pinene and β-caryophyllene (6 μL L−1 of air). At the same time, at the lowest concentration of constituents α-pinene and β-caryophyllene (3.5 μL L−1 of air) mortality rate was also low up to 15.6% and 4.3% after 24 h of exposure, respectively (Fig. 1 and Table S1).
Figure 1: The percentage of fumigant (A) α-pinene and (B) β-caryophyllene toxicity at different times in response to different doses. A percentage represented the mortality rate
Three-way analysis of variance (ANOVA) revealed significant mortality rates individually in treatments, doses, time, and the interactions between treatments × doses and doses × time (Table 3). However, the interactions between treatments × time and treatments × doses × time were non-significant (p < 0.05). The interactions between treatments × doses and doses × time were analyzed through two-way ANOVA, and results are given in Tables 4 and 5. The treatment × doses interactions revealed the highest mortality in the sequence of 6.0 µL L−1 > 5.5 µL L−1 > 5.0 µL L−1 > 4.0 µL L−1 > 3.5 µL L−1, while application of α-pinene reported more mortality than β-caryophyllene (Table 4). These doses cause a higher mortality rate as time duration increases (Table 5). Applying α-pinene and β-caryophyllene up to 72 h time reported maximum mortality followed by 48 and 24 h of incubation.
The interaction between treatment and doses was statistically significant at p < 0.05; the values that share different letters in the lower case along the column and row were statistically significant at p < 0.05 and vice versa; The treatments and doses values that share different letters in the upper case along last column and last row were statistically significant at p < 0.05 and vice versa; SE stands for standard error.
The interaction between doses and time was statistically significant at p < 0.05; the values that share different letters in the lower case along the column and row were statistically significant at p < 0.05 and vice versa; The doses and time values that share different letters in the upper case along last column and last row were statistically significant at p < 0.05 and vice versa; SE stands for standard error.
To find out the difference in gene expression patterns, gene expression profiling was performed on M. persicae (adult) at sub-lethal concentrations (LC30). Various researchers have examined gene expression responses to several treatments in relative quantity (RQ) to that in controlled M. persicae. A two-fold increase or decrease in expression was considered biologically significant [38]. The variation in the expression of five genes (HSP60, FPPS I, OSD, TOL, and ANT) was studied [39] in adult M. persicae exposed to α-pinene and β-caryophyllene for 24 h. OSD, TOL, and ANT (dispersal-related genes) have previously been shown to be overexpressed in M. persicae in response to stress. The results of this study indicate all three genes showed varying levels of overexpression, except for OSD genes that showed several times greater upregulation in M. persicae, at an LC30 dose of α-pinene and β-caryophyllene.
In adult M. persicae, a 5-fold increase in OSD gene expression was observed after exposure to the lethal dose (LC30) of α-pinene (Fig. 2A). The LC30 dose of β-caryophyllene has been shown to have a maximum expression of the OSD gene. At the same lethal dose (LC30) of β-caryophyllene, α-pinene causes a 2-fold less augmentation in the expression of OSD gene in M. persicae after the same period. However, essential oil constituents (α-pinene and β-caryophyllene) boosted strongly upregulated gene expression in comparison to the control (Fig. 2A). Initial studies reveal that fecundity can be suppers at enhanced levels of OSD in M. persicae, which correlates with reduced reproduction [36].
Figure 2: Relative gene expression of five different genes OSD (Olfactory Segment-D), TOL (Take-outlike), ANT (Adenosine nucleotide translocase), HSP 60 (Heat shock protein), and FPPS I (Farnesyl diphosphate synthase) were calculated at a sublethal concentration (LC30) of two constituents of essential oil (α-pinene and β-caryophyllene) in adults M. persicae [35]. Mean values and standard deviations (SDs) are indicated by the error bar. ***Significant difference (p < 0.001), **significant difference at p < 0.01
The constituents α-pinene and β-caryophyllene incite markedly increased TOL gene expression at LC30 doses. On exposure to α-pinene and β-caryophyllene, an unexpectedly different TOL gene expression pattern was recorded compared to the OSD gene. Compared to α-pinene and β-caryophyllene, the highest expression of the TOL gene was recorded at the LC30 dose of α-pinene (Fig. 2B). Previous results demonstrate that TOL can be overexpressed in response to starvation [40,41] and fluctuation in JH (Juvenile hormones) titers [42] during courtship and mating [43]. At an LC30 dose of α-pinene and β-caryophyllene, significant upregulation of the TOL gene was recorded. It has been previously reported that [41–43] upregulation of; the TOL gene causes starvation due to juvenile hormones binding proteins. It is carried out by fluctuation in JH titers, affecting antennal responses to food.
In contrast to OSD gene expression under treatment of α-pinene LC30 dose, ANT gene expression is high. However, its expression is lower than TOL gene. A comparison between α-pinene and β-caryophyllene also shows the variation at the same dose. At the LC30 dose of α-pinene, ANT gene expression is 2 folds high in M. persicae as compared to β-caryophyllene LC30 dose (Fig. 2C). ANT gene specifically regulates mitochondrial proteins. These proteins are responsible for vital functions such as carriers of essential metabolites, which facilitate several mitochondrial roles, catalyzing trans-membranous (mitochondrial) transport of ADP to synthesize ATP [44]. With the modest comeback of the ANT gene to an at LC30 dosage of β-caryophyllene, ANTgene expresses moderate response, which shows negligible energy expenditures for M. persicae.
One of the stress response genes is HSP 60, and its expression varies according to each stressor type. Multiple studies have proven the downregulation of the HSP gene family (HSP 60, HSP 70, and HSP 90) associated with a recovery response after prolonged exposure to even mild stress. On the other hand, the upregulation of HSP 60 demonstrates the buildup of impaired proteins after stress or injury to an organism [45]. In addition, HSP accumulation has been reported to decrease fecundity [46]. In this study, after 24 h exposure to topical treatment of adult M. persicae with LC30 dose of α-pinene and β-caryophyllene, an almost 6 and 9-fold upregulation in the expression of the HSP 60 gene was obtained (Fig. 2D). Meanwhile, three folds reduced expression was noticed in response to LC30 dose of α-pinene. Compared to previous studies [46], it can be concluded that α-pinene and β-caryophyllene at higher doses negatively affect M. persicae reproduction by reducing fecundity. Results show that α-pinene has a low effect on HSP 60 gene compared to β-caryophyllene.
FPPS I gene expression was enhanced post-treatment with LC30 of α-pinene and β-caryophyllene (Fig. 2E). At LC30 dose of α-pinene, FPPS Igene showed the highest (4 folds) upregulation. β-caryophyllene while 2-fold downregulation in FPPS Igene expression was noted in β-caryophyllene compared to α-pinene (Fig. 2E). Previously, it came to know that JH biosynthesis is affected by [47] FPPS I by catalyzing the synthesis of farnesyl diphosphate (FPP). The production of sexual pheromones in M. persicae is stimulated by JH. At the same time, increased JH titers in female insects promote the growth of apterous forms via inhibition of wing growth. FPPS I downregulation is related to reduced production of β-farnesene (EBF) [47,48], which may raise egg-laying capacity in M. persicae.
In short, it was concluded that an enhancement in the expression of FPPS I gene could negatively affect the reproduction and fecundity in M. persicae. Recent studies show significantly upregulated FPPS I gene expression after 24 h treatment with (LC30) of α-pinene and β-caryophyllene in adult M. persicae. Hence, the phytochemical constituents (α-pinene and β-caryophyllene) were found to be inversely related to the fecundity and reproduction of M. persicae.
In this study, α-pinene and β-caryophyllene of Rosemary and Piper cubeba oils have demonstrated potent fumigant toxic effects for controlling adults M. persicae. A comparative gene expression analysis has further supported that α-pinene upregulates the expression of TOL, ANT, and FPPS I genes more drastically than β-caryophyllene at the same dose (LC30) and time intervals (24 h).
In the present study, the major constituents of two essential oils, α-pinene and β-caryophyllene, demonstrate mild to excellent control against M. persicae at all concentrations due to their promising anti-insecticidal activity. Gene expression assay identified that α-pinene and β-caryophyllene could affect the reproduction of M. persicae even at low concentrations. The findings can offer appreciable strategies for the rational design and discovery of innovative constituents, artificially or from natural products, with improved insecticidal potential and a higher biological safety profile.
Acknowledgement: Authors would like to extend their sincere appreciation to the Researchers Supporting Project Number (RSP2023R123), King Saud University, Riyadh, Saudi Arabia.
Funding Statement: This work was funded by the Researchers Supporting Project Number (RSP2023R123), King Saud University, Riyadh, Saudi Arabia.
Author Contributions: Study conception and design: T. A. Chohan; data collection: T. A. Chohan, T. A. Chohan, M. Hassan; analysis and interpretation of results: S. Din, I. Naseer, A. Riaz, T. Naseem, Areeba Iftikhar, D. E. N. Ali, H. M. Ali; draft manuscript preparation: T. A. Chohan, M. W. Alam, M. Z. Mumtaz, D. N. Ali. All authors reviewed the results and approved the final version of the manuscript.
Conflicts of Interest: The authors declare they have no conflicts of interest to report regarding the present study.
References
1. Adhikari, B., Pendry, C. R., Pennington, R., Milne, R. (2012). A revision of Berberis ss (Berberidaceae) in Nepal. Edinburgh Journal of Botany, 69(3), 447–522. [Google Scholar]
2. Alyokhin, A., Nault, B., Brown, B. (2020). Soil conservation practices for insect pest management in highly disturbed agroecosystems. Entomologia Experimentalis et Applicata, 168(1), 7–27. [Google Scholar]
3. Dedryver, C. A., Le, R. A., Fabre, F. (2010). The conflicting relationships between aphids and men: A review of aphid damage and control strategies. Comptes Rendus Biologies, 333, 539–553. [Google Scholar] [PubMed]
4. Liu, F. H., Kang, Z. W., Tan, X. L., Fan, Y. L., Tian, H. G. et al. (2020). Physiology and defense responses of wheat to the infestation of different cereal aphids. Journal of Integrative Agriculture, 19(6), 1464–1474. [Google Scholar]
5. Edwards, O. R., Franzmann, B. B., Thackray, D., Micic, S. (2008). Insecticide resistance and implications for future aphid management in Australian grains and pastures: A review. Australian Journal of Experimental Agriculture, 48(12), 1523–1530. [Google Scholar]
6. Hagenbucher, S., Wackers, F. L., Romeis, J. (2014). Aphid honeydew quality as a food source for parasitoids is maintained in Bt cotton. PLoS One, 9(9), e107806. https://doi.org/10.1371/journal.pone.0107806 [Google Scholar] [PubMed] [CrossRef]
7. Panini, M., Dradi, D., Marani, G., Butturini, A., Mazzoni, E. (2014). Detecting the presence of target-site resistance to neonicotinoids and pyrethroids in Italian populations of Myzus persicae. Pest Management Science, 70(6), 931–938. [Google Scholar] [PubMed]
8. Foster, S., Denholm, I., Devonshire, A. (2000). The ups and downs of insecticide resistance in peach-potato aphids (Myzus persicae) in the UK. Crop Protection, 19, 873–879. [Google Scholar]
9. Dhungana, S. K., Adhikari, B., Adhikari, A., Kim, I. D., Kim, J. H. et al. (2020). Comparative effect of different insecticides on the growth and yield of soybeans. Plant Protection Science, 56(3), 206–213. [Google Scholar]
10. Isman, M. B., Grieneisen, M. L. (2014). Botanical insecticide research: Many publications, limited useful data. Trends in Plant Science, 19(3), 140–145. [Google Scholar] [PubMed]
11. Marrone, P. G. (2019). Pesticidal natural products-status and future potential. Pest Management Science, 75(9), 2325–2340. [Google Scholar] [PubMed]
12. Isman, M. B. (2006). Botanical insecticides, deterrents, and repellents in modern agriculture and an increasingly regulated world. Annual Review of Entomology, 51, 45–66. [Google Scholar] [PubMed]
13. Isman, M. B. (2020). Commercial development of plant essential oils and their constituents as active ingredients in bioinsecticides. Phytochemistry Reviews, 19(2), 235–241. [Google Scholar]
14. Czerniewicz, P., Chrzanowski, G., Sprawka, I., Sytykiewicz, H. (2018). Aphicidal activity of selected Asteraceae essential oils and their effect on enzyme activities of the green peach aphid Myzus persicae (Sulzer). Pesticide Biochemistry and Physiology, 145, 84–92. [Google Scholar] [PubMed]
15. Pavela, R. (2018). Essential oils from Foeniculum vulgare Miller as a safe environmental insecticide against the aphid Myzus persicae Sulzer. Environmental Science and Pollution Research, 25(11), 10904–10910. [Google Scholar] [PubMed]
16. Rajendran, S., Sriranjini, V. (2008). Plant products as fumigants for stored-product insect control. Journal of Stored Products Research, 44(2), 126–135. [Google Scholar]
17. Suthisut, D., Fields, P. G., Chandrapatya, A. (2011). Contact toxicity, feeding reduction, and repellency of essential oils from three plants from the ginger family (Zingiberaceae) and their major components against Sitophilus zeamais and Tribolium castaneum. Journal of Economic Entomology, 104(4), 1445–1454. [Google Scholar] [PubMed]
18. Dehliz, A., Lakhdari, W., Mlik, R., Chahbar, N., Acheuk, F. et al. (2022). Chemical composition and bioactivity of essential oil against the green peach aphid (Myzus persicae). Organic Agriculture, 12, 1–8. [Google Scholar]
19. Abdelatti, Z. A. S., Hartbauer, M. (2020). Plant oil mixtures as a novel botanical pesticide to control gregarious locusts. Journal of Pest Science, 9(1), 341–353. [Google Scholar]
20. González-Coloma, A., Guadaño, A., Tonn, C. E., Sosa, M. E. (2005). Antifeedant/insecticidal terpenes from Asteraceae and Labiatae species native to Argentinean semi-arid lands. Zeitschrift für Naturforschung C, 60, 855–861. [Google Scholar]
21. Zeghib, F., Tine-Djebbar, F., Zeghib, A., Bachari, K., Sifi, K. et al. (2020). Chemical composition and larvicidal activity of Rosmarinusofficinalis essential oil against west nile vector mosquito Culex pipiens (L.). Journal of Essential Oil-Bear Plants, 23(6), 1463–1474. [Google Scholar]
22. Langsi, J. D., Nukenine, E. N., Oumarou, K. M., Moktar, H., Fokunang, C. N. et al. (2020). Evaluation of the insecticidal activities of α-Pinene and 3-Carene on Sitophilus zeamais Motschulsky (Coleoptera: Curculionidae). Insects, 11(8), 540. [Google Scholar] [PubMed]
23. Baser, K. J. C., Buchbauer, G. (2009). Handbook of essential oils: Science, technology, and applications. Boca Raton, Florida, USA: CRC Press. [Google Scholar]
24. Dosoky, N. S., Satyal, P., Barata, L. M., da Silva, R. J. K., Setzer, W. N. (2019). Volatiles of black pepper fruits (Piper nigrum L.). Molecules, 24(23), 4244. https://doi.org/10.3390/molecules24234244 [Google Scholar] [PubMed] [CrossRef]
25. Traboulsi, A. F., Taoubi, K., El-Haj, S., Bessiere, J. M., Rammal, S. (2002). Insecticidal properties of essential plant oils against the mosquito Culex pipiens molestus (Diptera: Culicidae). Pest Management Science, 58(5), 491–495. [Google Scholar] [PubMed]
26. Shahriari, M., Sahebzadeh, N., Sarabandi, M., Zibaee, A. (2016). Oral toxicity of thymol, α-pinene, diallyl disulfide and trans-anethole, and their binary mixtures against Tribolium castaneum herbst larvae (Coleoptera: Tenebrionidae). Jordan Journal of Biological Science, 9(4), 213–219. [Google Scholar]
27. Wang, S. Y., Lai, W. C., Chu, F. H., Lin, C. T., Shen, S. Y. et al. (2006). Essential oil from the leaves of Cryptomeria japonica acts as a silverfish (Lepisma saccharina) repellent and insecticide. Journal of Wood Science, 52(6), 522–526. [Google Scholar]
28. Fan, L. S., Muhamad, R., Omar, D., Rahmani, M. (2011). Insecticidal properties of Piper nigrum fruit extracts and essential oils against Spodoptera litura. International Journal of Agriculture and Biology, 13(4), 512–522. [Google Scholar]
29. Arain, A., Hussain, S. T., Mahesar, S. A., Sirajuddin (2019). Essential oil from Psidium guajava leaves: An excellent source of β-caryophyllene. Natural Product Communications, 14(5), 1934578X19843007. [Google Scholar]
30. Ma, S., Jia, R., Guo, M., Qin, K., Zhang, L. (2020). Insecticidal activity of essential oil from Cephalotaxus sinensis and its main components against various agricultural pests. Industrial Crops and Products, 150, 112403. [Google Scholar]
31. Nararak, J., Sathantriphop, S., Kongmee, M., Mahiou-Leddet, V., Ollivier, E. et al. (2019). Excito-repellent activity of β-caryophyllene oxide against Aedes aegypti and Anopheles minimus. Acta Tropica, 197(Suppl 1), 105030. https://doi.org/10.1016/j.actatropica.2019.05.021 [Google Scholar] [PubMed] [CrossRef]
32. Huang, Y. Z., Hua, H. X., Li, S. G., Yang, C. J. (2011). Contact and fumigant toxicities of calamusenone isolated from Acorus gramineus rhizome against adults of Sitophilus zeamais and Rhizopertha dominica. Insect Science, 18(2), 181–188. [Google Scholar]
33. Lee, B. H., Choi, W. S., Lee, S. E., Park, B. S. (2001). Fumigant toxicity of essential oils and their constituent compounds towards the rice weevil, Sitophilus oryzae (L.). Crop Protection, 20(4), 317–320. [Google Scholar]
34. Rainen, L., Oelmueller, U., Jurgensen, S., Wyrich, R., Ballas, C. et al. (2002). Stabilization of mRNA expression in whole blood samples. Clinical Chemistry, 48(11), 1883–1890. [Google Scholar] [PubMed]
35. Pfaffl, M. W. (2001). A new mathematical model for relative quantification in real-time RT-PCR. Nucleic Acids Research, 29(9), e45. [Google Scholar] [PubMed]
36. Ayyanath, M. M., Cutler, G. C., Scott-Dupree, C. D., Prithiviraj, B., Kandasamy, S. et al. (2014). Gene expression during imidacloprid-induced hormesis in green peach aphid. Dose-Response, 12(3), 13–57. [Google Scholar]
37. Puinean, A. M., Foster, S. P., Oliphant, L., Denholm, I., Field, L. M. et al. (2010). Amplification of a cytochrome P450 gene is associated with resistance to neonicotinoid insecticides in the aphid Myzus persicae. PLoS Genetics, 6(6), e1000999. https://doi.org/10.1371/journal.pgen.1000999 [Google Scholar] [PubMed] [CrossRef]
38. Ghanim, M., Dombrovsky, A., Raccah, B., Sherman, A. (2006). A microarray approach identifies ANT, OS-D and takeout-like genes as differentially regulated in alate and apterous morphs of the green peach aphid Myzus persicae (Sulzer). Insect Biochemistry and Molecular Biology, 36(11), 857–868. [Google Scholar] [PubMed]
39. Stanley, K., Fenton, B. (2000). A member of the Hsp60 gene family from the peach potato aphid, Myzus persicae (Sulzer.). Insect Molecular Biology, 9(2), 211–215. [Google Scholar] [PubMed]
40. Fujikawa, K., Seno, K., Ozaki, M. (2006). A novel Takeout-like protein expressed in the taste and olfactory organs of the blowfly (Phormia regina). The FEBS Journal, 273(18), 4311–4321. [Google Scholar] [PubMed]
41. Weil, T., Korb, J., Rehli, M. (2009). Comparison of queen-specific gene expression in related lower termite species. Molecular Biology and Evolution, 26(8), 1841–1850. [Google Scholar] [PubMed]
42. Bohbot, J., Vogt, R. G. (2005). Antennal expressed genes of the yellow fever mosquito (Aedes aegypti L.); Characterization of odorant-binding protein 10 and takeout. Insect Biochemistry and Molecular Biology, 35(9), 961–979. [Google Scholar] [PubMed]
43. Dauwalder, B., Tsujimoo, S., Moss, J., Mattox, W. (2002). The Drosophila takeout gene is regulated by the somatic sex-determination pathway and affects male courtship behavior. Genes Development, 16(22), 2879–2892. [Google Scholar] [PubMed]
44. Zhang, Y. Q., Roote, J., Brogna, S., Davis, A. W., Barbash, D. A. et al. (1999). Stress sensitive B encodes an adenine nucleotide translocase in Drosophila melanogaster. Genetics, 153(2), 891–903. [Google Scholar] [PubMed]
45. Parsell, D., Lindquist, S. (1993). The function of heat-shock proteins in stress tolerance: Degradation and reactivation of damaged proteins. Annual Reviews of Genetics, 27, 437–497. [Google Scholar] [PubMed]
46. Huang, L. H., Chen, B., Kang, L. (2007). Impact of mild temperature hardening on thermotolerance, fecundity, and Hsp gene expression in Liriomyza huidobrensis. Journal of Insect Physiology, 53(12), 1199–1205. [Google Scholar] [PubMed]
47. Lewis, M., Prosser, I., Mohib, A., Field, L. (2008). Cloning and characterization of a prenyl transferase from the aphid Myzus persicae with potential involvement in alarm pheromone biosynthesis. Insect Molecular Biology, 17(4), 437–443. [Google Scholar] [PubMed]
48. Vandermoten, S., Charloteaux, B., Santini, S., Sen, S. E., Béliveau, C. et al. (2008). Characterization of a novel aphid prenyltransferase displaying dual geranyl/farnesyl diphosphate synthase activity. FEBS Letters, 582(13), 1928–1934. [Google Scholar] [PubMed]
Cite This Article
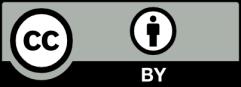
This work is licensed under a Creative Commons Attribution 4.0 International License , which permits unrestricted use, distribution, and reproduction in any medium, provided the original work is properly cited.