Open Access
ARTICLE
Identification of Resistance to Pathogenesis Related Protein GmPR1L in Tobacco Botrytis cinerea Infection
1 College of Agronomy, Jilin Agricultural University, Changchun, China
2 Northeast Institute of Geography and Agroecology, Chinese Academy of Sciences, Changchun, China
* Corresponding Author: Piwu Wang. Email:
# These authors contributed equally to this work
(This article belongs to the Special Issue: Symbiotic Associations for Nutrients Management and Complexes Formation for Better Agricultural Crops Productivity under Biotic and Abiotic Stresses)
Phyton-International Journal of Experimental Botany 2023, 92(6), 1907-1920. https://doi.org/10.32604/phyton.2023.027607
Received 05 November 2022; Accepted 22 February 2023; Issue published 11 April 2023
Abstract
Soybean (Glycine max (Linn.) Merr.) annual leguminous crop is cultivated all over the world. The occurrence of diseases has a great impact on the yield and quality of soybean. In this study, based on the RNA-seq of soybean variety M18, a complete CDS (Coding sequence) GmPR1L of the pathogenesis-related protein 1 family was obtained, which has the ability to resist fungal diseases. The overexpression vector and interference expression vector were transferred into tobacco NC89, and the resistance of transgenic tobacco (Nicotiana tabacum L.) to Botrytis cinerea infection was identified. The results show that: Compared with the control, the activities of related defense enzymes SOD (Superoxide dismutase), POD (Peroxidase), PAL (L-phenylalanine ammonia-lyase) and PPO (Polyphenol oxidase) in the over-expressed transgenic tobacco OEA1 and OEA2 increased to different degrees, and increased significantly at different infection time points. The activities of defense enzymes in the interfering strains IEA1 and IEA2 were significantly lower than those in the control strains. The results of resistance level identification showed that the disease spot rate of OEA1 was significantly lower than that of the control line, and the disease spot rate of OEA2 was significantly lower than that of the control line. The plaque rate of the interfering expression line IEA1-IEA2 was significantly higher than that of the control line. It is preliminarily believed that the process related protein GmPR1L can improve the resistance of tobacco to B. cinerea.Keywords
Supplementary Material
Supplementary Material FileAs the main food crop, everyone has favored soybean for its high yield, wide application, and high economic value [1]. In the process of plant growth, it is inevitable that plants will be subjected to various biotic and abiotic stresses, and plants will activate their defense system through a series of signal transduction in the body [2]. Through its own metabolic changes, the organism produces some substances that can resist the infection of these pathogens and adverse environments [3]. Among them, disease-resistant substances can be divided into three categories: plant secondary metabolites, non-protein substances, and protein substances. As the direct coding products of genes, protein-based disease-resistant substances play a more important role in plant disease resistance [4]. It is of great significance to study these protein-based disease-resistant substances and their metabolic pathways for improving soybean disease resistance. Pathogenesis-related protein is an important defense protein expressed in plants [5]. PRs (Pathogenesis-related protein) mainly distributes in vacuoles and intercellular cells of plants, and its relative molecular mass is small. Most of them are acidic proteins, which show strong stability at low pH (<3) and high temperature (60°C) [6]. Relevant studies have shown that pathogenesis-related proteins will participate in plant defense when infected by pathogens, directly destroying the integrity of pathogens and limiting their spread of pathogens. When plants are infected by pathogens, the expression of PR protein is significantly increased [7]. Pathogenesis-related proteins were first detected in tobacco leaves infected by the tobacco mosaic virus, and then found to exist widely in monocotyledonous and dicotyledonous plants. PRs can be divided into 17 families according to amino acid sequence homology, physical and chemical properties, biological activity, and serology relationship. Among them, PR2 (β-1,3-glucanase), PR3 (chitinase), PR5 (sweet-like protein), PR6 (protease inhibitor), PR7 (endoprotease), PR9 (peroxidase), PR10 (ribonuclease-like enzyme), PR12 (defense element), PR13 (Sulphur) and PR14 (lipid transporter) have been identified [8].
Among the members of PR family, PR1 protein is rich in variety, induced by a pathogen or salicylic acid, and is usually used as a marker of SAR (Systemic acquired resistance) [9]. PR1 belongs to the cysteine-rich protein superfamilly, and is an important protein in the process of plant resistance to fungal diseases and abiotic stress. It is formed around the infected parts of plants, has antifungal activity, can hydrolyze and kill fungal cells, and can fight against plant pathogenic fungi in plants, such as Urine mold of Vicia faba, Phytophthora and Wheat toxin [10]. PR1 gene has been widely used as an indicator gene for the activation of defense pathway and salicylic acid-mediated resistance response [11]. All PR1 proteins have a conserved CAP (Methylguanosine cap) domain, usually consisting of 150 amino acid residues, folded into 4 α-helices and 4 β-chains, which are stabilized by disulfide bonds. The antimicrobial activity of the CAP domain is due to a pit protein binding motif, which is responsible for binding and isolating sterols on the membrane of pathogens, and a CAP-derived peptide (CAPE), resulting from the cleavage of the last 11 residues of the CAP domain, trigger defense response through independent signal path [12]. These unique features determine their biological functions against biotic and abiotic stresses [13]. Studies have shown that transgenic tobacco and tomato expressing the PR1 gene can obviously enhance their resistance to oomycetes and fungi [14,15]. The existing research also found that the main stress-related transcription factor (TF) families (for example, WRKY, C2H2, and ERF) are specific regulators of PR1 gene expression [16–18].
In this study, a soybean pathogenesis-related proteins gene was obtained from the RNA-seq analysis of soybean variety M18 in the early stage, and it was named GmPR1L by NCBI search and comparison, and the over-expression vector and interference expression vector of the target gene were constructed. Tobacco was infected by the leaf disc method, and positive transgenic tobacco plants were successfully obtained by PCR detection. Then, the resistance of positive transgenic tobacco to B. cinerea was identified, and the activities of related defense enzymes in infected tobacco leaves were determined. The relative expression quantity of genes related to GmPR1L expression was determined, and the resistance mechanism of GmPR1L to fungal disease gray mold was further clarified.
2.1 Plant Sowing and Cultivation
In this experiment, soybean material M18 and tobacco variety NC89 were provided by the Biotechnology Center of Jilin Agricultural University, China. The pathogenic strain Phytophthora sojae root rot was provided by the Plant Protection Institute of Jilin Academy of Agricultural Sciences. Soybean variety M18 is planted 5–8 cm deep in the soil, watered regularly, and the room temperature is 22°C–26°C [19]. The planting of tobacco NC89 requires the seeds to be germinated through MS germination nutrient medium, and then through the process of elongation and rooting, transplanted into the soil at 25°C, and regularly watered.
Taking soybean variety M18 as experimental material, plump and round seeds were selected and planted in 12 cm × 9 cm × 11 cm sowing pots with 3 seeds in each pot. The volume ratio of organic nutrient soil and vermiculite (steam sterilization) was 8:2. Fully irrigate till the deep soil is wet, and place it in an artificial culture room at 25°C, with alternating day 12 h and night illumination 12 h. After emergence, irrigate a little every 5 days. A total of 12 pots and 36 M18 soybean seeds were sown. Analysis of the expression pattern of the target gene of soybean M18 plant material infected by fungal diseases.
2.2 Expression of GmPR1L Infected by Phytophthora sojae
When the soybean plant grows until the second pair of three compound leaves are fully unfolded, the inoculation identification is carried out. With the scalpel after high-temperature sterilization and cooling, the Phytophthora root rot fungus plate propagated on carrot culture medium in advance was divided into 0.3 cm × 0.3 cm pieces. Then cut the hypocotyl of the soybean plant at 45 degrees with a scalpel, insert the small fungus block obliquely into the wound, spray ddH2O, and wrap the wound and fungus block with plastic wrap. The inoculated plants were placed in an artificial climate culture room at 25°C, and the day and night were illuminated alternately. There are three pots of soybean plants in each inoculation time, with 3 plants in each pot. Soybean leaves infected for 0, 3, 5, and 7 days were placed in a refrigerator at −80°C to extract total RNA from soybean and analyze the expression pattern of GmPR1L.
2.3 Construction of Expression Vector and Obtaining of Transgenic Tobacco Plants
We amplified the protein encoded by GmPR1L by PCR, and the primers are shown in Supplementary Table 1. After obtaining the exact size of the target fragment, we constructed the over-expression vector of the target gene pCAMBIA3301-GmPR1L-over and the interference expression vector pCambia3301-GmPR1L-RNAi. The two expression vectors were transferred into Agrobacterium EHA105, and the leaves of tobacco NC89 were infected by the leaf disc method. Harvest seeds, detect marker gene bar, screen positive tobacco transformation strains, subculture to T2 generation, and conduct subsequent disease resistance identification.
2.4 Infections of Tobacco B. cinerea Infected by Fungal Diseases
Breeding of strains: Potato PDA medium was selected to breed B. cinerea, and the formula of the medium was as follows: 200 g fresh potato, wash, cut into small pieces, add about 1.2 L distilled water, boil for 15 min, and leave about 1 L of potato liquid. After filtering with three layers of gauze, add 10 g agar and 20 g sucrose, make the volume constant to 1 L, steam sterilization at 120°C for 20 min, and blow-dry by pouring. Soak the surgical blade in alcohol, burn and disinfect it for 3 times, cut 0.4 cm × 0.4 cm pieces of bacteria, inoculate them on PDA culture medium, double-layer sealing film, and put them in an incubator at 25°C for 7 days at room temperature. Plant inoculation: Tobacco B. cinerea was inoculated by foliar spraying, and the inoculation identification was carried out after the fifth leaf stage. Preparing 3% sucrose solution in advance, sterilizing at high temperature, and cooling to room temperature; Dip the surgical blade in alcohol, burn, and sterilize it three times. When the blade is cooled, gently scrape off the mycelium spores on the surface of the culture medium, pour them into sucrose solution for culture, and observe them under a high-power microscope. After the number of spores is about 20 in the 100-fold field of vision, spray them wet. To ensure even inoculation, it is necessary to use the same watering can, cover it with plastic film in an artificial room, and keep the temperature at about 25°C and keep the humidity at about 90%.
2.5 Resistance of Transgenic Tobacco to B. cinerea
Three positive plants were selected from each of the five tobacco strains WT, OEA1, OEA2, IEA1 and IEA2, and seven tobacco leaves were selected from the upper, middle, and lower positions for investigation. It mainly measures the leaf area and disease spot area, and calculates the disease spot rate according to the following formula. The disease spot rate of three positive plants of each strain is taken as the average value to judge the incidence of transgenic tobacco from the phenotype.
2.6 Determination of Defense Enzyme Activity of Transgenic Tobacco Infected by B. cinerea
Tobacco leaves infected with the appropriate amount of B. cinerea for 0, 3, 5 and 7 d, respectively. The activity of superoxide dismutase (SOD) was determined by the NBT photoreduction method [20,21]. The activity of peroxidase (POD) was determined by the guaiacol method [22,23] (PAL) and (PPO) activity were determined by spectrophotometry [24,25]. The above tests were repeated 3 times for each treatment.
2.7 Expression of GmPR1L and Related Genes in Transgenic Tobacco Infected by B. cinerea
Trizol (Takara) kit was used to extract total RNA from tobacco leaves [19]. cDNA was synthesized by reverse transcription kit (Takara). Use the SYBR Premix Ex Taq (Takara) test kit to proceed with qRT-PCR [26]. Melting curve analysis is carried out. The data were analyzed by 2−ΔΔCT method [21]. The internal reference primer is Actin2S/Actin2AS. All the above gene primers are shown in (Suppl. Table 1), the key instruments real-time PCR was 7500 Fast.
Data were tested by analysis of variance using SPSS software (SPSS USA). The data are the mean ± standard deviation (SD) of three biological replicates. The significance was analyzed using Student’s T-tests. The * and ** represent p < 0.05 and p < 0.01, respectively. The figures were prepared with GraphPad Prism 8.0.
3.1 The Expression of GmPR1L is Related to the Infection of Phytophthora Root Rot
In order to explore the resistance of GmPR1L to fungal diseases, we analyzed the expression pattern of GmPR1L under the infection of Phytophthora root rot fungus. Under the infection of Phytophthora root rot fungus, the expression of GmPR1L showed a trend of first increasing and then decreasing, the peak expression of GmPR1L was 5.53 times higher than that of the control after 5 days of pathogen infection (Fig. 1). Based on the above results, we preliminarily believe that overexpression of GmPR1L plays an important role in improving the resistance of soybean plants to fungal diseases.
Figure 1: Relative expression of GmPR1L under the infection of Phytophthora root rot fungus. 0, 5, 3, 7 days. Relative expression of GmPR1L after 0, 3, 5 and 7 days after infection by Phytophthora root rot fungus (*p < 0.05, **p < 0.01)
3.2 Bioinformatics Analysis of GmPR1L
The amino acid sequence of the target gene was searched online by NCBI, and pathogenes-related protein 1 family genes of soybean, corn, rice, barley, wheat, Arabidopsis, and other species were collected, using Mega7 to build a multi-species phylogenetic tree (Fig. 2). We found that these species are mainly divided into four branches, in which the target gene NM_ 001371209.1 and pathogenes related protein 1 (PR1-6) NM_ 001371185.1 has the highest genetic affinity. We named the target gene GmPR1L.
Figure 2: Phylogenetic tree of GmPR1L. Glycine soja [NM_001371209.1], Glycine soja [NC_038251.2:4797098-4797858], Glycine soja [NC_038251.2:4793226-4794056], Glycine soja [NM_001371185.1], Arabidopsis thaliana [CAA65420.1], Zea mays [ONM51202.1], Triticum aestivum [XP_044436102.1], Hordeum vulgare [KAE8813017.1], Medicago truncatula [XP_039686160.1], Zea mays [ABA34060.1], Triticum aestivum [AEH25631.1], Arabidopsis thaliana [OAP08110.1], Vitis vinifera [ADN43427.1], Nicotiana tabacum [XP_016477771.1], Capsicum annuum [KAF3675629.1], Nicotiana tabacum [CAA29022.1], Nicotiana tabacum [CAA29023.1], Solanum lycopersicum [NP_001234128.2], Capsicum annuum [XP_016582333.1], Capsicum annuum [PHT64208.1], Capsicum annuum [XP_016582335.1], Solanum lycopersicum [ATG24055.1], Gossypium hirsutum [XP_016718985.1], Trifolium pretense [XP_045818452.1], Cajanus cajan [XP_020234033.1], Dimocarpus longan [AKE49475.1], Abrus precatorius [XP_027346994.1], Pisum sativum [XP_050879783.1]
3.3 Acquisition of Transgenic Tobacco and Southern Blot Detection of T2 Generation Positive Plants
Taking tobacco variety NC89 as the receptor, the vector plasmid was transferred into tobacco by Leaf disk method, after tobacco germination (Fig. 3A), coculture (Fig. 3B), screening (Fig. 3C), elongation (Fig. 3D) and rooting culture (Fig. 3E). Finally, 7 positive T0 transgenic tobacco plants were obtained, including two transgenic expression vector pCAMBIA3301-GmPR1L-over plants and two transgenic interference expression vector pCAMBIA3301-GmPR1L-RNAi plants. Harvest the seeds, subculture them to T2, and detect the screening marker gene bar by southern blot. It is found that the overexpression strain OEA1-OEA2 and the interference expression strain IEA1-IEA2 all have hybridization signals, as shown in Fig. 3F. This indicates that the target gene expression vector has been successfully integrated into the tobacco genome.
Figure 3: Transformation of tobacco by leaf disc method. (A) Tobacco germination, (B) Co-culture infection, (C) Tobacco screening, (D) Tobacco elongation and rooting, (E) Transplanting seedlings, (F) Southern blot detection of T2 generation positive transgenic tobacco (M: maker; P: positive control; C: Untransformed plant 1-2: OEA1-OEA2 3-4: IEA1-IEA2)
3.4 Phenotype Identification of Transgenic Tobacco Infected by B. cinerea
The identification of B. cinerea on transgenic tobacco showed that the overexpression strain OEA1-OEA2 has a small lesion area in the leaves, showing light whorls. The lesion area of the interfering expression strain IEA1-IEA2 was large, and white hyphae were visible. After 7 days of inoculation, it was found that the whole OEA1-OEA2 strain were still robust, and the whole IEA1-IEA2 strain that interfered with the expression were seriously withered. The above phenotypes indicate that overexpression of GmPR1L can improve the resistance of tobacco to B. cinerea (Fig. 4).
Figure 4: Phenotype identification of B. cinerea in tobacco. (A) Identification of wild-type tobacco, (B) Identification of overexpression positive tobacco transformation strain OEA1, (C) Identification of overexpression positive tobacco transformation line OEA2, (D) Identification of tobacco transformation strain IEA1 with positive interference expression, (E) Identification of tobacco transformation strain IEA2 with positive interference expression
3.5 Occurrence of Transgenic Tobacco Infected by B. cinerea
We made statistics on the incidence of transgenic tobacco infected by B. cinerea. We found that the disease spot rate of overexpressed transgenic tobacco OEA1 was significantly lower than that of the control line, and the disease spot rate of overexpressed transgenic tobacco OEA2 was significantly lower than that of the control line. The disease spot rate of transgenic interference expression lines IEA1 and IEA2 was significantly higher than that of control lines. Therefore, concluded that overexpression of GmPR1L could reduce the incidence of transgenic tobacco plants and resist the infection of B. cinerea (Fig. 5).
Figure 5: Disease spot rate of transgenic tobacco plants infected by B. cinerea for 7 days. WT: Wild type tobacco; OEA1-OEA2: Overexpression positive plants; IEA1-IEA2: Interference with positive plants (*p < 0.05, **p < 0.01)
3.6 Changes of Related Defense Enzyme Activities after B. cinerea Infection in Tobacco
The SOD activity of transgenic tobacco leaves infected by B. cinerea was measured. The results showed that there was no significant difference in SOD activity among the strains after 0 days of infection. After 3 days of infection, SOD activity in over-expressed strains OEA1 and OEA2 was significantly higher than that of control strains. After 5 days of infection, the SOD activity of over-expressed strains OEA1 and OEA2 was still significantly higher than that of the control, while that of the interfering strain IEA1 was significantly lower than that of the control. After 7 days of infection, the SOD activity of the over-expressed strain OEA1 was significantly higher than that of the control strain, and that of the over-expressed strain OEA2 was significantly higher than that of the control strain. In general, the SOD activity in transgenic lines showed a continuous upward trend, with a large increase in the early stage of infection and a steady increase in the late stage of infection. Therefore, overexpression of GmPR1L can improve the activity of SOD in plants, thus improving the resistance of transgenic tobacco to B. cinerea (Fig. 6A).
Figure 6: Changes of defense enzyme activity in tobacco infected by B. cinerea. (A) Changes of SOD activity in the OEA1-OEA2 overexpression line and the IEA1-IEA2 interference expression line after 0, 3, 5, and 7 days of B. cinerea infection; (B) changes of POD activity in OEA1-OEA2 overexpression line and IEA1-IEA2 interference expression line after 0, 3, 5 and 7 days of Botrytis B. cinerea infection; (C) changes of PAL activity in OEA1-OEA2 over-expression line and IEA1-IEA2 interference expression line after 0, 3, 5 and 7 days of B. cinerea infection; (D) changes of PPO activity in OEA1-OEA2 overexpression line and IEA1-IEA2 interference expression line after 0, 3, 5 and 7 days of B. cinerea (*p < 0.05, **p < 0.01)
The POD activity of transgenic tobacco leaves infected by B. cinerea was measured. The results showed that there was no significant change in POD activity in transgenic tobacco leaves after the pathogen infected for 0–3 days. After 5 days of infection, the POD activity of over-expression strain OEA1 was significantly higher than that of the control strain, while that of interference expression strains IEA1 and IEA2 was significantly lower than that of the control strain. After 7 days of infection, the POD activity of the overexpressed strain OEA1 was significantly higher than that of the control strain. Generally speaking, the POD activity in transgenic tobacco plants began to increase greatly after 5 days of infection by B. cinerea, and remained until 7 days after infection. The results indicated that overexpression of GmPR1L could improve the activity of POD in plants, thus improving the resistance of transgenic tobacco to B. cinerea (Fig. 6B).
The PAL activity of transgenic tobacco leaves infected by B. cinerea was measured. The results showed that after 3 days of infection, the PAL activity of over-expressed strain OEA1 was significantly higher than that of the control strain, that of over-expressed strain OEA2 was significantly higher than that of the control strain, that of interference expression strain IEA1 was significantly lower than that of control strain, and that of interference expression strain IEA2 was significantly lower than that of the control strain. After 5 days of infection, the PAL activity in the overexpressed strain OEA1 was significantly higher than that in the control strain, while the PAL activity in the interfering strain IEA2 was significantly lower than that in the control strain. After 7 days of infection, the PAL activity of overexpressed strain OEA1 was significantly higher than that of the control strain, and that of overexpressed strain OEA2 was significantly higher than that of the control strain. The results indicated that overexpression of GmPR1L could improve the activity of PAL in plants, thus improving the resistance of transgenic tobacco to B. cinerea (Fig. 6C).
The PPO activity of transgenic tobacco leaves infected by B. cinerea was measured. The results showed that after 3 days of infection, the PPO activity of over-expressed strains OEA1 and OEA2 was significantly higher than that of the control strain, while the PPO activity of interfering expression strains IEA1 and IEA2 was significantly lower than that of the control strain. After 5 days of infection, PPO activity in overexpressed strains OEA1 and OEA2 was significantly higher than that in control strains. After 7 days of infection, the PPO activity of over-expressed strains OEA1 and OEA2 was significantly higher than that of the control strain, and the PPO activity of the interference expression strain was significantly lower than that of the control strain. We also found that PPO activity in the expression strains increased significantly after 5 days of pathogen infection. The above results indicated that overexpression of GmPR1L could improve PPO activity in plants, thus improving the resistance of transgenic tobacco to B. cinerea (Fig. 6D).
Superoxide dismutase SOD can remove reactive oxygen species, to avoid damage to the cells [27]. In recent years, the change of activity in vivo after tobacco infection is a hot spot in plant pathology research, however, consistent conclusions have not been drawn in different interaction systems [28,29]. Lavrova et al. found, after tobacco infection, the activity increases first and then decreases, and in the early stage of infection, the activity in the non-affinity interaction system is lower than that in the affinity interaction system [30]. However, in the late stage of infection, this relationship is reversed [31,32]. Akbudak et al. [33] believed that, for two different interaction systems of systemic infection and necrosis, the change of activity in tobacco is inconsistent. In this study, it was found that the SOD activity had been improved at the early stage of B. cinerea infection and SOD activity reached the highest value after 7 days of infection. Peroxidase POD can catalyze many compounds, such as the oxidation of fatty acids, aromatic amines, and phenols. It is closely related to the synthesis of lignin and cork, among them, lignification and corking produce defense mechanisms, to thicken the cell wall and improve the disease resistance of the plant [34]. In this study, the POD of transgenic tobacco plants infected by B. cinerea was determined. After 5 days of pathogen infection, the activity of POD in the overexpression strain was greatly enhanced, and it lasts steadily until 7 days after infection.
Phenylalanine ammonia-lyase PAL can catalyze phenylalanine deamination to produce cinnamic acid and finally convert it to lignin [35,36], It is the rate-limiting enzyme of the shikimic acid and phenylalanine metabolism pathway [37]. The products of this metabolic pathway, such as coumarin, chlorogenic acid, isoflavone phytoalexin, and lignin, play an important role in plant disease resistance. In addition, the lignin produced in it is deposited around the cell wall, limit the pathogen to a certain range to prevent it from further expanding the hazard. Phenolic compounds in transgenic tobacco plants infected by B. cinerea play an important role in plant disease resistance [38]. Our research confirms that, three days after B. cinerea infects tobacco plants, the PAL content of each transgenic tobacco line has been greatly improved, and the PAL content of overexpression tobacco strains was significantly higher than that of the control. Polyphenol oxidase PPO is one of the cell-resistant enzymes. It is the main enzyme for phenol oxidation. Its mechanism is to oxidize phenols in cell metabolites into quinones to kill host cells, so as to limit the further expansion of pathogens. The quinones produced are also highly toxic to pathogens. It can even kill the pathogen or form the precursor of prephenyl acid lignin synthesis to repair the wound and inhibit the propagation of pathogenic bacteria. In our research, under the infection of B. cinerea, the activity of PPO in overexpression transgenic tobacco began to increase significantly after 5 days of infection, and reached a peak after 7 days of infection.
In model plants, exogenous SA can cause plants to produce SAR and induce the expression of PR1 gene. Many studies have found that some plants’ PR1 gene show certain resistance to pathogens. Alexander et al. [39] transferred the PR1-a gene into tobacco and found that the PR1 gene enhanced the resistance to Phytophthora and Colletotrichum in a highly constitutive expression manner. Shin et al. [40] transferred PR1 gene into Arabidopsis for overexpression, which enhanced the resistance to rape downy mildew virus, and found that the induced expression of this gene was closely related to the accumulation of salicylic acid. Sarowar et al. [41] transferred the pepper PR1 protein gene into tobacco. After overexpression of this gene, it not only enhanced the resistance to heavy metal stress but also enhanced the resistance to a variety of pathogens such as tobacco black shank fungus and wildfire fungus. In addition, we also found that plant PR1 protein genes are polygenic families, but not all PR1 genes have disease-resistance functions. Li et al. [42] found that only the basic protein VvPR1b1 gene in grapes has disease resistance function against tobacco wildfire pathogen; Bonasera et al. [43] transferred PR-1a, PR-1b. and PR-1c into apples and found that apple seedlings did not show disease resistance. In this study, GmPR1L was transferred into tobacco. Overexpression of this gene significantly enhanced the resistance of tobacco plants to Botrytis cinerea, which is similar to previous studies, and also shows that GmPR1L has a resistance function to fungal diseases.
When dealing with pathogen infection, overexpression of GmPR1L in tobacco can increase the activities of transgenic tobacco-related defense enzymes. When infected by B. cinerea, it quickly induces its own defense mechanism. It can not only limit the spread of pathogens but also further damage the structure of pathogens so that they lose pathogenic activity. From what has been discussed above, Overexpression of GmPR1L can improve the resistance of tobacco to B. cinerea. The study on the resistance of GmPR1L is of great significance for the development of disease-resistant transgenic soybean varieties.
Acknowledgement: We would like to thank Jilin Agricultural University, Plant Biotechnology Center for facilitating the working environment to conduct the experiment.
Funding Statement: This work was supported by Major Science and Technology Projects (20210302002NC), Jilin Province Science and Technology Development Plan Project, Grant Number 20190103120JH. Jilin Province Science and Technology Development Plan—Outstanding Young Talents Fund Project, Grant Number 20190103120J. The Fourth Batch of Jilin Province Youth Science and Technology Talent Support Project, Grant Number QT202020 and National Natural Science Foundation of China Projects, Grant Number 31801381.
Conflicts of Interest: The authors declare that they have no conflicts of interest to report regarding the present study.
References
1. Pečenková, T., Pleskot, R., Žárský, V. (2017). Subcellular localization of arabidopsis pathogenesis-related 1 (PR1) protein. International Journal of Molecular Sciences, 18(4), 825. https://doi.org/10.3390/ijms18040825 [Google Scholar] [PubMed] [CrossRef]
2. Almeida-Silva, F., Venancio, T. M. (2022). Pathogenesis-related protein 1 (PR-1) genes in soybean: Genome-wide identification, structural analysis and expression profiling under multiple biotic and abiotic stresses. Gene, 809(6), 146013. https://doi.org/10.1016/j.gene.2021.146013 [Google Scholar] [PubMed] [CrossRef]
3. Viktorova, J., Krasny, L., Kamlar, M., Novakova, M., Mackova, M. et al. (2012). Osmotin, a pathogenesis-related protein. Current Protein & Peptide Science, 13(7), 672–681. https://doi.org/10.2174/138920312804142129 [Google Scholar] [PubMed] [CrossRef]
4. Breen, S., Williams, S. J., Outram, M., Kobe, B., Solomon, P. S. (2017). Emerging insights into the functions of pathogenesis-related protein 1. Trends in Plant Science, 22(10), 871–879. https://doi.org/10.1016/j.tplants.2017.06.013 [Google Scholar] [PubMed] [CrossRef]
5. Wang, L., Lu, H., Zhan, J., Shang, Q., Wang, L. et al. (2022). Pathogenesis-related protein-4 (PR-4) gene family in Qingke (Hordeum vulgare L. var. nudumGenome-wide identification, structural analysis and expression profile under stresses. Molecular Biology Reports, 49(10), 9397–9408. https://doi.org/10.1007/s11033-022-07794-3 [Google Scholar] [PubMed] [CrossRef]
6. Sinha, M., Singh, R. P., Kushwaha, G. S., Iqbal, N., Singh, A. et al. (2014). Current overview of allergens of plant pathogenesis related protein families. The Scientific World Journal, 2014(9), 543195. https://doi.org/10.1155/2014/543195 [Google Scholar] [PubMed] [CrossRef]
7. Anuradha, C., Chandrasekar, A., Backiyarani, S., Thangavelu, R., Giribabu, P. et al. (2022). Genome-wide analysis of pathogenesis-related protein 1 (PR-1) gene family from Musa spp. and its role in defense response during stresses. Gene, 821(6), 146334. https://doi.org/10.1016/j.gene.2022.146334 [Google Scholar] [PubMed] [CrossRef]
8. Hong, J. K., Hwang, I. S., Hwang, B. K. (2017). Functional roles of the pepper leucine-rich repeat protein and its interactions with pathogenesis-related and hypersensitive-induced proteins in plant cell death and immunity. Planta, 246(3), 351–364. https://doi.org/10.1007/s00425-017-2709-5 [Google Scholar] [PubMed] [CrossRef]
9. Ali, S., Ganai, B. A., Kamili, A. N., Bhat, A. A., Mir, Z. A. et al. (2018). Pathogenesis-related proteins and peptides as promising tools for engineering plants with multiple stress tolerance. Microbiological Research, 212–213(12), 29–37. https://doi.org/10.1016/j.micres.2018.04.008 [Google Scholar] [PubMed] [CrossRef]
10. Zaynab, M., Peng, J., Sharif, Y., Al-Yahyai, R., Jamil, A. et al. (2021). Expression profiling of pathogenesis-related protein-1 (PR-1) genes from Solanum tuberosum reveals its critical role in phytophthora infestans infection. Microbial Pathogenesis, 161, 105290. https://doi.org/10.1016/j.micpath.2021.105290 [Google Scholar] [PubMed] [CrossRef]
11. Goyal, R. K., Fatima, T., Topuz, M., Bernadec, A., Sicher, R. et al. (2016). Pathogenesis-related protein 1b1 (PR1b1) is a major tomato fruit protein responsive to chilling temperature and upregulated in high polyamine transgenic genotypes. Frontiers in Plant Science, 7(21), 901. https://doi.org/10.3389/fpls.2016.00901 [Google Scholar] [PubMed] [CrossRef]
12. Shin, S. H., Pak, J. H., Kim, M. J., Kim, H. J., Oh, J. S. et al. (2014). An acidic PATHOGENESIS-RELATED1 gene of Oryza grandiglumis is involved in disease resistance response against bacterial infection. The Plant Pathology Journal, 30(2), 208–214. https://doi.org/10.5423/PPJ.NT.11.2013.0112 [Google Scholar] [PubMed] [CrossRef]
13. Gamir, J., Darwiche, R., van’t Hof, P., Choudhary, V., Stumpe, M. et al. (2017). The sterol-binding activity of PATHOGENESIS-RELATED PROTEIN 1 reveals the mode of action of an antimicrobial protein. The Plant Journal, 89(3), 502–509. https://doi.org/10.1111/tpj.13398 [Google Scholar] [PubMed] [CrossRef]
14. Chen, Y. L., Lee, C. Y., Cheng, K. T., Chang, W. H., Huang, R. N. et al. (2014). Quantitative peptidomics study reveals that a wound-induced peptide from PR-1 regulates immune signaling in tomato. The Plant Cell, 26(10), 4135–4148. https://doi.org/10.1105/tpc.114.131185 [Google Scholar] [PubMed] [CrossRef]
15. Zhou, Z., Zhu, Y., Tian, Y., Yao, J. L., Bian, S. et al. (2021). MdPR4, a pathogenesis-related protein in apple, is involved in chitin recognition and resistance response to apple replant disease pathogens. Journal of Plant Physiology, 260(7), 153390. https://doi.org/10.1016/j.jplph.2021.153390 [Google Scholar] [PubMed] [CrossRef]
16. Numan, M., Bukhari, S. A., Rehman, M. U., Mustafa, G., Sadia, B. (2021). Phylogenetic analyses, protein modeling and active site prediction of two pathogenesis related (PR2 and PR3) genes from bread wheat. PLoS One, 16(9), e0257392. https://doi.org/10.1371/journal.pone.0257392 [Google Scholar] [PubMed] [CrossRef]
17. Zribi, I., Ghorbel, M., Brini, F. (2021). Pathogenesis related proteins (PRsFrom cellular mechanisms to plant defense. Current Protein & Peptide Science, 22(5), 396–412. https://doi.org/10.2174/1389203721999201231212736 [Google Scholar] [PubMed] [CrossRef]
18. Peng, Q., Su, Y., Ling, H., Ahmad, W., Gao, S. et al. (2017). A sugarcane pathogenesis-related protein, ScPR10, plays a positive role in defense responses under Sporisorium scitamineum, SrMV, SA, and MeJA stresses. Plant Cell Reports, 36(9), 1427–1440. https://doi.org/10.1007/s00299-017-2166-4 [Google Scholar] [PubMed] [CrossRef]
19. Anisimova, O. K., Shchennikova, A. V., Kochieva, E. Z., Filyushin, M. A. (2021). Pathogenesis-related genes of PR1, PR2, PR4, and PR5 families are involved in the response to Fusarium infection in garlic (Allium sativum L.). International Journal of Molecular Sciences, 22(13), 6688. https://doi.org/10.3390/ijms22136688 [Google Scholar] [PubMed] [CrossRef]
20. Bozbuga, R. (2020). Expressions of Pathogenesis related 1 (PR1) gene in Solanum lycopersicum and influence of salicylic acid exposures on host-Meloidogyne incognita interactions. Doklady Biochemistry and Biophysics, 494(1), 266–269. https://doi.org/10.1134/S1607672920050038 [Google Scholar] [PubMed] [CrossRef]
21. Ghorbel, M., Zribi, I., Missaoui, K., Drira-Fakhfekh, M., Azzouzi, B. et al. (2021). Differential regulation of the durum wheat Pathogenesis-related protein (PR1) by Calmodulin TdCaM1.3 protein. Molecular Biology Reports, 48(1), 347–362. https://doi.org/10.1007/s11033-020-06053-7 [Google Scholar] [PubMed] [CrossRef]
22. Xie, Y. R., Chen, Z. Y., Brown, R. L., Bhatnagar, D. (2010). Expression and functional characterization of two pathogenesis-related protein 10 genes from Zea mays. Journal of Plant Physiology, 167(2), 121–130. https://doi.org/10.1016/j.jplph.2009.07.004 [Google Scholar] [PubMed] [CrossRef]
23. Hou, M., Xu, W., Bai, H., Liu, Y., Li, L. et al. (2012). Characteristic expression of rice pathogenesis-related proteins in rice leaves during interactions with Xanthomonas oryzae pv. oryzae. Plant Cell Reports, 31(5), 895–904. https://doi.org/10.1007/s00299-011-1210-z [Google Scholar] [PubMed] [CrossRef]
24. Xu, P., Jiang, L., Wu, J., Li, W., Fan, S. et al. (2014). Isolation and characterization of a pathogenesis-related protein 10 gene (GmPR10) with induced expression in soybean (Glycine max) during infection with Phytophthora sojae. Molecular Biology Reports, 41(8), 4899–4909. https://doi.org/10.1007/s11033-014-3356-6 [Google Scholar] [PubMed] [CrossRef]
25. Pape, S., Thurow, C., Gatz, C. (2010). The Arabidopsis PR-1 promoter contains multiple integration sites for the coactivator NPR1 and the repressor SNI1. Plant Physiology, 154(4), 1805–1818. https://doi.org/10.1104/pp.110.165563 [Google Scholar] [PubMed] [CrossRef]
26. Zhang, J., Wang, F., Liang, F., Zhang, Y., Ma, L. et al. (2018). Functional analysis of a pathogenesis-related thaumatin-like protein gene TaLr35PR5 from wheat induced by leaf rust fungus. BMC Plant Biology, 18(1), 76. https://doi.org/10.1186/s12870-018-1297-2 [Google Scholar] [PubMed] [CrossRef]
27. Naz, R., Bano, A., Wilson, N. L., Guest, D., Roberts, T. H. (2014). Pathogenesis-related protein expression in the apoplast of wheat leaves protected against leaf rust following application of plant extracts. Phytopathology, 104(9), 933–944. https://doi.org/10.1094/PHYTO-11-13-0317-R [Google Scholar] [PubMed] [CrossRef]
28. Tellis, M., Mathur, M., Gurjar, G., Kadoo, N., Gupta, V. (2017). Identification and functionality prediction of pathogenesis-related protein 1 from legume family. Proteins: Structure Function and Bioinformatics, 85(11), 2066–2080. https://doi.org/10.1002/prot.25361 [Google Scholar] [PubMed] [CrossRef]
29. Yasmin, N., Saleem, M. (2014). Biochemical characterization of fruit-specific pathogenesis-related antifungal protein from basrai banana. Microbiological Research, 169(5–6), 369–377. https://doi.org/10.1016/j.micres.2013.08.010 [Google Scholar] [PubMed] [CrossRef]
30. Lavrova, V. V., Zinovieva, S. V., Udalova, Z. V., Matveeva, E. M. (2017). Expression of PR genes in tomato tissues infected by nematode Meloidogyne incognita (Kofoid et White, 1919) Chitwood, 1949. Doklady Biochemistry and Biophysics, 476(1), 306–309. https://doi.org/10.1134/S1607672917050064 [Google Scholar] [PubMed] [CrossRef]
31. Bai, S., Dong, C., Li, B., Dai, H. (2013). A PR-4 gene identified from Malus domestica is involved in the defense responses against Botryosphaeria dothidea. Plant Physiology and Biochemistry, 62, 23–32. https://doi.org/10.1016/j.plaphy.2012.10.016 [Google Scholar] [PubMed] [CrossRef]
32. Sels, J., Mathys, J., de Coninck, B. M., Cammue, B. P., de Bolle, M. F. (2008). Plant pathogenesis-related (PR) proteins: A focus on PR peptides. Plant Physiology and Biochemistry, 46(11), 941–950. https://doi.org/10.1016/j.plaphy.2008.06.011 [Google Scholar] [PubMed] [CrossRef]
33. Akbudak, M. A., Yildiz, S., Filiz, E. (2020). Pathogenesis related protein-1 (PR-1) genes in tomato (Solanum lycopersicum L.Bioinformatics analyses and expression profiles in response to drought stress. Genomics, 112(6), 4089–4099. https://doi.org/10.1016/j.ygeno.2020.07.004 [Google Scholar] [PubMed] [CrossRef]
34. Liu, W. X., Zhang, F. C., Zhang, W. Z., Song, L. F., Wu, W. H. et al. (2013). Arabidopsis Di19 functions as a transcription factor and modulates PR1, PR2, and PR5 expression in response to drought stress. Molecular Plant, 6(5), 1487–1502. https://doi.org/10.1093/mp/sst031 [Google Scholar] [PubMed] [CrossRef]
35. Breen, S., Williams, S. J., Winterberg, B., Kobe, B., Solomon, P. S. (2016). Wheat PR-1 proteins are targeted by necrotrophic pathogen effector proteins. The Plant Journal, 88(1), 13–25. https://doi.org/10.1111/tpj.13228 [Google Scholar] [PubMed] [CrossRef]
36. Pereira Menezes, S., de Andrade Silva, E. M., Matos Lima, E., Oliveira de Sousa, A., Silva Andrade, B. et al. (2014). The pathogenesis-related protein PR-4b from Theobroma cacao presents RNase activity, Ca2+ and Mg2+ dependent-DNase activity and antifungal action on Moniliophthora perniciosa. BMC Plant Biology, 14(1), 161. https://doi.org/10.1186/1471-2229-14-161 [Google Scholar] [PubMed] [CrossRef]
37. Lu, S., Faris, J. D., Sherwood, R., Friesen, T. L., Edwards, M. C. (2014). A dimeric PR-1-type pathogenesis-related protein interacts with ToxA and potentially mediates ToxA-induced necrosis in sensitive wheat. Molecular Plant Pathology, 15(7), 650–663. https://doi.org/10.1111/mpp.12122 [Google Scholar] [PubMed] [CrossRef]
38. Zhang, W. J., Pedersen, C., Kwaaitaal, M., Gregersen, P. L., Mørch, S. M. et al. (2012). Interaction of barley powdery mildew effector candidate CSEP0055 with the defence protein PR17c. Molecular Plant Pathology, 13(9), 1110–1119. https://doi.org/10.1111/j.1364-3703.2012.00820.x [Google Scholar] [PubMed] [CrossRef]
39. Alexander, D., Goodman, R. M., Gut-Rella, M., Glascock, C., Weymann, K. et al. (1993). Increased tolerance to two oomycete pathogens in transgenic tobacco expressing pathogenesis-related protein 1a. Proceedings of the National Academy of Sciences of the United States of America, 90(15), 7327–7331. https://doi.org/10.1073/pnas.90.15.7327 [Google Scholar] [PubMed] [CrossRef]
40. Shin, R., An, J. M., Park, C. J., Kim, Y. J., Joo, S. et al. (2004). Capsicum annuum tobacco mosaic virus-induced clone 1 expression perturbation alters the plant’s response to ethylene and interferes with the redox homeostasis. Plant Physiology, 135(1), 561–573. https://doi.org/10.1104/pp.103.035436 [Google Scholar] [PubMed] [CrossRef]
41. Sarowar, S., Kim, Y. J., Kim, E. N., Kim, K. D., Hwang, B. K. et al. (2005). Overexpression of a pepper basic pathogenesis-related protein 1 gene in tobacco plants enhances resistance to heavy metal and pathogen stresses. Plant Cell Reports, 24(4), 216–224. https://doi.org/10.1007/s00299-005-0928-x [Google Scholar] [PubMed] [CrossRef]
42. Li, Z. T., Dhekney, S. A., Gray, D. J. (2011). PR-1 gene family of grapevine: A uniquely duplicated PR-1 gene from a Vitis interspecific hybrid confers high level resistance to bacterial disease in transgenic tobacco. Plant Cell Reports, 30(1), 1–11. https://doi.org/10.1007/s00299-010-0934-5 [Google Scholar] [PubMed] [CrossRef]
43. Bonasera, J. M., Kim, J. F., Beer, S. V. (2006). PR genes of apple: Identification and expression in response to elicitors and inoculation with Erwinia amylovora. BMC Plant Biology, 6(1), 23. https://doi.org/10.1186/1471-2229-6-23 [Google Scholar] [PubMed] [CrossRef]
Cite This Article
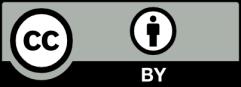