Open Access
ARTICLE
An in Vitro Approach to Investigate the Role of Abscisic Acid in Alleviating the Negative Effects of Chilling Stress on Banana Shoots
1
Pomology Department, Faculty of Agriculture, Cairo University, Giza, 2613, Egypt
2
College of Agriculture, Fujian Agriculture and Forestry University, Fuzhou, 350002, China
3
Institute of Field and Vegetable Crops, National Institute of the Republic of Serbia, Novi Sad, Serbia
* Corresponding Author: Ibrahim Hmmam. Email:
(This article belongs to the Special Issue: Physiological and Molecular Interventions in Improving Abiotic Stress Tolerance in Plants)
Phyton-International Journal of Experimental Botany 2023, 92(6), 1695-1711. https://doi.org/10.32604/phyton.2023.028317
Received 12 December 2022; Accepted 08 February 2023; Issue published 09 March 2023
Abstract
Banana is a tropical crop cultivated in warm places. Chilling stress in Egypt is making banana crops less productive. Abscisic acid (ABA), a key plant hormone, regulates metabolic and physiological processes and protects plants from a variety of stresses. In vitro growing banana shoots were pre-treated with ABA at four concentrations (0, 25, 50, and 100 mM) and chilled at 5°C for 24 h, followed by a six-day recovery period at 25°C. By comparing ABA treatments to both positive and negative controls, physiological and biochemical changes were investigated. Chilling stress (5°C) caused a considerable increase in lipid peroxidation and ion leakage and reduced photosynthetic pigments in cold-treated plantlets. Increasing the concentration of ABA to 100 µM enhanced the response to chilling stress. ABA had a major effect on mitigating chilling injury in banana shoots by keeping cell membranes stable and lowering the amount of ion leakage and lipid peroxidation. Also, ABA significantly maintained the photosynthetic pigment concentration of banana shoots; accumulated higher amounts of total soluble carbohydrates and proline; and increased DPPH radical scavenging activity. Furthermore, ABA treatment enhanced cold tolerance in chilling-stressed banana shoots through the regulation of antioxidant enzyme activity. Overall, the results show that ABA is a good choice for protecting banana shoots from the damage caused by chilling stress.Keywords
Banana (Musa sp., Musaceae family) is a valuable fruit crop worldwide. Bananas are the most widely grown fruit crop in the world, with an annual yield of more than 119 million tons [1]. Tropical and subtropical species, including bananas, are highly susceptible to chilling (0°C–5°C) temperatures [2].
Low temperatures have a negative impact on banana productivity in Mediterranean climates, and bananas are often damaged seriously by chilling during the winter season [3]. The damaging effects result in reduced growth, yields, and fruit quality, depending on the cold intensity and exposure time [4]. Changes in the weather, like high or low temperatures, have been linked to the environmental conditions that crops need to grow well [5]. Crop productivity could plummet by half because of unexpected weather conditions [6].
Recently, increasing attention has been paid to developing new agricultural practices that could prevent the negative effects of unexpected climate change on crop growth and productivity. Numerous attempts, such as modifying crop management practices and applying chemicals, have been made to increase chilling tolerance and avoid chilling injury [3,7,8].
Exposure to low temperatures induces oxidative stress through the accumulation of reactive oxygen species (ROS) [8,9]. Plants have evolved an efficient defense system against oxidative stress via enzymatic and non-enzymatic antioxidants [8–10]. Abscisic acid (ABA) is a key regulator of many aspects of plant growth and development in higher plants, such as the maturation of embryos, the dormancy of seeds, the development of flowers, and the growth of roots [11]. Abscisic acid is also a critical signal molecule in abiotic stress responses, affecting gene expression as well as physiological and biochemical reactions [12,13]. Plants produce more ABA when they are stressed, such as by heat [14], cold [15], or drought [16]. On the other hand, the ABA-deficient mutants of Arabidopsis were less freezing resistant than their wild types [17].
Previous studies showed that the increase in endogenous ABA content was closely related to the increase in chilling tolerance [18,19]. Exogenous application of ABA increased the chilling tolerance of different plant species, including Stylosanthes guianensis [10], Cucumis melo [20], and Zea mays [21]. ABA plays a major role in the stimulation of endogenous changes relating to cold acclimation [13,22]. It has been suggested that ABA could reduce chilling injury by suppressing ion leakage and lipid peroxidation [18] and increasing enzymatic or non-enzymatic antioxidant activity, which would increase ROS scavenging potential [19]. Furthermore, exogenous ABA application induces several biochemical changes, including an increased level of proline and soluble carbohydrates [23,24], reduces lipid peroxidation, alleviates cell membrane injury [24], and maintains photosynthetic activity [25].
In vitro-based techniques are a good approach for studying plant responses to various types of abiotic stress because they require little space, ensure uniformity of screening factors, and minimize the effect of environmental conditions [26–28]. In addition, in vitro evaluation is a relatively faster method compared with ex vitro evaluation [26]. Therefore, the main objective of the current study was to investigate the effect of different concentrations of ABA on the chilling tolerance of in vitro grown banana shoots.
2.1 Plant Materials and Culture Conditions
In vitro regenerated banana shoots (Musa sp., cv. Grand Naine, (AAA genome) were cultured on Murashige and Skoog (MS) basal medium [29] supplemented with 2.5 mg L–1 benzyl amino purine (BAP), 30 g L–1 sucrose, and 6 g L–1 agar. The pH was adjusted to 5.8 and the media were sterilized by autoclaving for 15 min at 121°C. The cultures were incubated in a growth chamber at 25°C ± 2°C under 4000 lux light intensity for 16 h light/8 h dark photoperiods supplied by a white cool fluorescent lamp.
2.2 Abscisic Acid (ABA) Treatments
Contamination-free shoots were transferred to MS medium supplemented with four levels of filtration sterilization (22 µ; Joanlab Equipment Co., Ltd., China) of ABA (0, 25, 50, and 100 µM) to investigate the role of cis, trans-Abscisic acid (Caisson Laboratory, Inc., Smithfield, Utah, USA) in mitigating the adverse effects of chilling stress on banana shoots. Each cluster with 3 shoots was sub-cultured into a 200 mL jar containing 50 mL of semi-solid medium (each treatment consisted of 20 jars) and incubated for one week at 25°C ± 2°C and 4000 lux light intensity (16 h light/8 h dark photoperiods) supplied by a white cool fluorescent lamp.
For chilling stress treatments, jars of each ABA treatment were incubated at 5°C for 24 h, followed by a period of six days of recovery at 25°C ± 2°C in growth chambers. After the recovery period, samples of all treatments were collected for measurements. The experiment included a comparative positive control (untreated plantlets that were exposed to chilling stress), while the negative control included untreated plantlets that were kept under growth chamber conditions (25°C ± 2°C) throughout the entire period of the experiment.
2.3.1 Ion Leakage and Membrane Stability Index
Ion leakage and membrane stability index (MSI) were determined according to Korkmaz et al. [30] with some modifications; 0.5 g of leaf discs from randomly chosen plants were collected and washed with distilled water to remove surface contamination with media residuals. The discs were placed in a 50 mL falcon tube containing 20 mL of deionized water (Aquinity2 P10, MembraPure GmbH, Germany), and incubated at room temperature for 24 h. The electrical conductivity (EC) of the solution (EC1) was determined using an electrical conductivity meter (BALRAMA, Digital EC Meter, India). The same samples were then placed in a boiling water bath for 20 min, and a second reading (EC2) was determined after cooling the solution to room temperature. The ion leakage was expressed as a percent value using the following formula:
The Membrane Stability Index (MSI) was calculated based on electrolyte leakage data and expressed as a percent value using the following formula:
Lipid peroxidation was determined as the amount of malondialdehyde (MDA) by the thiobarbituric acid (TBA) reaction method [31]. Leaf samples (0.5 g) were homogenized in 3 mL of 0.1% trichloroacetic acid (TCA) (w/v) and centrifuged at 15,000 g for 20 min. The supernatant (0.2 mL) was mixed with 1 mL of 20% TCA containing 0.5% (w/v) TBA. The mixture was heated at 95°C for 30 min before being quickly cooled on ice. The absorbance of the supernatant was recorded at 534 nm using a spectrophotometer (Sunostk-SBA-733PLUS).
2.3.3 Photosynthetic Pigments Concentration
Chlorophyll pigments were determined according to Lichtenthaler et al. [32]. Banana leaf samples (0.25 g) from randomly selected three plants per replicate were homogenized in 20 mL of 80% (v/v) acetone (LOBA Chemie PVT. Ltd., Mumbai, India). The absorbance was measured with a spectrophotometer (JENWAY, Model 6300 Instruments, Staffordshire, UK), at 663 and 646 nm, and chlorophyll concentrations were calculated using the following equations:
2.3.4 Total Soluble Sugars Concentration
Total soluble sugars concentration was determined by the phenol-sulfuric acid method [33]. A 0.25 g leaf sample was homogenized in 20 mL of 70% ethanol (Chem-Lab., Zedelgem, Belgium). One milliliter of ethanolic extract was treated with 1 mL of 5% (w/v) phenol (LOBA Chemie PVT. Ltd., Mumbai, India) followed by the addition of 5 mL of 98% sulfuric acid (Adwic Pharmaceutical Co., Cairo, Egypt). Absorbance was read at 490 nm using a spectrophotometer (JENWAY, Model 6300, Staffordshire, UK). A standard curve was generated using a pure glucose solution and total sugars concentration was expressed as mg glucose equivalent g–1 of fresh weight.
Proline concentration was determined using Bates’s method [34]; 0.5 g of leaf sample was homogenized in 10 mL of 3% aqueous sulfosalicylic acid (LOBA Chemie PVT. Ltd., Mumbai, India). Two milliliters of the filtrate were mixed with 2 mL of freshly prepared acid ninhydrin (Research Lab. Fine Chem. Industries, Mumbai, India) and 2 mL of glacial acetic acid (Merck-Darmstadt, Germany) and placed in a boiling water bath for one hour. The developed color was extracted with 4 mL toluene (Bio Basic Inc., Markham, Canada) and the absorbance was determined spectrophotometrically at 520 nm. The proline concentration was determined from a standard curve using pure proline (Caisson Laboratory, Inc., Smithfield, Utah, USA) and calculated on a fresh weight basis as follows:
2.3.6 Total Phenolic Concentration
A fresh leaf sample (0.25 g) was homogenized with 20 mL of methanol (Chem-Lab., Zedelgem, Belgium) and then used to determine the total phenolic concentration and DPPH scavenging capacity. Total phenols were determined spectrophotometrically using the modified Folin–Ciocalteu colorimetric method [35]. The methanolic extract (1 mL) was mixed with 1 mL of Folin–Ciocalteu reagent (LOBA Chemie PVT. Ltd., Mumbai, India) and allowed to stand for 6 min, followed by the addition of 4 mL of 1 M sodium carbonate (LOBA Chemie PVT. Ltd., Mumbai, India) and 3 mL of distilled water in a test tube. Samples were incubated for 90 min at room temperature in darkness, and absorbance was measured spectrophotometrically at 760 nm. The results were expressed as mg gallic acid equivalent g–1 of fresh weight.
2.3.7 DPPH Free Radical Scavenging Assay
The antioxidant activity was evaluated by the free radical scavenging method [36] using 1, 1-diphenyl-2-picrylhydrazyl (DPPH) (Sigma-Aldrich, Darmstadt, Germany). 1.6 mL of methanolic extracts were mixed with 2.4 mL of 0.004% DPPH (w/v in methanol). Then, the reaction mixture was vortexed and incubated in darkness at room temperature for a period of 30 min. Finally, the optical density of all samples was measured at 517 nm wavelength using a spectrophotometer. The DPPH radical scavenging activity was calculated by the following equation:
2.3.8 Extraction and Determination of Antioxidant Enzymes Activity
The crude enzyme extracts were prepared by grinding 0.5 g of fresh leaf samples in 10 mL of phosphate buffer pH 6.8 (0.1 M), then centrifuged at 4°C for 30 min at 12000 g in a refrigerated centrifuge. The supernatant was used as a crude enzyme extract.
Catalase (CAT, E.C.1.11.1.6) activity was determined by monitoring the decomposition of H2O2 using the method of Aebi [37]. The reaction mixture consisted of 1 mL of phosphate buffer (100 mM, pH 7.00), 1 mL of double-distilled water, and 0.2 mL of crude enzyme. The reaction was initiated by adding 0.1 mL of H2O2 (500 mM). The mixture was incubated at 37°C for 10 min. The decomposition of H2O2 was followed directly by the decrease in absorbance at 240 nm by the SBA-733PLUS (Sunostik Medical Technology Co., Ltd., China) spectrophotometer. The blank was made by using buffer instead of the enzyme extract. The results of CAT activity are expressed in units (U) g–1 FW.
Glutathione peroxidase (GPX, E.C.1.11.1.9) was assayed according to Paglia et al. [38] using a commercial enzyme kit. The reaction mixture contained 1 mL of 50 mM phosphate buffer (pH 7.0), 24 mol glutathione, 12 units of glutathione reductase, 4.8 µmol NADPH, and 0.1 mL of enzyme extract (dH2O as control). After incubating for 10 min at 37°C, GPX activity was measured using the SBA-733PLUS (Sunostik Medical Technology Co., Ltd., China) spectrophotometer for 3 min at 30 s intervals against deionized water. The results are expressed as U/g–1 FW.
Superoxide dismutase (SOD, E.C.1.15.1.1) was assayed by measuring its ability to inhibit the photo-reduction of nitro blue tetrazolium [39]. The reaction mixture consisted of 1 mL of phosphate buffer (50 mM, pH 8.3), 0.1 mL of phenazine methosulphate, 0.1 mL of nitroblue tetrazolium (1 mM), 0.1 mL of NADH (1 mM), 0.1 mL of enzyme extract, and 0.1 mL ddH2O. The reaction mixture was incubated at 30°C for 90 s, then 1 mL of glacial acetic acid was added to stop the reaction. The reaction solution was measured at 560 nm by SBA-733PLUS (Sunostik Medical Technology Co., Ltd., China) spectrophotometer against the phosphate buffer as a control. SOD activity was expressed in units (U) g–1 FW.
The experiment was carried out in a completely randomized design with three replications. The model assumptions of normality were tested using Shapiro–Wilk’s test [40] before performing the analysis of variance [41]. Analysis of variance was performed using MSTAT-C statistical package software. The mean and standard deviation (± SD) were calculated from three replicates per treatment, and the significant differences between treatments were assessed by means of the Duncan multiple range test at a significance level of 0.01 [42]. A hierarchical cluster analysis between the studied variables was performed and displayed in a heatmap using the ClustVis online tool [43].
3.1 Ion Leakage and Membrane Stability Index
Both chilling stress and ABA treatment had a significant effect (p ≤ 0.01) on ion leakage and cellular membrane stability of in vitro banana shoots. Chilling stress resulted in significant increases in ion leakage and a decreased membrane stability index of the chilling-stressed plantlets (Figs. 1A, 1B); banana shoots exposed to 5°C recorded a 3.54-fold increase in ion leakage than non-chilling-treated plants (negative control). The application of ABA significantly reduced the ion leakage in chilling-treated plants and maintained the membrane stability of banana shoots.
Figure 1: Impact of ABA concentrations on the ion leakage (A) and the membrane stability index (B) of chilled banana shoots. All results are expressed as means ± deviation (represented by vertical bars). Means with the same letters are not significantly different at p ≤ 0.01
3.2 MDA Level in Banana Shoots under Chilling Stress
The data illustrated in Fig. 2 showed the effect of ABA treatment on MDA levels in banana shoots growing under chilling stress compared to controls; the obtained results indicated that chilling stress significantly increased MDA concentration in banana shoots. Damage to cellular membrane lipids estimated as MDA concentration was significantly increased in response to chilling stress. The chilled banana shoots accumulated a 4.20-fold increase in MDA compared with the negative control treatment (Fig. 2). MDA concentration was significantly reduced with increasing ABA concentration in the growth media.
Figure 2: Impact of ABA concentrations on the MDA of chilled banana shoots. All results are expressed as means ± deviation (represented by vertical bars). Means with the same letters are not significantly different at p ≤ 0.01
3.3 Photosynthetic Pigments Concentration
Stable photosynthesis activity has a vital role in maintaining plant growth under stress conditions. Low-temperature stress and ABA treatments had a significant effect on chlorophyll concentration (Fig. 3). Chilling stress-induced degradation of chlorophyll concentration in banana shoots; chlorophyll a, b, and total chlorophyll concentration s of the chilling stressed plants without ABA treatment were significantly lower than those of the negative control plants (25°C).
Figure 3: Impact of ABA concentrations on the photosynthetic pigments (chlorophyll a, b, and total) concentration of chilled banana shoots. All results are expressed as means ± deviation (represented by vertical bars). Means with the same letters are not significantly different at p ≤ 0.01
3.4 Total Soluble Sugars Concentration
The effects of chilling stress and ABA treatments on soluble sugars concentration are summarized in Fig. 4. Overall, total sugars concentration was higher in chilled plantlets compared to those kept at 25°C; soluble sugars concentration increased significantly during the recovery period in shoots cultured on a medium supplemented with ABA compared to control shoots. The total soluble sugars concentration of ABA treatment increased significantly under chilling stress (5°C); total soluble sugars increased approximately by 23.3%, 75.7%, and 42.7% for ABA treatment at 25, 50, and 100 µM, respectively compared to the banana shoots kept at 25°C (Fig. 4).
Figure 4: Impact of ABA concentrations on the total soluble sugars concentration of chilled banana shoots. All results are expressed as means ± deviation (represented by vertical bars). Means with the same letters are not significantly different at p ≤ 0.01
The data illustrated in Fig. 5 showed that irrespective of ABA treatment, proline concentration in chilling-stressed plants was higher than in non-treated plants. The effect of ABA treatments on proline concentration was significant (p ≤ 0.01); banana shoots growing in media supplemented with ABA at 25 or 50 µM showed a slight but non-significant increase in proline concentration compared with chilled non-treated plantlets (positive control), while a marked increase in proline concentration was observed in ABA at 100 µM, which was around 59% higher than non-ABA-treated plants (Fig. 5).
Figure 5: Impact of ABA concentrations on the proline concentration of chilled banana shoots. All results are expressed as means ± deviation (represented by vertical bars). Means with the same letters are not significantly different at p ≤ 0.01
3.6 Total Phenolic Concentration
The effects of exogenous ABA on total phenolic concentration in banana shoots under low-temperature stress are presented in Fig. 6. ABA treatment and temperature regimes had a significant effect (p ≤ 0.01) on total phenolic concentration. Statistical analysis revealed a considerable increase in phenolic concentration in the leaves of chilling stressed banana shoots (positive control). The application of ABA significantly reduced the phenolic concentration in chilling-treated plants; phenolic concentration was gradually reduced with increasing ABA concentration in the growth media. There were no significant differences in total phenolic concentration between negative control treatment and ABA at 25 µM, while there was a sharp decrease in the phenolic concentration of ABA treatments at 50 and 100 µM (Fig. 6).
Figure 6: Impact of ABA concentrations on the total phenolic concentration of chilled banana shoots. All results are expressed as means ± deviation (represented by vertical bars). Means with the same letters are not significantly different at p ≤ 0.01
3.7 DPPH Radical Scavenging Activity
Total antioxidant activity of leaf tissue, as measured by the DPPH method, was significantly affected by cold stress and ABA treatments (Fig. 7). The lowest value was recorded for the plantlets growing at ambient temperature (negative control). Cold treatments led to a significant increase in DPPH value. There was a significantly higher level of DPPH-radical scavenging activity in ABA-treated plantlets compared with the non-chilled plantlets (Fig. 7). ABA-treated banana shoots maintained a higher DPPH radical scavenging rate compared with the control treatments.
Figure 7: Impact of ABA concentrations on the DPPH radical scavenging activity of chilled banana shoots. All results are expressed as means ± deviation (represented by vertical bars). Means with the same letters are not significantly different at p ≤ 0.01
3.8 Antioxidant Enzymes Activity
The activities of antioxidant enzymes, including SOD, CAT, and GPX, were determined to assess the role of ABA in the regulation of enzyme activity under chilling stress (Figs. 8A–8C).
Figure 8: Impact of ABA concentrations on the superoxide dismutase (A), catalase (B), and glutathione peroxidase (C) activities of chilled banana shoots. All results are expressed as means ± deviation (represented by vertical bars). Means with the same letters are not significantly different at p ≤ 0.01
SOD activity showed a marked increase in chilling-stressed plantlets (Fig. 8A). The SOD activity of chilling-stressed plantlets (positive control) was lower than that in ABA-treated plantlets. SOD activity in ABA-pretreated was 6.67% (ABA 25 µM) to 11.6% (ABA 100 µM) higher than in non-treated plantlets. Chilling stress caused a remarkable increase in CAT enzyme activities in chilling-stressed plantlets compared with the negative control (Fig. 8B). CAT activity was significantly influenced by ABA; a sharp increase in CAT activity was recorded for ABA-treated plantlets. Without ABA treatment, the CAT of the chilling-stressed plantlets was much lower than that of the negative control plantlets (25°C). In contrast, the GPX activity followed the opposite trend to that of the SOD and CAT. GPX activity was significantly higher in non-stressed seedlings. In the absence of ABA, chilling significantly reduced the activity of GPX (Fig. 8C). GPX activity was much higher in plantlets that had been treated with ABA than in plantlets that had not been treated (positive control).
3.9 Hierarchical Clustering with Heatmap
The hierarchical clustering heatmap showed the trend of measured parameters under chilling and ABA treatments. Based on the observed variations, the measured parameters were grouped into three column clusters (Fig. 9).
Figure 9: Hierarchical clustering with a heatmap illustrating the ABA-variable relationships under chilling stress. Each column represents a trait, whereas each row represents a treatment. The red color represents a higher relative level, and the blue color represents a lower relative level. Three distinct clusters were identified at the variable level. The variables included ion leakage, membrane stability index (MSI),DPPH, superoxide dismutase (SOD), catalase (CAT), glutathione peroxidase (GPX), malondialdehyde (MDA), chlorophyll a (Chl. a), chlorophyll b (Chl. b), total chlorophyll (total chl.), proline, total soluble sugar (TSS), and total phenolic concentration (TPhC)
Cluster-A includes MDA and ion leakage; in comparison with negative control treatment, parameters of cluster-A increased in chilling-stressed plants, while a decreasing trend was observed in ABA-treated plantlets compared with chilling-stressed only. The total sugars, DPPH, and SOD levels were grouped in cluster B. Compared with plantlets of both negative and positive control treatment; cluster-B parameters showed an increasing pattern in ABA-treated plants under chilling stress. Cluster-C comprises MSI, total phenols, proline, photosynthetic pigment (Chl. a, Chl. b, and total chl.), CAT, and GPX, which was slightly decreased in chilling-stressed plants compared with the negative control.
Moreover, these parameters showed a slight increase in ABA-treated chilling-stressed plants compared with chilling-stressed plants; only MSI of the ABA-treated plants showed a marked increase.
The application of ABA significantly reduced the ion leakage in chilling-treated plants; ABA treatment markedly inhibited the increases in ion leakage induced by chilling stress and maintained the membrane stability of banana shoots. The minimum ion leakage percentage was observed in the non-chilling treatment followed by ABA at 50 and 100 µM, which was 35% less than the positive control. The obtained results clearly indicated the protective role of ABA in maintaining membrane stability; the higher dose of ABA (50 and 100 µM) was more efficient in mitigating the stress by reducing ion leakage. It is well known that chilling stress induces oxidative damage, which results in cellular membrane injury and an increase in electrolyte leakage [17,44].
The results also indicated that exogenous ABA could effectively prevent the increase in MDA concentration under chilling stress. MDA concentration was significantly reduced with increasing ABA concentration in the growth media. Cold stress leads to an over-accumulation of ROS in plant tissues, which causes cellular damage [44,45]. Our investigation confirms the previous report that chilling stress increases the levels of MDA because of oxidative damage to cellular lipids under chilling stress [44]. Exogenous ABA enhanced chilling tolerance by suppressing the accumulation of MDA in ABA-treated plantlets under chilling stress [23].
Abscisic acid treatment had a significant effect on leaf chlorophyll concentration, as leaf chlorophyll concentration was higher in ABA-treated shoots than in control shoots. ABA treatments reduced the negative effect of chilling stress and maintained relatively high chlorophyll concentration in banana shoots. The lowest value of total chlorophyll concentration was recorded with the positive control treatment, while plantlets treated with a higher concentration of ABA recorded higher values of total chlorophyll concentration. Chlorophyll degradation affects plant photosynthetic activity under cold stress [46]. The obtained results agree with previously published research showing a decline in chlorophyll concentration following exposure to chilling-stress conditions [46,47]. ABA-treated banana shoots exhibited lower chlorophyll degradation than untreated ones, which may be explained by the higher activities of the ROS-scavenging system and the accumulation of different osmolytes measured in the current study (proline and soluble sugars), which protected the photosynthetic apparatus from oxidative stress [48].
Moreover, sugar accumulation at cold temperatures has been widely documented as a cryo-protectants in different plant species [49]. Soluble sugars act as osmo-regulators and protect cell membranes from chilling damage through their ability to lower the freezing point of plant tissues [50,51]. The obtained results showed that abscisic acid treatment had a significant effect on the leaf soluble sugars concentration of bananas growing under cold stress conditions, as leaf soluble sugars concentration was greater in ABA-treated shoots than in control shoots; previous results showed that exogenous abscisic acid application increased soluble sugars in cucumber and chickpea seedlings growing under low temperatures [23,52]. Soluble sugars had a positive role in membrane preservation via linking with the phospholipids and consequently increased cell membrane stability during exposure to cold stress [53,54].
Furthermore, our results confirm that the higher proline concentration of low-temperature stressed plants plays a vital role in conferring chilling tolerance [55]. ABA-induced proline accumulation appears to depend on the activation of genes and/or protein synthesis [56]. It is proposed that the expression of Δ1-pyrroline-5-carboxylate synthetase gene in A. thaliana is induced via ABA-biosynthesis-dependent and -independent pathways [57]. ABA is intimately involved in the processes that trigger chilling resistance through the accumulation of proline [58]. Plant cells accumulate proline under cold temperature conditions, which regulates osmotic potential and partially protects plant cells from dehydration stresses and maintains membrane stability [59].
Phenolic compounds are an important component of the non-enzymatic antioxidant defense system that provides protection against oxidative stress [60]. An increase in phenolic compounds is associated with exposure to stress conditions [54,61]. Phenolic compounds accumulation under cold stress has been confirmed in several plant species [62,63]. It is assumed that phenolic compounds increase membrane integrity, regulate cellular osmosis, scavenge ROS, and inhibit lipid peroxidation under stress conditions [64,65]. The antioxidant activity of phenolic compounds is mainly due to their redox potential, which plays an important role in decomposing free radicals and inhibiting lipid peroxidation [66].
DPPH radical scavenging activity was used to determine the total antioxidant capacity. Total antioxidant capacity plays an important role in the plant’s defense system against stress conditions. It protects the plants from oxidative damage by scavenging reactive oxygen species [67]. The higher levels of DPPH radical scavenging activity have been correlated with enhanced stress tolerance in different plant species [68,69]. DPPH-radical scavenging activity represents the potential activity of the non-enzymatic antioxidants in plants such as ascorbic acid, phenols, flavonoids, polysaccharides, proline, and glycine betaine [70]. Our data showed that DPPH-radical scavenging was improved with ABA treatment under low-temperature stress (Fig. 7). It may be considered that ABA increased chilling tolerance through the activation of the DPPH-radical scavenging system. Kang et al. [69] found a link between a higher chilling tolerance and a higher level of DPPH-radical scavenging activity.
The damage caused by chilling stress is associated with the accumulation of reactive oxygen species (ROS) at the cellular level [44]. ROS are highly toxic and cause damage to proteins, lipids, enzymes, DNAs, and proteins [44,71–73]. To cope with chilling stress, higher plants have evolved active ROS scavenging systems, consisting of antioxidant enzymes, such as superoxide dismutase (SOD), ascorbate peroxidase (APX), glutathione peroxidase (GPX), and catalase (CAT), that protect plants against oxidative stress damage [8,44,45,72,73]. SOD is the first detoxification enzyme and the most effective antioxidant in the cell. It catalyzes the formation of H2O2 and O2 from superoxide anion (•O2−). CAT is an antioxidant enzyme found in nearly all biological tissues that use oxygen. The enzyme employs iron or manganese as a cofactor and catalyzes the conversion or degradation of hydrogen peroxide (H2O2) to water and molecular oxygen, completing the detoxification process started by SOD. GPx is an essential enzyme that transforms hydrogen peroxide (H2O2) to water and lipid peroxides to their related alcohols, primarily in the mitochondria but also occasionally in the cytosol. In most cases, selenium, a micronutrient cofactor, is required for its function. For this reason, GPx is often considered a selenocysteine peroxidase. Also, GPx regulate plant growth and development, as well as responses to abiotic stressors such as intense light, high temperature, flood, and salt with an influence of both duration and intensity of the stress. As a result, a scavenging system might be quite active [74,75]. Moreover, ABA application led to enhanced activity of antioxidant enzymes (superoxide dismutase and catalase) in ABA-treated plants [44,76,77]. This could be because ABA can slow the breakdown of pigments used in photosynthesis, reduce ion leakage and lipid peroxidation, and speed up enzymes. Our research helps shed light on the problem of chilling stress, which has become a problem in many places around the world, especially Egypt, over the past few years and is a threat to many plants that come from tropical and subtropical areas.
In conclusion, the exogenous application of ABA effectively ameliorated the chilling injury of banana shoots exposed to chilling stress. Generally, the enhancement in susceptibility to chilling stress improved with increasing ABA concentration up to 100 µM. The effect of ABA treatment on chilling injury could be due to the ability of ABA to reduce the degradation of photosynthetic pigments, reduce ion leakage and lipid peroxidation, activate the enzymes (CAT, SOD, and GPX), DPPH scavenging capacity, and promote the accumulation of osmolytes (total soluble sugars and proline). In conclusion, this work helps to solve global climate change concerns, such as chilling stress, which has emerged in recent years, particularly in Egypt.
Acknowledgement: The authors would like to express their gratitude to the Science and Technology Development Fund (STDF) for the financial support, and the Faculty of Agriculture, Cairo University for all facilities during this study.
Funding Statement: This research was funded and supported by a research grant from the Science, Technology, and Innovation Funding Authority (STIFA), under STDF-RG, No. 35967.
Author Contributions: The authors confirm contribution to the paper as follows: conception and design, I.H.; methodology, A.A.; investigation, I.H., N.K., A.A.; resources, I.H., A.A.; data collection, N.K., A.A.; analysis and interpretation of results, I.H., A.R., A.A.; draft manuscript preparation, A.A.; writing-review and editing, I.H., A.R., I.D.; validation, N.K.; supervision, I.H.; project administration, I.H.; funding acquisition, I.H. All authors reviewed the results and approved the final version of the manuscript.
Conflicts of Interest: The authors declare that they have no conflicts of interest to report regarding the present study.
References
1. FAOSTAT, Food and Agriculture Organization of the United Nations (2023). https://www.fao.org/faostat/en/#data/QCL. [Google Scholar]
2. Ravi, I., Vaganan, M. M. (2016). Abiotic stress tolerance in banana. In: Rao, N., Shivashankara, K., Laxman, R. (Eds.Abiotic stress physiology of horticultural crops, pp. 207–222. New Delhi: Springer.https://doi.org/10.1007/978-81-322-2725-0_12 [Google Scholar] [CrossRef]
3. Kang, G., Wang, C., Sun, G., Wang, Z. (2003). Salicylic acid changes activities of H2O2-metabolizing enzymes and increases the chilling tolerance of banana seedlings. Environmental and Experimental Botany, 50(1), 9–15. https://doi.org/10.1016/S0098-8472(02)00109-0 [Google Scholar] [CrossRef]
4. Saltveit, M. E., Morris, L. L. (1990). Overview on chilling injury of horticultural crops. In: Wang, C. Y. (Ed.Chilling injury of horticultural crops, pp. 3–15. Boca Raton: CRC Press. [Google Scholar]
5. Rosenzweig, C., Iglesius, A., Yang, X. B., Epstein, P. R., Chivian, E. (2001). Climate change and extreme weather events-Implications for food production, plant diseases, and pests. Global Change Human Health, 2, 90–104. [Google Scholar]
6. Schlenker, W., Lobell, D. B. (2010). Robust negative impacts of climate change on African agriculture. Environmental Research Letters, 5(1), 014010. https://doi.org/10.1088/1748-9326/5/1/014010 [Google Scholar] [CrossRef]
7. Hmmam, I., Ali, A. E. M., Saleh, S. M., Khedr, N., Abdellatif, A. (2022). The role of salicylic acid in mitigating the adverse effects of chilling stress on “Seddik” mango transplants. Agronomy, 12(6), 1369. https://doi.org/10.3390/agronomy12061369 [Google Scholar] [CrossRef]
8. Raza, A., Su, W., Jia, Z., Luo, D., Zhang, Y. et al. (2022). Mechanistic insights into trehalose-mediated cold stress tolerance in rapeseed (Brassica napus L.) seedlings. Frontiers in Plant Science, 13, 857980. https://doi.org/10.3389/fpls.2022.857980 [Google Scholar] [PubMed] [CrossRef]
9. Raza, A., Salehi, H., Rahman, M. A., Zahid, Z., Madadkar, H. M. et al. (2022). Plant hormones and neurotransmitter interactions mediate antioxidant defenses under induced oxidative stress in plants. Frontiers in Plant Science, 13, 961872. https://doi.org/10.3389/fpls.2022.961872 [Google Scholar] [PubMed] [CrossRef]
10. Lei, Y., He, H., Raza, A., Liu, Z., Xiaoyu, D. et al. (2022). Exogenous melatonin confers cold tolerance in rapeseed (Brassica napus L.) seedlings by improving antioxidants and genes expression. Plant Signaling & Behavior, 17(1), 2129289. https://doi.org/10.1080/15592324.2022.2129289 [Google Scholar] [PubMed] [CrossRef]
11. Finkelstein, R. (2013). Abscisic acid synthesis and response. The Arabidopsis Book, 11, e0166. https://doi.org/10.1199/tab.0166 [Google Scholar] [PubMed] [CrossRef]
12. Tuteja, N. (2007). Abscisic acid and abiotic stress signaling. Plant Signaling and Behavior, 2(3), 135–138. https://doi.org/10.4161/psb.2.3.4156 [Google Scholar] [PubMed] [CrossRef]
13. An, J. P., Xu, R. R., Liu, X., Su, L., Yang, K. et al. (2022). Abscisic acid insensitive 4 interacts with ICE1 and JAZ proteins to regulate ABA signaling-mediated cold tolerance in apple. Journal of Experimental Botany, 73(3), 980–997. https://doi.org/10.1093/jxb/erab433 [Google Scholar] [PubMed] [CrossRef]
14. Sun, P., Chen, Q., Luo, S., Yu, X., Zhang, X. et al. (2022). The role and mechanism of abscisic acid in mitigating the adverse impacts of high temperature in Gracilariopsis lemaneiformis. Journal of Applied Phycology, 34(2), 1073–1087. https://doi.org/10.1007/s10811-021-02606-w [Google Scholar] [CrossRef]
15. Janowiak, F., Maas, B., Dörffling, K. (2002). Importance of abscisic acid for chilling tolerance of maize seedlings. Journal of Plant Physiology, 159(6), 635–643. https://doi.org/10.1078/0176-1617-0638 [Google Scholar] [CrossRef]
16. Ali, S., Hayat, K., Iqbal, A., Xie, L. (2020). Implications of abscisic acid in the drought stress tolerance of plants. Agronomy, 10(9), 1323. https://doi.org/10.3390/agronomy10091323 [Google Scholar] [CrossRef]
17. Llorente, F., Oliveros, J. C., Martínez-Zapater, J. M., Salinas, J. A. (2000). Freezing-sensitive mutant of Arabidopsis, frs1, is a new aba3 allele. Planta, 211(5), 648–655. https://doi.org/10.1007/s004250000340 [Google Scholar] [PubMed] [CrossRef]
18. Liu, Y., Jiang, H., Zhao, Z., An, L. (2011). Abscisic acid is involved in brassinosteroids-induced chilling tolerance in the suspension cultured cells from Chorispora bungeana. Journal of Plant Physiology, 168(9), 853–862. [Google Scholar] [PubMed]
19. Yu, J., Cang, J., Lu, Q., Fan, B., Xu, Q. et al. (2020). ABA enhanced cold tolerance of wheat ‘dn1’ via increasing ROS scavenging system. Plant Signaling and Behavior, 15(8), 1780403. https://doi.org/10.1080/15592324.2020.1780403 [Google Scholar] [PubMed] [CrossRef]
20. Kim, Y. H., Choi, K. I., Khan, A. L., Waqas, M., Lee, I. J. (2016). Exogenous application of abscisic acid regulates endogenous gibberellins homeostasis and enhances resistance of oriental melon (Cucumis melo var. L.) against low temperature. Scientia Horticulturae, 207, 41–47. https://doi.org/10.1016/j.scienta.2016.05.009 [Google Scholar] [CrossRef]
21. Tian, L., Li, J. (2018). The effects of exogenous ABA applied to maize (Zea mays L.) roots on plant responses to chilling stress. Acta Physiologiae Plantarum, 40(4), 1–13. https://doi.org/10.1007/s11738-018-2655-2 [Google Scholar] [CrossRef]
22. Gusta, L. V., Trischuk, R., Weiser, C. J. (2005). Plant cold acclimation: The role of abscisic acid. Journal of Plant Growth Regulation, 24(4), 308–331. https://doi.org/10.1007/s00344-005-0079-x [Google Scholar] [CrossRef]
23. Nayyar, H., Bains, T. S., Kumar, S. (2005). Chilling stressed chickpea seedlings: Effect of cold acclimation, calcium and abscisic acid on cryoprotective solutes and oxidative damage. Environmental and Experimental Botany, 54(3), 275–285. https://doi.org/10.1016/j.envexpbot.2004.09.007 [Google Scholar] [CrossRef]
24. Huang, X., Chen, M. H., Yang, L. T., Li, Y. R., Wu, J. M. (2015). Effects of exogenous abscisic acid on cell membrane and endogenous hormone contents in leaves of sugarcane seedlings under cold stress. Sugar Tech, 17(1), 59–64. https://doi.org/10.1007/s12355-014-0343-0 [Google Scholar] [CrossRef]
25. He, H. Y., Xue, L., Tian, L. P., Chen, Y. L. (2008). Effect of low-temperature stress on the chlorophyll contents and chlorophyll fluorescence parameters in muskmelon seedling leaves. Northern Horticulture, 4, 121–127. [Google Scholar]
26. Pérez-Tornero, O., Tallón, C. I., Porras, I., Navarro, J. M. (2009). Physiological and growth changes in micropropagated Citrus macrophylla explants due to salinity. Journal of Plant Physiology, 166(17), 1923–1933. https://doi.org/10.1016/j.jplph.2009.06.009 [Google Scholar] [PubMed] [CrossRef]
27. Pérez-Clemente, R. M., Gómez-Cadenas, A. (2012). In vitro tissue culture, a tool for the study and breeding of plants subjected to abiotic stress conditions. In: Leva, A., Rinaldi, L. (Eds.Recent advances in plant in vitro culture, pp. 91–108. London: Intech Open. https://doi.org/10.5772/50671 [Google Scholar] [CrossRef]
28. Kruglova, N. N., Seldimirova, O. A., Zinatullina, A. E. (2018). In vitro callus as a model system for the study of plant stress-resistance to abiotic factors (on the example of cereals). Biology Bulletin Reviews, 8(6), 518–526. https://doi.org/10.1134/S2079086418060063 [Google Scholar] [CrossRef]
29. Murashige, T., Skoog, F. (1962). A revised medium for rapid growth and bioassays with tobacco tissue cultures. Physiologia Plantarum, 15, 473–497. [Google Scholar]
30. Korkmaz, A., Uzunlu, M., Demirkiran, A. R. (2007). Acetyl salicylic acid alleviates chilling-induced damage in muskmelon seedlings. Canadian Journal of Plant Science, 87(3), 581–585. https://doi.org/10.4141/CJPS06035 [Google Scholar] [CrossRef]
31. Heath, R. L., Packer, L. (1968). Photoperoxidation in isolated chloroplast. I. Kinetics and stoichiometry of fatty acid peroxidation. Archives of Biochemistry and Biophysics, 125(1), 189–198. https://doi.org/10.1016/0003-9861(68)90654-1 [Google Scholar] [PubMed] [CrossRef]
32. Lichtenthaler, H. K., Wellburn, A. R. (1983). Determinations of total carotenoids and chlorophylls a and b of leaf extracts in different solvents. Biochemical Society Transactions, 11(5), 591–592. https://doi.org/10.1042/bst0110591 [Google Scholar] [CrossRef]
33. Dubois, M., Gilles, K. A., Hamilton, J. K., Rebers, P. T., Smith, F. (1956). Colorometric method for determination of sugars and related substances. Analytical Chemistry, 28(3), 350–356. https://doi.org/10.1021/ac60111a017 [Google Scholar] [CrossRef]
34. Bates, L. S., Waldren, R. P., Teare, I. D. (1973). Rapid determination of free proline for water-stress studies. Plant and Soil, 39(1), 205–207. https://doi.org/10.1007/BF00018060 [Google Scholar] [CrossRef]
35. Singleton, V. L., Rossi, J. A. (1965). Colorimetry of total phenolics with phosphomolybdic-phosphotungstic acid reagents. American Journal of Enology and Viticulture, 16(3), 144–158. [Google Scholar]
36. Rahman, M. M., Islam, M. B., Biswas, M., Alam, A. K. (2015). In vitro antioxidant and free radical scavenging activity of different parts of Tabebuia pallida growing in Bangladesh. BMC Research Notes, 8(1), 621. https://doi.org/10.1186/s13104-015-1618-6 [Google Scholar] [PubMed] [CrossRef]
37. Aebi, H. (1984). Catalase in vitro. Methods in Enzymology, 105, 121–126. https://doi.org/10.1016/S0076-6879(84)05016-3 [Google Scholar] [PubMed] [CrossRef]
38. Paglia, D. E., Valentine, W. N. (1967). Studies on the quantitative and qualitative characterization of erythrocyte glutathione peroxidase. The Journal of Laboratory and Clinical Medicine, 70(1), 158–169. https://doi.org/10.5555/uri:pii:0022214367900765 [Google Scholar] [CrossRef]
39. Nishikimi, M., Rao, N. A., Yagi, K. (1972). The occurrence of superoxide anion in the reaction of reduced phenazine methosulfate and molecular oxygen. Biochemical and Biophysical Research Communications, 46(2), 849–854. https://doi.org/10.1016/S0006-291X(72)80218-3 [Google Scholar] [PubMed] [CrossRef]
40. Shapiro, S. S., Wilk, M. B. (1965). An analysis of variance test for normality (complete samples). Biometrika, 3(4), 591–611. https://doi.org/10.2307/2333709 [Google Scholar] [CrossRef]
41. Snedecor, G. W., Cochran, W. G. (1967). Statistical methods. 6th editionAmes, Iowa: Iowa State University Press. [Google Scholar]
42. Duncan, D. B. (1955). Multiple range and multiple F tests. Biometrics, 11(1), 1–42. https://doi.org/10.2307/3001478 [Google Scholar] [CrossRef]
43. Metsalu, T., Vilo, J. (2015). ClustVis: A web tool for visualizing clustering of multivariate data using principal component analysis and heatmap. Nucleic Acids Research, 43(W1), W566–W570. https://doi.org/10.1093/nar/gkv468 [Google Scholar] [PubMed] [CrossRef]
44. Hasanuzzaman, M., Bhuyan, M. B., Zulfiqar, F., Raza, A., Mohsin, S. M. et al. (2020). Reactive oxygen species and antioxidant defense in plants under abiotic stress: Revisiting the crucial role of a universal defense regulator. Antioxidants, 9(8), 681. https://doi.org/10.3390/antiox9080681 [Google Scholar] [PubMed] [CrossRef]
45. Gill, S. S., Tuteja, N. (2010). Reactive oxygen species and antioxidant machinery in abiotic stress tolerance in crop plants. Plant Physiology and Biochemistry, 48(12), 909–930. https://doi.org/10.1016/j.plaphy.2010.08.016 [Google Scholar] [PubMed] [CrossRef]
46. Karimi, R., Ershadi, A., Rezaeinezhad, A., Khanizadeh, S. (2016). Abscisic acid alleviates the deleterious effects of cold stress on ‘Sultana’ grapevine (Vitis vinifera L.) plants by improving the anti-oxidant activity and photosynthetic capacity of leaves. The Journal of Horticultural Science and Biotechnology, 91(4), 386–395. https://doi.org/10.1080/14620316.2016.1162027 [Google Scholar] [CrossRef]
47. Liu, W., Yu, K., He, T., Li, F., Zhang, D. et al. (2013). The low temperature induced physiological responses of Avena nuda L., a cold-tolerant plant species. The Scientific World Journal, 2013, 658793. https://doi.org/10.1155/2013/658793 [Google Scholar] [PubMed] [CrossRef]
48. Krasensky, J., Jonak, C. (2012). Drought, salt, and temperature stress induced metabolic rearrangements and regulatory networks. Journal of Experimental Botany, 63(4), 1593–1608. https://doi.org/10.1093/jxb/err460 [Google Scholar] [PubMed] [CrossRef]
49. Palonen, P., Buszard, D., Donnelly, D. (2000). Changes in carbohydrates and freezing tolerance during cold acclimation of red raspberry cultivars grown in vitro and in vivo. Physiologia Plantarum, 110(3), 393–401. https://doi.org/10.1111/j.1399-3054.2000.1100314.x [Google Scholar] [CrossRef]
50. Jacobsen, S. E., Monteros, C., Corcuera, L. J., Bravo, L. A., Christiansen, J. L. et al. (2007). Frost resistance mechanisms in quinoa (Chenopodium quinoa Willd.). European Journal of Agronomy, 26(4), 471–475. https://doi.org/10.1016/j.eja.2007.01.006 [Google Scholar] [CrossRef]
51. Jonytiene, V., Burbulis, N., Kupriene, R., Blinstrubiene, A. (2012). Effect of exogenous proline and de-acclimation treatment on cold tolerance in Brassica napus shoots cultured in vitro. Journal of Food, Agriculture and Environment, 10(1), 327–330. [Google Scholar]
52. Meng, F. Z., Hu, L. P., Wang, S. H., Sui, X. L., Wei, L. et al. (2008). Effects of exogenous abscisic acid (ABA) on cucumber seedling leaf carbohydrate metabolism under low temperature. Plant Growth Regulation, 56(3), 233–244. https://doi.org/10.1007/s10725-008-9303-6 [Google Scholar] [CrossRef]
53. Sami, F., Yusuf, M., Faizan, M., Faraz, A., Hayat, S. (2016). Role of sugars under abiotic stress. Plant Physiology and Biochemistry, 109, 54–61. https://doi.org/10.1016/j.plaphy.2016.09.005 [Google Scholar] [PubMed] [CrossRef]
54. Karimi, R. (2017). Potassium-induced freezing tolerance is associated with endogenous abscisic acid, polyamines and soluble sugars changes in grapevine. Scientia Horticulturae, 215(23), 184–194. https://doi.org/10.1016/j.scienta.2016.12.018 [Google Scholar] [CrossRef]
55. Chen, J. Z., Xu, C. X., Liang, L. F. (1999). Effect of low temperature on protein and proline in banana (Musa spp.) leaves. Journal of South China Agricultural University, 20(3), 54–58. [Google Scholar]
56. Cao, X., Wu, L., Wu, M., Zhu, C., Jin, Q. et al. (2020). Abscisic acid mediated proline biosynthesis and antioxidant ability in roots of two different rice genotypes under hypoxic stress. BMC Plant Biology, 20(1), 198. https://doi.org/10.1186/s12870-020-02414-3 [Google Scholar] [PubMed] [CrossRef]
57. Yoshiba, Y., Nanjo, T., Miura, S., Yamaguchi-Shinozaki, K., Shinozaki, K. (1999). Stress-responsive and developmental regulation of Δ1-pyrroline-5-carboxylate synthetase 1 (P5CS1) gene expression in Arabidopsis thaliana. Biochemical and Biophysical Research Communications, 261(3), 766–772. https://doi.org/10.1006/bbrc.1999.1112 [Google Scholar] [PubMed] [CrossRef]
58. Savouré, A., Hua, X. J., Bertauche, N., Montagu, M. V., Verbruggen, N. (1997). Abscisic acid-independent and abscisic acid-dependent regulation of proline biosynthesis following cold and osmotic stresses in Arabidopsis thaliana. Molecular and General Genetics, 254(1), 104–109. https://doi.org/10.1007/s004380050397 [Google Scholar] [PubMed] [CrossRef]
59. Wang, Y., Zhu, M., Yang, Y., Alam, I., Cheng, X. et al. (2017). Exogenous application of abscisic acid (ABA) enhances chilling tolerance in seedlings of napier grass (Pennisetum purpureum Schum.). Agricultural Science and Technology, 18(3), 417–423. https://doi.org/10.16175/j.cnki.1009-4229.2017.03.008 [Google Scholar] [CrossRef]
60. Apel, K., Hirt, H. (2004). Reactive oxygen species: Metabolism, oxidative stress, and signal transduction. Annual Review of Plant Biology, 55(1), 373–399. https://doi.org/10.1146/annurev.arplant.55.031903.141701 [Google Scholar] [PubMed] [CrossRef]
61. Sivaci, A., Kaya, A., Duman, S. (2014). Effects of ascorbic acid on some physiological changes of pepino (Solanum muricatum Ait.) under chilling stress. Acta Biologica Hungarica, 65(3), 305–318. https://doi.org/10.1556/abiol.65.2014.3.7 [Google Scholar] [PubMed] [CrossRef]
62. Qsaib, S., Ikbal, F. E. Z., Faize, M., Koussa, T. (2014). Changes in levels of hydrogen peroxide and phenolic compounds in grapevine latent buds during the annual cycle. Febs Journal, 4, 1–5. [Google Scholar]
63. Karimi, R., Ershadi, A. (2015). Role of exogenous abscisic acid in adapting of ‘Sultana’ grapevine to low temperature stress. Acta Physiologiae Plantarum, 37(8), 37–151. https://doi.org/10.1007/s11738-015-1902-z [Google Scholar] [CrossRef]
64. Pennycooke, J. C., Cox, S., Stushnoff, C. (2005). Relationship of cold acclimation, total phenolic content and antioxidant capacity with chilling tolerance in petunia (Petunia x hybrida). Environmental and Experimental Botany, 53(2), 225–232. https://doi.org/10.1016/j.envexpbot.2004.04.002 [Google Scholar] [CrossRef]
65. Sharma, P., Jha, A. B., Dubey, R. S., Pessarakli, M. (2012). Reactive oxygen species, oxidative damage, and antioxidative defense mechanism in plants under stressful conditions. Journal of Botany, 2012(1), 1–26. https://doi.org/10.1155/2012/217037 [Google Scholar] [CrossRef]
66. Javanmardi, J., Stushnoff, C., Locke, E., Vivanco, J. M. (2003). Antioxidant activity and total phenolic content of Iranian Ocimum accessions. Food Chemistry, 83(4), 547–550. https://doi.org/10.1016/S0308-8146(03)00151-1 [Google Scholar] [CrossRef]
67. Shao, H. B., Chu, L. Y., Shao, M. A., Abdul Jaleel, C., Mi, H. M. (2008). Higher plant antioxidants and redox signaling under environmental stresses. Comptes Rendus Biologies, 331(6), 433–441. https://doi.org/10.1016/j.crvi.2008.03.011 [Google Scholar] [PubMed] [CrossRef]
68. Kang, H. M., Saltveit, M. E. (2002). Antioxidant enzymes and DPPH-radical scavenging activity in chilled and heat-shocked rice (Oryza sativa L.) seedlings radicles. Journal of Agricultural and Food Chemistry, 50(3), 513–518. https://doi.org/10.1021/jf011124d [Google Scholar] [PubMed] [CrossRef]
69. Kang, H. M., Saltveit, M. E. (2002). Effect of chilling on antioxidant enzymes and DPPH radical scavenging activity of high and low vigour cucumber seedling radicles. Plant, Cell and Environment, 25(10), 1233–1238. https://doi.org/10.1046/j.1365-3040.2002.00915.x [Google Scholar] [CrossRef]
70. Sanchez-Moreno, C. (2002). Review: Methods used to evaluate the free radical scavenging activity in foods and biological systems. Food Science and Technology International, 8(3), 121–137. https://doi.org/10.1106/108201302026770 [Google Scholar] [CrossRef]
71. Raza, A., Tabassum, J., Kudapa, H., Varshney, R. K. (2021). Can omics deliver temperature resilient ready-to-grow crops? Critical Reviews in Biotechnology, 41(8), 1209–1232. https://doi.org/10.1080/07388551.2021.1898332 [Google Scholar] [PubMed] [CrossRef]
72. Raza, A., Charagh, S., García-Caparrós, P., Rahman, M. A., Ogwugwa, V. H. et al. (2022). Melatonin-mediated temperature stress tolerance in plants. GM Crops and Food, 13(1), 196–217. https://doi.org/10.1080/21645698.2022.2106111 [Google Scholar] [PubMed] [CrossRef]
73. Aslam, M., Fakher, B., Ashraf, M. A., Cheng, Y., Wang, B. et al. (2022). Plant low-temperature stress: Signaling and response. Agronomy, 12(3), 702. https://doi.org/10.3390/agronomy12030702 [Google Scholar] [CrossRef]
74. Zhou, Y., Hu, L., Ye, S., Jiang, L., Liu, S. (2018). Genome-wide identification of glutathione peroxidase (GPX) gene family and their response to abiotic stress in cucumber. 3 Biotech, 8(3), 1–11. https://doi.org/10.1007/s13205-018-1185-3 [Google Scholar] [PubMed] [CrossRef]
75. Ighodaro, O. M., Akinloye, O. A. (2018). First line defence antioxidants-superoxide dismutase (SODcatalase (CAT) and glutathione peroxidase (GPXTheir fundamental role in the entire antioxidant defence grid. Alexandria Journal of Medicine, 54(4), 287–293. https://doi.org/10.1016/j.ajme.2017.09.001 [Google Scholar] [CrossRef]
76. Lu, S., Su, W., Li, H., Guo, Z. (2009). Abscisic acid improves drought tolerance of triploid bermudagrass and involves H2O2- and NO-induced antioxidant enzyme activities. Plant Physiology and Biochemistry, 47(2), 132–138. https://doi.org/10.1016/j.plaphy.2008.10.006 [Google Scholar] [PubMed] [CrossRef]
77. de Souza, T. C., Magalhães, P. C., de Castro, E. M., Carneiro, N. P., Padilha, F. A. et al. (2014). ABA application to maize hybrids contrasting for drought tolerance: Changes in water parameters and in antioxidant enzyme activity. Plant Growth Regulation, 73(3), 205–217. https://doi.org/10.1007/s10725-013-9881-9 [Google Scholar] [CrossRef]
Cite This Article
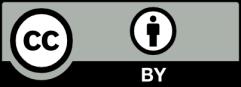