Open Access
ARTICLE
Evaluating the Effects of Sustainable Chemical and Organic Fertilizers with Water Saving Practice on Corn Production and Soil Characteristics
1 Institute of Agricultural Resources and Environment, Ningxia Academy of Agriculture and Forestry Sciences, Yinchuan, 750002, China
2 Faculty of Agricultural Sciences and Technology, Bahauddin Zakariya University, Multan, 60800, Pakistan
3 University of Layyah, Layyah, 31200, Pakistan
4 Key Laboratory of Nonpoint Source Pollution Control, Ministry of Agriculture, Institute of Agricultural Resources and Regional Planning, Chinese Academy of Agricultural Sciences (CAAS), Beijing, 100081, China
* Corresponding Author: Hongbin Liu. Email:
# The authors have contributed equally, should be considered as 1st authors
Phyton-International Journal of Experimental Botany 2023, 92(5), 1349-1360. https://doi.org/10.32604/phyton.2023.026952
Received 05 October 2022; Accepted 18 November 2022; Issue published 09 March 2023
Abstract
The rapidly growing world population, water shortage, and food security are promising problems for sustainable agriculture. Farmers adopt higher irrigation and fertilizer applications to increase crop production resulting in environmental pollution. This study aimed to identify the long-term effects of intelligent water and fertilizers used in corn yield and soil nutrient status. A series of field experiments were conducted for six years with treatments as: farmer accustomed to fertilization used as control (CON), fertilizer decrement (KF), fertilizer decrement + water-saving irrigation (BMP1); combined application of organic and inorganic fertilizer + water-saving irrigation (BMP2), and combined application of controlled-release fertilizer (BMP3). A significant improvement was observed in soil organic matter (14.9%), nitrate nitrogen (106.7%), total phosphorus (23.9%), available phosphorus (26.2%), straw yield (44.8%), and grain yield (54.7%) with BMP2 treatment as compared to CON. The study concludes that integrating chemical and organic fertilizers with water-saving irrigation (BMP2) is a good approach to increasing corn productivity, ensuring water safety and improving soil health. The limitations of the current study include the identification of fertilizer type and its optimum dose, irrigation water type, and geographical position.
Keywords
The world’s population increased to 7.4 billion in 2016 and is expected to reach 9.4 billion in 2050. With this diverse increase in population, ensuring global food security is a huge challenge [1]. Successful agricultural production is widely dependent on chemical fertilizers, which have significantly increased grain production globally for the last six decades [2]. Besides this, to overcome nutrient deficiency, farmers use various doses and types of fertilizers to achieve the maximum yield [3]. Farmers use high doses of nitrogen (N) based fertilizers to enhance plant growth which also exhausts soil phosphorus (P) and potassium (K) and leads to nutrient-depleted soils [4]. As a result, long-term excessive use of chemical fertilizers has resulted in soil fertility and quality decline and increased environmental pollution worldwide, especially in developing countries [5].
According to the World Bank, China’s fertilizer consumption is 30% higher than global fertilizer consumption. However, China’s total cultivated land is only 9% of the world’s cultivated land [2]. The excessive use of fertilizers can adversely affect water quality, soil acidification, organic matter depletion, and higher gas emissions contributing to environmental pollution [6]. Keeping the current scenario in view, the Chinese Ministry of Agriculture announced the “Zero Increase Action Plan” in 2015 to reduce the use of chemical fertilizers and increase crop yield with minimizing environmental concerns by 2020 [7,8]. So, it is challenging for the scientific community to sustain food security, improve crop yield, reduce environmental costs, and achieve sustainable agriculture production [9].
Water is essential for plant growth, and crops consume a huge amount of water [5]. Along with chemical fertilizers, overuse of irrigation water also depletes groundwater quality, ultimately affecting food security [9]. In addition, the sole long-term application of chemical fertilizers without organic inputs also triggers depletion of soil organic matter (SOM), disrupted soil structure, soil fertility decline, and reduced soil biodiversity [10]. Therefore, it is crucial to identify and understand the role of chemical fertilizers on plant growth, the association of irrigation and fertilization management, and countermeasures to sustainable agricultural production and soil quality [5].
Corn (Zea mays L.) is an important crop worldwide for agriculture and the economy as it is a food, fuel, and feed source. In China, corn is a major cereal crop and is intensively cultivated in two regions, Northeast China and North-Central China, which ranks China as the largest corn producer [11]. In North China, Ningxia Plain has a crucial role in agricultural production, highly dependent on the yellow river [12]. To achieve the aim of higher agricultural output by the farming community, overdose fertilization and increasing unnecessary irrigation practices have emerged the problems of non-point pollution [13]. Irrespective of higher production, China is not a significant exporter because corn’s consumption is higher than its production.
Moreover, the gap between the supply and demand of corn is also increasing in China [14]. In addition, corn is a source of 365 kcal 100 g−1 and contains about 9.4 g protein, 1.2 g ash, 7.3 g fibre, and 74.3 g carbohydrates [15]. With the growing population of China, challenges regarding food security, increasing purchase power, and demand for animal feed and fuel have also been faced. The agriculture sector is the backbone of China for the citizens and the country’s economy [1]. Various studies have documented the effects of long-term fertilization on corn production, but information regarding the interaction of long-term fertilizer management with reduced water application is lacking. This raises the need for the current study to ensure higher corn production with low water consumption, which will help ensure food security and minimize environmental risk.
Keeping all the aspects in view, a series of long-term field experiments were conducted with the objectives to (1) evaluate the impact of the six-year application of different strategies of fertilizer and water management on corn production, (2) assess the interaction of fertilizers and water management on soil properties, and (3) identification of optimum management strategies for sustainable agriculture to save the soil health. Our study hypothesises that the integrated application of organic and inorganic fertilizers and limited water irrigation will improve the soil properties and help the farmers achieve desired maize yield.
A series of field experiments were conducted at Nongfeng village (42.32°N, 106.65°E) Wanghong, District Yongning, Ningxia, China, at an altitude of 1,108 m. The site is located on the arid and semi-arid plains of the country. This experiment was conducted six consecutive years (May 2015 to October 2020). Corn (Z. mays) cv. Zhengda12 was used as a test crop and was cultivated once a year. The soil texture of this area is characterized as heavy loam (USDA system).
According to the local conditions, five treatments were applied to the corn, including; farmer accustomed to fertilization (CON, 525:180:30 kg NPK ha−1) used as control, fertilizer decrement (KF: 450:150:15 kg NPK ha−1); fertilizer decrement + water-saving irrigation (BMP1, 450:150:15 kg NPK ha−1); combined application of organic and inorganic fertilizer + water-saving irrigation (BMP2, 375:120:0 kg NPK ha−1 + 4.5 tons organic fertilizer ha−1), and combined application of controlled-release fertilizer (BMP3, 180:120:15 kg NPK ha−1). Urea (46% N), triple superphosphate (46% P2O5), and K sulfate (50% K2O) were used as sources of chemical fertilizers. Moreover, commercial organic fertilizers and slow-control fertilizers (44% N) were used in respective treatments.
The corn plants were planted in the field, maintaining a row spacing of 60 cm and plant spacing of 22.2 cm, and the seedlings quantity was 75,000 ha−1. Each treatment was replicated thrice and arranged in a randomized completely block design (RCBD). Following the local conventional practices, weeds were removed manually, and pesticide (50 mL Nicosulfuron mixed in 25 L water) was applied. Groundwater was used for irrigation purposes. The crop was harvested at maturity, and plant straw and corn samples were collected for yield measurement. In addition, soil samples were collected from 0–20 cm depth with the help of a soil auger and stored for further analysis.
To determine soil properties, the samples collected from the plots of the same treatment were mixed, and a homogenous sample was prepared. Soil pH was examined with 1:5 soil/water using a potentiometer. Soil moisture content was determined by the weight loss method [16], and SOM was determined by the potassium dichromate oxidation method [17]. Soil TN was measured using the Kjeldahl digestion method (KDY-9830, Beijing, China) [18]. The soil NH4-N and NO3-N were identified by extracting the soil with 0.01 CaCl2 and using a Flow Injector Auto analyzer (AutoAnalyzer 3, High-Resolution Digital Colorimeter). For TP, soil samples were digested using H2SO4-HClO4 and determined using the molybdate-ascorbic acid method [19]. Soil Available P was noted by calorimetry after extracting with 0.5 M sodium bicarbonate (NaHCO3) [20]. TK was analyzed after oxidative digestion with H2SO4-HClO4, and soil available K was determined after extraction with 1 M ammonium acetate [21] using a flame photometer (FP 6410, Shanghai Jingke, Shanghai, China).
Data were statistically analyzed using Statistix 8.1® software and R studio. In all the graphs, data sets are presented as means and standard deviation (SD) and were obtained from three replicates (means ± SD, n = 3). All variables for every year fit the normal distribution (p > 0.05) and were subjected to analyses of variance (ANOVAs). The statistically significant comparisons were determined using least significant difference (LSD) tests and a 5% probability level. Moreover, the correlation between soil and plant factors was examined by Pearson correlation analysis using R-studio. Data processing and visualization were done using Microsoft Excel 2016 and R-studio.
3.1 Soil Properties and Nutrient Status
The annual comparison showed that no significant differences were found in soil pH during the first four years (2015–2018), but was significantly higher in 2019 with CON and BMP3, followed by CON in 2020 compared to the other treatments (Fig. 1a). The treatment-wise comparison results showed that BMP3 and CON have significantly increased the soil pH as compared to KF, BMP1, and BMP2 (Fig. 1b). Soil moisture content was increased considerably in CON compared to other treatments in 2016 and 2017, respectively (Fig. 1c). However, there were no significant differences in other years. Treatment comparison shows that CON improved the soil moisture content significantly as compared to the other treatments (Fig. 1d). The SOM was significantly enhanced with BMP2 and BMP3 in 2015, was significantly reduced in 2016 (CON), 2017 (CON, KF), 2018 (CON), and 2019 (CON) compared with other treatments. The treatment-wise comparison showed that SOM was significantly reduced with CON compared to the other treatments (Figs. 1e–1f).
Figure 1: Effect of treatments on soil properties during 2015–2020, soil pH (a,b), soil moisture content (c,d), organic matter (e,f). Average annual treatment response in the control (CON), fertilizer decrement (KF), fertilizer decrement + water-saving irrigation (BMP1), combined application of organic and inorganic fertilizer + water-saving irrigation (BMP2) and combined application of controlled-release fertilizer (BMP3) with relative to CON (2015–2020). The error bars indicate the SDs
The annual results showed that soil TN was significantly reduced in 2015, 2017, and 2018 compared to the other treatments (Fig. 2a). Treatment-wise comparison showed that soil TN was significantly lower in CON as compared to other treatments (Fig. 2b). The soil NH4+-N was significantly higher in 2015 (KF), 2016 (KF), 2017 (KF, BMP1, BMP2), 2018 (KF, BMP1), 2019 (2019), and 2020 (KF, BMP1), respectively (Fig. 2c) Treatment-wise comparison demonstrated that KF significantly enhanced the soil NH4+-N, followed by BMP1, as compared to the other treatments (Fig. 2d). For the NO3--N, the higher soil NO3--N was in 2015 (BMP1, BMP2), 2016 (CON, KF, BMP2, BMP3), 2017 (CON, BMP2), 2018 (BMP2), 2019 (BMP2), and 2020 (BMP2), respectively (Fig. 2e). The treatment-wise comparison revealed that BMP2 improved the soil NO3--N concentration significantly (Fig. 2f).
Figure 2: Effect of treatments on soil N status during 2015–2020. Soil TN (a,b), soil NH4-N (c,d), NO3-N (e,f). Average annual treatment response in the control (CON), fertilizer decrement (KF), fertilizer decrement + water-saving irrigation (BMP1), combined application of organic and inorganic fertilizer + water-saving irrigation (BMP2) and combined application of controlled-release fertilizer (BMP3) with relative to CON (2015–2020). The error bars indicate the SDs
Soil TP was significantly increased in 2015 (BMP3), 2016 (KF, BMP1, BMP2, BMP3), 2017 (BMP1, BMP2), and 2020 (BMP2), respectively (Fig. 3a). Moreover, the treatment-wise comparison indicated that CON reduced the soil TP significantly as compared to the other treatments (Fig. 3b). Year-wise comparison of results revealed that soil available P was significantly improved in 2015 (KF), 2016 (KF), 2018 (KF), 2019 (KF, BMP2), 2020 (BMP2), and was insignificant in 2017 compared with other treatments (Fig. 3c). While, it was significantly improved with KF, followed by BMP2 as compared to other treatments (Fig. 3d).
Figure 3: Effect of treatments on soil P status during 2015–2020. Soil TP (a,b), soil available P (c,d). Average annual treatment response in the control (CON), fertilizer decrement (KF), fertilizer decrement + water-saving irrigation (BMP1), combined application of organic and inorganic fertilizer + water-saving irrigation (BMP2) and combined application of controlled-release fertilizer (BMP3) with relative to CON (2015–2020). The error bars indicate the SDs
The annual comparison of treatments showed that soil TK was improved significantly in 2016 (BMP2) and was reduced significantly in 2015 (CON, KF) and 2019 (CON) as compared to the other treatments (Fig. 4a). In addition, treatment-wise comparison also indicated that BMP2 has significantly improved the soil TK as compared to the other treatments (Fig. 4b). Soil available K was significantly higher in each year KF treatment compared with other treatments (Fig. 4c). The treatment-wise comparison also indicated that KF has significantly enhanced the soil available K contents as compared to all other treatments (Fig. 4d).
Figure 4: Effect of treatments on soil K status during 2015–2020. Soil TK (a,b), soil available K (c,d). Average annual treatment response in the control (CON), fertilizer decrement (KF), fertilizer decrement + water-saving irrigation (BMP1), combined application of organic and inorganic fertilizer + water-saving irrigation (BMP2) and combined application of controlled-release fertilizer (BMP3) with relative to CON (2015–2020). The error bars indicate the SDs
The annual comparison should that straw yield was significantly improved in 2016 (BMP2), 2018 (KF, BMP2, BMP3) and was significantly reduced in 2016, 2017, 2019, and 2020 with CON as compared with other treatments (Fig. 5a). Treatment-wise comparison showed that straw yield was significantly higher with KF, BMP2, and BMP3, followed by BMP1 as compared to the CON treatment (Fig. 5b). Grain yield was significantly enhanced in 2015 (BMP2), 2016 (BMP2), 2017 (KF), 2018 (KF, BMP1, BMP2, BMP3), 2019 (BMP2), and 2020 (BMP2), respectively, compared with other treatments (Fig. 5c). Treatment-wise comparison showed that the application of BMP2 significantly improved the grain yield as compared to other treatments (Fig. 5d).
Figure 5: Effect of treatments on corn yield during 2015–2020. Straw yield (a,b), Grain yield (c,d). Average annual treatment response in the control (CON), fertilizer decrement (KF), fertilizer decrement + water-saving irrigation (BMP1), combined application of organic and inorganic fertilizer + water-saving irrigation (BMP2) and combined application of controlled-release fertilizer (BMP3) with relative to CON (2015–2020). The error bars indicate the SDs
3.3 Pearson Correlation Analysis
Soil pH showed a strong negative relation with NH4-N and a moderate negative correlation with NO3-N and TK. In contrast, a weak negative correlation with grain yield, SOM, TP, and available P was observed. Moreover, SOM showed a strong positive correlation with grain yield and a moderate negative correlation with soil moisture content. A strong negative correlation was observed between soil moisture content and grain yield. Soil TN showed a moderate positive correlation with organic matter and a moderate negative correlation with soil moisture content. NO3-N showed a strong positive influence on grain yield. Soil TP has a strong positive interaction with grain yield and a moderately positive effect on TN and NO3-N. In addition, it has a moderate negative correlation with soil moisture content. TK has a strong positive correlation interaction between TP, SOM, NO3-N, and grain yield.
In comparison, a moderate positive correlation with TN and a moderate negative correlation with soil moisture content was observed. Soil available K was positively influenced by TP and NH4-N. While it is negatively correlated with NO3-N (Fig. 6).
Figure 6: Pearson correlation indicating the relationship between soil and plant. Whereas, NO3-N is nitrate nitrogen, NH4-N is ammonium nitrogen, TN is total nitrogen, TP is total phosphorus, TK is total K, Available P is Olsen phosphorus, Available K is available K, and pH is soil pH
A six-year repeated corn field experiment with different fertilization and water management practices showed significant changes in soil properties (Figs. 1–5). The results indicated that organic fertilizer combined with chemical fertilization significantly reduced the soil pH (Figs. 1a–1b). This might be associated with to release of organic acids from organic inputs [22,23]. Chemical fertilization deteriorates the soil quality and is the cause for the accumulation of various salts that increase the soil pH as well. Soil moisture content was highest in CON compared to other treatments where water-saving irrigation techniques were used (Figs. 1c–1d). High moisture levels might be due to excessive irrigation. The SOM was significantly improved with BMP2 application (Figs. 1e–1f) and might be associated with using organic fertilizer and water-saving irrigation. The use of organic amendments improves aggregate stability and SOM content. Moreover, it also enhances soil-water retention and improves soil structure [24].
Soil TN was significantly improved with the application of BMP3 (Figs. 2a and 2b). It can be related to the use of controlled released fertilizers that minimizes nutrient losses and improves N-use efficiency and soil N status in soil [25]. Moreover, soil NH4-N was increased with higher doses of N-based chemical fertilizers (Figs. 2c and 2d). Comparatively, slow-release fertilizers slowly released N; thus, the soil NH4-N concentration is also low [26]. Soil NO3-N increased with organic fertilizers (Figs. 2e and 2f) as organic matter improves the soil’s water-holding capacity and minimizes nitrate leaching [27]. Studies also reported that the organic substitution under equal N conditions improves the soil nutrient balance and prevents soil nutrient leaching. Additionally, manures can improve soil structure and cation exchange capacity and ultimately increase NO3-N immobilization in soil [28].
The combined use of organic and inorganic fertilizers enhanced the soil’s total and available P concentration (Figs. 3a–3d). This might be due to the P content in manure [29], mobilization of native P in soil, inhibition formation of soil complexes, increasing the labile pool, and decreasing the non-labile P pool [30]. However, the excessive use of mineral fertilizers can also increase the soil available P concentration, which can cause P leaching and environmental pollution [31]. Organic fertilization also improves the soil’s nutrient status, including K (Figs. 4a–4d). Soil TK contents were improved by applying organic fertilisers as they are a source of nutrients and improve soil fertility and nutrient status [32]. The highest available K level in the soil can be associated with the high inorganic K usage and irrigation, which causes higher percolation of K+ ions and K leaching in soil.
Straw and grain yield improved with the combined use of organic and inorganic fertilizers with managed irrigation practice (Figs. 5a–5d). It might be associated with organics that increase soil nutrient levels, enhance organic matter levels, reduce acidification, and improve soil physical, chemical, and biological properties, as similar results have been identified in various studies [19,21,33]. As published literature describes, organics also improve soil nutrient availability and plant uptake, which is a reason behind improved crop yield [23]. In addition, releasing macro and micronutrients from the poultry manure can increase the maize yield [34]. Organic manure application also has the potential to enhance the maize yield in subsequent years due to the improved SOM and microbial activity [28].
Correlation analysis showed a positive relationship between crop yield and soil nutrient status and identifies that improving soil fertility status will solve the issue of food security and sustainable agriculture (Fig. 6). The study concludes that proper fertilizer and water management practices can improve soil fertility status and corn productivity. Furthermore, the quality and nutrient concentration of corn needs to be addressed. The impact of such fertilization and irrigation management practices on nutrient losses, global warming, and greenhouse gas emissions is a research gap. In addition, studies are required focusing on irrigation water type, fertilizer type, geographical position, and optimum dose for chemical fertilizer input. The study recommends the practical implementation of organic fertilizer, controlled irrigation, and inorganic fertilisers for the betterment of corn production and sustainable agriculture.
The results of this study suggest that combining organic and inorganic fertilizers with controlled irrigation improves the soil’s physical and chemical properties along with corn yield and productivity. The long-term balanced use of chemical fertilizers can effectively sustain agriculture, reduce environmental pollution, and cope with food security and water shortage challenges. The impacts of such strategies on the biological properties of soil, groundwater quality, and global warming need to be addressed in the future. Furthermore, studies are required focusing on irrigation water type, fertilizer type, geographical position, and optimum dose for chemical fertilizer input.
Funding Statement: This study was supported by the National Natural Science Foundation of China [Grant No. U20A20114], and the soil N losses in the greenhouse field in the Yellow River Irrigation as affected by the annual changes of groundwater depth [Grant No. 41361062].
Author Contributions: The authors confirm their contribution to the paper as follows: study conception and design: Xuejun Zhang, Muhammad Amjad Bashir, Yucong Geng, Hongbin Liu; data collection: Xuejun Zhang, Muhammad Amjad Bashir, Qurat-Ul-Ain Raza, Xiaotong Liu, Jianhang Luo, Ying Zhao; analysis and interpretation of results: Xuejun Zhang, Muhammad Amjad Bashir, Qurat-Ul-Ain Raza, Qiuliang Lei, Hafiz Muhammad Ali Raza, Abdur Rehim; draft manuscript preparation: Xuejun Zhang, Muhammad Amjad Bashir, Qurat-Ul-Ain Raza, Hongbin Liu. All authors reviewed the results and approved the final version of the manuscript.
Conflicts of Interest: The authors declare that they have no conflicts of interest to report regarding the present study.
References
1. Mukhopadhyay, K., Thomassin, P. J., Zhang, J. (2018). Food security in China at 2050: A global CGE exercise. Journal of Economic Structures, 7(1), 1–29. https://doi.org/10.1186/s40008-017-0097-4 [Google Scholar] [CrossRef]
2. Xin, L. (2022). Chemical fertilizer rate, use efficiency and reduction of cereal crops in China, 1998–2018. Journal of Geographical Sciences, 32(1), 65–78. https://doi.org/10.1007/s11442-022-1936-2 [Google Scholar] [CrossRef]
3. Lu, J., Hu, T., Zhang, B., Wang, L., Yang, S. (2021). Nitrogen fertilizer management effects on soil nitrate leaching, grain yield and economic benefit of summer maize in Northwest China. Agricultural Water Management, 247(5), 106739. https://doi.org/10.1016/j.agwat.2021.106739 [Google Scholar] [CrossRef]
4. Nawaz, A., Farooq, M., Ul-Allah, S., Gogoi, N., Lal, R. et al. (2021). Sustainable soil management for food security in South Asia. Journal of Soil Science and Plant Nutrition, 21(1), 258–275. https://doi.org/10.1007/s42729-020-00358-z [Google Scholar] [CrossRef]
5. Wang, J., Qin, L., Cheng, J., Shang, C., Li, B. et al. (2022). Suitable chemical fertilizer reduction mitigates the water footprint of maize production: Evidence from Northeast China. Environmental Science and Pollution Research, 29(15), 22589–22601. https://doi.org/10.1007/s11356-021-17336-2 [Google Scholar] [PubMed] [CrossRef]
6. Rahman, K. M. A., Zhang, D. (2018). Effects of fertilizer broadcasting on the excessive use of inorganic fertilizers and environmental sustainability. Sustainability, 10(3), 759. https://doi.org/10.3390/su10030759 [Google Scholar] [CrossRef]
7. Guo, J., Fan, J., Zhang, F., Yan, S., Zheng, J. et al. (2021). Blending urea and slow-release nitrogen fertilizer increases dryland maize yield and nitrogen use efficiency while mitigating ammonia volatilization. Science of the Total Environment, 790(1–2), 148058. https://doi.org/10.1016/j.scitotenv.2021.148058 [Google Scholar] [PubMed] [CrossRef]
8. Raza, A., Salehi, H., Rahman, M. A., Zahid, Z., Madadkar Haghjou, M. et al. (2022). Plant hormones and neurotransmitter interactions mediate antioxidant defenses under induced oxidative stress in plants. Frontiers in Plant Science, 13, 3061. https://doi.org/10.3389/fpls.2022.961872 [Google Scholar] [PubMed] [CrossRef]
9. Wang, X., Yang, Y., Zhao, J., Nie, J., Zang, H. (2020). Yield benefits from replacing chemical fertilizers with manure under water deficient conditions of the winter wheat–summer maize system in the North China Plain. European Journal of Agronomy, 119, 126118. https://doi.org/10.1016/j.eja.2020.126118 [Google Scholar] [CrossRef]
10. Zhao, J., Ni, T., Li, J., Lu, Q., Fang, Z. et al. (2016). Effects of organic-inorganic compound fertilizer with reduced chemical fertilizer application on crop yields, soil biological activity and bacterial community structure in a rice-wheat cropping system. Applied Soil Ecology, 99, 1–12. https://doi.org/10.1016/j.apsoil.2015.11.006 [Google Scholar] [CrossRef]
11. Xiao, D., Bai, H., Liu, D. L., Tang, J., Wang, B. et al. (2022). Projecting future changes in extreme climate for maize production in the North China plain and the role of adjusting the sowing date. Mitigation and Adaptation Strategies for Global Change, 27(3), 1–21. https://doi.org/10.1007/s11027-022-09995-4 [Google Scholar] [CrossRef]
12. Geng, Y., Bashir, M. A., Zhao, Y., Luo, J., Liu, X. et al. (2022). Long-term fertilizer reduction in greenhouse tomato-cucumber rotation system to assess N utilization, leaching, and cost efficiency. Sustainability, 14(8), 1–15. https://doi.org/10.3390/su14084647 [Google Scholar] [CrossRef]
13. Zhao, Y., Luo, J. H., Chen, X. Q., Zhang, X. J., Zhang, W. L. (2012). Greenhouse tomato-cucumber yield and soil N leaching as affected by reducing N rate and adding manure: A case study in the Yellow River Irrigation Region China. Nutrient Cycling in Agroecosystems, 94(2), 221–235. https://doi.org/10.1007/s10705-012-9535-8 [Google Scholar] [CrossRef]
14. Wang, J., Hu, X. (2021). Research on corn production efficiency and influencing factors of typical farms: Based on data from 12 corn-producing countries from 2012 to 2019. PLoS One, 16(7), e0254423. https://doi.org/10.1371/journal.pone.0254423 [Google Scholar] [PubMed] [CrossRef]
15. Nuss, E. T., Tanumihardjo, S. A. (2010). Maize: A paramount staple crop in the context of global nutrition. Comprehensive Reviews in Food Science and Food Safety, 9(4), 417–436. https://doi.org/10.1111/j.1541-4337.2010.00117.x [Google Scholar] [PubMed] [CrossRef]
16. O’Kelly, B. C., Sivakumar, V. (2014). Water content determinations for peat and other organic soils using the oven-drying method. Drying Technology, 32(6), 631–643. https://doi.org/10.1080/07373937.2013.849728 [Google Scholar] [CrossRef]
17. Norman, A. G., Allison, L. E. (1965). Organic carbon. In: Methods of soil analysis: Part 2, chemical and microbiological properties, 9.2. The American Society of Agronomy, Inc. [Google Scholar]
18. Lu, R. (2000). Soil and agro-chemical analysis methods, pp. 205–266. Beijing, China: Agricultural Science and Technology Press. [Google Scholar]
19. Bashir, M. A., Wang, H., Pan, J., Khoshnevisan, B., Sun, W. et al. (2021). Variations in soil nutrient dynamics and their composition in rice under integrated rice-crab co-culture system. Journal of Cleaner Production, 281(1), 125222. https://doi.org/10.1016/j.jclepro.2020.125222 [Google Scholar] [CrossRef]
20. Olsen, S. R. (1954). Estimation of available phosphorus in soils by extraction with sodium bicarbonate. USA: US Department of Agriculture. [Google Scholar]
21. Bashir, M. A., Rehim, A., Liu, J., Imran, M., Liu, H. et al. (2019). Soil survey techniques determine nutrient status in soil profile and metal retention by calcium carbonate. Catena, 173(2), 141–149. https://doi.org/10.1016/j.catena.2018.10.015 [Google Scholar] [CrossRef]
22. Naveed, S., Rehim, A., Imran, M., Bashir, M. A., Anwar, M. F. et al. (2018). Organic manures: An efficient move towards maize grain biofortification. International Journal of Recycling of Organic Waste in Agriculture, 7(3), 189–197. https://doi.org/10.1007/s40093-018-0205-y [Google Scholar] [CrossRef]
23. Raza, Q. U. A., Bashir, M. A., Rehim, A., Raza, H. M. A. (2021). Role of sugarcane industrial byproducts on soil physicochemical properties and metal accumulation in rice. Environmental Science and Pollution Research, 29(17), 24726–24736. https://doi.org/10.1007/s11356-021-17428-z [Google Scholar] [PubMed] [CrossRef]
24. Bai, Z., Caspari, T., Gonzalez, M. R., Batjes, N. H., Mäder, P. et al. (2018). Effects of agricultural management practices on soil quality: A review of long-term experiments for Europe and China. Agriculture, Ecosystems & Environment, 265(6), 1–7. https://doi.org/10.1016/j.agee.2018.05.028 [Google Scholar] [CrossRef]
25. dos Santos Cordeiro, C. F., Rodrigues, D. R., Echer, F. R. (2022). Cover crops and controlled-release urea decrease need for mineral nitrogen fertilizer for cotton in sandy soil. Field Crops Research, 276(6), 108387. https://doi.org/10.1016/j.fcr.2021.108387 [Google Scholar] [CrossRef]
26. Liang, Z., Jin, X., Zhai, P., Zhao, Y., Cai, J. et al. (2022). Combination of organic fertilizer and slow-release fertilizer increases pineapple yields, agronomic efficiency and reduces greenhouse gas emissions under reduced fertilization conditions in tropical areas. Journal of Cleaner Production, 343(6), 131054. https://doi.org/10.1016/j.jclepro.2022.131054 [Google Scholar] [CrossRef]
27. Abd-Elrahman, S. H., Saudy, H. S., El-Fattah, D. A. A., Hashem, F. A. (2022). Effect of irrigation water and organic fertilizer on reducing nitrate accumulation and boosting lettuce productivity. Journal of Soil Science and Plant Nutrition, 22(2), 2144–2155. https://doi.org/10.1007/s42729-022-00799-8 [Google Scholar] [CrossRef]
28. Geng, Y., Cao, G., Wang, L., Wang, S. (2019). Effects of equal chemical fertilizer substitutions with organic manure on yield, dry matter, and nitrogen uptake of spring maize and soil nitrogen distribution. PLoS One, 14(7), e0219512. https://doi.org/10.1371/journal.pone.0219512 [Google Scholar] [PubMed] [CrossRef]
29. Rehim, A., Khan, M., Imran, M., Bashir, M. A., Ul-allah, S. et al. (2020). Integrated use of farm manure and synthetic nitrogen fertilizer improves nitrogen use efficiency, yield and grain quality in wheat. Italian Journal of Agronomy, 15(1), 29–34. https://doi.org/10.4081/ija.2020.1360 [Google Scholar] [CrossRef]
30. Ahmed, K., Qadir, G., Nawaz, M. Q., Saqib, A. I., Rizwan, M. et al. (2018). Integrated phosphorus management improves production of rice-wheat cropping-system under salt affected conditions. International Journal of Plant Production, 12(1), 25–32. https://doi.org/10.1007/s42106-017-0003-x [Google Scholar] [CrossRef]
31. Ahmed, W., Jing, H., Kaillou, L., Qaswar, M., Khan, M. N. et al. (2019). Changes in phosphorus fractions associated with soil chemical properties under long-term organic and inorganic fertilization in paddy soils of Southern China. PLoS One, 14(5), e0216881. https://doi.org/10.1371/journal.pone.0216881 [Google Scholar] [PubMed] [CrossRef]
32. Zhong, W., Gu, T., Wang, W., Zhang, B., Lin, X. et al. (2010). The effects of mineral fertilizer and organic manure on soil microbial community and diversity. Plant and Soil, 326(1–2), 511–522. https://doi.org/10.1007/s11104-009-9988-y [Google Scholar] [CrossRef]
33. Liu, J., Shu, A., Song, W., Shi, W., Li, M. et al. (2021). Long-term organic fertilizer substitution increases rice yield by improving soil properties and regulating soil bacteria. Geoderma, 404, 115287. https://doi.org/10.1016/j.geoderma.2021.115287 [Google Scholar] [CrossRef]
34. Adeyemo, A. J., Akingbola, O. O., Ojeniyi, S. O. (2019). Effects of poultry manure on soil infiltration, organic matter contents and maize performance on two contrasting degraded alfisols in southwestern Nigeria. International Journal of Recycling of Organic Waste in Agriculture, 8(S1), 73–80. https://doi.org/10.1007/s40093-019-0273-7 [Google Scholar] [CrossRef]
Cite This Article
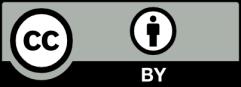
This work is licensed under a Creative Commons Attribution 4.0 International License , which permits unrestricted use, distribution, and reproduction in any medium, provided the original work is properly cited.