Open Access
REVIEW
Biochemical Mechanism Unlocking Their Potential Role in Salt Tolerance Mechanism of Zizyphus Germplasm
1 Department of Horticulture, The University of Agriculture, Dera Ismail Khan, 29050, Pakistan
2 Department of Horticulture, Bahauddin Zakariya University, Multan, 60060, Pakistan
3 College of Horticulture, Hainan University, Haikou, 570228, China
* Corresponding Authors: Riaz Ahmad. Email: ,
(This article belongs to the Special Issue: Physiological and Molecular Interventions in Improving Abiotic Stress Tolerance in Plants)
Phyton-International Journal of Experimental Botany 2023, 92(5), 1539-1553. https://doi.org/10.32604/phyton.2023.026672
Received 20 September 2022; Accepted 01 December 2022; Issue published 09 March 2023
Abstract
Salinity is one of the major constraints reducing plant growth and yield. Irrigation with poor quality and brackish water to orchards is a major cause of stunted growth and low yield. The salt tolerance mechanism is one of the complicated genomic characters that is very problematic to develop in fruit trees and becomes much more severe at any growth and developmental stage. Osmotic stress and hormonal imbalances are major constraints causing low biomass production. Fruit tree tolerance/sensitivity is chiefly based on the activation of a defense system comprised of super-oxidase dismutase (SOD), peroxidase (POD) and catalases (CAT), non-enzymatic compounds including ascorbic acid, phenolics, flavonoids, stress indicators [i.e., hydrogen peroxide (H2O2), lipid peroxidation, malondialdehyde (MDA), reactive oxygen species (ROS) and osmolytes containing proline, glycine-betaine (GB), ascorbates (APX), glutathione peroxidase (GPX) and glutathione reductase (GR)]. Tolerant genotypes must have higher antioxidant assays to cope with the adverse effects of salinity stress because their defense system had the potential to scavenge toxic ROS and protect from membrane leakage. Some work is conducted on agronomic and horticultural crops; however, underutilized fruit crops are still neglected and need serious consideration from plant researchers. Minor fruit crops especially Zizyphus had excellent nutritional aspects. The current study provides detailed insights into the physiological and biochemical mechanisms of Zizyphus species to cope with the adverse effects of salinity by improving their plant defense system. The development of salt-tolerant germplasm is a requisite and can be developed by utilization of physiological, biochemical, and molecular mechanisms. Application of different molecular approaches (i.e., genome mapping, genome editing, genetic transformation, proteomics, transcriptomics, and metabolites) are effective for higher yield by improving tolerance mechanisms.Keywords
The world population is going to be increased in the future and may reach 9.3 billion from 6 billion by 2050 [1]. It is imperative to enhance productivity from the soil with mitigation of adverse effects of abiotic stresses. In the recent era, climate change is becoming a global concern for food security because it is associated with environmental impacts of salinity, drought, flooding, heavy metals, ultraviolet radiation, and temperature-related extremities [2]. The decrease in production is due to climate change and becoming a global threat to food security in the future [3]. Environmental stresses occurring from climate change are going to reduce the productivity of fruit crops around the globe. There is an urgent need for time to develop salt-tolerant germplasm to get a sustainable production. Among abiotic stresses, salinity is a critical stress resulting in an average yield reduction and poor quality of fruits. Approximately, 20% yield reduction was recorded due to salinity in the world [4].
Reduction in fruit yield due to salinity is becoming a challenging point for horticultural sectors, especially fruit crops. Efforts in crop improvement programs are more vital by modifying different agronomic practices to regulate salinity stress. The application of traditional breeding strategies is eliminated because of having a laborious and time-consuming nature. Application of transgenic approaches is also limited and not used due to their poor performances in farmers’ field conditions as studied by Srivastava et al. [5]. Fruit crops in field conditions face multiple abiotic stresses, while transformed trees have tolerance against one stress type. However, sometimes transformed genes may possibly not perform because stress tolerance against salinity is governed by polygenes that regulate different metabolic pathways and signaling molecules. It has been evaluated that the development of transgenic plants against salinity stress is also a good solution for the reduction to adverse effects of salinity under field conditions [5]. Characterization and identification of salt tolerant/sensitive germplasm are also important for higher yield with superior fruit quality of Zizyphus.
Excess of salt accumulation in soil depressed Zizyphus fruit production. Higher salinity accumulation occurs in the root zone and it is transported toward other plant parts. Osmotic stress and nutritional imbalances occur due to salinity in the root zone resulting in stunted growth and poor fruit yield [6]. Disturbances in the metabolism of some Z. mauritiana cultivars like Karela, Suffan, Delhi White, and Mehmood Wali are because of induced salt concentrations in the root zone as reported by Sherani et al. [7]. Exceeded uptake, transportation and accumulation of ions in cellular organelles and compartments reach toxic levels because of higher salinity levels [7]. Stomatal regulation is disturbed because of a reduction in CO2 diffusion, photosynthesis, photosystem II, chlorophyll content, carbohydrates accumulation, and transpiration in those fruit seedlings treated with high NaCl levels [8]. The toxic ROS generation is because of higher salinity stress resulting from oxidative stress. To manage oxidative stress caused by over-accumulation of ROS, fruit trees activate their defense mechanism through enzymatic and non-enzymatic processes, and osmolytes [9]. A plant defense system efficiently scavenges the overproduction of ROS under salt stress in fruit crops [1].
Mineral nutrients, plant growth regulators, genetic variability, and molecular mechanisms are imperative strategies that can exploit for the alleviation of salt tolerance in Zizyphus germplasm. To overcome osmotic, salt-specific, and oxidative stresses, fruit trees have the potential to decrease toxic ions by the production of different enzymatic and non-enzymatic activities, and osmolytes naturally [9]. Zizyphus is an underutilized fruit species that originated from Indo-Pak and China subcontinents. It is comprised of 135–170 species. Z. ocenoplia, Z. rotundifolia, Z. spina-christi Z. nummularia and Z. xylocarpa are wild species, while Z. jujube and Z. mauritiana are well known edible species of Zizyphus [10,11]. Among these, Z. mauritiana has a wide adoption due to early age bearing, higher nutritional profiling, and good economic value. Fruits of Zizyphus species are rich in carbohydrates, total soluble solids (TSS), different sugars, total phenolics, flavonoids, antioxidants, proteins, ascorbic acid, fiber, carotenes, calcium, phosphorus, calcium, iron, and vitamins like A, B, and C [12].
Plant researchers are focusing on sustainable approaches necessary for improving the yield of Zizyphus germplasm growing under salinity stress by improving plant defense mechanisms. The exploitation of biochemical and physiological mechanisms under salinity is still unclear and needs detailed investigation in Zizyphus germplasm. The current study provides detailed insights into physiological and biochemical mechanisms occurring in Zizyphus germplasm under salinity stress.
Freshwater is depleting due to urbanization and industrialization. Irrigation with poor quality (brackish) water to fruit orchards is being practiced in arid and semi-arid regions of the world. The accumulation of salts in the soil revealed induced accumulation and uptake of toxic ions, and decreased uptake of essential minerals which contributed to growth and yield [9]. Salinity and sodicity both had adverse effects on soil and plant health. Sodicity is not only damaging to plants’ health, but it also depleting the soil texture and structure, porosity reduction, and poor water permeability. Under field conditions, irrigated water is recorded as electrical conductivity (EC) or osmotic potential. Pure water exhibited very poor conductance regarding electric current. The conductivity of water samples is mainly based on dissolved ions in water. EC and ESP were recorded higher. Osmotic pressure was measured lower under higher salt levels in water (Tables 1 and 2).
The salt content in the Colorado River about 2000 km downstream was 50 mg L−1, South California 900 mg L−1, and Texas 2000–3000 mg L−1 in the same river. All salinity levels are dangerous for plants, therefore numerous effective practices are necessary for the development and identification of tolerant germplasm of Zizyphus [13]. Disturbances in photosynthesis, metabolism, oxidative injury, and osmotic stress due to access to salts level in fruit trees as well explored (Fig. 1). Modulation of numerous physiological and biochemical mechanisms is necessary for the improvement of plant growth, yield and defense system under salinity stress (Fig. 2).
Figure 1: Zizyphus fruit tree responses under salinity stress
Figure 2: Zizyphus tolerance mechanism by the modulation of distinctive physiological and biochemical mechanisms under salinity stress
2 Uptake of Minerals Nutrition under Salt Stress
Nutrient absorption level is mainly based on root architecture. Exceeded salinity levels showed adverse effects on roots’ weight and size, and further disturbed the absorption of necessary nutrients that contributed to tree growth, development, yield, and quality [14]. The reduction in root characters, i.e., weight, number, and length decreased the crop yield due to restricted absorption of mineral nutrients [15]. Optimum conditions in the root zone were improving the minerals uptake and their transportation to other plant parts for food synthesis. The restriction in the uptake of higher concentrations of minerals (Na+/Cl−) via roots is effective for fruit trees by improving the plant defense system [15]. The uptake of these exceeded ionic concentrations reduces the production of antioxidant activities and secondary metabolites naturally within the fruit tree, and their cell compartments [1]. Nutrient minerals are up-taken via roots from the soil. The restriction in the uptake of minerals is ultimately dangerous for plant health, yield, size, and quality of Zizyphus. Nutrient deficiency and toxicity are major causes of crop failure growing in saline areas.
Under salinity, osmotic stress occurs due to restriction in water uptake from the root zone [16]. The cultivation of salt-tolerant germplasm is the only way to increase crop yield. Tolerant germplasm had excellent root systems which restrict the absorption, uptake, and transportation of toxic ions. The seedlings of Z. mauritiana were studied and evaluated that EC 15 dS m−1 was found to be more dangerous. Significant uptake and transportation of higher Na/Cl− ions were recorded due to induced-salinity concentrations in the root zone of Z. mauritiana as reported by Asrar et al. [17].
2.1 Restriction in Growth and Yield under Salt Stress
Excess NaCl level is reducing the growth and yield of Zizyphus germplasm. Induced-salinity in the root zone is causing nutritional imbalances and osmotic stress resulting in poor absorption of water and minerals necessary for sufficient growth, yield, and quality of Zizyphus plants [8]. Zizyphus plants showed stunted leaf and stem elongation, rupturing of cell membranes [8], disturbance in chlorophyll stability [18], increase of lipid peroxidation, low relative water content [18], rupturing of photosynthetic pigments [19], impairing gaseous exchange process [20], enhanced Na+ and Cl−, and reduction of K+ ions [21]. Zizyphus plants exhibited adaptive strategies by improved growth of roots for uptake of minerals and water [22], reduction in uptake of Na+ and Cl− [22], regulation of leaf turgidity [20], higher osmolytes generation and antioxidants production for scavenging of toxic ROS against salinity [6]. Therefore, it is more important to explore the importance of biochemical and molecular processes in Zizyphus plants subjected to salinity stress.
2.2 Salinity and ROS Generation
ROS production remains continue within plant cell compartments and organelles. The over-generation of ROS to the optimum level becomes more toxic for Zizyphus fruit trees [22]. Plant metabolism and oxidation process of biomolecules are disturbed due to higher concentrations of salts [23]. Over-production of ROS and disturbance in metabolic activities are major causes of oxidative stress. The interruption in the cellular homeostasis mechanism is damaging the biomolecules, cellular organelles, and redox homeostasis of fruit trees [1]. The oxidation process might be triggered due to salinity, damaging numerous proteins, sugars, lipids, and nucleic acids. The disturbed functioning of these bio-molecules due to the occurrence of oxidative stress is causing fruit tree death [24]. Electrochemical induced condition is also a cause of over-production of ROS and H2O2 within plants cells and organelles [25]. Hence, ROS over-production is dangerous for plants, therefore different management approaches are necessary to implement for improved fruit yield with superior fruit quality. The optimum production of ROS is contributed to fruit tree growth and is also involved in different developmental processes focusing on the fruit production of Zizyphus germplasm.
The regulation of stomatal conductance is important to improve the fruit tree’s tolerance against salinity stress. Elevated salinity has been causing stomatal closure and restriction of photosynthesis. Osmotic stress occurs due to alteration in stomatal enzymes and restriction in CO2 [26]. Photosynthetic pigments and chlorophyll content are involved in the regulation of photosynthesis [27]. The rupturing of photosynthetic reactions like photosystem II also disturbed the photosynthetic pigments and stomatal conductance, and ultimately fruit tree growth and yield. The impairing of electron transfer capacity is also a major cause of imbalance in photosynthetic machinery [28]. The alteration in metabolic processes is causing the restriction in photosynthesis. The optimum production of ROS is necessary for different metabolic activities and their proper functioning in the cells and organelles of fruit trees [29]. However, the over-generation of ROS becomes more toxic to plant’s health. ROS over-production increased the activities of MDA and H2O2 content which damages cell membranes [30]. The rupturing of membrane cells is very dangerous for plants’ survival against induced salinity stress [31]. Gaseous exchange and chlorophyll fluorescence are a major indicators of salt stress in plants growing under saline stress. The balance in gaseous exchange and chlorophyll fluorescence is necessary for the normal functioning of fruit trees attaining higher fruit yield with excellent quality fruits.
2.3 Salt Injury Involves Specific Ions Effects
Soil water potential is reduced due to low osmotic potential. Low osmotic potential is due to the presence of excess dissolved solutes in the root zone [32]. The development of a downhill mechanism between leaves and roots for the uptake of water is necessary for food synthesis in the leaves. The disturbance in the downhill gradient level is resulting in the reduced potential of food synthesis and translocation towards other plant parts [33]. Therefore, low water potential is toxic for sufficient plant growth and yield. The presence of higher dissolved solutes in the root zone are increasing the chances of water deficit conditions [34]. Induced salinity and water stress are reducing the fruit tree growth and poor yield with inferior fruit quality. Moreover, low water potential was also observed in the saline lands [35].
Ion toxicity occurs in the root zone due to increased concentrations of Na+ and Cl− in tree cells and organelles. Under normal conditions, the cytosol of higher trees is 1–10 mM Na+ and 100–200 mM K+. This ionic environment is optimum for the proper functioning of enzyme activities [5]. The increase/decrease from trees level is toxicity/deficit causing alteration in tree defense mechanism by inactivating numerous enzymes and protein biosynthesis. The higher concentration of Na+ depletes Ca2+ in the root zone which potentially increased the leakage of K+ from membrane cells [5]. The permeability of membranes is greatly damaged by induced salinity stress [36].
Higher concentrations of toxic ions such as Na+ and Cl− accumulated in chloroplasts under salinity stress. Electrolyte leakage indicates the sensitive/tolerant mechanism of fruit crops due to membrane damage from the over-production of ROS, H2O2, and MDA contents. Carbon metabolism/photophosphorylation disturbances in tree cell organelles and compartments were found to be higher in sensitive fruit cultivars [1]. Different antioxidants, osmolytes, and secondary metabolites are indicating tolerance levels off rut tress even at the seedling stage growing under saline conditions. The toxicity of different minerals in the root zone of fruit trees can be replaced through the ions exclusion strategy. Ions exclusion is important for the avoidance of salt injury. Ions exclusion and avoidance are the main approaches to protect fruit trees from the adverse effects of salinity focusing on fruit production [30].
Disturbances in cellular homeostasis are due to the toxicity of ions and the over-generation of ROS. The toxic ROS is a cause of osmotic stress. These ROS are causing the rupturing of membranes, DNA damage, carbohydrate oxidation, photosynthetic pigments breakdown, chlorophyll contents, protein denaturation, disturbance in antioxidant activities, and impairment of osmolytes production [37].
The exclusion of ions is considered one of the more critical aspects of the acclimation and adaptation of Zizyphus trees against salinity stress [16]. The balance through amino acids/carbohydrates utilization is considered a higher energy cost mechanism as compared to the use of ions for the balance of water potential [35]. Ionic balance is involved in the regulation of osmotic potential in Zizyphus trees. However, it has been assessed that higher concentrations of ions are more toxic for numerous cytosolic enzymes. Therefore, ions may possibly be accumulated in the vacuoles of cells to reduce toxic concentrations present in the cytosol [9].
Sodium chloride (NaCl) is one of the most abundant salts found in the soil. However, different transport systems are critically involved in the facilitation of Na+ into vacuole [26]. Potassium and calcium ions are greatly affecting the intracellular concentrations of Na+. High-affinity potassium transporters (HKTs) are greater affinity K+ transporters that belong to the class of integrated membrane proteins only present in plants. These HKTs may be varied in the differentiation of Na+/K+ due to higher selectivity for Na+ and a few others for K+ [1]. The uptake mechanism of Na+ was enhanced due to the inhibition of HKT1 and higher transport affinity of K+ and Na+ transporter under higher concentrations of Na+ and K+ [1]. Different mechanisms were involved in tree cells and compartments to increase tree tolerance against adverse growing conditions [31]. However, defense behavior is mainly based on the intensity of stress, type of species, and management practices. Calcium could increase the selectivity of K+/Na+ resulting in the enhanced tolerance mechanism of fruit trees [24].
Zizyphus fruit trees are facing multiple abiotic stresses in their living environment. The increase in the generation of ROS is causing oxidative injury within the plant cell compartments. Fruit trees could protect themselves by activating their natural defense system, however, the defense system of plants is very complex and needs deep exploitation for better understanding because this system could cope with multiple stresses [17]. Plant defense system is considered more inducible because it normally activates when plants faced environmental extremities [2]. Fruit tree resistance/tolerance germplasm is mainly based on the activation of the defense system as well as recognition of the prevailing stress situations coupled with rapid and effective initiation of oxidative defense reactions [7].
Oxidative injury is a major outcome of abiotic stresses i.e., salinity, drought, temperature extremities, heavy metals, and nutrient deficiency. Activation of oxidative enzymes under salinity had the potential to mitigate adverse effects of salinity in Zizyphus by lessening the oxidation of macromolecules, i.e., proteins, denaturing of nucleotide, and lipid peroxidation. Hence, the production of oxidative enzymes had the potential to scavenge toxic ROS under oxidative-induced conditions [38].
Z. nummularia and Z. rotundifolia both are wild species of Indian jujube. These species were found to be more tolerant against salinity because of greater CO2 assimilation, regulation of stomatal conductance, hormonal balance, activation, and accumulation of numerous antioxidants. Both species had the excellent capability in the translocation of required nutrients via roots. This is one of the basic reasons for utilizing both species as rootstocks [39]. Rootstock plays a major role in the translocation of toxic ions from roots to other plant parts. The graft union is also involved in the translocation of nutrients, and ions toward other plant parts in fruit trees [39]. The accumulation and transport of Na+ ions in excess from root to shoot are found to be much toxic for metabolism and photosynthesis [40]. Therefore, ion homeostasis regulation in cell compartments is imperative for sufficient growth, yield and superior quality of fruits.
The maximum Na+ accumulation was recorded in the roots of cv. Gola. Moreover, a higher accumulation of Na+ was measured in leaves of cv. Umran. Gola cultivar had better tolerance against salinity due to restricted translocation of Na+ from roots towards leaves. This cultivar could restrict Na+ for translocation towards other parts by maintaining ionic balance in leaves. Better plant metabolism, photosynthetic system, the activation of the defense system, and higher K+/Na+ ratio are major factors which identify the tolerance of fruit trees against adverse conditions, and regulation in all these factors weas observed in the Gola cultivar. Hence, this cultivar is more tolerant and can be grown in saline stress [41].
Different enzymatic activities, i.e., SOD, POD, CAT, APX, GPX, and GR, are stimulating naturally within cell compartments under salinity to improve tree defense mechanism. Moreover, enzymatic activities had the potential to scavenge toxic ROS by reducing disturbances in metabolism, and photosynthetic system [9].
SOD could remove exceeded amount of superoxide anions from cell compartments revealed in the improvement of plant defense systems [30]. SOD is present in three major forms, i.e., manganese SOD, copper/zinc SOD, and iron SOD (Fe-SOD) in higher plants. SOD had good potential to imbalance O2 from the formation of H2O2 because H2O2 is harmful to plants [9]. However, it is considered an indicator of stress conditions in those plants growing under saline conditions.
APX could remove the harmful production of H2O2 [42]. The scavenging of toxic ROS is very effective due to the production of APX activity in fruit trees. APX based on chloroplast mainly reduced the production of H2O2. Gene expression related to APX is encouraged through the ozone. So, it has been noted that tree tolerance can be enhanced with the increased activity of APX [43].
CAT is majorly present in glyoxylic acid-circulating bodies and peroxisomes in fruit trees that efficiently converted the H2O2 into H2O. Moreover, H2O2 is more toxic for the plants and CAT majorly reduces the level of H2O2. The generation of CAT activity within cell compartments indicated the tolerance of the fruit trees against salinity stress. The maximum production of CAT decreased the generation of H2O2 because H2O2 is the best indicator of salt stress [44].
Different non-enzymatic activities, i.e., ascorbic acid, different soluble sugars, flavonoids, phenols, and tocopherols are generated in fruit trees under saline conditions. These have excellent capability to scavenge toxic ROS over-produced under salinity. These antioxidants may possibly be varied regarding gene expression, achieved redox buffers, and metabolic boundary to control the optimum stimulus of acclimation reactions as studied by Miller et al. [45].
Ascorbic acid (vitamin C) is effective for the determination of tolerance levels in fruit trees growing under saline conditions. The increased production of ascorbic acid is effective for improved fruit tree tolerance against salinity stress. The improved antioxidants in fruit trees are important for the identification of tolerant rootstocks, and helpful for the restriction of Na+ from roots towards leaves. Moreover, the reduction in ascorbic acid was recorded in sensitive fruit trees however improved level was recorded in the tolerant germplasm of Zizyphus like Gola cultivar [46].
Tocopherol is recorded in all the plant parts. These have a good ability to scavenge toxic ROS and restrictlipid radicals. These important biological constituents are contributing to the antioxidant and non-antioxidant functioning. These are four isomers, i.e., δ_, α_, β_ and γ_ related to tocopherols are present in higher plants [47]. However, higher antioxidant activity was recorded in α-tocopherol also known as vitamin E. Moreover, α-tocopherol in considerable amounts is found in fruit trees of chloroplast membranes. Hence, photo-oxidative injury can be eliminated with the generation of tocopherols [48]. Tocopherols and carotenoids had the potential to repair oxidized radicals and restrict transmission chain duration during the auto-oxidation of lipids [49]. These are necessary for the protection of thylakoid membranes under salinity stress conditions in fruit trees. Protection of thylakoid membranes from injury is important for the proper functioning of a plant cell.
Flavonoids are rich in the aerial plant parts, i.e., foliage, pollens, floral, and fruit parts. These are frequently stored in vacuoles of fruit trees in the form of glycosides. These are also good scavengers of ROS in higher plants [30]. Moreover, it is also contributed to lipoxygenases, and reduced lipid peroxidation. Approximately, 1 mM of flavonoids had an excellent ability to restrict the lipoxygenase under saline conditions as studied by plant researchers Potapovich et al. [50].
Zizyphus fruit crop is a rich source of antioxidants and phenolic compounds. The consumption of fruits is important due to their nutritional components contributed to human’s good health [10]. The reduction in phenolic compounds was measured in leaves, roots, and fruits in those plants growing under saline environments. Nutritional characteristics of fruits are drastically disturbed because of the adverse effects of climatic conditions [12]. Oleuropein is a well known phenolic compound which involved in glucose reservoirs. This is necessary for the osmoregulation of fruit trees growing under saline conditions [51]. This is more helpful for the improvement of the fruit tree defense system of higher plants under salinity stress. The injurious due to Na+ and Cl− within the cell compartments can be reduced by the generation of these phenolics as oleuropeins in fruit trees [51].
Fruits of two species (Z. mauritiana and Z. jujube) are a rich source of different sugars than other species. However, the reduction in different sugars was also due to the adverse effects of abiotic stresses [52]. The reduction in sugars was observed in the leaves of fruit trees in those plants growing under salinity stress conditions. Sugars level may possibly be based on the type of genotypes and intensity of salt stress. Therefore, salt-resistant germplasm is necessary for superior quality, excellent fruit size, and nutritional profiling. Sucrose and glucose act like osmolytes, and are involved in cellular respiration as substrate [53]. Moreover, fructose is found to be supportive of the generation of secondary metabolites in salinity-stressed plants as studied by Rosa et al. [54]. The increase of sugars was observed in the tolerant plants, while a decreased level of sugars was recorded in the sensitive plants and this phenomenon was recorded in the Troyer citrange as well as in Cleopatra mandarin as reported by Anjum [55].
Over-generation of ROS, H2O2, MDA, and lipid peroxidation are considered as stress indicating markers in plants [37]. The timely control of salt-stressed plants is effective for higher production and yield by measuring these activities occurring within plant cells and compartments. The membrane injury was due to exceeding the level of salinity within plants is determined by measuring lipid peroxidation. The increased level of lipid peroxidation was indicating the increased level of injury in the membrane of plant cells [9].
3.2 Stress Signaling Molecules
Salinity can be detected by the estimation of stress-signaling molecules produced within cell compartments and organelles. Osmolytes are more effective in indicating salt stress in fruit trees [56]. Osmotic irregularity is found in the root zone of fruit trees due to exceeded concentration of salinity in the root zone and accumulation in leaves. Osmoprotectants have been produced within cell organelles and compartments naturally. However, their accumulation is effective to cope over-generation of toxic ROS and alleviates salt tolerance in fruit trees for better vegetative development, reproductive development, flowering induction, fruit yield, and superior fruit quality [57].
Different osmolytes, i.e., proline, GB, and ascorbates are produced naturally within plant cells to mitigate adverse effects of salinity by scavenging toxic ROS and are helpful for the activation of trees’ defense system [45]. Lipid peroxidation was reduced due to osmolytes generation. These are helpful to protect plant cells from injury to different membranes. Gene expression related to salinity stress is controlled by the production of these osmolytes naturally [58].
The suitable level of compatible osmolytes plays a major role in the regulation of osmotic gradient [59]. The accumulation of osmolytes, i.e., proline, GB, and ascorbates goes higher due to exceeded concentrations of salinity found in the root zone and is further translocated to other plant parts [60]. Even under abiotic stress, production and dramatic accumulation of proline, GB and ascorbates were exceeded naturally to cope with adverse effects that occur from harsh environmental conditions [61].
Proline is involved in stabilizing proteins, subcellular structures, and membranes protecting these from damaging of ROS. It is also known as a scavenger of toxic ROS [62]. The concentration of proline indicates that fruit species are tolerant, while sensitive genotypes had low proline concentration within cell compartments. Hence, proline generation is effective for the identification of tolerant/sensitive genotypes. However, different plant researchers also concluded that proline is a stress indicator which is not contributed to the alleviation of tolerance against salt stress [63].
Glycine betaine is another osmolyte produced in higher amounts in those fruit trees growing under saline stress [64]. It is greatly contributed to protein stability and photosynthetic pigments. The rupturing of photosynthetic machinery is protected by the natural production of GB [65]. Fruit quality and defense systems are also affected due to excess salinity (Table 3). However, its production might be increased by mineral application and cultivation of salt-tolerant germplasm.
3.3 Hormonal Regulation in Response to Salt Stress
Hormonal regulation is necessary to increase the tolerance against salinity stress in Zizyphus as reported in cucumber [66]. Two Zizyphus species (Z. rotundifolia and Z. nummularia) were found to be more tolerant against salinity stress because of better potential in hormonal regulation, stomatal conductance, and photosynthetic pigments [39]. Among exogenous plant hormones, auxin is more effective for the increase of tolerance against salinity stress by increasing the defense mechanism of fruit trees and the similar phenomenon was observed in cucumber [67]. The increased potential of antioxidant profiling is necessary to increase the scavenging capability of fruit trees against toxic ROS [68]. The reduction in MDA and H2O2 content is an indication of stress tolerance in fruit trees and the same phenomenon was observed in the Gola cultivar of Zizyphus [69]. Smart reprogramming using molecular basis [70] and hormonal regulation [71] of underutilized fruit crops under salinity stress is a more imperative strategy focusing on fruit production.
The increased population of the globe is further going to be enhanced in future. Hence, this is necessary and time demand to improve the productivity of fruit crops. There is a need for time to develop salt-tolerant germplasm to fulfill fruit requirements.
Minor fruit crops are neglected and need more consideration because these are rich sources of mineral nutrients. The cultivation of suitable germplasm according to climatic conditions is more vital for the higher economy of the country.
For sustainable fruit production, exploration of physiological and biochemical mechanisms can bring a great revolution and be found to be safer for the development of salt-tolerant germplasm to achieve “zero hunger”.
Salinity is considered one of the biggest challengings and constrain among all the abiotic stresses after drought adversely affecting the Zizyphus growth and yield.
Molecular insights should be further investigated for the development of higher-yielding cultivars and tolerance against salt stress. Modern biotechnological tools, i.e., proteomics, transcriptomics, metabolites, genome mapping, genome sequencing, quantitative trait loci (QTLs) mapping, genome editing, and genomic transformation are important for the development of salt resistant germplasm.
Physiological and biochemical mechanisms have been explored in the present study to provide detailed insights for the improvement of fruit tree tolerance against salinity stress. Gola cultivar among Zizyphus germplasm is found to be more tolerant against salt stress because of better regulating mechanism for photosynthetic machinery, antioxidant defense activities, osmolytes, compatible solutes, metabolites, and low oxidative stress are the basic reasons for his tolerant behavior. It has been recommended that these deeper insights can be explored and utilized by plant breeders for the development of salt-tolerant germplasm.
Funding Statement: The authors received no specific funding for this study.
Author Contributions: Riaz Ahmad and H. M. Din Muhammad designed and wrote the paper, Safina Naz, Ahsan Altaf and Meryam Manzoor reviewed and edit the manuscript.
Availability of Data and Materials: The availability of data is not applicable and all the required data included within the manuscript.
Conflicts of Interest: The authors declare that they have no conflicts of interest to report regarding the present study.
References
1. Ahmad, R., Anjum, M. A. (2020). Physiological and molecular basis of salinity tolerance in fruit crops. Fruit Crops, 36(5), 445–464. https://doi.org/10.1016/B978-0-12-818732-6.00032-0 [Google Scholar] [CrossRef]
2. Saqib, M., Anjum, M. A., Ali, M., Ahmad, R., Sohail, M. et al. (2022). Horticultural crops as affected by climate change. In: Building climate resilience in agriculture, pp. 95–109. Cham: Springer. https://doi.org/10.1007/978-3-030-79408-8_7 [Google Scholar] [CrossRef]
3. Haokip, S. W., Shankar, K., Lalrinngheta, J. (2020). Climate change and its impact on fruit crops. Journal of Pharmacognosy and Phytochemistry, 9(1), 435–438. [Google Scholar]
4. Aragüés, R., Medina, E. T., Martínez-Cob, A., Faci, J. (2014). Effects of deficit irrigation strategies on soil salinization and sodification in a semiarid drip-irrigated peach orchard. Agricultural Water Management, 142, 1–9. https://doi.org/10.1016/j.agwat.2014.04.004 [Google Scholar] [CrossRef]
5. Srivastava, A. K., Pasala, R., Minhas, P. S., Suprasanna, P. (2016). Plant bioregulators for sustainable agriculture: Integrating redox signaling as a possible unifying mechanism. Advances in Agronomy, 137, 237–278. https://doi.org/10.1016/bs.agron.2015.12.002 [Google Scholar] [CrossRef]
6. Hesami, A., Bazdar, L., Shahriari, M. H. (2020). Effect of soil salinity on growth, proline, and some nutrient accumulation in two genotypes seedlings of Ziziphus Spina-christi (L.) willd. Communications in Soil Science and Plant Analysis, 51(6), 804–815. https://doi.org/10.1080/00103624.2020.1729366 [Google Scholar] [CrossRef]
7. Sherani, J., Jillani, M. S., Ahmad, T. (2017). Ber (Zizyphus mauritiana L.) production and quality as influenced by different salinity levels in water. Pakistan Journal of Agricultural Sciences, 54(3), 645–652. https://doi.org/10.21162/PAKJAS/17.6192 [Google Scholar] [CrossRef]
8. Singh, A., Kumar, A., Kumar, R., Sheoran, P., Yadav, R. K. et al. (2022). Multivariate analyses discern shared and contrasting eco-physiological responses to salinity stress of Ziziphus rootstocks and budded trees. South African Journal of Botany, 146(4), 573–584. https://doi.org/10.1016/j.sajb.2021.11.049 [Google Scholar] [CrossRef]
9. Muhammad, H. M. D., Abbas, A., Ahmad, R. (2022). Fascinating role of silicon nanoparticles to mitigate adverse effects of salinity in fruit trees: A mechanistic approach. Silicon, 14(14), 1–8. https://doi.org/10.1007/s12633-021-01604-4 [Google Scholar] [CrossRef]
10. Anjum, M. A., Rauf, A., Bashir, M. A., Ahmad, R. (2018). The evaluation of biodiversity in some indigenous Indian jujube (Zizyphus mauritiana) germplasm through physico-chemical analysis. Acta Scientiarum Polonorum Hortorum Cultus, 17(4), 39–52. https://doi.org/10.24326/asphc.2018.4.4 [Google Scholar] [CrossRef]
11. Ahmad, R., Malik, W., Anjum, M. A. (2019). Genetic diversity and selection of suitable molecular markers for characterization of indigenous Zizyphus germplasm. Erwerbs-Obstbau, 61(4), 345–353. https://doi.org/10.1007/s10341-019-00438-0 [Google Scholar] [CrossRef]
12. Anjum, M. A., Haram, A., Ahmad, R., Bashir, M. A. (2020). Physico-chemical attributes of fresh and dried Indian jujube (Zizyphus mauritiana) fruits. Pakistan Journal of Agricultural Sciences, 57(1), 165–176. https://doi.org/10.21162/PAKJAS/20.7845 [Google Scholar] [CrossRef]
13. Gupta, N. K., Meena, S. K., Gupta, S., Khandelwal, S. K. (2002). Gas exchange, membrane permeability, and ion uptake in two species of Indian jujube differing in salt tolerance. Photosynthetica, 40(4), 535–539. https://doi.org/10.1023/A:1024343817290 [Google Scholar] [CrossRef]
14. Parihar, P., Singh, S., Singh, R., Singh, V. P., Prasad, S. M. (2015). Effect of salinity stress on plants and its tolerance strategies: A review. Environmental Science and Pollution Research, 22(6), 4056–4075. https://doi.org/10.1007/s11356-014-3739-1 [Google Scholar] [PubMed] [CrossRef]
15. Zait, Y., Shtein, I., Schwartz, A. (2019). Long-term acclimation to drought, salinity and temperature in the thermophilic tree Ziziphus spina-christi: Revealing different tradeoffs between mesophyll and stomatal conductance. Tree Physiology, 39(5), 701–716. https://doi.org/10.1093/treephys/tpy133 [Google Scholar] [PubMed] [CrossRef]
16. Li, M., Guo, Y., Liu, S., Zhao, Y., Pang, X. et al. (2019). Autotetraploidization in Ziziphus jujuba mill. var. spinosa enhances salt tolerance conferred by active, diverse stress responses. Environmental and Experimental Botany, 165(4), 92–107. https://doi.org/10.1016/j.envexpbot.2019.05.016 [Google Scholar] [CrossRef]
17. Asrar, S., Zahoor, A. (2011). Effect of root zone salinity on mineral nutrition and growth of beri (Zizyphus mauritiana lam) and jaman (Eugenia jambolana lamk). Journal of Horticulture and Forestry, 3(12), 366–371. [Google Scholar]
18. Bagdi, D. L., Bagri, G. K. (2016). Effect of saline irrigation water on gas exchange and proline metabolism in ber (Ziziphus). Journal of Environmental Biology, 37(5), 873. [Google Scholar] [PubMed]
19. Gorai, M., Romdhane, R., Maraghni, M., Neffati, M. (2019). Relationship between leaf gas-exchange characteristics and the performance of Ziziphus spina-christi (L.) wild. seedlings subjected to salt stress. Photosynthetica, 57(3), 897–903. https://doi.org/10.32615/ps.2019.093 [Google Scholar] [CrossRef]
20. Nejat, N., Sadeghi, H. (2012). Response of Ziziphus spina-christi (L.) wild seedlings to NaC-induced salinity. Agricultural Science Digest, 32(1), 61–65. [Google Scholar]
21. Meir, M., Zaccai, M., Raveh, E., Ben-Asher, J., Tel-Zur, N. (2014). Performance of Ziziphus jujuba trees correlates with tissue mineral content under salinity conditions. Agricultural Water Management, 142, 47–55. https://doi.org/10.1016/j.agwat.2014.05.002 [Google Scholar] [CrossRef]
22. Bhatt, M. J., Patel, A. D., Bhatti, P. M., Pandey, A. N. (2008). Effect of soil salinity on growth, water status and nutrient accumulation in seedlings of Ziziphus mauritiana (Rhamnaceae). Journal of Fruit and Ornamental Plant Research, 16(1), 383–401. [Google Scholar]
23. Yang, M., Zhang, F., Wang, F., Dong, Z., Cao, Q. et al. (2015). Characterization of a type 1 metallothionein gene from the stresses-tolerant plant Ziziphus jujuba. International Journal of Molecular Sciences, 16(8), 16750–16762. https://doi.org/10.3390/ijms160816750 [Google Scholar] [PubMed] [CrossRef]
24. Li, M., Zhang, C., Hou, L., Yang, W., Liu, S. et al. (2021). Multiple responses contribute to the enhanced drought tolerance of the autotetraploid Ziziphus jujuba mill. var. spinosa. Cell and Bioscience, 11(1), 1–20. https://doi.org/10.1186/s13578-021-00633-1 [Google Scholar] [PubMed] [CrossRef]
25. Sharma, P., Jha, A. B., Dubey, R. S., Pessarakli, M. (2012). Reactive oxygen species, oxidative damage, and antioxidative defense mechanism in plants under stressful conditions. Journal of Botany, 2012, 1–26. https://doi.org/10.1155/2012/217037 [Google Scholar] [CrossRef]
26. Fu, X., Zhang, J., Zhou, L., Mo, W., Wang, H. et al. (2022). Characterizing the development of photosynthetic capacity in relation to chloroplast structure and mineral nutrition in leaves of three woody fruit species. Tree Physiology, 42(5), 989–1001. https://doi.org/10.1093/treephys/tpab154 [Google Scholar] [PubMed] [CrossRef]
27. Arndt, S. K., Clifford, S. C., Wanek, W., Jones, H. G., Popp, M. (2001). Physiological and morphological adaptations of the fruit tree Ziziphus rotundifolia in response to progressive drought stress. Tree Physiology, 21(11), 705–715. https://doi.org/10.1093/treephys/21.11.705 [Google Scholar] [PubMed] [CrossRef]
28. Liu, Z., Zhao, J., Liu, M. (2016). Photosynthetic responses to phytoplasma infection in Chinese jujube. Plant Physiology and Biochemistry, 105(2), 12–20. https://doi.org/10.1016/j.plaphy.2016.04.003 [Google Scholar] [PubMed] [CrossRef]
29. Gadi, B. R. (2016). Effect of fluoride on metabolic patterns and nitrate reductase activity in Ziziphus seedlings. Journal of Global Biosciences, 5, 3694–3698. [Google Scholar]
30. Ahmad, R., Hussain, S., Anjum, M. A., Khalid, M. F., Saqib, M. et al. (2019). Oxidative stress and antioxidant defense mechanisms in plants under salt stress. Plant Abiotic Stress Tolerance, 191–205. https://doi.org/10.1007/978-3-030-06118-0_8 [Google Scholar] [CrossRef]
31. Wang, B., Wan, Y., Zheng, Y., Lee, X., Liu, T. et al. (2019). Alginate-based composites for environmental applications: A critical review. Critical Reviews in Environmental Science and Technology, 49(4), 1–39. https://doi.org/10.1080/10643389.2018.1547621 [Google Scholar] [PubMed] [CrossRef]
32. Jia, J., Liang, Y., Gou, T., Hu, Y., Zhu, Y. et al. (2020). The expression response of plasma membrane aquaporins to salt stress in tomato plants. Environmental and Experimental Botany, 178, 104190. https://doi.org/10.1016/j.envexpbot.2020.104190 [Google Scholar] [CrossRef]
33. Moles, T. M., Pompeiano, A., Reyes, T. H., Scartazza, A., Guglielminetti, L. (2016). The efficient physiological strategy of a tomato landrace in response to short-term salinity stress. Plant Physiology and Biochemistry, 109, 262–272. https://doi.org/10.1016/j.plaphy.2016.10.008 [Google Scholar] [PubMed] [CrossRef]
34. Song, Q., Joshi, M., Joshi, V. (2020). Transcriptomic analysis of short-term salt stress response in watermelon seedlings. International Journal of Molecular Sciences, 21(17), 6036. https://doi.org/10.3390/ijms21176036 [Google Scholar] [PubMed] [CrossRef]
35. Hasanuzzaman, M., Bhuyan, M. H. M., Zulfiqar, F., Raza, A., Mohsin, S. M. et al. (2020). Reactive oxygen species and antioxidant defense in plants under abiotic stress: Revisiting the crucial role of a universal defense regulator. Antioxidants, 9(8), 681. https://doi.org/10.3390/antiox9080681 [Google Scholar] [PubMed] [CrossRef]
36. Alam, H., Khattak, J. Z., Ksiksi, T. S., Saleem, M. H., Fahad, S. et al. (2021). Negative impact of long-term exposure of salinity and drought stress on native Tetraena mandavillei L. Physiologia Plantarum, 172(2), 1336–1351. https://doi.org/10.1111/ppl.13273 [Google Scholar] [PubMed] [CrossRef]
37. Shahzad, S., Ali, S., Ahmad, R., Ercisli, S., Anjum, M. A. (2022). Foliar application of silicon enhances growth, flower yield, quality and postharvest life of tuberose (Polianthes tuberosa L.) under saline conditions by improving antioxidant defense mechanism. Silicon, 14(4), 1511–1518. https://doi.org/10.1007/s12633-021-00974-z [Google Scholar] [CrossRef]
38. Demidchik, V. (2015). Mechanisms of oxidative stress in plants: From classical chemistry to cell biology. Environmental and Experimental Botany, 109, 212–228. https://doi.org/10.1016/j.envexpbot.2014.06.021 [Google Scholar] [CrossRef]
39. Meena, S. K., Gupta, N. K., Gupta, S., Khandelwal, S. K., Sastry, E. V. D. (2003). Effect of sodium chloride on the growth and gas exchange of young Ziziphus seedling rootstocks. Journal of Horticultural Sciences and Biotechnology, 78(4), 454–457. https://doi.org/10.1080/14620316.2003.11511649 [Google Scholar] [CrossRef]
40. Chakraborty, K., Sairam, R. K., Bhattacharya, R. C. (2012). Differential expression of salt overly sensitive pathway genes determines salinity stress tolerance in Brassica genotypes. Plant Physiology and Biochemistry, 51, 90–101. https://doi.org/10.1016/j.plaphy.2011.10.001 [Google Scholar] [PubMed] [CrossRef]
41. Agrawal, R., Gupta, S., Gupta, N. K., Khandelwal, S. K., Bhargava, R. (2013). Effect of sodium chloride on gas exchange, antioxidative defense mechanism and ion accumulation in different cultivars of Indian jujube (Ziziphus mauritiana L.). Photosynthetica, 51(1), 95–101. https://doi.org/10.1007/s11099-013-0003-8 [Google Scholar] [CrossRef]
42. Wang, H., Ye, X., Li, J., Tan, B., Chen, P. et al. (2018). Transcriptome profiling analysis revealed co-regulation of multiple pathways in jujube during infection by ‘Candidatus Phytoplasma ziziphi’. Gene, 665(1), 82–95. https://doi.org/10.1016/j.gene.2018.04.070 [Google Scholar] [PubMed] [CrossRef]
43. Sofo, A., Scopa, A., Nuzzaci, M., Vitti, A. (2015). Ascorbate peroxidase and catalase activities and their genetic regulation in plants subjected to drought and salinity stresses. International Journal of Molecular Sciences, 16(12), 13561–13578. https://doi.org/10.3390/ijms160613561 [Google Scholar] [PubMed] [CrossRef]
44. Ashraf, M. (2009). Biotechnological approach of improving plant salt tolerance using antioxidants as markers. Biotechnological Advances, 27(1), 84–93. https://doi.org/10.1016/j.biotechadv.2008.09.003 [Google Scholar] [PubMed] [CrossRef]
45. Miller, G. A. D., Suzuki, N., Yilmaz, C. S., Mittler, R. O. N. (2010). Reactive oxygen species homeostasis and signaling during drought and salinity stresses. Plant Cell and Environment, 33(4), 453–467. https://doi.org/10.1111/j.1365-3040.2009.02041.x [Google Scholar] [PubMed] [CrossRef]
46. Bai, T., Ma, P., Li, C., Yin, R., Ma, F. (2013). Role of ascorbic acid in enhancing hypoxia tolerance in roots of sensitive and tolerant apple rootstocks. Scientia Horticulturae, 164, 372–379. https://doi.org/10.1016/j.scienta.2013.10.003 [Google Scholar] [CrossRef]
47. Ma, J., Qiu, D., Pang, Y., Gao, H., Wang, X. et al. (2020). Diverse roles of tocopherols in response to abiotic and biotic stresses and strategies for genetic biofortification in plants. Molecular Breeding, 40(2), 1–15. https://doi.org/10.1007/s11032-019-1097-x [Google Scholar] [CrossRef]
48. Shabala, S., Munns, R. (2017). Salinity stress: Physiological constraints and adaptive mechanisms. In: Plant stress physiology, pp. 24–63. Wallingford, USA: CABI. https://doi.org/10.1079/9781780647296.002 [Google Scholar] [CrossRef]
49. Sairam, R. K., Tyagi, A. (2004). Physiological and molecular biology of salinity stress tolerance in plants. Current Science, 86, 407–420. [Google Scholar]
50. Potapovich, A. I., Kostyuk, V. A. (2003). Comparative study of antioxidant properties and cytoprotective activity of flavonoids. Biochemistry, 68(5), 514–519. https://doi.org/10.1023/A:1023947424341 [Google Scholar] [PubMed] [CrossRef]
51. Mohammadkhani, N. (2018). Effects of salinity on phenolic compounds in tolerant and sensitive grapes. Agriculture and Forestry, 64(2), 73–86. https://doi.org/10.17707/AgricultForest.64.2.05 [Google Scholar] [CrossRef]
52. Ruiz, M., Quiñones, A., Martínez-Alcántara, B., Aleza, P., Morillon, R. et al. (2016). Effects of salinity on diploid (2x) and doubled diploid (4x) Citrus macrophylla genotypes. Scientia Horticulturae, 207, 33–40. https://doi.org/10.1016/j.scienta.2016.05.007 [Google Scholar] [CrossRef]
53. Schmutz, U. (2000). Effect of salt stress (NaCl) on whole plant CO2-gas exchange in mango. Acta Horticulturae, 509, 269–276. https://doi.org/10.17660/ActaHortic.2000.509.29 [Google Scholar] [CrossRef]
54. Rosa, M., Prado, C., Podazza, G., Interdonato, R., González, J. A. et al. (2009). Soluble sugars: Metabolism, sensing and abiotic stress: A complex network in the life of plants. Plant Signaling Behavior, 4(5), 388–393. https://doi.org/10.4161/psb.4.5.8294 [Google Scholar] [PubMed] [CrossRef]
55. Anjum, M. A. (2008). Effect of NaCl concentrations in irrigation water on growth and polyamine metabolism in two citrus rootstocks with different levels of salinity tolerance. Acta Physiologiae Plantarum, 30(1), 43–52. https://doi.org/10.1007/s11738-007-0089-3 [Google Scholar] [CrossRef]
56. Rabey, E. H. A., Al-Malki, A. L., Abulnaja, K. O. (2016). Proteome analysis of date palm (Phoenix dactylifera L.) under severe drought and salt stress. International Journal of Genomics, 2016(22), 1–8. https://doi.org/10.1155/2016/7840759 [Google Scholar] [PubMed] [CrossRef]
57. Munns, R. (2002). Comparative physiology of salt and water stress. Plant Cell and Environment, 25(2), 239–250. https://doi.org/10.1046/j.0016-8025.2001.00808.x [Google Scholar] [PubMed] [CrossRef]
58. Soni, A., Dhakar, S., Kumar, N. (2017). Mechanisms and strategies for improving salinity tolerance in fruit crops. International Journal of Current Microbiology and Applied Sciences, 6(8), 1917–1924. [Google Scholar]
59. Lima, L. K. D. S., de Jesus, O. N., Soares, T. L., dos Santos, I. S., de Oliveira, E. J. et al. (2020). Growth, physiological, anatomical and nutritional responses of two phenotypically distinct passion fruit species (Passiflora L.) and their hybrid under saline conditions. Scientia Horticulturae, 263, 109037. https://doi.org/10.1016/j.scienta.2019.109037 [Google Scholar] [CrossRef]
60. Mittler, R. (2002). Oxidative stress, antioxidants and stress tolerance. Trends in Plant Science, 7(9), 405–410. https://doi.org/10.1016/S1360-1385(02)02312-9 [Google Scholar] [PubMed] [CrossRef]
61. Rains, D. W., Epstein, E., Zasoski, R. J., Aslam, M. (2006). Active silicon uptake by wheat. Plant and Soil, 280(1), 223–228. https://doi.org/10.1007/s11104-005-3082-x [Google Scholar] [CrossRef]
62. Mittler, R., Vanderauwera, S., Gollery, M., van Breusegem, F. (2004). Reactive oxygen gene network of plants. Trends in Plant Science, 9(10), 490–498. https://doi.org/10.1016/j.tplants.2004.08.009 [Google Scholar] [PubMed] [CrossRef]
63. Rahneshan, Z., Nasibi, F., Moghadam, A. A. (2018). Effects of salinity stress on some growth, physiological, biochemical parameters and nutrients in two pistachio (Pistacia vera L.) rootstocks. Journal of Plant Interaction, 13(1), 73–82. https://doi.org/10.1080/17429145.2018.1424355 [Google Scholar] [CrossRef]
64. Karimi, H. R., Kuhbanani, A. M. (2015). The evaluation of inter-specific hybrid of P. atlantica P. vera cv. ‘Badami Zarand’ as a pistachio rootstock to salinity stress. Journal of Nutrition, 6, 113–122. [Google Scholar]
65. Abdollahi, F., Takhti, G. S. (2013). Effect of GA3 on growth and chemical composition of jujube leaf (Ziziphus spina-christi) under salinity condition. Journal of Plant Process and Function, 2, 53–64. [Google Scholar]
66. Ahmad, R., Anjum, M. A., Balal, R. M. (2020). From markers to genome based breeding in horticultural crops: An overview. Phyton-International Journal of Experimental Botany, 89(2), 183–204. https://doi.org/10.32604/phyton.2020.08537 [Google Scholar] [CrossRef]
67. Sharif, R., Su, L., Chen, X., Qi, X. (2022). Involvement of auxin in growth and stress response of cucumber. Vegetable Research, 2, 1–8. [Google Scholar]
68. Mansour, M. M. F. (2000). Nitrogen containing compounds and adaptation of plants to salinity stress. Biologia Plantarum, 43(4), 491–500. https://doi.org/10.1023/A:1002873531707 [Google Scholar] [CrossRef]
69. Verma, S. S., Verma, R. S., Verma, S. K., Yadav, A. L., Verma, A. K. (2018). Impact of salt stress on plant establishment, chlorophyll and total free amino acid content of ber (Zizyphus mauritiana Lamk.) cultivars. Journal of Pharmacognosy and Phytochemistry, 7, 556–559. [Google Scholar]
70. Raza, A., Tabassum, J., Fakhar, A. Z., Sharif, R., Chen, H. et al. (2022). Smart reprograming of plants against salinity stress using modern biotechnological tools. Critical Reviews in Biotechnology, 1–28. https://doi.org/10.1080/07388551.2022.2093695 [Google Scholar] [PubMed] [CrossRef]
71. Raza, A., Salehi, H., Rahman, M. A., Zahid, Z., Madadkar, H. M. et al. (2022). Plant hormones and neurotransmitter interactions mediate antioxidant defenses under induced oxidative stress in plants. Frontiers in Plant Science, 13. https://doi.org/10.3389/fpls.2022.961872 [Google Scholar] [PubMed] [CrossRef]
Cite This Article
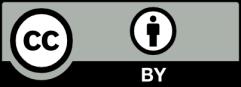