Open Access
ARTICLE
Evaluation of Pre-Emergence and Post-Emergence Herbicides for Weed Management in Miscanthus sacchariflorus and Miscanthus sinensis
1 Department of Crop Science, College of Sanghuh Life Science, Konkuk University, Seoul, 05029, Korea
2 Bioherb Research Institute, Kangwon National University, Chuncheon, 24341, Korea
* Corresponding Author: Ill Min Chung. Email:
(This article belongs to the Special Issue: Integrating Agronomy and Plant Physiology for Improving Crop Production)
Phyton-International Journal of Experimental Botany 2023, 92(5), 1439-1467. https://doi.org/10.32604/phyton.2023.023076
Received 11 April 2022; Accepted 28 November 2022; Issue published 09 March 2023
Abstract
Miscanthus, is a promising bioenergy crop, considered superior to other bioenergy crops because of its higher water and nutrient use efficiency, cold tolerance, and higher production of biomass. Broadleaf weeds and grass weeds, cause major problems in the Miscanthus field. A field experiment was conducted in 2018 and 2019, to assess the effects of pre-emergence (alachlor and napropamide) and post-emergence herbicides (nicosulfuron, dicamba, bentazon, and glufosinate ammonium) on broadleaf and grass weeds in M. sinensis and M. sacchariflorus fields. The weed control efficiency and phytotoxicity of pre- and post-emergence herbicides were evaluated at 30 days after treatment (DAT) and compared to those of the control plots. The results showed wide variations in the susceptibility of the weed species to the treated herbicides. Treatment with nicosulfuron 40 g.a.i.ha−1 provided the most effective overall weed control (with 10% visual injury), without affecting the height and biomass of neither Miscanthus species in the field. Post-emergence herbicides such as glufosinate ammonium 400 g.a.i.ha−1 and dicamba 482 g.a.i.ha−1 were effective and inhibited the growth and density of the majority of weeds to a 100%; however, they showed significant phytotoxicity (toxicity scale of 1–10) to both species of Miscanthus. The application of glufosinate ammonium caused severe injuries to the foliar region (90% visual injury) of both Miscanthus sps. Comparatively, M. sinensis showed a slightly higher tolerance to the herbicides nicosulfuron, bentazon and napropamide with 10% visual injury at the recommended dose than M. sacchariflorus. The present study clearly showed that infestation of broadleaf and grass weeds in Miscanthus fields can cause significant damage to the growth and biomass of Miscanthus and applying pre-emergence and post-emergence herbicides effectively controls the high infestation of these weeds.Keywords
Supplementary Material
Supplementary Material FileMiscanthus sacchariflorus (Maxim.) Hack., a rhizomatous C4 grass species, also called as silver banner grass has received considerable attention as a potential bioenergy crop because of its high biomass production [1]. M. sacchariflorus has a broad geographic range (up to 50° N in Eastern Russia) [2–4]. It has also been investigated as a feedstock, for ensilage, large-scale paper production [5–7] and higher bioremediation capacity of heavy metals [7]. The vigorous rhizomes of M. sacchariflorus are useful in preventing soil erosion [8]. Some ornamental accessions of M. sacchariflorus are sold in nurseries [9] and the inflorescence is used as a winter bouquet [10]. Its annual biomass production is higher than any other capable perennial bioenergy crop [7]. It is fairly tolerant to a wide range of environmental conditions and propagates in varied water and nitrogen contents with minimal input [5,11–13]. Miscanthus sinensis Andersson (Poaceae), a C4 perennial bioenergy crop and also called Chinese silver grass is native to Asia, primarily in China, Korea and Japan, and grows in a wide range of environments and altitudes (from sea level to 2500 m) [14,15]. They originate in a region ranging as far north as Sakhalin and as far south as the Indochinese peninsula [4]. It is suitable for thatching, animal feeds and the production of compost, sold as an ornamental plant in nurseries, and as raw material for industrial use for the production of paper pulp [15].
Both species differ morphologically, phylogenetically, and ecologically [14,15]. Compared to M. sacchariflorus, the accessions of M. sinensis are not an aggressive competitor at the early growing stages due to a weak rhizome spread [12,16]. They occasionally grow in the same area forming a sympatric population from ~29° N to 43° N [3], and are considered a promising renewable biomass resource [14,17]. M. sinensis usually has a caespitose form, prefers aerobic soils and grows mostly in hills, and seminatural grasslands on sloppy lands [18] and is typically diploid with a monoploid genome size of about 2.5–2.8 pg. M. sinensis is an early colonizer [15,19] with great cold tolerance and yield in cold regions [15,20,21], whereas M. sacchariflorus, which has a rhizomatous habit, prefers to grow under wetter soil conditions, in riparian environments, on river banks, mostly on mesic, viscid, sandy soil formed by floodings, and dominates fertile lowland habitats on alluvial plains [14,22,23]. M. sacchariflorus can be diploid or tetraploid with a monoploid genome size of about 2.1–2.3 pg [22,24,25]. The population of M. sacchariflorus from China and Korea are generally diploid [26,27], whereas its accessions from Japan are predominantly tetraploid [28]. M. sacchariflorus flowers less readily than M. sinensis [11,29,30] and produce a lower number of stems per plant (average 26 stems during 3rd year of cultivation) than M. sinensis (average 49 stems) [31]. Climate change is posing a serious threat to the global environment. Fossil fuels emit a huge amount of polluting gases contributing to an increased greenhouse effect, triggering environmental pollution and climate change [32]. Due to the fast depletion of fossil reserves and the rapid growth of the world population, the demand for bioenergy is expected to increase by 10 fold by 2050 in both energy and transport sectors [33,34]. Therefore, the use of renewable energy (bioenergy) is inevitable and must be carried out in a sustainable way to reduce the environmental impacts [35]. Miscanthus is characterized by a rapid growth, and thus a high productivity, that can be grown with a low input of nutrients and water consumption, and may confer a high capacity to sequester carbon in the soil that enables to mitigate the global warming by reducing greenhouse gas emissions [35].
Weeds are a major threat to the growth of bioenergy crops as they constantly compete with them for nutrients, space, sunlight, and moisture. Some important broadleaf weeds and grass weeds that commonly grow in Miscanthus fields contribute to the loss of biomass yield of the crop [36]. The growth rate of Miscanthus, at the beginning of the establishment year, is slow and sensitive to weed interference [36], which reduces its ability to compete with weeds for growth. Previous studies on Miscanthus revealed difficulties in its establishment due to prolonged and various degrees of dormancy, cold stress, and water deficiency during growth [36]. These plants take considerable time to fully develop the canopy and fill the wide gap between seedlings [36]. The bare land between the seedlings resulting from low densities allows weeds to grow and compete with the young shoots of Miscanthus. In the absence of weed control, approximately a 97% reduction in M. sacchariflorus biomass yield was reported under field conditions by grass weeds such as Echinochloa crus-galli, Digitaria sanguinalis, Setaria viridis, and broadleaf weeds including Stellaria aquatic, Amaranthus lividus, Galinsoga ciliate, Calystegia sepium, Chenopodium album, Acalypha australis, Viola mandshurica, Rorippa palustris, Ipomoea hederacea and Trifolium repens [36]. The higher rate of competition of Miscanthus plants with weeds was observed during the first year of growth of Miscanthus in the field, and it was reduced in the subsequent years [37–41]. As the rate of growth of these weeds is higher than that of Miscanthus, it has a detrimental effect on the growth of the crop due to the deprivation of nutrients, space, light, and moisture. In the absence of a proper weed control system, young shoots and canopy die, show poor growth, or produce less biomass. Therefore, controlling or suppressing weed growth at or before the critical point of weed growth not only minimizes yield loss but also protects plants from diseases. Moreover, several previous studies indicated an improvement in the biomass of bioenergy crops by using pre-emergence or post-emergence herbicides [42,43].
Previous work on weed control on these perennial crops has mainly focused on Miscanthus × giganteus, which is widely cultivated in the UK, European countries and USA for biomass production [1,44]. Several pre-emergence and post-emergence herbicides have been tested for use in the early growth of Miscanthus × giganteus [1,40,41,44]. The herbicides such as pendimethalin, S-metolachlor, and isoxaflutole, applied at planting, and POST herbicides, such as 2,4-D (1060 g ai ha–1), bromoxynil (840 g ai ha–1), and dicamba (560 g ai ha–1) were found to be effective on controlling the weeds and safety for Miscanthus × giganteus [44]. Other herbicides such as atrazine were effective in controlling a wide range of broadleaves weeds, whereas, acetochlor was less effective in controlling the emerged weeds (68%) in a Miscanthus × giganteus field [45]. Recently, interest in M. sinensis and M. sacchariflorus has been increased for use as biomass energy crops in Europe, North America, and South Korea for productivity trials [44,46,47]. However, very few field studies have been conducted on weed control systems for M. sacchariflorus using pre-and post-emergence herbicides [36]. Also, no study has comparatively evaluated herbicide-based weed management systems for M. sinensis and M. sacchariflorus under field conditions. This research hypothesized that (1) all the tested herbicides will reduce the emergence of weeds, sustain toxic effects and lowers the survivability of the distinct weed community of a M. sinensis and M. sacchariflorus field, (2) mulches pretreated with pre-emergence herbicides would impact early Miscanthus growth and development but have no adverse effect on yield, and (3) mulches pretreated with the pre-emergence and post-emergence herbicides would provide effective weed control by reducing the density and biomass (FW and DW) of weeds. Therefore, the main objective of the present study was to determine the effectiveness of specific pre-and post-emergence herbicides to control weeds. In addition, we investigated the safety of pre-and post-emergence applications of herbicides on M. sinensis and M. sacchariflorus under field conditions, and also evaluated the phytotoxicity of herbicides on M. sinensis and M. sacchariflorus.
The bioefficacy of pre-and post-emergence herbicides was evaluated in the M. sinensis and M. sacchariflorus fields at the Kangwon National University, South Korea, (37°56009.96″ N; 127°46055.21″ E; Fig. 1) at an average altitude of 100 m for two years (during the years 2018, 2019). Two different plots were used to study the effect of herbicides on the two species of Miscanthus and weeds. Meteorological data were obtained from the database of the Korea Meteorological Administration. The average temperature of the cultivated field ranged from −9.9°C (January) to 29.8°C (August). Soil samples in each plot were collected in April 2018 after plot construction by randomly selecting three sampling points. Briefly, 10 g of soil samples (10–20 cm) were gathered using a sterilized spatula. The collected soil samples were placed in individual whirl-pack bags and immediately transported to laboratory on ice at 4°C until use. Before the analysis, soil samples were air-dried, milled and sieved using a 2 mm sieve. The pH value and the characteristics of the soil samples were determined in the Department of Biological Environment, Kangwon National University, Chuncheon 24341, South Korea by following the methods described by Kim et al. [48].
Figure 1: Location of experimental plots for the weed management in M. sinensis and M. sacchariflorus field
The soil of the experimental field had a sandy loam texture. The M. sinensis and M. sacchariflorus experimental field was irrigated during the initial year of establishment on a regular basis. The research field was located in a temperate monsoon climate, with a wet and humid summer. In the first set of experiments, the harvested rhizomes of M. sinensis and M. sacchariflorus were planted 8 cm deep in the field (100 m × 100 m in size) in the year 2015. The same M. sinensis and M. sacchariflorus accessions were maintained at the experimental field until 2017 and transferred to the plots for herbicides treatment. There were six herbicide treatments and a control (without herbicide treatments) in each plot. Each experimental plot consisted of six rows of 10 m in length, spaced 1 m apart. Each row contained eight plants (in a 10 m length row), with an inter-plant distance of 1.25 m. The treatments consisted of two pre-emergence herbicides and four post-emergence herbicides (applied in recommended standard doses). Pre-emergence herbicides (alachlor and napropamide) were sprayed a week after planting M. sinensis and M. sacchariflorus in the field. Post-emergence herbicides (nicosulfuron, dicamba, bentazon, and glufosinate ammonium) were sprayed 30 days after planting the Miscanthus spp. (Table 1). None treated Miscanthus spp. in the areas were considered as the control for evaluating injury. The field experiments were a randomized block design, each with three replications. All the herbicides were applied within two days of planting Miscanthus plants. Both pre- and post-emergence herbicides were applied using a pressurized backpack sprayer equipped with a flat fan nozzle fitted to a knapsack stainless steel sprayer (MT-009, Taizhou, Zhejiang, China) with the capacity of 18 L at 1.0 MPa. The amount of herbicide sprayed on the Miscanthus spp. was based on the recommended rate. The fresh weight (FW) and dry weight (DW) of the weeds were measured at 30 DAT, after the post-emergence herbicide treatment. Weed density and measurement of FW and DW of weed samples were taken from three randomly selected spots with the help of a quadrat (0.5 m2). After 30 days, all weeds were collected and classified into broadleaf and grass weeds. Different weed species (both broadleaf species and grasses) were counted, and the FW of each weed plant was measured. To measure the DW, the collected weeds were dried at 90°C in an oven for 24 h. Weed densities in both the herbicide-treated and control plots were recorded 30 days after planting of Miscanthus spp. in the field.
2.1 Density, FW, and DW of Weeds
The number of weed species that emerged in the M. sinensis and M. sacchariflorus fields was counted at 30 DAT using a 0.5 m2 quadrat. The counted weeds were harvested and placed in paper envelopes, and the FW was recorded immediately using an electronic balance. The weeds were dried at 90°C in an oven for 24 h, and the DW of the weeds was recorded.
2.2 Scoring for Herbicide Tolerance of M. sinensis and M. sacchariflorus
In the second set of experiments, fifty M. sinensis and fifty M. sacchariflorus were planted in a plot of size 100 m × 100 m and used for screening and assessing the phytotoxicity of herbicides. After the screening, herbicide-tolerant, partially tolerant, sensitive, and highly sensitive Miscanthus were separated and characterized to assess the effect of herbicides on morphological traits and biomass yield. The degree of damage by the treated herbicides was evaluated on a scale of 1 (No visible leaf injury, healthy tissues) to 10 (Damage to 100% of plants) (Table 2). Phytotoxicity of the sprayed herbicides on the M. sinensis and M. sacchariflorus was evaluated by observing symptoms such as chlorosis, epinasty, hyponasty, necrosis, stunting, wilting, death or no phytotoxicity at 30 days after treatment (DAT).
2.3 Effect of Pre- and Post-Emergence Herbicides on Morphological Traits and Biomass of M. sinensis and M. sacchariflorus
The effect of pre- and post-emergence herbicide treatments on the morphological traits of M. sinensis and M. sacchariflorus was measured and compared with the Miscanthus spp. grown as a control. Six random plants from each Miscanthus spp. were taken to measure plant height, DW, and FW. The plant height of all the treated and control plants was taken from the base of the plant to the tip of the main shoot at the time of maturity. To measure the DW, collected herbicide-treated Miscanthus shoots were dried at 90°C in an oven for 24 h.
2.4 Relative Chlorophyll Content
The relative chlorophyll content of the control and treated M. sinensis and M. sacchariflorus was measured using a chlorophyll meter SPAD-520 (Minolta Co., Ltd., Osaka, Japan). Data were collected from ten healthy leaves. Measurements were made on a sunny day (at 1 PM). Leaves were selected from five different plants from each Miscanthus plot.
The number of weeds, fresh weight and dry weight of weeds, height, visual injuries, fresh weight, and dry weight of Miscanthus species were collected during the vegetative season. Weeds were collected from the area of 1 m2 after the herbicide treatment, 30 days after the last herbicide treatment. They were then separated according to species and counted and weighed immediately. The collection of the above-ground Miscanthus was carried out in the month of November 2018 and 2019. Normal distribution and homogeneity of variances of the collected data were assessed. The average of two years (2018 and 2019) data were subjected to Two-way ANOVA analysis, and significant differences between the means were assessed using Duncan’s multiple range test at a significance level of P < 0.05 using SPSS version 20 (SPSS, 2011). All the linear correlation analysis was subsequently established to obtain a correlation coefficient between various parameters and the p-value for the significance of the correlation, using the EXCEL extension XLSTAT software (Version, 2021.2.2) at P < 0.05. Relationships within and between the parameters were determined by Pearson correlation analysis.
3.1 Effect of Herbicide Treatments on Density, FW, and DW of Weeds in the M. sinensis Growing Field
The efficacy of pre-emergence and post-emergence herbicides on the various weeds species was assessed visually at 30 DAT. The major weeds recorded in the M. sinensis and M. sacchariflorus fields were Digitaria ciliaris (Retz.) Koeler, Erigeron canadensis L., Chenopodium album L., Alopecurus aequalis Sobol., Calystegia sepium L., Ixeris dentate Thunb. Ex Thunb., Setaria viridis L., Oenothera biennis L., Capsella bursa-pastoris (L.) Medik., Trifolium repens L., Agropyron tsukushiense var. transiens (Hack.), Rumex crispus L., and Artemisia princeps Pamp. Different pre- and post-emergence herbicide treatments had significant influences on the FW and DW of weeds compared to the control plots. All the treated herbicides inhibited the growth, reduced the FW and DW, and the density of weed species. The density and FW of both broadleaf and grass weeds decreased more in the second year of weed management (2019).
Pre-emergence herbicides, including napropamide (21.8% of a.i.) and alachlor (43.7% of a.i.), displayed a higher rate of injuries to the foliar part of the broadleaf and grass weed species at the recommended dose, resulting in a reduced weed density, FW, and DW after 30 DAT with minimal negative effects on the growth of M. sinensis plants in the field after 30 DAT in both 2018 and 2019 (Table 3). Treatment of napropamide reduced the FW and DW of the emerged broadleaf weeds Chenopodium album var. centrorubrum (FW: 1.2 g, DW: 0.4 g), Oenothera biennis (FW: 2.0 g, DW: 1.00 g), and Plantago asiatica (FW: 16.5 g, DW: 2.0 g), and the grass weed Digitaria ciliaris (FW: 8.0 g, DW: 2.53 g). Similarly, the broadleaf weeds O. biennis, and Erigeron canadensis L. emerged in the alachlor (43.7% of a.i.) treated M. sinensis field (Table S1). Application of post-emergence herbicides such as Dicamba and glufosinate ammonium showed 100% control of grass weeds such as Setaria viridis, Digitaria ciliarie, Agropyron tsukushiense during both weed management years (2018 and 2019). Treatment with these herbicides completely inhibited the growth of the broadleaf weeds including Oenothera biennis, Chenopodium album A. princeps, A. tsukushiense, and B. frondosa (Tables S2–S4). However, the emergence of some grass weeds in the Miscanthus field indicated that a single application of these herbicides is not enough to restrict the growth of weeds due to insufficient injuries to the foliar part of them, and weeds may re-emerge later in the season and cause M. sinensis biomass reduction.
Data having the same letter in a column did not differ significantly according to Duncan’s multiple comparison test (P < 0.05).
3.2 Effect of Herbicides Treatments on Density, FW, and DW of Weeds in M. sacchariflorus Growing Field
Application of pre-emergence herbicides and post-emergence herbicides showed a wide range of effects on the growth, density, FW, and DW of weed species (Table 4). In the present study, pre-emergence herbicide treatment suppressed weeds but was not effective in controlling all weed species (Table 4) that emerged under field conditions. Both the pre-emergence herbicides that were tested showed great efficacy in controlling broadleaf and grass weeds and significantly reduced the density of weed species that emerged in the M. sacchariflorus field. Application of pre-emergence herbicides significantly reduced the FW, DW, and density of major broadleaf and grass weeds to various degrees. Treatment with pre-emergence herbicides reduced weed density, FW and DW of Trifolium repens L., Agropyron tsukushiense Hack., Rumex crispus L., Artemisia princeps Pamp., Taraxacum platycarpus H., and I. dentata to 100% (Table S5). Treatment of napropamide incompletely suppressed the growth of Oenothera biennis (FW: 6.5 g, DW: 2.2 g), Digitaria ciliaris (FW: 3.2, FW: 0.9 g), and C. album (FW: 4.0 g, DW: 1.3 g) in 2018 and this trend continued in 2019. Comparatively, alachlor was more effective in controlling both broadleaf and grass weeds in the M. sacchariflorus field when compared to napropamide at the recommended dose of treatment. Overall the efficacy of pre-emergence herbicides was higher in 2019 than in 2018 in M. sacchariflorus growing field (Tables S5–S9).
Application of post-emergence herbicides showed great efficacy in controlling broadleaf and grass weeds in the M. sacchariflorus field (Table 4). The majority of the broadleaf weeds that emerged in the M. sacchariflorus field were inhibited to 100% by the treatment of post-emergence herbicides. The highest FW and DW of weeds were recorded in the nicosulfuron, which was statistically superior to all the other treatments. Comparatively, treatment with dicamba and glufosinate ammonium reduced the majority of the weed species at the recommended dose. The efficacy of these herbicides for the inhibition of grass weeds and broadleaf weeds was higher in 2019 than in 2018 in M. sacchariflorus growing field. Most of the weed species showed leaf discoloration, followed by foliar injuries within a week of herbicide treatment. These weeds showed various degrees of tolerance and their biomass was affected to different extents. On average for the year 2019, the treatment of post emergence herbicides including bentazon, nicosulfuron, dicamba and glufosinate ammonium reduced the FW of weeds by 93.66%, 90.13%, 99.5%, 99.7%, respectively. Significant changes among the treatments were observed for the density (F = 54.11, P < 0.01), FW (F = 19.21, P < 0.01), and DW (F = 11.35, P < 0.002) of weeds for M. sinensis. Similar trends were observed for M. sacchariflorus (Table S5). Likewise, significant differences were observed in the interaction between years and treatments in density (F = 4.77, P < 0.001), FW (F = 6.52, P < 0.003), and DW (F = 3.22, P < 0.025) of weeds for the M. sinensis. Similar trends were observed for M. sacchariflorus (Table S5). This emphasizes that for proper control of weeds, proper application of pre-and post-emergence herbicides can effectively control them. Additional research is required to evaluate combinations with other herbicides, as well as sequential application of herbicides to identify those that cause higher levels of injury to the weeds and provide a sufficient level of weed control in the M. sinensis field, indicating that these herbicides might also be useful in the management of grass weeds in the Miscanthus field.
3.3 Effect of Herbicides Treatments on Miscanthus spp. Height
Treatment with pre- and post-emergence herbicides significantly affected the height of Miscanthus plants under field conditions (Fig. 2). In the present study, it was found that treatment with the herbicide glufosinate ammonium resulted in the lowest height of M. sinensis (70.00 ± 8.14 cm), which differed (not significantly) from the other treatments. This was followed by treatment with dicamba (105.00 ± 10.80 cm). Treatment with nicosulfuron resulted in taller plants (176.67 ± 12.47 cm); however, it was not statistically different from the height of M. sinensis in the control plots (170.00 cm). Similar results were also observed for M. sacchariflorus. A significant reduction in the M. sacchariflorus plant height was observed when treated with the pre- and post-emergence herbicides under field conditions (Fig. 2). Treatment of M. sacchariflorus plots with dicamba showed the lowest height (80.00 ± 8.05 cm), followed by glufosinate treatment (90.00 ± 8.16 cm). Treatment with nicosulfuron resulted in taller plants (183.33 ± 9.43 cm); however, it was not statistically different from the height of M. sacchariflorus in the control plots (180.00 ± 8.17 cm).
Figure 2: Effect of pre-emergence and post-emergence herbicides on the plant height of M. sinensis and M. sacchariflorus. Data are means ± standard deviation (n = 3). Means with different small letters indicate significant differences at P < 0.05. NS, Nicosulfuron; DI, Dicamba; BTZ, Bentazon; GLA, Glufosinate ammonium; ALA, Alachlor; NAP, Napropamide
3.4 Assessment of Visual Injury and Biomass Production of M. sinensis and M. sacchariflorus Resulted by Herbicides Treatment
Herbicidal toxicity was assessed after treating the two Miscanthus species with pre- and post-emergence herbicides. Significant variation in the degree of tolerance to the treated herbicides was observed in the Miscanthus species (Fig. 3, Supplementary Figs. 1 and 2). Comparatively, M. sinensis showed slightly higher tolerance to the herbicides nicosulfuron (10%), bentazon (10%), and napropamide (10%) at the recommended dose than M. sacchariflorus (Fig. 3). As a result, the FW and DW of M. sinensis were higher in the pre- and post-emergence herbicide application than in unweeded control plots in 2019 (Table 5). The DW of M. sinensis treated with nicosulfuron, bentazon and napropamide were lower than the unweeded control plots in 2018 (Table 5). Similarly, except in the case of napropamide, the FW and DW of M. sacchariflorus were significantly reduced by the Pre- emergence and Post emergence herbicides treatment in 2018 (Table 5). Interestingly, with some exception, the treatment of herbicides increased the biomass of M. sacchariflorus in the second year (2019) of weed management (Table 5), demonstrating that the herbicides are effective in improving the biomass yield of M. sacchariflorus. Among these herbicides, napropamide treatment showed a greater increase in the FW and DW (4900.0 and 2700.3 g, respectively), compared to the FW and DW of control M. sinensis (1800.0 and 158.0 g, respectively). The results showed that the application of dicamba and glufosinate ammonium caused severe injuries to the foliar region, chlorosis, and stunt growth on both species of Miscanthus plants and resulted in a significant loss in biomass at its recommended dose during its initial year of weed management. It is interesting to note that the degree of visual injuries caused by the application of glufosinate ammonium was similar (90%) for both M. sinensis and M. sacchariflorus plants (Fig. 3), which may be due to its higher phytotoxic effects on the growing plant in 2018. Both species were susceptible to dicamba (visual injury of 80% and 90%, respectively), resulting in a significant reduction in FW (467.7 and 328.7 g, respectively) compared to the control plants in the first year (2018) of weed management (Table 5). Such damage in the biomass appeared temporal as there was significant increase in the FW and DW of both M. sinensis and M. sacchariflorus in the second year (2019), which may be due to the effective controls of weeds and the lower competition with weeds for light, moisture, space and nutrients at the critical stages of growth (Table S10). This in turn favoured Miscanthus to utilize available resources for growth and gaining biomass, demonstrating that herbicides treatments are effective in reducing the biomass loss of M. sinensis and M. sacchariflorus plants. Significant changes were recorded to FW (F = 2.66, P < 0.05), DW (F = 4.82, P < 0.007) among the years for M. sinensis. Similar trend were recorded in FW (F = 31.78, P < 0.05), and DW (F = 11.10, P < 0.02) among the years for M. sacchariflorus. Likewise, significant differences were observed in the interaction between years and treatments for M. sinensis FW (F = 1.74, P < 0.05) and DW (F = 4.89, P < 0.006). Similar trend were recorded in FW of M. sacchariflorus (F = 6.19, P < 0.02) and DW (F = 3.46, P < 0.03) (Table S10). Furthermore, the present results support the hypothesis that sequences of pre-emergence and post-emergence herbicides will be necessary to achieve maximum control of weeds and increase the biomass of M. sinensis and M. sacchariflorus.
Figure 3: Visual damage of pre-emergence and post-emergence herbicides on M. sinensis and M. sacchariflorus. NS, Nicosulfuron; DI, Dicamba; BTZ, Bentazon; GLA, Glufosinate ammonium; ALA, Alachlor; NAP, Napropamide
3.5 Total Chlorophyll Content (SPAD Value)
The total chlorophyll (TC) content in M. sinensis and M. sacchariflorus leaves under the different herbicide treatments is shown in Fig. 4. In the present study, there was a significant difference in the chlorophyll content between herbicide-treated and control plants. At 30 days after application of pre- and post-emergence herbicides to the M. sinensis, higher TC content was observed for the herbicide alachlor (38.35 ± 2.37 SPAD unit), followed by the treatment with Napropamide (35.03 ± 3.01 SPAD unit) and nicosulfuron (34.80 ± 2.51 SPAD unit). A significant reduction in TC was observed in the plants treated with dicamba (20.30 ± 4.21 SPAD unit) and glufosinate ammonium (20.90 ± 4.23 SPAD unit), with no significant difference between them. Similar to M. sinensis, the chlorophyll content of M. sacchariflorus was affected by treatment with different herbicides. The TC content of M. sacchariflorus, when exposed to napropamide, was similar to that of M. sacchariflorus grown in the control plots. Chlorophyll content was higher in M. sacchariflorus treated with napropamide and nicosulfuron (43.15 ± 2.86 and 41.53 ± 3.69 SPAD units, respectively). The chlorophyll content of M. sacchariflorus was significantly affected by the treatment with glufosinate ammonium, dicamba, and alachlor. However, this value was significantly lower from the chlorophyll content of M. sacchariflorus in the control plots. In contrast, the highest reduction in chlorophyll content was observed in M. sacchariflorus treated with glufosinate ammonium followed by dicamba (22.45 ± 3.84 and 28.55 ± 3.95 SPAD units, respectively).
Figure 4: Effect of pre-emergence and post-emergence herbicides on the total chlorophyll content of M. sinensis and M. sacchariflorus. Data are means ± standard deviation (n = 3). Mean with different lowercase letters indicate a significant difference at P < 0.05. NS, Nicosulfuron; DI, Dicamba; BTZ, Bentazon; GLA, Glufosinate ammonium; ALA, Alachlor; NAP, Napropamide
3.6 Pearson’s Correlations among Selected Variables
The Pearson’s correlation analysis shows a wide range of correlations between the different parameters (Table S11). The weed density had a significant negative correlation with the fresh weight (r = −0.595, P < 0.05) and dry matters (r = −0.425, P < 0.05) of M. sinensis (Figure), implying that the fresh weight of the M. sinensis increased with a proportional decrease in the weed density and its biomass in the field. A similar relationship was also observed between the fresh weight of M. sinensis with the fresh weight (r = −0.612, P < 0.05) and dry weight (r = −0.604, P < 0.05) of weeds. Another attribute such as plant height was positively correlated with the total chlorophyll content (r = 0.773, P < 0.05) and fresh weight of M. sinensis (r = 0.327, P < 0.05). These results indicate that the decrease in the weed density using per-emergence and post-emergence herbicides significantly impact the plant growth and biomass of M. sinensis. The Pearson’s correlation analysis shows that the density of weeds was significantly and negatively correlated to the fresh weight of M. sacchariflorus (r = −0.227, P < 0.05), and dry weight of it (r = −0.220, P < 0.05). A similar trend was observed between fresh weight of M. sacchariflorus with the fresh weight and dry weight of weeds, indicating a significant impact on the plant growth and biomass of M. sacchariflorus (Table S12). In contrast, plant height and chlorophyll content of the M. sacchariflorus showed a positive correlation with its biomass, indicating a direct impact of weed competition on the reduction in M. sacchariflorus growth rate and total biomass.
Proper control of weeds in bioenergy crop fields is necessary as the infestation of weeds causes competition for available nutrients, water, light, and space, which may cause the inhibition of the growth of bioenergy crops, reducing their biomass [49,50]. These challenges can be addressed by the appropriate application of registered herbicides for weed control [51]. In this study, Miscanthus grown in the control plots without weeding resulted in poor growth and less biomass production compared to weed management by herbicides treatments. This is because the application of herbicides reduced the density of broadleaf and grass weeds in the field, which reduced competition and caused the surplus flow of nutrients to the growing Miscanthus plants. In the present study, comparatively, the biomass of both the M. sinensis and M. sacchariflorus was reduced by the treated herbicides during the first year of weed management (2018), and then in the second year (2019). These results corroborate the report of Everman et al. [52], who observed an increase in the biomass of M. giganteus after the treatment of herbicides in field experiments. In the present study, treatment with pre-emergence herbicides showed a significant variation in the impact on weeds and achieved good control over the broadleaf and grass weeds.
In this study, treatment with the pre-emergence herbicides showed that recommended dose of alachlor when compared to napropamide was more effective in controlling both broadleaf and grass weed species that grew in the M. sinensis and M. sacchariflorus fields. A significant decrease in weed density, FW, and DW was observed in the plot treated with alachlor. Higher efficiency of weed growth control was also reported for other crops treated with alachlor [53]. It has been reported that alachlor causes a reduction in plant growth by inhibiting cell division and cell enlargement [49]. Furthermore, Hemanth Kumar et al. [49] observed nuclear lesions and chromosomal abnormalities in alachlor-treated weeds. Moreover, they observed that the treatment of alachlor caused the inhibition of the activity of elongase and geranylgeranyl pyrophosphate (GGPP) enzymes and inhibited cell division, leading to the ultimate death of emerging weeds. In this study, treatment with post-emergence herbicides effectively reduced the density, FW, and DW of dominant broadleaf and grass weed species grown in the M. sinensis and M. sacchariflorus fields. The efficacy of these herbicides for the inhibition of the broadleaf and grass weeds was higher in 2019 than in 2018 in Miscanthus growing field.
The treatment of Pre-emergence herbicides, partially controls the density, FW, and DW of D. ciliaris, C. album L., and O. biennis L. whereas the treatment of post-emergence herbicides such as Bentazon, dicamba, Glufosinate ammonium completely suppressed these weeds species in Miscanthus field. According to Somerville et al. [54], a shorter length of soil residual activity of pre-emergence herbicides does not provide sufficient weed control when used alone. Moreover, others argued that due to the continuous use of different chemical herbicides in the cultivation land, many weeds have evolved resistance against different herbicides and its mode of action [55]. Other than chemical herbicides, genetic factors, biological characteristics of weed species, agronomic practices and characteristics of herbicides also play important role the evalution of and spread of herbicides resistance in weeds species [55]. In the present study, presence of D. ciliaris, C. album L., and O. biennis L. in the pre-emergence herbicides treated Miscanthus field could be due to insufficient weed control, rather than herbicides resistance of weeds. Others believed that weeds escapes are due to their ability to germinate for a long time during the growing season [56], indicating that the timing of herbicides applications is the key to its efficacy. Based on the present study, the combination of both pre-and post-emergence herbicides is necessary to obtain a good efficacy in total control of weeds in Miscanthus fields.
Among the four post-emergence herbicides, treatment with nicosulfuron inhibited the growth of most of the dominant weeds without affecting the biomass of the growing Miscanthus plants, indicating that the application of nicosulfuron, in particular, is necessary to control the late emergence or escape weeds. Moreover, in a similar study, nicosulfuron has been reported to control weed species without adversely affecting the growth of bioenergy crops [57–59]. In the present study, treatment with nicosulfuron partially inhibited the growth and density of grass weeds D. ciliaris (Retz.) and S. viridis L., which is in line with an earlier report by Zhang et al. [60], who observed nicosulfuron tolerance in D. sanguinalis. It has been reported that nicosulfuron herbicides are responsible for inhibiting acetolactate synthase (ALS), which is located in the photosynthetic tissues (chloroplast and plastids) of plants and is more susceptible in young foliar tissues [61]. In contrast, the application of dicamba and glufosinate ammonium resulted in severe visual injuries and caused various degrees of necrosis, stunting of growth, leaf distortion, twisting of leaves, and reduction in the FW and DW of the weeds in both M. sinensis and M. sacchariflorus fields. In the present study, broadleaf weeds P. asiatica L. and E. canadensis appeared in the glufosinate ammonium-treated Miscanthus plot. In contrast, the treatment with glufosinate ammonium completely inhibited the growth and density of grass weeds. The lower susceptibility of grass weeds to glufosinate ammonium was attributed to the presence of multiple meristematic zones in a single plant, which makes grasses generally possess a higher tendency to survive than broadleaf plants when exposed to glufosinate ammonium [62].
Recently, the application of herbicides has increased in weed management programs due to the scarcity of labour and the higher efficiency of herbicides in controlling their growth. Several studies have reported an increase in the FW of newly established bioenergy crop fields. However, herbicides may degrade the growth of bioenergy crops and cause severe losses in biomass yield. Therefore, it is important to assess the phytotoxicity of herbicides on bioenergy crops. Application of pre- and post-emergence herbicides at the recommended dose caused various degrees of visual injury in the two species of Miscanthus. Treatment with the recommended dose of bentazon resulted in lower phytotoxicity to the growing M. sinensis. However, treatment with bentazon at the same dose caused higher visual injury to the M. sacchariflorus plants. Application of glufosinate ammonium on plants either damaged plant tissues or killed them completely due to disruption in the biochemical and physiological processes of the cells. Both M. sinensis and M. sacchariflorus were susceptible to dicamba and glufosinate ammonium, resulting in a significant reduction in FW and DW compared to the control plants. In the present study, the phytotoxicity of dicamba and glufosinate ammonium was characterized by severe leaf chlorosis in the midrib region. Eventually, the plant showed rapid recovery after 30 DAT. Chlorophyll content of M. sinensis and M. sacchariflorus was significantly reduced by treatment with glufosinate ammonium, bentazon, dicamba, and alachlor compared to the chlorophyll content of plants in the control plot.
In this study, the application of dicamba and glufosinate ammonium in the Miscanthus field showed higher efficacy in reducing the weed density, FW, and DW of both broadleaf and grass weeds. However, application of these herbicides resulted in severe intoxication in both species of Miscanthus, leading to stunting of the plant and necrosis in the foliar part of the plants, which contributed to the reduced FW and DW. Glufosinate ammonium targets glutamine synthetase (the second most abundant protein in plant leaves [63–66] and is responsible for the production of the amino acid glutamine and ammonia detoxification [52]). Inhibition of glutamine synthetase causes decreased glutamine production and rapid accumulation of ammonia in plant tissues [63,67,68] and toxic accumulation of glyoxylate, which inhibits RuBP-carboxylate and carbon dioxide fixation [69]. It has been further observed that an interruption of photorespiration results from a deficiency of intermediates of the Calvin cycle [70,71]. Accumulation of higher levels of ammonia causes acute toxicity to plants, causing severe chlorosis of leaves, suppression of growth, and eventually plant death [72]. According to Takano and Dayan [73], glufosinate ammonium disrupts both photorespiration and the light reactions of photosynthesis, which causes photoreduction of molecular oxygen, which generates reactive oxygen species. Moreover, it can also inhibit carbon assimilation and plant growth [63,74]. In the present study, the application of dicamba and glufosinate ammonium not only suppressed the dominant broadleaf and grass weeds but also reduced the growth of Miscanthus plants. Therefore, before applying these herbicides, all of these factors should be considered. In the present study, M. sinensis showed higher tolerance to nicosulfuron and napropamide. In particular, treatment with nicosulfuron resulted in higher FW and DW of Miscanthus. Moreover, Miscanthus treatment with nicosulfuron caused higher chlorophyll content in both species of Miscanthus, which could contribute to the increased biomass in the plant. According to Barroso et al. [75], some plant species can absorb nicosulfuron in non-toxic compounds and can reduce phytotoxicity. Moreover, in the present study, M. sinensis was less susceptible to bentazon. However, treatment with bentazon resulted in reduced FW and DW, which is possibly due to the insufficient injuries to the broadleaf weeds such as Erigeron canadensis, Artemisia princeps, and Plantago asiatica L., which causes the re-growth of weeds in the M. sinensis field and competition for space, light, water, and nutrition. In the present study, treatment with bentazon resulted in lower chlorophyll content, higher visual injuries, and reduced FW and DW of Miscanthus species. Bentazon, a photosystem II (PSII) electron flow inhibitor, causes changes in the chloroplast ultrastructure, photosynthetic pigment ratios, and levels of chlorophyll proteins in the photosynthetic apparatus of plants [76]. Therefore, in the present study, it is possible that the reduction in photosynthetic pigments could reduce the FW and DW of Miscanthus species. In similar research, Hassannejad et al. [77] observed a lower photosynthetic performance index (PIABS) in Xanthium strumarium L. when treated with bentazon as compared to nicosulfuron.
In the present study, none of the herbicides alone treatments were found effective in controlling all the types of weeds. It was observed that the application of pre-emergence herbicides such as alachlor and napropamide at 951 and 1120 g.a.i.ha−1, respectively, caused less visual injuries and biomass loss in M. sinensis and M. sacchariflorus, while the effect was inadequate to control invasive weeds such as biennis L., D. ciliaris (Retz.) and O. biennis L. The highest weed control was achieved by the treatment of glufosinate ammonium and dicamba at 400 and 482 400 g.a.i/ha−1, respectively, which also showed severe phytotoxicity with a considerable amount of loss in the Miscanthus biomass, but these were often temporary. Treatment with nicosulfuron (40 g.a.i/ha−1) showed good control of the broadleaf and grass weeds at the recommended dose with lower phytotoxicity and caused least visual injuries to the M. sinensis and M. sacchariflorus. The present study can be relevant for weed management in Miscanthus fields with multiple pre- and post-emergence herbicide options.
Acknowledgement: This work was supported by funding from the KU Research Professor Program.
Funding Statement: This work was supported by a BrainKorea21 Plus (BK21+, Grant No. 22A20153813519, Team: Omics Research of Crop Bioresources for Future, Konkuk University), the National Research Foundation of Korea, Republic of Korea.
Author Contributions: Bimal Kumar Ghimire contributed by doing the experiment and writing the manuscript; Chang Yeon Yu supervised the experiment; Seung Hyun Kim and Ill Min Chung contributed by analyzing data and editing the revised manuscript. All authors have read and agreed to the published version of the manuscript.
Conflicts of Interest:: The authors declare that they have no conflicts of interest to report regarding the present study.
References
1. Clifton-Brown, J. C., Lewandowski, I. (2000). Overwintering problems of newly established Miscanthus plantations can be overcome by identifying genotypes with improved rhizome cold tolerance. New Phytologist, 148(2), 287–294. [Google Scholar]
2. Atkinson, C. (2009). Establishing perennial grass energy crops in the UK: A review of current propagation options for Miscanthus. Biomass Bioenergy, 33(5), 752–759. [Google Scholar]
3. Sacks, E. J., Juvik, J. A., Lin, Q., Stewart, J. R., Yamada, T. (2013). The gene pool of Miscanthus species and its improvement. In: Paterson, A. H. (Ed.Genomics of the saccharinae, pp. 73–101. New York, NY, USA: Springer. [Google Scholar]
4. Clark, L. V., Jin, X., Petersen, K. K., Anzoua, K. G., Bagmet, L. et al. (2018). Population structure of Miscanthus sacchariflorus reveals two major polyploidization events, tetraploid-mediated unidirectional introgression from diploid M. sinensis, and diversity centered around the yellow sea. Annals of Botany, 20, 1–18. [Google Scholar]
5. Dwiyanti, M. S., Stewart, J. R., Yamada, T. (2013). Germplasm resources of Miscanthus and their application in breeding. In: Saha, M. C., Bhandari, H. S., Bouton, J. H. (Eds.Bioenergy feedstocks: Breeding and genetics, pp. 49–66. Oxford, UK: John Wiley & Sons. [Google Scholar]
6. Visser, P., Pignatelli, V., Jørgensen, U., Oliveira, J. F. S. (2001). Utilisation of Miscanthus. In: Jones, M. B., Walsh, M. (Eds.Miscanthus–For energy and fibre, pp. 109–154. London, UK: James & James. [Google Scholar]
7. Liao, C. B., Deng, Y. H., Wang, X. Z., Fan, X. L., Yu, T. et al. (2013). Manufacture and mechanical properties of biocomposite made of reed and silver grass. Applied Mechanics and Materials, 248, 237–242. [Google Scholar]
8. Trinklein, D. (2006). Ornamental grasses. In: Plants of wisconsin. Columbia, MO, USA: Robert W. Freckmann Herbarium at University of Wisconsin-Stevens Point: Stevens Point, WI, USA; University of Missouri-Extension USA; University of Missouri-Extension. [Google Scholar]
9. Sacks, E. J., Juvik, J. A., Lin, Q., Stewart, J. R., Yamada, T. (2012). The gene pool of Miscanthus species and its improvement. In: Paterson, A. H. (Ed.Genomics of the saccharinae, pp. 73–101. New York: Springer. [Google Scholar]
10. Pohl, R. W. (1959). Introduced weedy grasses in Iowa. Proceedings of the Iowa Academy of Science, 66(1), 160–162. [Google Scholar]
11. Clifton-Brown, J. C., Lewandowski, I., Andersson, B., Basch, G., Christian, D. G. et al. (2001). Performance of 15 Miscanthus genotypes at five sites in Europe. Agronomy Journal, 93(5), 1013–1019. [Google Scholar]
12. Lewandowski, I., Clifton-Brown, J. C., Scurlock, J. M. O., Huisman, W. (2000). Miscanthus: European experience with a novel energy crop. Biomass Bioenergy, 19(4), 209–227. [Google Scholar]
13. Lewandowski, I., Clifton-Brown, J. C., Andersson, B., Basch, G., Christian, D. et al. (2003). Environment and harvest time affect the combustion qualities of Miscanthus genotypes. Agronomy Journal, 95(5), 1274–1280. [Google Scholar]
14. Clifton-Brown, J. C., Chiang, Y. C., Hodkinson, T. R. (2008). Miscanthus: Genetic resources and breeding potential to enhance bioenergy production. In: Vermerris, W. (Ed.Genetic improvement of bioenergy crops, pp. 273–294. New York, NY, USA: Springer Science. [Google Scholar]
15. Stewart, J. R., Toma, Y., Fernandez, F. G., Nishiwaki, A., Yamada, T. et al. (2009). The ecology and agronomy of Miscanthus sinensis, a species important to bioenergy crop development, in its native range in Japan: A review. GCB-Bioenergy, 1(2), 126–153. [Google Scholar]
16. Meyer, M. H., Paul, J., Anderson, N. O. (2010). Competive ability of invasive Miscanthus biotypes with aggressive switchgrass. Biological Invasions, 12(11), 3809–3816. [Google Scholar]
17. Jorgensen, U., Muhs, H. J. (2001). Miscanthus breeding and improvement. In: Jones, M. B., Walsh, M. (Eds.Miscanthus for energy and fibre, pp. 68–85. London: James & James. [Google Scholar]
18. Numata, M. (1975). Ecological studies in Japanese grasslands with special reference to the IBP area: Productivity of terrestrial communities. In: JIBP synthesis, vol. 13. Tokyo: University of Tokyo Press. [Google Scholar]
19. Numata, M., Mitsudera, M. (1969). Efficient environmental factors to the growth and production of the Miscanthus sinensis grasslands in Japan: Ecological judgement of grassland condition and trend V. Japanese Journal of Botany, 20, 135–151. [Google Scholar]
20. Hodgson, E. M., Lister, S. J., Bridgwater, A. V., Clifton-Brown, J., Donnison, I. S. (2010). Genotypic and environmentally derived variation in the cell wall composition of Miscanthus in relation to its use as a biomass feedstock. Biomass and Bioenergy, 34(5), 652–660. [Google Scholar]
21. Anzoua, G. K., Suzuki, K., Fujita, S., Toma, Y., Yamada, T. (2015). Evaluation of morphological traits, winter survival, and biomass potential in wild Japanese Miscanthus sinensis Anderss. populations in Northern Japan. Grassland Science, 61(2), 83–91. [Google Scholar]
22. Rayburn, A. L., Crawford, J., Rayburn, C. M., Juvik, J. A. (2009). Genome size of three Miscanthus species. Plant Molecular Biology Reporter, 27(2), 184–188. [Google Scholar]
23. Jiang, J., Zhu, M., Ai, X., Xiao, L., Deng, G. et al. (2013). Molecular evidence for a natural diploid hybrid between Miscanthus sinensis (Poaceae) and M. sacchariflorus. Plant Systematics and Evolution, 299(7), 1367–1377. [Google Scholar]
24. Li, X., Hu, D., Luo, M., Zhu, M., Li, X. et al. (2013). Nuclear DNA content variation of three Miscanthus species in China. Genes and Genomics, 35(1), 13–20. [Google Scholar]
25. Chae, W. B., Hong, S. J., Gifford, J. M., Rayburn, A. L., Sacks, E. J. et al. (2014). Plant morphology, genome size, and SSR markers differentiate five distinct taxonomic groups among accessions in the genus Miscanthus. GCB-Bioenergy, 6(6), 646–660. [Google Scholar]
26. Moon, Y. H., Cha, Y. L., Choi, Y. H., Yoon, Y. M., Koo, B. C. et al. (2013). Diversity in ploidy levels and nuclear DNA amounts in Korean Miscanthus species. Euphytica, 193(3), 317–326. [Google Scholar]
27. Feng, X. P., Lourgant, K., Castric, V., Saumitou-Laprade, P., Zheng, B. S. et al. (2014). The discovery of natural Miscanthus accessions related to Miscanthus × giganteus using chloroplast DNA. Crop Science, 54(4), 1645–1655. [Google Scholar]
28. Hirayoshi, I., Nishikawa, K., Kubono, M., Murase, T. (1957). Cytogenetical studies on forage plants (VI) on the chromosome number of Ogi (Miscanthus sacchariflorus). Research Bulletin of the Faculty of Agriculture, Gifu University, 8, 8–13. [Google Scholar]
29. Jensen, E., Farrar, K., Thomas-Jones, S., Hastings, A., Donnison, I. et al. (2011a). Characterization of flowering time diversity in Miscanthus species. GCB-Bioenergy, 3(5), 387–400. [Google Scholar]
30. Yan, J., Chen, W., Fan, L., Ma, H. Z., Meng, A. P. et al. (2011). Variability and adaptability of Miscanthus species evaluated for energy crop domestication. GCB-Bioenergy, 4(1), 49–60. [Google Scholar]
31. Stéphanie, A., Maryse, B. H. (2015). A review on Miscanthus biomass production and composition for bioenergy use: Genotypic and environmental variability and implications for breeding. BioEnergy Research, 8(2), 502–526. [Google Scholar]
32. Acheampong, M., Ertem, F. C., Kappler, B., Neubauer, P. (2017). In pursuit of Sustainable Development Goal (SDG) number 7: Will biofuels be reliable? Renewable and Sustainable Energy Reviews, 75(7), 927–937. [Google Scholar]
33. IEA (2011). Technology roadmap: Biofuels for transport. Paris, France: OECD/IEA. [Google Scholar]
34. IEA (2012). Technology roadmap: Bioenergy for heat and power. Paris, France: OECD/IEA. [Google Scholar]
35. Verdade, L. M., Pina, C. I., Rosalino, L. M. (2015). Biofuels and biodiversity: Challenges and opportunities. Environmental Development, 15, 64–78. [Google Scholar]
36. Song, J. S., Lim, S. H., Lim, Y., Nah, G., Lee, D. K. et al. (2016). Herbicide-based weed management in Miscanthus sacchariflorus. BioEnergy Research, 9(1), 326–334. [Google Scholar]
37. Koncekova, L., Feher, A., Halmova, D. (2014). Ecological and socio-economic evaluation of weed vegetation in stands of energy grass Miscanthus × giganteus. Acta Universitatis Agriculturae et Silviculturae Mendelianae Brunensis, 62(5), 985–990. [Google Scholar]
38. Winkler, B., Mangold, A., von Cossel, M., Iqbal, Y., Kiesel, A. et al. (2020). Implementing Miscanthus into sustainable farming systems: A review on agronomic practices, capital and labor demand. Renewable and Sustainable Energy Reviews, 132, 110053. [Google Scholar]
39. Borkowska, H., Molas, R. (2010). Weeds density of perennial energy crop species as dependent on plantation maturity. Acta Agrophysica, 15(1), 13–21. [Google Scholar]
40. Everman, W. J., Lindsey, A. J., Henry, G. M. (2011). Response of Miscanthus × giganteus and Miscanthus sinensis to postemergence herbicides. Weed Technology, 25(3), 398–403. [Google Scholar]
41. Maksimovic, J., Pivic, R., Stanojkovic-Sebic, A., Vucic-Kisgeci, M., Kresovic, B. et al. (2016). Planting density impact on weed infestation and the yield of Miscanthus grown on two soil types. Plant, Soil and Environment, 62(8), 384–388. [Google Scholar]
42. Boydston, R. A., Collins, H. P., Fransen, S. C. (2010). Response of three switchgrass (Panicum virgatum) cultivars to mesotrione, quinclorac, and pendimethalin. Weed Technology, 24(3), 336–341. [Google Scholar]
43. Curran, W. S., Ryan, M. R., Myers, M. W., Adler, P. R. (2012). Effect of seeding date and weed control on switchgrass establishment. Weed Technology, 26(2), 248–255. [Google Scholar]
44. Smith, L. L., Askew, S. D., Hagood Jr, E. S., Barney, J. N. (2015). Screening pre-emergence and post-emergence herbicides for safety in bioenergy crops. Weed Technology, 29(1), 135–146. [Google Scholar]
45. Haines, S. A., Gehl, R. J., Havlin, J. L., Ranney, T. G. (2014). Nitrogen and phosphorus fertilizer effects on establishment of giant Miscanthus. BioEnergy Research, 8(1), 17–27. [Google Scholar]
46. Ma, X. F., Jensen, E., Alexandrov, N., Troukhan, M., Zhang, L. et al. (2012). High resolution genetic mapping by genome sequencing reveals genome duplication and tetraploid genetic structure of the diploid Miscanthus sinensis. PLoS One, 7(3), e33821. [Google Scholar] [PubMed]
47. Swaminathan, K., Alabady, M. S., Varala, K., de Paoli, E., Ho, I. et al. (2010). Genomic and small RNA sequencing of Miscanthus × giganteus shows the utility of sorghum as a reference genome sequence for Andropogoneae grasses. Genome Biology, 11(2), R12. [Google Scholar] [PubMed]
48. Kim, S. C., Hong, Y. K., Oh, S. J., Oh, S. M., Lee, S. P. et al. (2017). Effect of chemical amendments on remediation of potentially toxic trace elements (PTEs) and soil quality improvement in paddy fields. Environmental Geochemistry and Health, 39(2), 345–352. [Google Scholar] [PubMed]
49. Hemanth Kumar, N. K., Jagannath, S. (2015a). Herbicide induced alterations in physiological responses to the maize (Zea mays L.) seedlings. World Research Journal of Agricultural Biotechnology, 4(1), 53–59. [Google Scholar]
50. Hemanth Kumar, N. K., Jagannath, S. (2015b). Cytological effects of herbicide butachlor 50 EC on somatic cells of Triticum aestivum L. Journal of Applied Biology & Biotechnology, 3(2), 30–34. [Google Scholar]
51. Knezevic, S. Z., Pavlovic, P., Osipitan, O. A., Barnes, E. R., Beiermann, C. et al. (2019). Critical time for weed removal in glyphosate-resistant soybean as influenced by pre-emergence herbicides. Weed Technology, 33, 393–399. [Google Scholar]
52. Everman, W. J., Thomas, W. E., Burton, J. D., York, A. C., Wilcut, J. W. (2009). Absorption, translocation, and metabolism of glufosinate in transgenic and nontransgenic cotton, Palmer amaranth (Amaranthus palmeriand pitted morning glory (Ipomoea lacunosa). Weed Science, 57(4), 357–361. [Google Scholar]
53. Pandey, A. K., Joshi, O. P., Billore, S. D. (2007). Effect of herbicidal weed control on weed dynamics and yield of soybean [Glycine max (L.) Merrill]. Soybean Research, 5, 26–32. [Google Scholar]
54. Somerville, G. J., Powles, S. B., Walsh, M. J., Renton, M. (2017). Why was resistance to shorter-acting pre-emergence herbicides slower to evolve? Pest Management Science, 73(5), 844–851. [Google Scholar] [PubMed]
55. Nakka, S., Godar, A. S., Wani, P. S., Thompson, C. R., Peterson, D. E. et al. (2017). Physiological and molecular characterization of hydroxyphenylpyruvate dioxygenase (HPPD)-inhibitor resistance in Palmer Amaranth (Amaranthus palmeri S.Wats.). Frontier in Plant Science, 8, 555. [Google Scholar] [PubMed]
56. Hennen, S., Scursoni, J., Forcella, F. (2002). Delayed weed emergence and escape from control in glyphosate-tolerant soybean. North Central Weed Science Society Abstracts, 57, 126. [Google Scholar]
57. Chikoye, D., Udensi, U. E., Lum, A. F., Ekeleme, F. (2007). Rimsulfuron for postemergence weed control in corn in humid tropical environments of Nigeria. Weed Technology, 21(4), 977–981. [Google Scholar]
58. Lum, A. F., Chikoye, D., Adesiyan, S. O. (2005b). Control of Imperata cylindrica (L.) Raeuschel (speargrass) with nicosulfuron and its effects on the growth, grain yield, and food components of maize. Crop Protection, 24(1), 41–47. [Google Scholar]
59. Lum, A. F., Chikoye, D., Adesiyan, S. O. (2005a). Effect of nicosulfuron dosages and timing on the postemergence control of cogongrass (Imperata cylindrica) in corn. Weed Technology, 19(1), 122–127. [Google Scholar]
60. Zhang, H., Wang, G., Liu, X., Bian, Q. (2013). Resistance of Digitaria sanguinalis to nicosulfuron. Weed Science, 1, 34–36 (in Chinese). [Google Scholar]
61. Roman, E. S., Beckie, H., Vargas, L., Hall, L., Rizzardi, M. A. et al. (2007). Como funciona os herbicidas : da biologia à aplicac, ã o. Passo Fundo : Gráfica Editora Berthier. [Google Scholar]
62. Takano, H. K., Beffa, R., Preston, C., Westra, P., Dayan, F. E. (2019). Reactive oxygen species trigger the fast action of glufosinate. Planta, 249(6), 1837–1849. [Google Scholar] [PubMed]
63. Coetzer, E., Al-Khatib, K. (2001). Photosynthetic inhibition and ammonium accumulation in Palmer amaranth after glufosinate application. Weed Science, 49, 454–459. [Google Scholar]
64. Fraser, A. R., Ridley, S. M. (1984). Kinetics for glutamine-synthetase inhibition by phosphinothricin and measurement of other enzyme activities in situ in isolated asparagus cells using a freeze-thaw technique. Planta, 161(5), 470–474. https://doi.org/10.1007/BF00394580 [Google Scholar] [PubMed] [CrossRef]
65. Kocher, H. (1989). Inhibitors of glutamine synthetase and their effects in plants. British Crop Protection Council, 42, 173–181. [Google Scholar]
66. Leason, M., Cunliffe, D., Parkin, D., Lea, P. J., Miflin, J. (1982). Inhibition of pea leaf glutamine synthetase by sulphoximine, phosphinothricin and other glutamate analogues. Phytochemistry, 21(4), 855–857. https://doi.org/10.1016/0031-9422(82)80079-4 [Google Scholar] [CrossRef]
67. Lea, P. J., Joy, K. W., Ramos, J. L., Guerrero, M. G. (1984). The action of 2-amino-4-(methylphosphinyl)-butanoic acid (phosphinothricin) and its 2-oxo-derivative on the metabolism of cyanobacteria and higher plants. Phytochemistry, 23(1), 1–6. https://doi.org/10.1016/0031-9422(84)83066-6 [Google Scholar] [CrossRef]
68. Wild, A., Manderscheid, A. (1984). The effect of phosphinothricin on the assimilation of ammonia in plants. Zeitschrift für Naturforschung C, 39C(5), 500–504. https://doi.org/10.1515/znc-1984-0539 [Google Scholar] [CrossRef]
69. Pline, W. A., Wu, J., Hatzios, K. K. (1999). Absorption, translocation, and metabolism of glufosinate in five weed species as influenced by ammonium sulfate and pelargonic acid. Weed Science, 47(6), 636–643. https://doi.org/10.1017/S0043174500091268 [Google Scholar] [CrossRef]
70. Lacuesta, M., Munoz-Rueda, A., Gonzalez-Murua, C., Sivak, M. N. (1992). Effect of phosphinothricin (glufosinate) on photosynthesis and chlorophyll fluorescence by barley leaves illuminated under photorespiratory and non-photorespiratory conditions. Journal of Experimental Botany, 43(2), 159–165. https://doi.org/10.1093/jxb/43.2.159 [Google Scholar] [CrossRef]
71. Sauer, H., Wild, A., Ruhle, W. (1987). The effect of phosphinothricin (glufosinate) on photosynthesis. II. The causes of inhibition of photosynthesis. Zeitschrift für Naturforschung C, 42(3), 270–278. https://doi.org/10.1515/znc-1987-0317 [Google Scholar] [CrossRef]
72. Britto, D. T., Kronzucker, H. J. (2002). NH4+ toxicity in higher plants: A critical review. Journal of Plant Physiology, 159(6), 567–584. https://doi.org/10.1078/0176-1617-0774 [Google Scholar] [CrossRef]
73. Takano, H. K., Dayan, F. E. (2020). Glufosinate-ammonium: A review of the current state of knowledge. Pest Management Science, 76(12), 3911– 3925. https://doi.org/10.1002/ps.5965 [Google Scholar] [PubMed] [CrossRef]
74. Wendler, C., Putzer, A., Wild, A. (1992). Effect of glufosinate (phosphinothricin) and inhibitors of photorespiration on photosynthesis and ribulose-1,5-bisphosphate carboxylase activity. Journal of Plant Physiology, 139(6), 666–671. [Google Scholar]
75. Barroso, A. L. L., Ferreira Filho, W. C., Menezes, C. C. E., San, H. A., San, L. G. M. et al. (2012). Selectivity of nicosulfuron and atrazine on different corn hybrids. Comunicata Scientiae, 3(4), 255–262. [Google Scholar]
76. Cobb, A. H., Reade, J. P. H. (2010). Herbicides and plant physiology. 2nd edition. West Sussex, UK: Wiley-Blackwell. [Google Scholar]
77. Hassannejad, S., Ghafarbi, S. P., Lotfi, R. (2020). The effect of nicosulfuron and bentazon on photosynthetic performance of common cocklebur (Xanthium strumarium L.). Environmental and Sustainability Indicators, 6, 100026. [Google Scholar]
Supplementary Materials
Figure S1: M. sinensis field in Chuncheon, South Korea treated with (i) Nicosulfuron, (ii) Dicamba, (iii) Bentazon, (iv) Glufosinate ammonium, (v) Alachor, (vi) Napropamide, (vii) Control
Figure S2: M. sacchariflorus field in Chuncheon, South Korea treated with (i) Nicosulfuron, (ii) Dicamba, (iii) Bentazon, (iv) Glufosinate ammonium, (v) Alachor, (vi) Napropamide, (vii) Control
Cite This Article
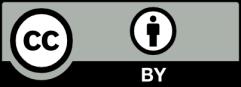
This work is licensed under a Creative Commons Attribution 4.0 International License , which permits unrestricted use, distribution, and reproduction in any medium, provided the original work is properly cited.