Open Access
ARTICLE
Antifungal Potential of Beauveria bassiana on Solanum lycopersicum L. Infected with Fusarium oxysporum f. sp. lycopersici
1 Laboratorio de Biotecnología vegetal, Tecnológico Nacional de Mexico/Campus Tuxtla Gutiérrez, Tuxtla Gutiérrez, 29050, Mexico
2 CONACYT -Tecnológico Nacional de Mexico/Campus Tuxtla Gutiérrez, Tuxtla Gutiérrez, 29050, México
3 ABACUS, Cinvestav, Ciudad de México, 07360, México
* Corresponding Author: Joaquín Adolfo Montes-Molina. Email:
Phyton-International Journal of Experimental Botany 2023, 92(4), 1235-1255. https://doi.org/10.32604/phyton.2023.025716
Received 27 July 2022; Accepted 17 November 2022; Issue published 06 January 2023
Abstract
The objective of this work was to evaluate the effect of Beauveria bassiana (Bb 1205) on controlling Fusarium oxysporum f. sp. lycopersici (Fol 17108) in tomato plants in greenhouse conditions. Inoculation of Bb 1205 was the most promising among the agronomic variables and expression of the activity of the enzymes β-1,3-glucanases and chitinases. Inoculation of Bb 1205 occurred at a concentration of 1 × 108 conidia·mL−1, which was administered onto the leaves, directly into the soil and via injection. Infection with Fol 17108 occurred with 1 × 106 spores·mL−1, which were added directly to the soil. Spectrophotometry was used for measuring agronomic parameters, namely activity of chitinases and β-1,3-glucanases in foliage and roots. When Bb 1205 was added to the soil, the chlorophyll index and aerial part length showed significant differences. In addition, it was determined that root length, fresh weight of foliage, flower, and fruit count increased 82 days after inoculation (dai). Chitinase activity induced by Bb 1205 in leaves and roots of tomato plants infected with Fol 17108 was observed when injected into the stem at 32 dai (41.8 and 11.6-fold, respectively). Inoculation on the foliage showed a 10-fold increase of β-1,3-glucanases in the roots after 82 dpi. As for leaves, a 3.8-fold increase was found when the stem was inoculated. In the different in vivo applications, Bb 1205 activated its defenses by expressing the chitinase enzymes and β-1,3-glucanase, thus reducing the damage caused by Fol 17108, demonstrating increase plant growth thereafter.Keywords
The excessive use of chemical pesticides for pathogen control has led to pesticide-resistant weeds. The bioaccumulation of pesticides in plant tissues causes potential risks to human health [1]. Therefore, biopesticides focus on having an effect on the target pathogen ecologically and sustainably for the environment. In this way, they are an alternative for more sustainable management in the phytosanitary field [2].
Entomopathogenic fungi (EF) are highly whidespread geographically and are highly capable of colonizing soil, which has led to their use as biological control agents worldwide. One of these EF is Beauveria bassiana fungus, which is an ascomycete that belongs to the Cordycipitaceae family of the Hypocreales order, capable of infecting more than 700 species of invertebrates through the cuticle via its conidia [3,4]. Furthermore, this order has great incidence as an asymptomatic endophyte in tissues of different plant species (Zea mays, Theobroma cacao, Phoenix dactylifera, Coffea L., Musa paradisiaca L., Pinus radiata, Vicia faba, Papaver somniferum, Gossypium, Manihot esculenta, Phaseolus vulgaris, and Solanum lycopersicum L.). Also, EF in this order can significantly modify the host plant while significantly modifying the presence of insect pests and diseases in the host plant [5–7].
Based on the needs for more environmentally friendly crop production and a more efficient use of resources, the management of some EF has been attempted in order to aid plants, and it has been shown that they can provide benefits by competing over the rhizosphere, phylosphere or intracellularly. They actively dispute the sources of carbon, nitrogen, and various microelements with pathogens [8]. B. bassiana can achieve these functions by producing secondary metabolites and extracellular enzymes with biological activity. Chitinase and glucanase enzymes (among others) are considered to be the most important metabolites produced by this EF [9,10]. Even when used only in minimal applications, they can help establish long-term protection to plants. Because of the smaller amounts used, they can benefit farmers by offering cost reductions as compared to the use of synthetic pesticides and can also reduce health risks for consumers [11].
The culture of S. lycopersicum L. L. is very important due to worldwide demand for bioactive compounds (lycopene, ascorbic acid, phenolic compounds, tocopherol, β-carotene, flavonoids, folates, fiber, and others). In addition, it has high economic value due to its consumption as fresh or processed product [12,13]. The production of S. lycopersicum L. has continued increasing because the producer has observed a cost-benefit relationship; for each peso invested, he acquires between 1.04 and 2.16 pesos of profit (this will depend on the system in place). However, in his total production costs, he invests 4.93% ($79,049.99) in inputs for pest and disease control, 38.08% ($609,500) in labor, including activities for the application of such inputs, as well as preventive or corrective management [14].
Known species of Fusarium cause a wide range of diseases in an extraordinary variety of plants. This soil-borne, cosmopolitan filamentous fungi group is vital because many members are the causative agents of vascular root rot or wilt diseases in agricultural and ornamental crops worldwide [15]. The losses caused in the culture of S. lycopersicum L. by this genus are due to Fusarium oxysporum f. sp. lycopersici (Fol), which favor vascular wilting due to the colonization of vascular tissue (forming a dark brown color), after which chlorosis in the lower leaves extending to the apex through the vascular bundle (xylem and phloem) can be seen, causing wilting, collapse, and plant death. This disease is on the rise due to the combination of mycotoxin production, tyloses production, mycelial accumulation, and the inactivation of host defenses [16].
A possible solution to these phytosanitary problems is the implementation of strategies such as the mycoparasitism of phytopathogenic fungi (PF), where EF present a good chemotropic growth of the mycelium towards the pathogenic fungus through recognition. With the help of appressoria, fungi can adhere effectively, facilitating the secretion of various hydrolytic enzymes. These will finally degrade the cell wall and membrane [2]. To date, the process of mycoparasitism of entomopathogenic fungi (for example, Lecanicillium spp. and Trichoderma spp.) has been thoroughly described in vitro in laboratory conditions but remains undemonstrated in the plant [17]. Some studies that have been carried out for the control of Fol in greenhouses with B. bassiana strain B2 have demonstrated the reduction of the pathogen, an increase in the number of branches, petioles, and a rise in fruit yields up to by 54%, 59%, 17%, and 36%, respectively. In addition, the expression of defense enzymes such as peroxidase, polyphenol oxidase, and lipoxygenase has been reported [18].
Similarly, endophytic colonization could be observed by inoculating seeds of Triticum aestivum L. with B. bassiana, obtaining a decrease in the incidence and severity of F. culmorum at 24 days after inoculation with 50% and 47% colonization in seeds, respectively [19]. In vitro and in vivo tests of B. bassiana allowed an observational study of the colonization of S. lycopersicum L. plants via the leaves with an effectiveness of 48%, reducing Botrytis cinerea infection to 36% [20]. The ability to antagonize B. bassiana on Fol race 3 in the laboratory showed an inhibition of 72% in the colonization of seeds, as well as an increase in chitinase enzyme production of up to 21% [10]. Aside from these findings, little evidence has demonstrated the antagonism of B. bassiana to plant-pathogenic fungi in the greenhouse.
Thus, the present study focused on controlling the pathogen F. oxysporum f. sp lycopersici (strain 17108) in vivo in S. lycopersicum L. plants with the B. bassiana strain 1205 with the objective of observing a promising application in agronomic variables and expression of enzymatic activity (β-1,3-glucanases and chitinases).
Seeds were provided by Eterno S. A. de C. V. and packaged in Guadalajara, Jalisco, Mexico. Seedlings of a Fusarium-susceptible Saladette supreme variety tomato (Solanum lycopersicum L.) were grown in 200 cavity trays from surface-sterilized seeds. This was done in accordance to the modified protocol established by [21]. 30 days after germination, they were transplanted into pots with sterilized peat moss (a substrate manufactured in Canada, endorsed by Premier Horticulture L TÉE1, Quebec, Canada GSR), and placed in a greenhouse. To keep plants in good conditions during the experiment, they were fertilized with different nutrient solution percentages, depending on the crop’s different phenological phases according to [22].
The study was carried out in the Biotechnology and greenhouse soil laboratory of the Tecnológico Nacional de México Campus Tuxtla Gutiérrez (latitud of 16.758311°N, longitude-93.172347 W). The experiments used for evaluation were treatments without inoculation, with entomopathogen inoculum, treatments with interactions, and treatments that were inoculated only with the pathogen. Treatments without inoculation were control plant + foliar application of distilled water (PCf), control plant + injected application of distilled water (PCi), and control plant + application in the soil of distilled water (PCs). As for treatments with entomopathogen inoculum, these were plant + foliar application of B. bassiana (PBf), plant + injected application of B. bassiana (PBi), and plant + soil application of B. bassiana (PBs). The interaction treatments were plant + Fol + foliar application of B. bassiana (PFBf), plant + Fol + injected application of B. bassiana (PFBi), and plant + Fol + application in the soil of B. bassiana (PFBs). Lastly, treatments including only the pathogen were plant + Fol + foliar application of distilled water (PFf), plant + Fol + injected application of distilled water (PFi), and plant + Fol + application in the soil of distilled water (PFs). The distribution of the experiment was carried out in a completely randomized design with 12 treatments and nine repetitions per treatment, whereby the experimental unit was a pot with a plant.
2.2 Inoculation of Fusarium oxysporum f. sp. lycopersici
The Fol strain (17108) (Not registred) was provided by the Plant Breeding Department of the University Autonomous Agrarian Antonio Narro, based in Saltillo, Coahuila, Mexico. To guarantee infection, plants were inoculated with a stock solution with a concentration of 1 × 106 spores·mL−1 was used according to descriptions by [23] with modifications. Consecutively, 10 mL of the Fol 17108 spore suspension were used to infect the substrate directly for the PFf, PFi, PFs, PFBf, PFBi, and PFBs treatments. In addition, 10 mL of sterilized distilled water were applied to the control plants and to those treated with B. bassiana, and 10 mL of sterilized distilled water plus the Bb spores were also applied. This application happened only once and occurred five days after transplanting (dat).
2.3 Inoculation of Beauveria bassiana (Bb)
The entomopathogenic fungus Bb 1205 (accession number in the Genbank KX232465.1) was donated by the Technological Institute of Tlajomulco, located in Tlajomulco de Zúñiga, Jalisco, México. The inoculum preparation followed the description provided by [24], where a solution of 1 × 108 conidia·mL−1 was obtained. Ten mL of the inoculum were taken and applied directly on the substrate for PBs and PFBs treatments, and 10 mL were used for spraying foliage for PBf and PFBf treatments. For the corresponding PBi and PFBi treatments, 0.5 mL of the conidia suspension were taken out with the help of a hypodermic needle and injected at the base of the stem, at a height of 1 cm above the substrate. Sterile distilled water was used as the control and PFf, PFi, and PFs treatments (foliar, injected, and soil application). Subsequent applications of Bb 1205 occurred in the same manner as described above and were applied every eight days, until reaching 90 days.
2.4 The Effect of Fusarium oxysporum f. sp. lycopersici and Beauveria bassiana on the Chlorophyll Index and Agronomic Variables
Six evaluations were carried out at 8, 16, 24, 32, 58, and 82 days after inoculation (dai). Three measurements were done for each plant in order to report the chlorophyll index, for which a Minolta SPAD 502 plus chlorophyll meter was used by directly measuring the intensity of chlorophyll in the leaf according to [25]. In addition, the length of the aerial parts of S. lycopersicum L. plants was measured with a fluxmeter. Measurements were taken from the base of the plant to the axil of the youngest leaf. Stem diameter was measured at the base of the stem at a height of 1 cm above the substrate with the help of a vernier caliper. Chlorophyll measurements were performed in triplicate, for which three measurements were done in three different leaves per plant following the methodology of [26]. For all the variables mentioned above, readings were taken every eight days after inoculation day (dai) with Bb 1205. Additional agronomic data obtained included the 82 dai root length with a measuring tape, fresh plant weight (foliage and root) in grams with the help of an analytical balance (Ohaus) according to [27], number of flowers, and number of fruits by visual count as described by [28].
2.5 Enzyme Assay of S. lycopersicum L. Plants Inoculated with Beauveria bassiana and Infected with Fusarium oxysporum f. sp. lycopersici
Total protein extraction was performed from leaves and roots of tomato plants as described by [10]. Samples were collected at eight dai with Bb 1205 and then evaluated 6 times (8, 16, 24, 32, 58, 58, and 82 dai), which were frozen in liquid nitrogen and preserved at −70°C until processing. Total protein concentration was determined with the Bio-Rad kit (Bio-Rad Laboratories, USA), which is based on the Bradford method [29], where three replicates for each treatment were performed.
For the identification of chitinases in leaves and roots, the discontinuous colorimetric method described by [30] and modified by [10] was implemented, for which colloidal chitin made from reagent-grade chitin was used (Sigma-Aldrich). Absorbance readings were carried out at 585 nm in a spectrophotometer. For the enzymatic activity of ß-1,3-glucanases in leaves and roots, the discontinuous and colorimetric method modified by [10] was carried out, where laminarin (Sigma-Aldrich) is used as a substrate. The generated sugars were quantified with a spectrophotometer by the Nelson-Somogyi method [31] at an absorbance of 660 nm. In addition, the specific activity of each enzyme was converted and reported in microkatals (µKat) and picokatals (pKat) per milligram of total protein (pKat/mg protein).
The data obtained from the chlorophyll index, agronomic variables, quality, specific activity of chitinases and ß-1,3-glucanases, was analyzed with analysis of variance (ANOVA). Obtained data was compared with a test of means by Tukey’s method (p ≤ 0.05) with the help of the Statgraphics Centurion XVI.I. computer package.
Plants in the control treatment with Fusarium oxysporum began to show signs of wilting 60 days after the transfer. At the end of the study, it was observed that 60% of the control plants that were contaminated with F. oxysporum were dead, which helped in verifying the severity of the damage of this pathogen. As for the other treatments in which F. oxysporum was also ammended, all plants survived.
3.1 Effect of the Application of B. bassiana (1205) on S. lycopersicum L. Plants Infected with Fusarium oxysporum f. sp. lycopersici (17108) in Agronomic Variables and Chlorophyll Index
The application of Bb 1205 on Fol 17108-infected plants as a potential biological control method could prove to be a suitable method of biological control. Results in Table 1 show the chlorophyll content value found in the greenhouse trial, showing a decrease in chlorophyll content after 24 dai for all treatments where only Fol 17108 appeared, as well as the death of plants in the same treatments after 58 dai. A more significant loss was observed due to the PFs treatment as compared to other forms of infection (PFf and PFi). In general, it was shown that Bb 1205 preserves 53.6% (at 24 dai) of SPAD levels in S. lycopersicum L. plants infected with Fol 17108. The effect demonstrated by Bb 1205 on SPAD values in S. lycopersicum L. L. showed a statistically significant difference in the PFBi treatments at 32 dai and PFBs at 16 and 24 dai, with an increase of 36%, 50%, and 23%, respectively.
When analyzing the effect of Bb 1205 for the length variable of S. lycopersicum L. (Table 2) infected with Fol 17108, it was shown that the PFBs treatment presented a statistically significant difference in three stages of the experiment (16, 24, and 32 dai), as compared to other treatments, with 26.6%, 27.8%, and 20.3%, respectively.
In regard to the stem diameter parameter of S. lycopersicum L. (Table 3), it is evident that the PFBf treatment was the one that best induced an increase as of 16 dai, which continued at 24, 32, and 82 dai. At 58 dai, it was shown that, when inoculating S. lycopersicum L. plants foliarly with Bb 1205, an increase of 17.4% was observed, whereby a statistically significant difference associated to all other treatments was evident.
In order to evaluate the effect of Bb 1205 on S. lycopersicum L. at the end of the experiment, other morphometric and harvest variables also were considered (Table 4). It was shown that at 82 dai, the interaction of Bb 1205 and Fol 17108 did not generate significant statistical differences in total plant length, foliage length, fresh root weight, and whole plant. However, an average increase of 30% in total height, 54% in root length and up to 20.7% in canopy length was found.
3.2 Enzymatic Assay of Solanum lycopersicum Plants Inoculated with Beauveria bassiana and Infected with Fusarium oxysporum f. sp. lycopersici
The chitinase enzyme activity in the root of S. lycopersicum L. inoculated with Bb 1205 appears in Table 5. Before S. lycopersicum L. plants were attacked by Fol 17108, they showed low activity since the beginning of the experiment until 32 dai, where all treatments resulted in death. The PFBi treatment presented specific activity with a statistically significant difference in regard to the control on days 8, 32, and 82 dai (1.9, 41.8, and 9.5 times more in the enzyme activity, respectively). Thus, they are inducing a decrement in the intensity of the attack by the phytopathogenic fungus.
Chitinase activity in S. lycopersicum L. leaves (Table 6) showed that the enzyme activity decreased in the first two evaluations of the experiment. For 24 and 32 dai, statistically significant values were obtained in the PFBi treatment with 2.3 and 11.6 times more than the control. PFBf also showed activity at 58 dai with 4.3 times greater activity. Finally, PFBs treatment showed 5.8 times greater activity at 82 dai.
The enzymatic activity of β-1,3-glucanase in S. lycopersicum L. roots inoculated with Bb 1205 and infected with Fol 17108 can be seen in Table 7. The results indicate that the expression of the enzyme remained basal in the first three evaluations. Subsequently, they presented statistically significant differences compared with the control in PFBf treatment at 32 and 82 dai, as the enzmatic activity increased by 3.2 and 10 times, respectively. On the other hand, PFBs treatment generated only increased activity after 58 dai, showing a 35.7-fold rise.
Regarding the specific activity of the β-1,3 enzyme-glucanase, results were obtained from leaf samples of S. lycopersicum L. infected with Fol 17108, which were subsequently treated with Bb 1205 via foliar and soil applications, as well as by injections (Table 8). It was demonstrated that the enzyme activity had a statistically significant difference in the PFBi treatment at 24 and 82 dai, expressing 1.1 and 3.8 times more activity of β-1,3-glucanase, respectively. Also, the PFBf treatment showed an increase of 1.1 and 1.6 times after 32 and 82 dai, correspondingly.
4.1 Effect on Agronomic Variables and Chlorophyll Index after Application of B. bassiana (1205) on S. lycopersicum L. Plants Infected with Fusarium oxysporum f. sp. lycopersici (17108)
Our study demonstrated the ability of Bb 1205 in the PFBs treatment in doubling the chlorophyll index (Table 1), as compared to the data published by [32], where they obtained an increase in total chlorophyll (22.5%) from S. lycopersicum L. plants inoculated with T. asperellum in leaves infected with F. oxysporum. However, at the end of the experimentation, the PBi (58 and 82 dai) and PBs (82 dai) treatments presented statistically significant differences, increasing SPAD values in the plants of 26.6%, 5.4%, and 11%, respectively. Our results at 58 dai show that Bb 1205 behaves as an agent in the control of Fol and also increases the chlorophyll content, relating to the data obtained by [33] in S. lycopersicum L. plants inoculated with T. asperellum and infected with Fol at 60 dat, showing that the total chlorophyll content also increased by 15.5%. It was possible to determine that B. bassiana is a mutualistic fungus and it is likely that there was plant tissue colonization without generating an apparent negative effect (i.e., absence of phytopathogenic activity). On the contrary, it has been described as contributing towards the acquisition of nutrients (such as iron) for the development of the host, towards improving photosynthetic productivity and concentration of total chlorophyll, and towards promoting tolerance to salinity in the plant [34–36]. Also relating to this, Quesada et al. [37] reported evidence of the endophytic and mutualistic colonization of B. bassiana on Papaver somniferum L. and its capacity to be transmitted vertically throughout a period of 120 dai. Positive development in S. lycopersicum L. plants by B. bassiana has been shown to occur when applied as a solid on the substrate, or by foliar spray, root dipping, and stem injection [38–40]. Recently, Nicotiana benthamiana inoculated with B. bassiana showed significant increases in chlorophyll a and b at 40 dai, from 37% to 46% and 33% to 40%, respectively [41]. Our results show low chlorophyll contents in treatments where Bb 1205 occurred, coinciding with those reported in Manihot esculenta plants with B. bassiana, where nutrient allocation occurs on the stem and root growth and tiny leaves [6]. Because of this, it is possible that lower leaf area and less adequate chlorophyll production occurred in S. lycopersicum L. It has also become apparent that the host plant determines the level of photosynthetic pigments when subjected to stress caused by a fungus or insect. Environmental factors can also influence chlorophyll synthesis and the adaptive process of the plant [42].
The results in Table 2 coincide with reports for S. lycopersicum L., where it is likely that the colonization of B. bassiana is limited to the inoculation region. It enters the mesophyll when applied in the substrate at concentrations of 1 × 108 conidia·mL−1, and it is localized mainly in the stem or roots, and a colonization efficiency of 100% will be reached at 14 dai [43,44] achieved greater plant heights, reporting an increase of 12.5% after 30 dai in S. lycopersicum L. plants inoculated at the root with B. bassiana. An increase of 19.2% was also described for Vitis vinifera at 53 dai [45].
Parsa et al. [24] reported that Beauveria bassiana is capable of colonizing Phaseolus vulgaris cv. Calima regardless of the inoculation technique, when a solution is sprayed at a concentration of 1 × 108 conidia/mL on leaves, is capable of colonizing 30% of the plants; when applied to the soil it reaches a colonization index of 25% in roots and colonizes stems by 10% when sprayed and when placed in soil it reaches a colonization of 15%.
At 82 dai, it was found that the best treatment was when Bb 1205 was injected into the stem of the S. lycopersicum L. plant and infection with Fol 17108 occurred (Table 2), where its ability to provide a protective effect in a complete production cycle was demonstrated. Our results were very comparable to those obtained for Triticum durum cv. Carpio with the application of B. bassiana in the soil, where an increase of 9.5% in the length of the plant at 31 dai was achieved [46]. The colonization of B. bassiana in S. lycopersicum L. plants has been reported as needing as much of 30 dai of EF, whereby proper colonization throughout the plant and a reduction in the survival of pests such as Tuta absoluta was observed, regardless of application type [44]. The studies carried out for Gossypium hirsutum L. used treatments with B. bassiana (1 × 107 conidia·mL−1). At 28 dai, the pathogens Rhizoctonia solani, Xanthomonas axonopodis pv. Malvacearum were controlled. Also, an increase in the height of up to 33% was achieved and a 10% decrease in the severity was observed in plants infected by the pathogens Rhizoctonia solani, Xanthomonas axonopodis pv. Malvacearum, respectively. There was also evidence of possible mycoparasitism of EF on these fungi phytopathogens [47]. Similarly, it showed that Trichoderma spirale had been used for the control of F. oxysporum on S. lycopersicum L. cherry type in a greenhouse, presenting an increase in plant length of up to 20% after 60 dat [48]. In comparison with our results, it appeared that Bb 1205 shortened the expression time needed for increasing length in S. lycopersicum L. plants.
It could be observed that Bb 1205 impacted solely on increased enzymatic activity of chitinases and β-1,3-glucanase (II). As noted in the in vitro assays with Arabidopsis thaliana with different applications of B. bassiana against Sclerotinia sclerotium attack, a significant reduction in the lesion area of the phytopathogen was positively regulating up to 142 differentially expressed genes, which were related to jasmonic acid, salicylic acid, and ethylene. Also, the induction of 31 genes was observed, all of which are involved in cell organization, division, and transport in the plant, cell wall formation (10 genes). One of these genes is also related to the photosynthesis process [49]. Salicylic acid was expressed (9.34% at flowering), as well as jasmonic acid (shoot development by 18.9%) when B. bassiana was available in the substrate. This was similar to what was reported by [46], where Triticum durum plants showed an increased expression of abscisic acid (10.34% at the flowering stage). Similarly, Pieterse et al. [50] mentioned that EF can be plant growth promoters (PGPF) by inducing salicylic acid, lipopolysaccharides, and iron regulatory metabolites such as pyoverdine.
Steiner [22] identified 180 B. bassiana metabolites that induce virulence factors (purine metabolic pathway activation), reactive oxygen and nitrogen responses; antioxidant compounds (acting on methionine and cysteine metabolic pathways), AMP-activated protein kinase expression, Janus-kinase family signaling pathways, and activators of transcription (JAK/STAT, which increase enzyme activity). They act to regulate the cell cycle, vegetative growth and strengthen the plant’s immune system against pathogen infections. In addition, it shows that it can influence abiotic stress (thermotolerance), conidia quality and antioxidant activity in plants through genes such as cat2 and cat5.
In regard to the stem diameter parameter of S. lycopersicum L. (Table 3), a study carried out on plants of Cucumis melo var. reticulatus inoculated foliarly with B. bassiana showed via histological studies that a penetration and colonization capacity of EF in intercellular spaces and on the surface of the leaves of up to 40% of colonization can occur in as little as 96 h after inoculation. Endophytes and the production of secondary metabolites were reported, which prevented the spread of Bemisia tabaci throughout the plant [51]. Our results show similar timeframes to those of [44], where the colonization of B. bassiana in S. lycopersicum L. leaves was achieved at 14 dai with an increase in 53% in stem thickness at 25 and 30 dai. The ability of B. bassiana to induce positive effects on agronomic variables in plants could also be observed in the V. vinifera trial, where a stem growth by 6.8% was observed at 53 dai. These variations in stem increment could be due to the physiological circumstances of EF, such as a relationship between level of tissue specificity and the response of the host plant system [45]. The greenhouse experiment with T. harzianum applied on roots of S. lycopersicum L. with F. oxysporum showed a four-fold increase in stem diameter at 45 dat as compared to the control and to plants infected only with the pathogen, suggesting antifungal activity [52]. This is highly likely because of the support induced by the fungus, whereby the ability to absorb nitrogen and phosphorus is enhanced, likely improving the development of the plant. Thus, the host provides a carbon source to EF [53].
This behavior is observed in our results, the content of chlorophyll, diameter of the stem as well as in some morphometric variables are higher when the plants are co-inoculated, probably the development of the plant improves due to the solubilization of phosphorus and nitrogen caused by both microorganisms. It has been reported that B. bassiana in crop development can promote shoot and root biomass when the status of macronutrients such as nitrogen, carbon and essential microelements in plant nutrition are high [8]. On the other hand, the production of hydroxamate-type siderophores and the solubilization of phosphate by B. bassiana promote the development of biomass, height, and photosynthetic activity [20].
The effect of Bb 1205 on S. lycopersicum L. at the end of the experiment is shown in Table 4. These results are very similar to those obtained by [54] when inoculating seeds of P. vulgaris L. with B. bassiana, where after 14 dai an increase in the fresh weight of the root and the length of the foliage was observed (27.3% and 47.3%, respectively). Also, by applying B. bassiana in the soil, Culebro et al. [10] generated an increase of 13.7% in foliage length in S. lycopersicum L. plants infected with Fol race 3, as well as an increase in root length of 33.3% by the end of the experiment (25 dai). Compared with our results of the PFBs treatment, these parameters had increased by 21.6% and 64.3%, respectively. In experiments with Capsicum annuum L. seeds inoculated with B. bassiana, a 33% increase in root length was achieved at 121 dai [35] and for P. vulgaris L. a 12.5% increase was observed when applied to the ground [55]. The average increase in fresh root weight of the PFBf, PFBi, and PFBs treatments was as high as 41%, despite the attack by the phytopathogen. As for quality parameters, in spite of not finding any statistically significant differences, it was possible to generate an increase the quantity of flowers by 37.6% than in the control, out of which 52.8% became fruit. Sánchez et al. [56] demonstrated for the first time that B. bassiana promotes the assimilation of phosphorus, potassium, iron, and zinc in S. lycopersicum L. plants, causing a significant increase in the biomass of the plant, in the length of its different organs, and in the possibility to decrease the pH of alkaline soils, which would imply an increase in production. In Sorghum bicolor L., it showed that there is also an increase in the number of leaves and in iron uptake for plant growth [36]. In Brassica oleracea var. capitata L., the ability to absorb nitrogen, tolerance to water stress, and plant health rose to 25% [57]. From the reported results, the decrease in the attack of Fol 17108 could be replicated on the fields when using strain Bb 1205. An increased production of S. lycopersicum L. may be related to the ability of another EF (T. asperellum) in reducing the incidence of the disease caused by Fol as much as 70.6% at 60 dat in S. lycopersicum L. plants [33].
4.2 Enzymatic Assay of Solanum lycopersicum Plants Inoculated with Beauveria bassiana and Infected by Fusarium oxysporum f. sp. lycopersici
Based on the results in Table 5, chitinase enzyme activity in the plant that is induced by Bb 1205 inoculation throughout the crop cycle is confirmed. One of the two organic and renewable polysaccharides that are abundant in the ecosystem is chitin (poly-β-1,4-N-acetylglucosamine), which is present in marine organisms, insect exoskeletons, and fungal cell walls [58]. In filamentous fungi, the wall essentially consists of 10% to 20% chitin, as well as β-1,3-and β-1,6-glucans. Also, it is part of the fungal defense against chemical compounds and physical environmental conditions [59,60]. In Fol, it is found as an outer layer [61]. Plants detecting and triggering defenses against fungi take advantage of pathogen-associated molecular pattern receptors (MAMPs), which are pattern recognition receptors (PRRs) and pathogenesis-related protein (PR) synthesis [62]. Despite having these defense mechanisms, the pathogenic fungus protects its chitin fibrils against detection and hydrolysis with effectors (for example, AVR and LysM), and with expression of deaminases to change chitin to chitosan [59]. Also, the exudation of proteins retains MAMPs, interrupting the downstream responses of the plant [63].
The use of B. bassiana is based on evidence available regarding the ability to produce extracellular lytic enzymes, chitinases and ß-1,3 glucanases to degrade the cell wall of the phytopathogenic fungus through mechanisms of mycoparasitism, competition, or local accumulation at the infection site and the surrounding tissues [64,65]. From the in vitro assay in which F. oxysporum served as a carbon source for B. bassiana, it was possible to verify the production of the CHIT1 gene encoding chitinase with a 2.2-fold increase, degrading and taking advantage of the pathogen as a food source as of seven dai [66]. A somewhat similar phenomenon was demonstrated when R. solani was used as a substrate for B. bassiana, reaching peak enzymatic activity at four dai (1.1 U·mL−1 of catalytic activity). However, it also showed that the enzyme is slow in being expressed at three dai, and that its activity is reduced after the peak is reached (four dai) until the end of the assay at eight dai [67].
Similarly, chitinase inhibits F. oxysporum by cleaving the C1 and C4 bonds of the chitin in the cell wall, causing the release of elicitors, inducing a plant response in Linum usitatissimum L. plants. Once infected with F. oxysporum, there is a decrease in chitinase genes expression (CMT1 and CMT3) by up to 50% after six h post-infection, and there is also a decrease in the ROS1 gene by 20% at 36 h [68]. Something similar occurred in Cucumis sativus L. plants, which produce chitinases from the GH19 family (CsChi23) in a basal manner, which have more significant activity in setting up defense and activating a rapid immune response against F. oxysporum f. sp. Cucumerinum [69]. Our results are related to the expression of chitinases in the control treatments in roots (Table 5) and leaves (Table 6) of S. lycopersicum L. inoculated with Bb 1205. Data published by [70] demonstrated the ability of B. bassiana to produce an endochitinase (Bb chit1) to improve virulence and interact with proteases, thus hydrolyzing the cuticle of insects and possibly also the wall of filamentous fungi. The tests carried out by [10] showed that B. bassiana can induce chitinase activity at two dai in S. lycopersicum L. infected with Fol race 3. Consistent results with the PFBi treatment showed a 96% increase in chitinase production during the early stages of our experiment. The expression of chitinases induced by EF has demonstrated the ability in plants to decrease cell death and in improving resistance to F. oxysporum [71]. When T. erinaceum was used on S. lycopersicum L. contaminated with Fol, the ability to increase chitinase expression up to 3-fold at two dai in plant roots was observed (as compared with control), as demonstrated by RT-PCR. This is in addition to the increased expression of the SlWRKY31 gene by 16.5% after 24 h, where these genes (WRKY) are responsible for inducing the defense reaction in the plant, resulting in induced systemic resistance (ISR) and in causing plant growth [72].
The stimulation of B. bassiana colonization in plants depends on its ability to express transcription factors (such as C2H2, BBA_00971), G protein-coupled receptors (such as Pth11, BBA_03214), and signal transduction kinases that translate amino acids into the cell. surface of the cuticle of the leaf, as well as mobilization of nitrogenous nutrients within the plant; this suggests a general strategy for the development of B. bassiana and the production of lytic enzymes such as chitinases to protect plants from vascular pathogens [73].
It was observed that enzyme activity is lower in leaves of S. lycopersicum L., compared to activity observed in roots. Results derived from the use of Trichoderma sp. in the soil of Fol-infected S. lycopersicum L. plants showed a 1.1-fold increase in chitinase gene expression at 128 dat compared to the control [74]. This activity is much higher in our PFBs treatment, confirming that Bb 1205 is a biological control agent of phytopathogenic fungi. It has been reported that B. bassiana is capable of producing up to 20 chitinases from the GH18 family due to the need to secrete them before host arrival [75]. This could explain why a more significant activity of the enzyme in roots and leaves of S. lycopersicum L. was observed, especially in the PFBi treatment. Our results were lower in chitinase yields than values reported by [72], which was up to 18-fold higher in leaves. It was also proven that SlWRKY37 gene transcription could be induced up to 14% for activating plant immunity against a Fol attack.
On the other hand, the enzymatic activity of β-1,3-glucanase in roots is shown in Table 7. It can be seen that our data are higher reduced activity to those obtained by [72], where they used T. erinaceum in the Fol control, from which enzyme production was up to 5 times higher than for control. Furthermore, our results demonstrated the ability to induce the expression of β-1,3-glucanases by Bb 1205 in a higher proportion in the greenhouse, compared to those presented by [10], where activity was much lower throughout the trial. The β-glucan polysaccharides are the most abundant in the fungal wall, comprising between 50% and 60% of the dry weight. These are synthesized at the tip of the hyphae during cell growth [59,76], but in Fol it is found as an internal layer [61]. The ability of B. bassiana to use F. oxysporum as a carbon source in the production of β-1,3-glucanases has been observed in the in vitro test carried out by [77], where expression of 60% more Exo-β-1,3-glucanase genes was reported as of 7 dai. The results found by [78] show that the β-1,3-glucanases produced by Bacillus sp., show a peak activity in the middle of experimentation (15.61 U mL−1·min−1), which subsequently decrease. This is probably related to the activity of using Fol as a carbon source. In the same manner, the use of Pseudomona fluorescens in roots of S. lycopersicum L. displayed (13 dai) a 2.2-fold increase in enzymatic activity promoting an ISR in the plants at the end of the experiment, which resulted in a reduction of the severity of of disease and in the optimization of the other enzymes, which could be related to the phenylpropanoid pathway [79]. The PFBf treatment’s effects at the end of the trial demonstrated a much more significant increase of up to 7.8-fold, according to the data mentioned above. The accumulation and expression of the enzyme are affected by the penetration site of Fol, which determines the release of oligosaccharides, and will be inducing responses for the synthesis of lignification and phytoalexins in the plant [80].
Results in Table 8 demonstrated that Bb 1205 is a fungus with a similar potential to that of T. erinaceum against Fol attack on tomato plants, as T. erinaceum exhibits a 4.5-fold increase in the induction of β-1,3-glucanases in leaves [72]. This is in close proximity to our results. In addition, Cucumerinum, β-1,3-glucanase activity was observed all along the experimentation (2.3, 3.5, 1.5, and 1.5 times more than the control), similar to levels expressed in our experiment [71]. From the above, it was confirmed that β-1,3-glucanases can be found in chloroplasts before infection by a pathogen, after which they are transferred to the vacuole and finally to the wall, in order to control the infection. Therefore, the induction by some external agent is essential in triggering the active defense of the plant [81]. For this reason, amplification of the enzyme expression was probably achieved in the PFBf treatment (Table 8) in our in vivo assay. It has become evident that entomopathogenic fungi such as Trichoderma can induce the formation of phenolic compounds and reactive oxygen species in the plant, which improve the mechanical resistance in the wall, thus contributing in inhibiting the development of phytopathogenic fungi [82]. Also, the control of Fol by Bacillus spp. on tomato plants under greenhouse conditions generated siderophores, which decreased disease incidence (up to 55%), plant growth (44%), and β-1,3-glucanase activity by up to a factor of 1.4 [83]. Likewise, the production of jasmonic acid by Bacillus sp. SJ-5 with the antifungal activity of F. oxysporum in Glycine max L. Merrill of 99% has been reported [84].
Our results show that the activity of the enzymes chitinase (Tables 5 and 6) and glucanase (Tables 7 and 8) increase in tissues that were not inoculated (root). This increase could be occurring because B. bassiana is capable of colonizing and moving systemically to different tissues, regardless of the method of inoculation. as well as the tissue where it is inoculated, this behavior has been reported in other species such as Helicoverpa armigera, Vitis vinifera, Arabidopsis thaliana and Phaseolus vulgaris L. [40,45,49,55].
In this study, death was observed in 60% of the control plants that were contaminated with Fusarium oxysporum, with which the severity of the damage by this pathogen was confirmed. The entomopathogenic fungus Beauveria bassiana 1205 presented endophytism in roots and leaves of Solanum lycopersicum L. without affecting the development of the plant. The presence of Beauveria bassiana 1205 on S. lycopersicum L. infected with Fusarium oxysporum f. sp. lycopersici 17108 is responsible for increasing the greenness index and the height of the aerial part when applied to the soil. Application to the soil also caused improvements in the quality variables. Stem diameter proved to increase when application was done on the leaves. Regarding the production of chitinases in the root part and leaves of S. lycopersicum L. infected with Fusarium oxysporum f. sp. lycopersici 17108, the best treatment was when applied by injection into the stem (presenting higher specific activity of chitinases in roots). The specific activity of β-1,3-glucanase was higher in roots when used on the leaves, and enzyme activity in leaves occurred when injected into the stem. In short, Beauveria bassiana 1205 stimulated the biological control on Fusarium oxysporum f. sp. lycopersici 17108, inducing and enhancing an active defense of the plants and allowing maturity of the fruits to occur. Finally, the fungistatic potential of Beauveria bassiana 1205 in the expression of lytic enzymes for the control of Fusarium oxysporum f. sp. lycopersici and its possible incorporation into integrated disease management for sustainable agriculture was confirmed.
Acknowledgement: We would like to thank the Universidad Autonoma Agraria Antonio Narro and the Tecnológico de Tlajomulco for donating the strains used in this research. Tecnológico Nacional de México (TecNM). We also show gratitude towards CINVESTAV Zacatenco, Soil Ecology Laboratory and Dr. Luc Dendooven, to the Consejo Nacional de Ciencia y Tecnología (CONACyT)-México for the scholarship awarded to Henry López López and the Electrón S.A. de C.V. Drinking Water Company.
Authorship: The authors confirm contribution to the paper as follows: Study conception and design: M-M JA., M-G R., R-L N., L-L H.; Analysis and interpretation of results: L-L H., M-M JA., D L., R-V VM.; Supervision: M-M JA., V-M JJ., L-G CA.; Translation and editing of the manuscript: R-L JG., L-G CA.; All authors reviewed the results and approved the final version of the manuscript.
Funding Statement: The project was funded by the Tecnológico Nacional de México (TECNM): Project No. 6602.18-P.
Conflicts of Interest: The authors declare that they have no conflicts of interest to report regarding the present study.
References
1. Paramanandham, P., Rajkumari, J., Pattnaik, S., Busi, S. (2017). Biocontrol potential against Fusarium oxysporum f. sp. lycopersici and Alternaria solani and tomato plant growth due to plant growth-promoting rhizobacteria. International Journal of Vegetable Science, 23(4), 294–303. DOI 10.1080/19315260.2016.1271850. [Google Scholar] [CrossRef]
2. Ram, R. M., Keswani, C., Bisen, K., Tripathi, R., Singh, S. P. et al. (2018). Biocontrol technology: Eco-friendly approaches for sustainable agriculture. En omics technologies and bio-engineering: Towards improving quality of life. Omics Technologies and Bio-Engineering, 2(15), 177–190. DOI 10.1016/B978-0-12-815870-8.00010-3. [Google Scholar] [CrossRef]
3. Doolotkeldieva, T., Bobusheva, S., Kulmanbetova, A., Zholdoshbekova, S., Kyzy, A. A. (2019). Characterization of Beauveria bassiana isolates from Kyrgyzstan. Journal of Invertebrate Pathology, 167(1–4), 107–243. DOI 10.1016/j.jip.2019.107243. [Google Scholar] [CrossRef]
4. Filippou, C., Diss, R. M., Daudu, J. O., Coutts, R. H. A., Kotta, L. I. (2021). The polymycovirus-mediated growth enhancement of the entomopathogenic fungus Beauveria bassiana is dependent on carbon and nitrogen metabolism. Frontiers in Microbiology, 12, 1–11. DOI 10.3389/fmicb.2021.606366. [Google Scholar] [CrossRef]
5. Busby, P. E., Ridout, M., Newcombe, G. (2016). Fungal endophytes: Modifiers of plant disease. Plant Molecular Biology, 90(6), 645–655. DOI 10.1007/s11103-015-0412-0. [Google Scholar] [CrossRef]
6. Greenfield, M., Gómez, J. M. I., Ortiz, V., Vega, F. E., Kramer, M. O. et al. (2016). Beauveria bassiana and Metarhizium anisopliae endophytically colonize cassava roots following soil drench inoculation. Biological Control, 95(2), 40–48. DOI 10.1016/j.biocontrol.2016.01.002. [Google Scholar] [CrossRef]
7. Russo, M. L., Scorsetti, A. C., Vianna, M. F., Cabello, M., Ferreri, N. et al. (2019). Endophytic effects of Beauveria bassiana on corn (Zea mays) and its herbivore, Rachiplusia nu (Lepidoptera: Noctuidae). Insects, 10(110), 1–9. DOI 10.3390/insects10040110. [Google Scholar] [CrossRef]
8. Tall, S., Meyling, N. V. (2018). Probiotics for plants? Growth promotion by the entomopathogenic fungus Beauveria bassiana depends on nutrient availability. Microbial Ecology, 76(4), 1002–1008. DOI 10.1007/s00248-018-1180-6. [Google Scholar] [CrossRef]
9. Amobonye, A., Bhagwat, P., Pandey, A., Singh, S., Pillai, S. (2020). Biotechnological potential of Beauveria bassiana as a source of novel biocatalysts and metabolites. Critical Reviews in Biotechnology, 40(7), 1019–1034. DOI 10.1080/07388551.2020.1805403. [Google Scholar] [CrossRef]
10. Culebro, R. J. M., Ruiz, V. V. M., Rodriguez, M. M. A., Avila, M. M. E., Gutierrez, M. F. A. et al. (2017). Antifungal properties of Beauveria bassiana strains against Fusarium oxysporum f. sp. lycopersici race 3 in tomato crop. Journal of Environmental Biology, 38(5), 821–827. DOI 10.22438/jeb/38/5/MRN-412. [Google Scholar] [CrossRef]
11. Harith, F. N., Abd, G. I., Hassan, M. (2021). Omics-based approach in characterizing mechanisms of entomopathogenic fungi pathogenicity: A case example of Beauveria bassiana. Journal of King Saud University-Science, 33(2), 101332. DOI 10.1016/j.jksus.2020.101332. [Google Scholar] [CrossRef]
12. Navarro, G. I., Periago, M. J. (2016). Is tomato a healthy and/or functional food? Revista Española de Nutrición Humana y Dietética, 20(4), 323–335. DOI 10.14306/renhyd.20.4.208. [Google Scholar] [CrossRef]
13. Olveira, B. V., Pita, C. C., Vázquez, O. M. L., Romero, R. M. A. (2021). Evaluation of a modified atmosphere packaging system in pallets to extend the shelf life of the stored tomato at cooling temperature. Food Chemistry, 364(4), 130309. DOI 10.1016/j.foodchem.2021.130309. [Google Scholar] [CrossRef]
14. Terrones, C. A., Sanchez, T. Y., Robles, F. V. H., Vargas, S. J. R. (2020). Rentabilidad económica de la producción de jiS. lycopersicum L. en valle de Tulancingo, Hidalgo, México: 2018–2019. Revista Mexicana de Agronegocios, 52(47), 595–606. DOI 10.22004/ag.econ.308715. [Google Scholar] [CrossRef]
15. Singha, I. M., Kakoty, Y., Unni, B. G., Das, J., Kalita, M. C. (2016). Identification and characterization of Fusarium sp. using ITS and RAPD causing Fusarium wilt of tomato isolated from Assam, North East India. Journal of Genetic Engineering and Biotechnology, 14(1), 99–105. DOI 10.1016/j.jgeb.2016.07.001. [Google Scholar] [CrossRef]
16. Srinivas, C., Nirmala, D. D., Narasimha, M. K., Mohan, C. D., Lakshmeesha, T. R. et al. (2019). Fusarium oxysporum f. sp. Lycopersici causal agent of vascular wilt disease of tomato: Biology to diversity–A review. Saudi Journal of Biological Sciences, 26(7), 1315–1324. DOI 10.1016/j.sjbs.2019.06.002. [Google Scholar] [CrossRef]
17. Jaber, L. R., Ownley, B. H. (2017). Can we use entomopathogenic fungi as endophytes for dual biological control of insect pests and plant pathogens? Biological Control, 107(10), 50–59. DOI 10.1016/j.biocontrol.2017.01.013. [Google Scholar] [CrossRef]
18. Prabhukarthikeyan, R., Saravanakumar, D., Raguchander, T. (2014). Combination of endophytic Bacillus and Beauveria for the management of Fusarium wilt and fruit borer in tomato. Pest Management Science, 70(11), 1742–1750. DOI 10.1002/ps.3719. [Google Scholar] [CrossRef]
19. Jaber, L. R. (2018). Seed inoculation with endophytic fungal entomopathogens promotes plant growth and reduces crown and root rot (CRR) caused by Fusarium culmorum in wheat. Planta, 248(6), 1525–1535. DOI 10.1007/s00425-018-2991-x. [Google Scholar] [CrossRef]
20. Barra, B. L., France, I. A., Gerding, G. M., Silva, A. G., Carrasco, F. J. et al. (2020). Antifungal activity of Beauveria bassiana endophyte against Botrytis cinerea in two solanaceae crops. Microorganisms, 8(1), 1–15. DOI 10.3390/microorganisms8010065. [Google Scholar] [CrossRef]
21. de la Noval, B. M., Pérez, E., Olalde, V., Délano, J. P., Martínez, N. (2004). Inducción de β-1,3-glucanasas y quitinasas en plántulas de S. lycopersicum L. por hongos micorrízicos arbusculares y sistemina. Cultivos Tropicales, 25(1), 5–12. https://www.redalyc.org/articulo.oa?id=193230179001. [Google Scholar]
22. Steiner, A. A. (1961). A universal method for preparing nutrient solutions of a specific desired composition. Plant and Soil, 15(2), 134–154. DOI 10.1007/BF01347224. [Google Scholar] [CrossRef]
23. Akanmu, A. O., Odebode, A. C., Abiala, M. A., Aiyelaagbe, O. O., Olaoluwa, O. O. (2014). Inhibition of Fusarium pathogens in millet by extracts of Jatropha Curcas and Mangifera Indica. International Journal of Plant Biology and Research, 2(1), 1–8. https://www.jscimedcentral.com/PlantBiology/plantbiology-2-1007.pdf. [Google Scholar]
24. Parsa, S., Ortiz, V., Vega, F. E. (2013). Establishing fungal entomopathogens as endophytes: Towards endophytic biological control. Journal of Visualized Experiments, 74(74), 1–5. DOI 10.3791/50360. [Google Scholar] [CrossRef]
25. González, B. M. L., Fortis, M. H., Preciado, P. R., Segura, M. A. C., Salazar, S. E. et al. (2016). Calidad fitoquímica de S. lycopersicum L. Saladette producido con sustratos orgánicos bajo condiciones de invernadero. Revista Internacional de Botánica Experimental, 85, 71–78. http://www.revistaphyton.fund-romuloraggio.org.ar/vol85/Gonzalez_B.pdf. [Google Scholar]
26. Jiménez, C., Sanabria de, A. N., Altuna, G., Alcano, M. (2011). Efecto de Trichoderma harzianum (Rifai) sobre el crecimiento de plantas de S. lycopersicum L. (Lycopersicon esculentum L.). Revista de la Facultad de Agronomía de La Universidad del Zulia, 28(1), 1–10. https://www.revfacagronluz.org.ve/PDF/enero_marzo2011/v28n1a20111101.pdf. [Google Scholar]
27. Ortega, M. L. D., Ocampo, M. J., Martínez, V. C., Pérez, S. A., Sánchez, O. J. (2013). Efecto de las giberelinas sobre el crecimiento y calidad de plántulas de tomate. Revista de Ciencias Biológicas y de la Salud, 15(3), 56–60. [Google Scholar]
28. Terry, A. E., Falcón, R. A., Ruiz, P. J., Carrillo, S. Y., Morales, M. H. (2017). Respuesta agronómica del cultivo de S. lycopersicum L. al bioproducto QuitoMax®. Cultivos Tropicales, 38(1), 147–154. http://scielo.sld.cu/scielo.php?script=sci_arttext&pid=S0258-59362017000100019&lng=es&nrm=iso&tlng=pt. [Google Scholar]
29. Bradford, M. M. (1976). A rapid and sensitive method for the quantitation of microgram quantities of protein utilizing the principle of protein-dye binding. Analytical Biochemistry, 1(2), 248–254. DOI 10.1016/0003-2697(76)90527-3. [Google Scholar] [CrossRef]
30. Boller, T., Gehri, A., Mauch, F., Vögeli, U. (1983). Chitinase in bean leaves: Induction by ethylene, purification, properties, and possible function. Planta, 157(1), 22–31. DOI 10.1007/BF00394536. [Google Scholar] [CrossRef]
31. Somogyi, M. (1945). A new reagent for the determination of sugars. Journal of Biological Chemistry, 160(1), 61–68. DOI 10.1016/s0021-9258(18)43097-9. [Google Scholar] [CrossRef]
32. Ruiz, C. M. F., Ornelas, de Jesús Ornelas-Paz, J., Olivas, O. G. I., Acosta, M. C. H., Sepúlveda, A. D. R. et al. (2018). Efecto de Trichoderma spp. Y hongos fitopatógenos sobre el crecimiento vegetal y calidad del fruto de jitomate. Revista Mexicana de Fitopatología, 36(3), 444–456. DOI 10.18781/r.mex.fit.1804-5. [Google Scholar] [CrossRef]
33. Patel, S., Saraf, M. (2017). Biocontrol efficacy of Trichoderma asperellum MSST against tomato wilting by Fusarium oxysporum f. sp. lycopersici. Archives of Phytopathology and Plant Protection, 50(5–6), 228–238. DOI 10.1080/03235408.2017.1287236. [Google Scholar] [CrossRef]
34. Ferus, P., Barta, M., Konôpková, J. (2019). Endophytic fungus Beauveria bassiana can enhance drought tolerance in red oak seedlings. Trees-Structure and Function, 33(4), 1179–1186. DOI 10.1007/s00468-019-01854-1. [Google Scholar] [CrossRef]
35. Emmanuel, O. E., Villegas Rodríguez, F., Ramírez-Tobías, H. M., Hernández-Arteaga, L. E. S., Villegas, R. F. et al. (2020). La inoculación con hongos endófitos entomopatógenos en semilla genera una respuesta fisiológica y promueve el crecimiento vegetal en plantas de chile poblano en invernadero. Nova Scientia, 12(25), 1–27. DOI 10.21640/ns.v12i25.2586. [Google Scholar] [CrossRef]
36. Raya, D. S., Sánchez, R. A. R., Segura, F. J. M., Del Campillo, M. D. C., Kuramae, E. E. et al. (2017). Entomopathogenic fungi-based mechanisms for improved Fe nutrition in sorghum plants grown on calcareous substrates. PLoS One, 12(10), 1–28. DOI 10.1371/journal.pone.0185903. [Google Scholar] [CrossRef]
37. Quesada, M. E., López, D. C., Landa, B. B. (2014). The hidden habit of the entomopathogenic fungus Beauveria bassiana: First demonstration of vertical plant transmission. PLoS One, 9(2), 8–13. DOI 10.1371/journal.pone.0089278. [Google Scholar] [CrossRef]
38. Allegrucci, N., Velazquez, M. S., Russo, M. L., Perez, E., Scorsetti, A. C. (2017). Endophytic colonization of tomato by the entomopathogenic fungus Beauveria bassiana: The use of different inoculation techniques and their effects on the tomato leafminer Tuta absoluta (Lepidoptera: Gelechiidae). Journal of Plant Protection Research, 57(4), 331–337. DOI 10.1515/jppr-2017-0045. [Google Scholar] [CrossRef]
39. Nishi, O., Sushida, H., Higashi, Y., Iida, Y. (2021). Epiphytic and endophytic colonization of tomato plants by the entomopathogenic fungus Beauveria bassiana strain GHA. Mycology, 12(1), 39–47. DOI 10.1080/21501203.2019.1707723. [Google Scholar] [CrossRef]
40. Qayyum, M. A., Wakil, W., Arif, M. J., Sahi, S. T., Dunlap, C. A. (2015). Infection of Helicoverpa armigera by endophytic Beauveria bassiana colonizing tomato plants. Biological Control, 90(24), 200–207. DOI 10.1016/j.biocontrol.2015.04.005. [Google Scholar] [CrossRef]
41. Qin, X., Zhao, X., Huang, S., Deng, J., Li, X. et al. (2021). Pest management via endophytic colonization of tobacco seedlings by the insect fungal pathogen Beauveria bassiana. Pest Management Science, 77(4), 2007–2018. DOI 10.1002/ps.6229. [Google Scholar] [CrossRef]
42. Mazen, A., Hussen, G., El, G. H., Omar, M. (2020). Determination of specific growth parameters of cotton (Gossypium barbadense L.) duo to Mealy Bug, Phenacoccus solenopsis (Tinsley) infection and endophytic, Beauveria bassiana treatments. Journal of Environmental Studies, 22, 13–20. http://www.jes.sohag.edu.eg/Content/JES/JES-2020-SEP225_8090.pdf. [Google Scholar]
43. Wei, Q. Y., Li, Y. Y., Xu, C., Wu, Y. X., Zhang, Y. R. et al. (2020). Endophytic colonization by Beauveria bassiana increases the resistance of tomatoes against Bemisia tabaci. Arthropod-Plant Interactions, 14(3), 289–300. DOI 10.1007/s11829-020-09746-9. [Google Scholar] [CrossRef]
44. Silva, A. C. L., Silva, G. A., Abib, P. H. N., Carolino, A. T., Samuels, R. I. (2020). Endophytic colonization of tomato plants by the entomopathogenic fungus Beauveria bassiana for controlling the South American tomato pinworm, Tuta absoluta. CABI Agriculture and Bioscience, 1(1), 1–9. DOI 10.1186/s43170-020-00002-x. [Google Scholar] [CrossRef]
45. Mantzoukas, S., Lagogiannis, I., Mpousia, D., Ntoukas, A., Karmakolia, K. et al. (2021). Beauveria bassiana endophytic strain as plant growth promoter: The case of the grapevine Vitis vinifera. Journal of Fungi, 7(2), 1–14. DOI 10.3390/jof7020142. [Google Scholar] [CrossRef]
46. Sánchez, R. A. R., Raya Díaz, S., Zamarreño, Á. M., García, M. J. M., del Campillo, M. C. et al. (2018). An endophytic Beauveria bassiana strain increases spike production in bread and durum wheat plants and effectively controls cotton leafworm (Spodoptera littoralis) larvae. Biological Control, 116, 90–102. DOI 10.1016/j.biocontrol.2017.01.012. [Google Scholar] [CrossRef]
47. Ownley, B. H., Griffin, M. R., Klingeman, W. E., Gwinn, K. D., Moulton, J. K. et al. (2008). Beauveria bassiana: Endophytic colonization and plant disease control. Journal of Invertebrate Pathology, 98(3), 267–270. DOI 10.1016/j.jip.2008.01.010. [Google Scholar] [CrossRef]
48. Vargas, I. L., Fuenmayor, A. Y., Luzardo, M. M., da Costa, J. M., Vera, A. et al. (2018). Use of different Trichoderma species in cherry type tomatoes (Solanum lycopersicun L.) Against Fusarium oxysporum wilt in tropical greenhouses. Agronomía Costarricense, 43(1), 85–100. DOI 10.15517/rac.v43i1.35671. [Google Scholar] [CrossRef]
49. Raad, M., Glare, T. R., Brochero, H. L., Müller, C., Rostás, M. (2019). Transcriptional reprogramming Arabidopsis thaliana defense pathways by the entomopathogen Beauveria bassiana correlates with resistance against a fungal pathogen but not against insects. Frontiers in Microbiology, 10, 1366. DOI 10.3389/fmicb.2019.00615. [Google Scholar] [CrossRef]
50. Pieterse, M. J. C., van Wees, S. C. M. (2015). Induced disease resistance. In: Lugtenberg, B. (Ed.Principles of plant-microbe interactions: Microbes for sustainable agriculture, pp. 1–448. Cham: Springer. DOI 10.1007/978-3-319-08575-3) [Google Scholar] [CrossRef]
51. Garrido, J. I., Resquín, R. G., Amarilla, S. P., Ríos, M. A., Carrasco, L. et al. (2017). Transient endophytic colonization of melon plants by entomopathogenic fungi after foliar application for the control of Bemisia tabaci Gennadius (Hemiptera: Aleyrodidae). Journal of Pest Science, 90(1), 319–330. DOI 10.1007/s10340-016-0767-2. [Google Scholar] [CrossRef]
52. Arenas, O. R., Olguín, J. F. L., Ramón, D. J., Sangerman, J. D. M., Lezama, C. P. et al. (2018). Biological control of Fusarium oxysporum in tomato seedling production with mexican strains of Trichoderma. In: En Fusarium-plant diseases, pathogen diversity, genetic diversity, resistance and molecular markers, pp. 155–168. Tulin Askun: IntechOpen. DOI 10.5772/intechopen.72878 [Google Scholar] [CrossRef]
53. Bamisile, B. S., Dash, C. K., Akutse, K. S., Keppanan, R., Afolabi, O. G. et al. (2018). Prospects of endophytic fungal entomopathogens as biocontrol and plant growth-promoting agents: An insight on how artificial inoculation methods affect endophytic colonization of host plants. Microbiological Research, 217, 34–50. DOI 10.1016/j.micres.2018.08.016. [Google Scholar] [CrossRef]
54. Dash, C. K., Bamisile, B. S., Ravindran, K., Qasim, M., Lin, Y. et al. (2018). Endophytic entomopathogenic fungi enhance the growth of Phaseolus vulgaris L. (Fabaceae) and negatively affect the development and reproduction of Tetranychus urticae koch (Acari: Tetranychidae). Microbial Pathogenesis, 125(1), 385–392. DOI 10.1016/j.micpath.2018.09.044. [Google Scholar] [CrossRef]
55. Afandhi, A., Widjayanti, T., Emi, A. A. L., Tarno, H., Afiyanti, M. et al. (2019). Endophytic fungi Beauveria bassiana Balsamo accelerates growth of common bean (Phaseolus vulgaris L.). Chemical and Biological Technologies in Agriculture, 6(1), 1–6. DOI 10.1186/s40538-019-0148-1. [Google Scholar] [CrossRef]
56. Sánchez, R. A. R., Del Campillo, M. C., Quesada, M. E. (2015). Beauveria bassiana: An entomopathogenic fungus alleviates Fe chlorosis symptoms in plants grown on calcareous substrates. Scientia Horticulturae, 197(270), 193–202. DOI 10.1016/j.scienta.2015.09.029. [Google Scholar] [CrossRef]
57. Dara, S. K., Dara, S. S. R., Dara, S. S. (2017). Impact of entomopathogenic fungi on the growth, development, and health of cabbage growing under water stress. American Journal of Plant Sciences, 8(6), 1224–1233. DOI 10.4236/ajps.2017.86081. [Google Scholar] [CrossRef]
58. Karthik, N., Akanksha, K., Binod, P., Pandey, A. (2014). Production, purification and properties of fungal chitinases—A review. Indian Journal of Experimental Biology, 52(11), 1025–1035. http://nopr.niscair.res.in/bitstream/123456789/29743/1/IJEB%2052%2811%29%201025-1035.pdf. [Google Scholar]
59. Fesel, P. H., Zuccaro, A. (2016). β-glucan: Crucial component of the fungal cell wall and elusive MAMP in plants. Fungal Genetics and Biology, 90(7), 53–60. DOI 10.1016/j.fgb.2015.12.004. [Google Scholar] [CrossRef]
60. Pontón, J. (2008). La pared celular de los hongos y el mecanismo de acción de la anidulafungina. Revista Iberoamericana de Micología, 25(2), 78–82. DOI 10.1016/s1130-1406(08)70024-x. [Google Scholar] [CrossRef]
61. Schoffelmeer, E. A. M., Klis, F. M., Sietsma, J. H., Cornelissen, B. J. C. (1999). Biochemical aspects of the cell wall of Fusarium oxysporum. Fungal Genetics and Biology, 282(2), 275–282. DOI 10.1006/fgbi.1999.1153. [Google Scholar] [CrossRef]
62. Huang, H. P., Jing, X. H., Wu, L. Y., Huang, J. S. (2017). Chitin elicitor receptor kinase 1 (CERK1) is required for the non-host defense response of Arabidopsis to Fusarium oxysporum f. sp. cubense. European Journal of Plant Pathology, 147(3), 571–578. DOI 10.1007/s10658-016-1026-3. [Google Scholar] [CrossRef]
63. Geoghegan, I., Steinberg, G., Gurr, S. (2017). The role of the fungal cell wall in the infection of plants. Trends in Microbiology, 25(12), 957–967. DOI 10.1016/j.tim.2017.05.015. [Google Scholar] [CrossRef]
64. Acuña, P. R., Quiroz, F. D., Laynes, Z. P., Gutierrez, R. A. (2017). Hypothenemus hampei (Coleoptera: Curculionidae) induce in vitro laminarinasa y quitinasa de Beauveria bassiana (Hypocreales: Clavicipitaceae). Revista Colombiana de Entomología, 43(1), 7–13. http://www.scielo.org.co/pdf/rcen/v43n1/0120-0488-rcen-43-01-00007.pdf. [Google Scholar]
65. Ownley, B. H., Gwinn, K. D., Vega, F. E. (2010). Endophytic fungal entomopathogens with activity against plant pathogens: Ecology and evolution. BioControl, 55(1), 113–128. DOI 10.1007/s10526-009-9241-x. [Google Scholar] [CrossRef]
66. Paredes, M., Nolasco, O., Acuña, R., Gutiérrez, A. I. F. (2016). Expresión de genes quitinasas de Beauveria bassiana frente a sustratos de Fusarium oxysporum y Peronospora variabilis. Scientia Agropecuaria, 7(3), 239–244. DOI 10.17268/sci.agropecu.2016.03.11. [Google Scholar] [CrossRef]
67. Azadi, N., Shirzad, A., Mohammadi, H. (2016). A study of some biocontrol mechanisms of Beauveria bassiana against Rhizoctonia disease on tomato. Acta Biologica Szegediensis, 60(2), 119–127. http://abs.bibl.u-szeged.hu/index.php/abs/article/view/2898/2890. [Google Scholar]
68. Wojtasik, W., Boba, A., Preisner, M., Kostyn, K., Szopa, J. et al. (2019). DNA methylation profile of β-1,3-glucanase and chitinase genes in flax shows specificity towards Fusarium oxysporum strains differing in pathogenicity. Microorganisms, 7(12), 1–19. DOI 10.3390/microorganisms7120589. [Google Scholar] [CrossRef]
69. Bartholomew, E. S., Black, K., Feng, Z., Liu, W., Shan, N. et al. (2019). Comprehensive analysis of the chitinase gene family in cucumber (Cucumis sativus L.From gene identification and evolution to expression in response to Fusarium oxysporum. International Journal of Molecular Sciences, 20(21), 5309. DOI 10.3390/ijms20215309. [Google Scholar] [CrossRef]
70. Fang, W. G., Bo, L., Xiao, Y. H., Jin, K., Ma, J. C. et al. (2005). Cloning of Beauveria bassiana chitinase gene Bbchit1 and its application to improve fungal strain virulence. Applied and Environmental Microbiology, 71(1), 363–370. DOI 10.1128/AEM.71.1.363-370.2005. [Google Scholar] [CrossRef]
71. Zhang, F., Chen, C., Zhang, F., Gao, L., Liu, J. et al. (2017). Trichoderma harzianum containing 1-aminocyclopropane-1-carboxylate deaminase and chitinase improved growth and the diminished adverse effect caused by Fusarium oxysporum soybean. Journal of Plant Physiology, 210, 84–94. DOI 10.1016/j.jplph.2016.10.012. [Google Scholar] [CrossRef]
72. Xiao, G., Ying, S. H., Zheng, P., Wang, Z. L., Zhang, S. et al. (2012). Genomic perspectives on the evolution of fungal entomopathogenicity in Beauveria bassiana. Scientific Reports, 2(1), 1–10. DOI 10.1038/srep00483. [Google Scholar] [CrossRef]
73. Aamir, M., Kashyap, S. P., Zehra, A., Dubey, M. K., Singh, V. K. et al. (2019). Trichoderma erinaceum bio-priming modulates the WRKYs defense programming in tomato against the Fusarium oxysporum f. sp. lycopersici (Fol) challenged condition. Frontiers in Plant Science, 10. DOI 10.3389/fpls.2019.00911. [Google Scholar] [CrossRef]
74. Cucu, M. A., Gilardi, G., Pugliese, M., Gullino, M. L., Garibaldi, A. (2020). An assessment of the modulation of the population dynamics of pathogenic Fusarium oxysporum f. sp. lycopersici in the tomato rhizosphere by means of the application of Bacillus subtilis QST 713, Trichoderma sp. TW2 and two composts. Biological Control, 142(4), 104158. DOI 10.1016/j.biocontrol.2019.104158. [Google Scholar] [CrossRef]
75. Dionisio, G., Kryger, P., Steenberg, T. (2016). Label-free differential proteomics and quantification of exoenzymes from isolates of the entomopathogenic fungus Beauveria bassiana. Insects, 7(4), 54. DOI 10.3390/insects7040054. [Google Scholar] [CrossRef]
76. de la Torre, H. M. E., Sánchez, R. D., Galeana, S. E., Plasencia, de la P. J. (2014). Fumonisinas-síntesis y función en la interacción Fusarium verticillioides-maíz. TIP Revista Especializada en Ciencias Químico-Biológicas, 17(1), 77–91. DOI 10.1016/S1405-888X(14)70321-3. [Google Scholar] [CrossRef]
77. Montoya, E. W. J., Nolasco, C. O. P., Acuña, P. R. K., Gutiérrez, A. I. F. (2016). Expresión de β-1,3-Glucanasas de Beauveria bassiana en cultivo con extracto de los fitopatógenos Peronospora variabilis y Fusarium oxysporum. Scientia Agropecuaria, 7(3), 253–257. DOI 10.17268/sci.agropecu.2016.03.13. [Google Scholar] [CrossRef]
78. Jangir, M., Pathak, R., Sharma, S., Sharma, S. (2018). Biocontrol mechanisms of Bacillus sp., isolated from tomato rhizosphere, against Fusarium oxysporum f. sp. lycopersici. Biological Control, 123, 60–70. DOI 10.1016/j.biocontrol.2018.04.018. [Google Scholar] [CrossRef]
79. Manikandan, R., Raguchander, T. (2014). Fusarium oxysporum f. sp. Lycopersici retardation through induction of defensive response in tomato plants using a liquid formulation of Pseudomonas fluorescens (Pf1). European Journal of Plant Pathology, 140(3), 469–480. DOI 10.1007/s10658-014-0481-y. [Google Scholar] [CrossRef]
80. Mohamed, M. A., Shousha, W. G., Mahdy, E. S. M., Ghazy, A. E. M., Mohamed, M. M. (2007). Biochemical alterations induced in tomato in response to Fusarium oxysporum infection: Purification and characterization of an acidic β-1,3-glucanase. Research Journal of Agriculture and Biological Sciences, 3(6), 939–949. http://www.aensiweb.net/AENSIWEB/rjabs/rjabs/2007/939-949.pdf. [Google Scholar]
81. Singh, D., Ambroise, A., Haicour, R., Sihachakr, D., Rajam, M. V. (2014). Increased resistance to fungal wilts in transgenic eggplant expressing alfalfa glucanase gene. Physiology and Molecular Biology of Plants, 20(2), 143–150. DOI 10.1007/s12298-014-0225-7. [Google Scholar] [CrossRef]
82. Solanki, M. K., Singh, N., Singh, R. K., Singh, P., Srivastava, A. K. et al. (2011). Plant defense activation and management of tomato root rot by a chitin-fortified Trichoderma/Hypocrea formulation. Phytoparasitica, 39(5), 471–481. DOI 10.1007/s12600-011-0188-y. [Google Scholar] [CrossRef]
83. Shanmugam, V., Kanoujia, N. (2011). Biological management of vascular wilt of tomato caused by Fusarium oxysporum f. sp. lycospersici by plant growth-promoting rhizobacterial mixture. Biological Control, 57(2), 85–93. DOI 10.1016/j.biocontrol.2011.02.001. [Google Scholar] [CrossRef]
84. Jain, S., Vaishnav, A., Kumari, S., Varma, A., Tuteja, N. et al. (2017). Chitinolytic Bacillus-mediated induction of jasmonic acid and defense-related proteins in soybean (Glycine max L. Merrill) plant against Rhizoctonia solani and Fusarium oxysporum. Journal of Plant Growth Regulation, 36(1), 200–214. DOI 10.1007/s00344-016-9630-1. [Google Scholar] [CrossRef]
Cite This Article
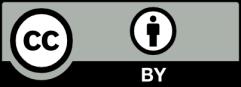