Open Access
ARTICLE
Drought Tolerance in Mung Bean is Associated with the Genotypic Divergence, Regulation of Proline, Photosynthetic Pigment and Water Relation
1
Agronomy Division, Regional Agricultural Research Station, Bangladesh Agricultural Research Institute (BARI), Pabna, 6600,
Bangladesh
2
Chief Research Scientist, New Edge Microbials, Ltd., Albury, 2641, Australia
3
Horticulture Research Centre, Regional Agricultural Research Station, Bangladesh Agricultural Research Institute (BARI), Pabna,
6600, Bangladesh
4
Pulses Research Centre, BARI, Pabna, 6600, Bangladesh
5
Agronomy Division, Bangladesh Wheat and Maize Research Institute, Dinajpur, 5200, Bangladesh
6
Department of Agronomy, Hajee Mohammad Danesh Science and Technology University, Dinajpur, 5200, Bangladesh
7
Department of Plant Production, College of Food and Agriculture, King Saud University, Riyadh, 11451, Saudi Arabia
8
Department of Agronomy, Faculty of Agriculture, Kafrelsheikh University, Kafrelsheikh, 33156, Egypt
9
Grassland and Forage Division, National Institute of Animal Science, Rural Development Administration, Cheonan, 31000, Korea
10 Department of Agronomy, Faculty of Agriculture, University of Poonch Rawalakot, Rawalakot, 12350, Pakistan
11 Department of Genetics and Plant Breeding, Faculty of Agriculture, Bangladesh Agricultural University, Mymensingh, 2202,
Bangladesh
* Corresponding Authors: Mohammad Sohidul Islam. Email: ; Ayman EL Sabagh. Email:
Phyton-International Journal of Experimental Botany 2023, 92(3), 955-981. https://doi.org/10.32604/phyton.2023.025138
Received 23 June 2022; Accepted 07 October 2022; Issue published 29 November 2022
Abstract
Drought is one of the critical conditions for the growth and productivity of many crops including mung bean (Vigna radiata L. Wilczek). Screening of genotypes for variations is one of the suitable strategies for evaluating crop adaptability and global food security. In this context, the study investigated the physiological and biochemical responses of four drought tolerant (BARI Mung-8, BMX-08010-2, BMX-010015, BMX-08009-7), and four drought sensitive (BARI Mung-1, BARI Mung-3, BU Mung-4, BMX-05001) mung bean genotypes under wellwatered (WW) and water deficit (WD) conditions. The WW treatment maintained sufficient soil moisture (22% ± 0.5%, i.e., 30% deficit of available water) by regularly supplying water. Whereas, the WD treatment was maintained throughout the growing period, and water was applied when the wilting symptom appeared. The drought tolerant (DT) genotypes BARI Mung-8, BMX-08010-2, BMX-010015, BMX-08009-7 showed a high level of proline accumulation (2.52–5.99 mg g−1 FW), photosynthetic pigment (total chlorophyll 2.96–3.27 mg g−1 FW at flowering stage, and 1.62–2.38 mg g−1 FW at pod developing stage), plant water relation attributes including relative water content (RWC) (82%–84%), water retention capacity (WRC) (12–14) as well as lower water saturation deficit (WSD) (19%–23%), and water uptake capacity (WUC) (2.58–2.89) under WD condition, which provided consequently higher relative seed yield. These indicate that the tolerant genotypes gained better physiobiochemical attributes and adaptability in response to drought conditions. Furthermore, the genotype BMX- 08010-2 showed superiority in terms of those physio-biochemical traits, susceptibility index (SSI) and stress tolerance index (STI) to other genotypes. Based on the physiological and biochemical responses, the BMX-08010-2 was found to be a suitable genotype for sustaining yield under drought stress, and subsequently, it could be recommended for crop improvement through hybridization programs. In addition, the identified traits can be used as markers to identify tolerant genotypes for drought-prone areas.Keywords
Drought stress is an important constraint that limits growth, development, yield, and productivity in plants globally [1–4]. It has been recognized as one of the limiting factors for successful crop production in dry land [5], which induces a series of physiological and molecular alterations. In physiological processes, like plant photosynthesis, stomatal conductivity, and transpiration are reduced due to drought-induced reduction of CO2 accumulation in leaves [1,2].
Mung bean [Vigna radiata (L.) Wilczek; Fabaceae], an important pulse crop is a vital source of the daily human diet in South Asian countries [6,7]. It is a good source of digestible dietary protein [7], and radically contributes to the soil by fixing environmental N2 [8]. Mung bean is generally cultivated during the dry season (March–May), while the scarcity of water or unavailable soil moisture is a common incidence in Bangladesh. Nonetheless, it has been reported that the growth, development and yield of mung bean are severely affected by water deficit stress at the early, late vegetative and flowering stages, whereas the grain yield is found to be reduced by 25%, 39% and 59%, respectively [9]. In addition, the response of plants to drought depends on the intensity and duration of stress as well as the plant species. Thus, it is necessary to screen out drought tolerant mung bean genotypes for sustaining potential yield under drought condition.
Drought tolerance can be evaluated through bio-physiological parameters, such as metabolic compatible solutes (e.g., proline, soluble sugar), chlorophyll content, and plant water relations under drought condition [10,11]. The accumulation of proline is highly responsive in various plant species under water deficit condition [12–14]. Mung bean plants show higher accumulation of free proline and sugar under drought in field experiments [15]. Soil moisture has also been reported to affect plant water potential, and chlorophyll content which is the most important factor for grain development [16]. Moisture stress enhances changes in the internal environment within the crops, consequently affecting the physiological and biochemical processes of crop plants [17]. The shortage of water, i.e., drought, during the crop growing period inhibits the chlorophyll a (Chl a) & chlorophyll b (Chl b) synthesis, and decreases the quantity of Chl a & Chl b binding proteins, ultimately reducing the light-harvesting pigment protein which is linked with photosystem II [18]. Moreover, under this condition, the concentration of solutes in the soil increases, which reduces the access of the roots, thereby decreasing water reception by the roots, and consequently declining the leaf water potential [19].
Drought stress can be mitigated through different management practices, like supplemental irrigation, water conservation practices, i.e., mulching, use of plant growth regulators (PGRs), and a diversified farming system [20,21]. However, they have cost involvement and are sometimes quite impossible for the socio-economic conditions of the farmers. So, the absolute alleviation of drought is not possible. The best option is, thus, to select drought resistance cultivar(s) for sustainable crop production in the moisture deficit stress. Therefore, this study was conducted to explore physiological and biochemical traits that are associated with the drought tolerance of mung bean.
2.1 Experimental Site and Test Material
A trial was conducted at the research field of the Agronomy Department, Hajee Mohammad Danesh Science and Technology University (HSTU), Dinajpur, Bangladesh (The geographical location of the area constitutes 25°38′ N latitude and 88°41′ E longitude, with an elevation of 38.20 m above sea level) during the Kharif (summer) season under the rain-out shelter, which belongs to the Old Himalayan Piedmont Plain that is designated as Agro-Ecological Zone-1. The rain-out shelter was constructed by using a transparent polythene sheet. Four drought tolerant and four drought susceptible mung bean genotypes were used in this study, which were chosen under a laboratory screening based on their germination indices, seedling growth characteristics and their relative performance with PEG induced water deficit stress [22]. The tested seeds were collected from Pulses Research Centre, Bangladesh Agricultural Research Institute (BARI), and Bangabandhu Sheikh Mujibur Rahman Agricultural University (BSMRAU), Bangladesh. The pedigree and distinction of all genotypes of mung bean under the study are presented in Table 1.
2.2 Experimental Design and Treatments
The study was conducted with two factors consisting of eight mung bean genotypes (four drought susceptible and four tolerant ones) (as described in Table 1), and two moisture conditions (well-watered and water deficit stress). Total 16 treatments were arranged in a Completely Randomized Design (CRD) with four replications.
2.3 Pot Preparation and Seed Sowing
The plastic pots of size 23 cm diameter (inside) and 23 cm height with a 17 cm base (8 L volume) were used in the present study. Each plastic pot was prepared with 10 kg well-pulverized air-dried soil including compost (1/4th of the soil mass). The soil was collected from the research field of the Agronomy Department (HSTU), Dinajpur, Bangladesh. It was of sandy loam type with 25.8% field capacity, 1.49 g/cc bulk density, and 11.6% permanent wilting point. The physical and chemical properties of the experimental soil have been mentioned in Table 2. The pots were supplied with fertilizers as 0.103, 0.088, 0.093, 0.046 and 0.007 g of urea, triple superphosphate, and boric acid corresponding to 20-17-18-10-2 kg Nitrogen (N), Phosphorus (P), Potassium (K), Sulphur (S) and Boron (B) per hectare, respectively [23]. Twenty seeds were sown in each pot after sterilizing with 0.1% mercuric chloride solution for 2 min [24–26], and subsequently washing with tap water. The seeding depth was maintained at 3 cm. After emergence and establishment, five homogenous and healthy seedlings were kept in each pot to grow up to maturity.
Primarily water was applied in all pots comprising of both treatments of well-watered (WW) and water deficit (WD) just after sowing of seeds, and subsequently water was applied at 5 days after sowing (DAS) for proper germination and seedling establishment. From 10 DAS, the WW treatment pots maintained the most favorable moisture for optimum plant growth and development throughout the growing period providing irrigation, which remained around 20% of the moisture content (MC) (Fig. 1a). As it, soil moisture was maintained at 22% ± 0.5%, i.e., 30% deficit of available water. The WD treatment pots were maintained at moisture deficit condition throughout the growing period, and irrigation was applied when the appearance of wilting symptom was shown (Fig. 1b). At wilting symptoms, the MC has prevailed about 50% of field capacity. However, water was applied in both treatments by frequent observation of soil MC through digital soil moisture metter (Model PMS-714), and water was applied up to the field capacity (FC) level in each watering. The net amount of water (d) required per irrigation was calculated according to [27] as follows:
where, FC = Field capacity of the soil (%)
MC = Moisture content of the soil at the time of irrigation (%)
p = Bulk density of the soil (g cm−3)
D = Root zone depth (cm)
Figure 1: Changes of soil moisture content under WW and WD conditions. MC = Moisture content; FC = Field capacity; PWP = Permanent wilting point
2.5 Crop Management and Harvest
Weeding was done as and when necessary to keep the pot reasonably weeds-free throughout the growing period. The plants were protected from flower thrips by spraying with Imidachloprid (Imitaf 20 SL) @ 0.5 ml litre−1 of water at the flowering and the pod developing stage. For controlling pod borer, Lambda-Cyhalothrin (Karate 2.5 EC/Reeva 2.5 EC) @ 1 ml litre−1 of water was applied two times maintaining 7 days intervals from pod developing stage. Harvesting was done when the pods of mung bean genotypes had turned blackish-brown in color, and dried to such a point that they were about to shatter. The matured pods were collected thrice manually. After harvesting, the samples were sun-dried for three days, and then threshing was carried out. The threshed seeds were cleaned, sun-dried, and weighed per plant (g).
2.6 Measurement of Physiological Traits
Fresh third trifoliate leaf samples from all genotypes of mung bean in each replication were used for assessments of proline content to minimize the error of results. It was collected from 0.5 g fresh weight (FW) of leaf samples that were homogenized with 3% sulfosalicylic acid, and acid ninhydrin reagent method was used to estimate the proline content [28]. The optical density of the solutions (sample and standard solution) was estimated through an Ultra Violet (UV)-visible spectrophotometer (Model T60 UV, Japan) at 520 nm wave length. The proline content was calculated from a standard curve, and stated as mg proline g−1 FW.
For getting uniform results in each treatment, the third trifoliate leaf was used to determine the chlorophyll content by the method described by Witham et al. [29]. Exactly 0.1 g of fresh leaf tissues of mung bean leaf were taken in a test tube containing 10 mL of 80% acetone. The sample was then shaken overnight by using an electric horizontal shaker. For measuring chlorophyll a (Chl a), chlorophyll b (Chl b) and total chlorophyll content, the optical density or absorbance of the supernatant were recorded through an UV-visible spectrophotometer at 663 and 645 nm wavelength. The concentration of Chl a, Chl b and total chlorophyll were measured using the following formula:
where, D = Absorbance reading of the chlorophyll extract at the specific wavelength
V = Final volume of the 80% acetone-chlorophyll extract
W = Fresh weight in gram of the tissue extracted
Chlorophyll stability index (CSI) was estimated according the formulae given by [30]
The terminal leaflets of the fully expanded leaves for each treatment were collected during noon time. Immediately, the FW of the collected leaves was measured. The leaf samples were soaked in distilled water and kept in dark condition for 24 h at ambient room temperature for 24 h. The leaf samples were weighed after removing excess water from the leaf surface through gently wiping them with a paper towel, which is called turgid weight (TW). The dry weights (DW) of the leaf samples were taken after drying the samples in the oven at 80°C for 72 h. The estimated fresh, turgid and dry weights of the leaves were used to calculate relative water content [31], water saturation deficit [32], water retention capacity [33], and water uptake capacity [34]. The formula of all the parameters was presented below:
The xylem exudation rate (XER) was measured at 5 cm above the stem base of the plant at 7:00 am. For measuring XER, clean cotton dry weight was taken. The stem was made a slanting cut with a sharp knife, and the weighted cotton was placed on the cut surface. The oozing sap was collected from the stem after one hour at normal temperature. To prevent evaporation, the cotton was covered with a cellophane bag. Thereafter, cotton with sap weight was taken. The calculation of XER was done by the following formula:
Drought tolerance indices like relative performance [35], stress susceptibility index [36], tolerance [37], and stress tolerance index [38] were measured based on the values obtained under water deficit and control conditions. The formulas of tolerance indices have been given in below:
where, Y = mean grain yield of a genotype under a stress environment; Yp = mean yield of the same genotype under a stress-free environment; X = mean Y of all genotypes; Xp = mean Yp of all genotypes.
where,
Yp = The yield of a particular genotype under control condition,
Ys = The yield of a particular genotype under stress condition.
The data were compiled and subjected to statistical analysis with the help of a computer-based statistical program using the ‘R’ platform [39] following the basic procedure outlined by [40]. Correlation analysis was performed using the ‘Agricolae’ package [41]. The mean separation test was done with the least significant difference (LSD) test at p = 0.05.
From variance analysis, it was revealed that the studied genotypes (at different stress levels) and stress levels (at different genotypes) were significant for all the studied variables (Supplementary Tables 1 and 2). The interaction effects (genotypes x stress levels) were also significant for all the measured variables (Tables 3–5; Figs. 2, 4 and 5).
Figure 2: Proline content (mg g−1 FW) at flowering stage (45 days after sowing) of selected mung bean genotypes under water deficit and well-watered (control) conditions
Figure 3: Chlorophyll stability index under two growth stages of selected mung bean genotypes under water deficit and well-watered conditions (Error bars symbolize standard error (±) which fit within the line symbol if not displayed)
Figure 4: Xylem exudation rate (45 DAS) of selected mung bean genotypes under well-watered (control) and water deficit conditions
Figure 5: Seed yield (g plant−1) of mung bean genotypes under well water (control) and water deficit conditions
There was a distinct variation observed in proline accumulation under WD and WW (control) conditions. The studied genotypes exhibited different magnitudes of proline accumulation both under WW and WD conditions (Fig. 2). The proline accumulation increased in all the tested genotypes with WD condition than those of WW condition. This is due to the diminishing in the internal water status of the plant, which could be evident from the reduction in leaf water potential. Alternatively, the effect was recorded with sufficient moisture ensured by WW at all stages. However, the genotype BMX-08010-2 showed a significantly superior response to both WW and WD conditions for giving the highest proline accumulation. While the least value of proline accumulation was recorded in BARI Mung-1 genotype under the WW and WD stress conditions. In addition, BMX-08010-2 genotype gave about 1 fold higher proline accumulation than BMX-08009-7; 2 fold higher than BARI Mung-8 and BMX-01015; 4 fold higher than BARI Mung-3, BU Mung-4, BMX-05001 and 5 fold higher than BARI Mung-1 genotypes under WD condition. This means that the genotype BMX-08010-2 is more capable to sustain under WD condition than the other genotypes.
The results revealed that all the mung bean genotypes exhibited a substantial variation in the leaf chlorophyll content under WD and WW conditions (Table 3). However, it seemed that Chl a content reduced to 11.48%–30.06% over control (WW) at flowering stages. Among the genotypes, the BMX-08010-2 contained the maximum Chl a content both in control and WD conditions. This genotype also showed the minimum reduction of Chl a content (11.48%) under WD condition over WW. The variety BARI Mung-1 showed the minimum Chl a content under WW and WD condition with the highest reductions of 30.06% over control.
Similarly, Chl b content in different genotypes decreased at WD condition. The data displayed a significant effect among the tested mung bean genotypes under stress condition in terms of Chl b content. However, numerically the highest Chl b content was obtained in BMX-08010-2 under WD condition, whereas the minimum value was recorded in BARI Mung-1 (Table 3). The genotype BMX-08010-2 grown under WW condition produced the highest Chl b, and BARI Mung-1 showed the lowest values. On the other side, the lowest diminishing in Chl b (16.10%) was recorded in BMX-08010-2 under WD condition, while the highest reduction (35.00%) was documented in BARI Mung-1. Moreover, it was also noticed that a higher reduction of the Chl b content over the Chl a was recorded under WD condition.
The chlorophyll a/b ratio showed a considerable dissimilarity among the genotypes of both WW and WD conditions (Table 3). The mung bean genotype BARI Mung-1 showed the highest chlorophyll a/b ratio under control condition. The genotype BMX-08010-2 exhibited the lowest values of chlorophyll a/b ratio. However, a similar observation was also found under WD condition. The increment of chlorophyll a/b ratio with WD stress might be imposed to lower deterioration of Chl a than Chl b under such stress.
The drought stress (DS) reduced higher total chlorophyll content among the entire genotypes. The total chlorophyll content ranged from 2.43 to 3.27 mg g−1 FW under WW, and from 1.67 to 2.83 mg g−1 FW under WD conditions (Table 3). However, the highest reduction (31.28%) with minimum total chlorophyll content under control and WD condition was documented in the genotype BARI Mung-1 which was followed by BARI Mung-3. In contrast, the genotype BMX-08010-2 showed the lowest reduction (13.46%) due to WD stress showing the highest amount of total chlorophyll content. A reduction of total chlorophyll content under WD condition implies a lowered capability for light absorption. The genotype BMX-08010-2 produced the highest values of Chl a, Chl b as well as total chlorophyll under WD condition than other tested genotypes indicating much capability to uptake water under WD condition which is directly related to producing higher yield (Table 3).
The chlorophyll stability index showed the disparity values among the mung bean genotypes (Fig. 3). However, the genotype BMX-08010-2 showed the highest CSI values (0.87). On the other hand, BARI Mung-1 gave the least value (0.69) under such stage. Furthermore, the BMX-08010-2 genotype gave about 2% higher CSI than BARI Mung-8 and BMX-08009-7, concomitantly 5%, 26%, 23%, 17% and 9% higher than BMX-01015, BARI Mung-1 BARI Mung-3, BU Mung-4, and BMX-05001 genotypes, respectively. This indicates the BMX-08010-2 showed minimum chlorophyll degradation under WD condition than the other genotypes.
Owing to genetic variation, the dissimilar results of RWC were obtained, and the RWC in the leaves of mung bean genotypes was reduced meaningfully by the WD stress (Table 4). The RWC varied from 78.58% to 84.16%, and 64.04% to 81.37% under WW and WD conditions, respectively. The highest RWC was found in the BMX-0801-2 genotype both under WW and WD conditions, whereas the lowest RWC was observed in BARI Mung-1 in the same conditions. In case of reduction percentages over control, the BMX-0801-2 showed the minimum reduction (3.32%) of RWC, whereas the maximum reduction (18.50%) was observed in BARI Mung-1. Significantly higher RWC with lower reduction in the genotype BMX-0801-2 at a specific condition of water stress pointed out that it had superior tolerance to drought than BARI Mung-1, and also indicated that the genotype maintained higher amount of water in plant cell.
A considerable variation of WSD in the leaves of mung bean genotypes under WD condition was found in this study. The values of WSD ranged from 15.84% to 21.42% under WW, and from 18.63% to 35.96% in WD conditions (Table 4). However, at both WW and WD conditions, the highest WSD was obtained in BARI Mung-1, whereas the least value was found in BMX-08010-2 genotype. At WD stress, the WSD were notably increased more irrespective of all mung bean genotypes as compared to WW condition. The results also revealed that the WSD increased due to WD condition from 17.61%–67.88% over WW, and the genotype BMX-08010-2 showed the minimum increment (17.61%), while the genotype BARI Mung-1 showed the maximum increment (67.88%). A higher WSD indicates that the plants are subjected to a greater degree of water shortage.
The maximum WUC in a plant subjected to a greater degree of water deficit. The WUC of studied mung bean genotypes was greatly influenced by WD stress, and it increased under stress condition. However, it ranged from 2.13 to 2.56 under WW, and from 2.58 to 4.73 under WD conditions (Table 4). The findings revealed that sufficient supply of water (WW) exhibited minimum values of WUC. The genotype BARI Mung-1 showed the highest WUC, while BMX-08010-2 demonstrated the least value under WW and WD conditions. The WUC increased up to 84.77% in BARI Mung-1, whereas the least (21.13%) was in BMX-08010-2 under WD condition as compared to WW condition. These results indicate that BARI Mung-1 suffered more from WD stress than that of BMX-08010-2.
The WRC showed significant variation among the genotypes under WW and WD treatments (Table 4). The decrease of WRC was higher in plants raised under WD stress condition than that in the plants raised under an adequate supply of water (control condition). The present results displayed that the WRC decreased from 8.59% to 28.61% among the studied mung bean genotypes under WD as compared to their WW condition. However, the genotype BMX-08010-2 gave the minimum relative reduction, whereas the genotype BARI Mung-1 gave the maximum relative reduction.
The XER represented the discharge rate of plant cell-sap against gravitational force through the plant xylem vessels that remained higher under WW conditions compared to WD stress (Fig. 4). The diminished value of XER indicates inferior plant water uptake under DS. The XER ranged from 20.00 to 25.53 mg h−1 in the WW plants, whereas the corresponding value for WD was 8.13 to 12.97 mg h−1. Moreover, the genotype BMX 05001 gave the highest XER in WW condition. Furthermore, under the WD stress condition, BARI Mung-1 showed the lowest exudation rate with the maximum relative reduction (66%), and BMX-08010-2 demonstrated the highest value with the minimum reduction (42%).
3.4 Yield and Yield Contributing Traits
The pods plant−1 is the function of potential yield of any pulse crops. However, the number of pods plant−1 was significantly reduced in all the studied mung bean genotypes due to WD stress (Table 5). Under WW condition, the highest number of pods plant−1 was found in BARI Mung-8 which was significantly different from other genotypes, while the lowest in BMX-08009-7. At WD stress condition, BARI Mung-8 also showed the maximum pod bearing capacity, at the same time the lowest in BMX-05001. Considering the relative value, it varied among the genotype from 0.51 to 0.76. However, the BMX-08010-2 genotype showed the highest relative value (0.76) followed by BMX-08009-7 (0.75) as compared to others, and BARI Mung-1 demonstrated the lowest relative value (0.51) followed by BARI Mung-3 (0.55).
A remarkable decrease of pod length was observed among the mung bean genotypes under WD stress (Table 5). The genotype BMX-08010-2 produced the longest pod as compared to others both under WW and WD stress. At WD condition, the BARI Mung-1 gave the shortest pod. Regarding the relative performance, the maximum relative value (0.81) was obtained in BMX-08010-2 followed by BMX-08009-7 (0.80), whereas the minimum value (0.68) was in BARI Mung-1.
The number of seeds pod−1 in the mung bean genotypes considerably varied due to water deficit condition (Table 5). In WW condition, the highest number of seeds pod−1 was observed in the genotype BMX-01015, while the least was observed in BMX-05001. On the other hand, the genotype BMX-08010-2 gave the highest number of seeds pod−1 in WD stress condition. The relative values varied from 0.61 to 0.84 among the genotypes, and the highest value was in BMX-08010-2 followed by BMX-08009-7, whereas the lowest relative value was in BARI Mung-1.
Water stress condition remarkably decreased the 100-seed weight among the mung bean genotypes inconsistently (Table 5). The maximum 100-seed weight was recorded in BMX-05001 genotype under both WW and WD conditions. Similarly, BARI Mung-8 genotype gave the minimum 100-seed weight in both conditions. But the BMX-08010-2 genotype performed top by giving the highest relative value (0.89) followed by BMX-08009-7 (0.87). The least relative value (0.65) was observed in BARI Mung-1.
As per the findings of our study, a considerable decline in seed yield was observed in all mung bean genotypes indicating that WD stress imparts a remarkable adverse effect on plant growth (Fig. 5). Under WW condition, the genotype BMX-08010-2 remained unmatched by producing the highest seed yield, whereas the genotype BMX-08010-2 produced the highest seed yield under the WD stress condition. Nevertheless, the rate of decrease over control (relative values) ranged from 0.20 to 0.65 varying with the genotypes. The highest relative value was obtained in genotype BMX-08010-2 followed by BMX-08009-7, and the lowest was in BARI Mung-1. The highest relative values in BMX-08010-2 might be contributed influentially for giving their higher relative seed yield as compared to other genotypes. The canopy, as well as the growth architectural view of the genotypes under WD condition, also indicated that the plant growth appearance of BMX-08010-2 was found superior resulting in higher relative seed yield (Fig. 6), but reverse results were recorded in BARI Mung-1. In addition, Fig. 7 presented the heatmap using hierarchical cluster analysis, and the results underlined different responses of mung bean genotypes to drought among the studied traits. The hierarchical cluster analysis was partitioned into two groups, in which tolerant genotypes were plotted from group I and susceptible genotypes were from group II. Results showed that the genotype BMX-08010-2 was the most stress-tolerant (group I), whereas BARI Mung-1 was susceptible to drought stress (group II). These results reconfirmed the relative WD stress tolerance of BMX-08010-2 among the genotypes.
Figure 6: Growth and development of mung bean genotypes under well-watered (WW) and water deficit (WD) conditions
Figure 7: Graphical display of the relationships among the eight investigated mung bean genotypes including drought tolerant and susceptible, and 18 measured traits. Here, relative values obtained from studied traits of the mung bean genotypes were normalized and clustered. Two-row clusters (cluster numbers were determined by the machine language of gap statistic) were obtained at the genotype level. The kinds of colors and color intensity were adjusted supported association among genotypes and traits. These colors are representative of a relative scale (−2 to +1.5) derived after data standardization. The darker blue indicates susceptibility, conversely the darker red indicates tolerance against water deficit stress. WSD = Water saturation deficit; WUC = Water uptake capacity; CSI = Chlorophyll stability index; PL = Pod length; NSPP = Number of seeds pod−1; XER= Xylem exudation rate; NPPP = Number of pods plant−1; WRC = Water retention capacity; Chl = Chlorophyll; Tchl = Total chlorophyll; DF = Days to flowering; DPD = Days to pod development; SY = Seed yield; RWC = Relative water content; HSW = Hundred seed weight
3.5 Assortment of Mung Bean Genotypes Based on Tolerance Indices
The results of stress tolerance indices investigated in this experiment are presented in Table 6. On point of tolerance (TOL) level, the lowest value was observed in the genotype BMX-08010-2. This result indicates that the lesser the TOL value, the lower grain yield reduction under stress conditions, and as a result inferior stress sensitivity. However, the maximum values of TOL and SSI were observed in BARI Mung-1. The highest stress tolerance index (STI) was exhibited in the genotypes BMX-08010-2 followed by BARI Mung-8, whereas the least value was in BARI Mung-1. Based on the STI values recorded in this experiment, the genotype BMX-08010-2 could be considered as relatively the most drought tolerant genotype, whereas BARI Mung-1 remained the most drought susceptible genotype.
In this study, WD stress significantly changes the physio-chemical mechanism of the mung bean plant, which directly influenced the yield attributes resulting decreased seed yield. The levels of physio-chemical traits viz., proline, chlorophylls, RWC, WSD, WUC, WRC and XER under drought stress indicate the stress tolerance of crop plants. So, observation of these traits based on their variability and diversity could be a useful tool for identifying tolerance genotypes.
In our result, the proline accumulation in WD stress was higher than in the WW condition (Fig. 1). This is because of the reduction in the internal water content of the plant under WD condition which could be a reason for decreasing in leaf water potential. Besides, the optimum supply of moisture (WW) exhibited a lower level of free proline content. Genotypes having different levels of drought resistance differ in their capacity to accumulate proline under stress. Accumulation of proline content in the leaf tissues of the BMX-08010-2 genotype was higher under both conditions due to osmoregulation in cells. It also implies that the genotype BMX-08010-2 provided better recovery during stress conditions having its higher proline accumulation indicating more tolerance capacity to the imposed drought and better potential for osmoprotectants. Under prolonged stress conditions, proline acts hard on the yield [42]. A similar result was observed in young bean [43]. These results are also in line with the finding of Ludlow and Muchow [44], who reported higher accumulated proline possibly contributed to osmotic adjustment which plays a major role in maintaining turgor over fluctuating soil water potentials. In contrast, the lowest accumulation of proline in genotype BARI Mung-1 indicates its least potential for osmoprotectants during WD stress. Proline tends to regulate osmotic adjustments, producing several osmoprotectants, scavenging free radicals and antioxidants, and protecting macromolecules from degradation and denaturation. Furthermore, it reduces water losses from the cell under water deficit conditions by regulating cytosolic acidity, and by sustaining a reserve for carbon and nitrogen post-stress [45–49]. Higher proline levels in plants enable the maintenance of higher water potentials which permits the maintenance of water status under a stressful environment; hence it buffers the instant WD effect within the plants [50]. Higher proline accumulation in mung bean genotypes is possibly a constructive adaptive mechanism for overcoming the WD stress effect. Therefore, it is implied that drought-tolerant new mung bean genotype selection based on the higher accumulation of proline is advocated, and it is considered a vital indicator to identify drought-tolerant genotypes [51,52].
Usually, chlorophyll contents act as an important role in photosynthesis, which allow plants to absorb energy from light [53]. It is significantly decreased with increasing the level of drought stress in the plants of mung bean [54], and common bean [55]. However, the downward changes in chlorophyll contents under drought have been responsible for a distinctive indication of oxidative stress, and may be the outcome of pigment photooxidation and chlorophyll degradation [56]. Photosynthetic pigments are chief representatives of mung bean plants under DS conditions as reported by [57]. In this study, WD stress showed a considerable dissimilarity in Chl a, Chl b and chlorophyll a/b ratio at flowering stage (Table 3). The minimum relative reduction of Chl a, Chl b as well as total chlorophyll content was recorded in BMX-08010-2 under WD stress over WW condition, whereas the reverse result was found in BARI Mung-1. It might be owing to the remarkable reduction of the chlorophyllase function in BARI Mung-1 under WD stress situation, and this statement was highlighted earlier [58]. A similar conclusion is urged in a recent study where WD stress remarkably reduced the leaf chlorophyll contents [54,59]. The results are in also accordance with that drought stress significantly decreased the total chlorophyll content in mung bean [60–62]. In the study, a higher value of CSI in BMX-08010-2 in contrast to other genotypes indicated the comparatively superior tolerance to DS which might have been responsible for greater photosynthesis, and finally carrying on higher yield. The least value was found in BARI Mung-1 which might be happened due to early senescence of leaves, and it has been noticed earlier that lower CSI value under stress is a consequence of premature senescence of mung bean leaves [63].
The RWC is an effective measurement tool of the water status in the plant that reflects the metabolic activity in the plants, and is used as a most evocative indicator for stress tolerance [64]. Moreover, when plants were fallen water stress, leaves demonstrate a great reduction in RWC and water potential [65]. Among the studied genotypes, the highest relative reduction of RWC under WD condition was opined in BARI Mung-1, while the least one was in BMX-0801-2. The variation in RWC within genotypes might be imputed to the differences in their potential to survive and adapt under DS conditions [66]. Several researchers also recognized that the reduction of RWC affected the growth and development of crop plants like mung bean [54,67], chickpea [68], soybean [69], wheat [59], foxtail millet and proso millet [70]. The finding of the present study confirmed the above statements and supports the option that the RWC could be used as a pointer to stress tolerance. It is assumed that WD condition reduces the soil water potential ultimately trim downs the RWC by dehydration at a cellular level and also due to osmotic stress. WSD refers to the amount of water shortage in plant parts at an individual condition [71]. The maximum WSD was observed in plants under WD condition, and the highest value of WSD signposts the plants that are facing countless marks of DS [72]. The results exhibited that the genotype BMX-08010-2 displayed the least increment of WSD, while the genotype BARI Mung-1 showed the most increment over WW condition. However, a genotype with a higher deviation of WSD as compared to WW exposed that they suffered severely due to WD stress compared to other genotypes. The present result agrees with the finding of [54,73] in mung bean, and [74] in bush bean who also observed the increasing trends of WSD under WD condition. The WUC represents the efficiency of a plant to uptake water per unit of dry weight at a particular stage. A large amount of WUC indicates that plants absorb a colossal amount of water to reach turgid weight under moisture stress [75]. Under this experiment, the genotype BMX-08010-2 showed the lowest relative increment of WUC, and the highest relative increment was in BARI Mung-1. The highest relative value (% over control) of WUC of a genotype indicated that the genotype suffered more from WD stress than the others, and vice-versa. These results are in concurrence with the previously reported studies for mung bean [54], and bush bean [74], where it was reported that the tolerant genotypes acquired the lowest WUC under DS compared to the susceptible genotypes. The WRC (the proportion of turgid and dry weight) interprets the water holding capacity of a leaf under particular conditions [71]. This ratio is also ascertained by the cell structures [76]. The plant rising under optimal supply of water preserves a more WRC, and it might be due to lesser demolition of plant tissues under DS [75]. Furthermore, a reduction of leaf WRC signposted a shrinkage of cell size [77] that constitutes one of the most general anatomical modifications in leaves under DS [78]. In the present study, plants raised under an optimum soil moisture condition (WW) had a maximum ratio than that of the plant raised under WD stress conditions. However, the minimum relative reduction was obtained in the BMX-08010-2 genotype, and the maximum was in BARI Mung-1. The higher relative reduction of WRC in BARI Mung-1 indicated superior impairment in cell structure due to water deficit stress. Similar results were observed earlier with mung bean [54], and black gram [75,77]. The decrease in the leaf WRC could be the outcome of hemicellulose and cellulose derivatives in the cell [79]. An adequate supply of water (WW) also helps to repair damaged cells of plants as well as enhanced the WRC of plants. The XER can be used as an effective tool to determine the intensity of water stress. Our findings reveal that BARI Mung-1 passes through more water stress compared to that of BMX-08010-2 due to a decline of XER under WD stress resulting in lower uptake of water in the plant. This result is in agreement with the findings of [54] in mung bean, and [80] in wheat, who found a reduction of water uptake in the plant due to WD stress. The traits RWC and XER are also directly related to the flow of the transpiration stream [75]. Under WD stress condition, the soil moisture can be expected to be lower in different growth spans (Fig. 1), which affected the growth and development of plants especially produced lower branches, ultimately produced a little number of pods plant−1, and finally decreasing grain yield. The results pointed out that BMX-08010-2 and BMX-08009-7 exposed lower stress injury as compared to BARI Mung-1 and BARI Mung-3 with the relative number of pods plant−1. The present result which is the diminishing in the number pods plant−1 due to water stress is consistent with the findings in French bean [81,82], soybean [83,84], cowpea [85], pigeonpea [86], faba bean [87], and mung bean [73]. Moreover, the reproductive stage under water stress maximized the number of aborted flowers, and decreased the number of pods plant−1 in common bean [88]. A similar outcome could be projected for mung bean [89]. The pod length is an important parameter, which significantly influences the grain yield of mung bean. Stunted pod length under WD condition reduced the number of seeds pod−1 resulting reduced total yield. This result supports the findings in soybean [90], and mung bean [91]. The findings of the study pointed out that the genotypic differences appeared in the number of seed pod−1 under WD conditions. Nonetheless, drought-induced by water stress decreased the number of seeds pod−1 in faba bean [87], and snap bean [81]. Earlier results also reported that the number of pods plant−1, and the number of seeds pod−1, might be reduced due to the decrease in pollen fertility under DS [81]. The BMX-08010-2 genotype produced the highest relative value of the 100-seed weight, and the lowest was found in BARI Mung-1. Flowering and seed formation phases might be the sensitive periods for soil moisture in leguminous crops which affect 100-seed weight [92]. A similar trend was found in the case of seed yield plant−1, indicating the genotype BMX-08010-2 was the most WD stress tolerant, while BARI Mung-1 was the most sensitive genotype to water stress. The previous study reported that the mung bean yield reduced to 64% and 34% due to the imposition of WD stress of 75% and 50% of field capacity, respectively [93]. The results of the present study were also closely in agreement with the findings obtained in mung bean [54,94], faba bean [87] and common bean [88], who reported that WD stress severely declined the grain yield when it imposed at reproductive stage, particularly at flowering and pod formation stages. Earlier studies also depicted that the decreased number of pods plant−1 under drought stress was owing to an increased rate of abortion of reproductive organs resulting in lower seed yield [84,95–97].
There were several tolerance indices that have predicted from the yield data of WW and WD conditions with a specific genotype, and they also indicating the susceptibility and tolerance of a genotypes. The TOL, SSI and STI are some of them. The lower value of TOL indicating the reduction of grain yield under stress condition, and categorized as stress sensitivity. The SSI also succeeded the trend as like as TOL in the same genotypes. The value of SSI is lower than 0.5 (<0.5) indicates the crop is highly tolerant to WD stress or very low drought susceptibility or higher yield stability, if SSI greater than 0.5 but less than l.0, then it is moderately tolerant, and values are higher than 1 (>1.0) signify susceptible to water stress or poor yield stability [36]. A higher STI value for a genotype is an indicator of greater drought tolerance, and the potential yield for that genotype [98]. In our study, BMX-08010-2 genotype showed the lowest TOL and SSI value along with the highest STI, and could be considered as drought tolerant genotype, whereas reverse result found in case of BARI Mung-1.
Accordance to the results, the BMX-08010-2 performed as the most drought tolerant genotype of mung bean due to higher proline accumulation, plant water status, relative performances of yield traits, STI, and less degradation chlorophyll contents and lower yield penalty under WD conditions than other genotypes, whereas BARI Mung-1 showed the most drought susceptible genotype generating reverse values of the traits. Therefore, BMX-08010-2 could be advocated for further varietal improvement programs in water-deficient farming areas.
Acknowledgement: The authors extend their appreciation to the Researchers Supporting Project No. (RSP-2021/298), King Saud University, Riyadh, Saudi Arabia. The author is highly thankful to the Head and lab Technicians, Department of Agricultural Chemistry, HSTU, Dinajpur, for providing laboratory facilities for chemical analysis of the plant samples. The author wishes to express special thanks to Hassan Nuur Ismaan, Md. Obaidullah Shaddam and Eunis Ali of Ex-MS student in the Department of Agronomy, HSTU, Dinajpur for their help for sample collection, data recording and biochemical analysis of experimental samples during the research. Finally, the author is very gratitude to the authority of Bangladesh Agricultural Research Institute (BARI) for granting the scholarship and allowing him leave for completion of the study.
Funding Statement: The authors extend their appreciation to the researchers Supporting Project No. (RSP-2021/298), King Saud University, Riyadh, Saudi Arabia.
Conflicts of Interest: The authors declare that they have no conflicts of interest to report regarding the present study.
References
1. Ghotbi-Ravandi, A., Shahbazi, M., Shariati, M., Mulo, P. (2014). Effects of mild and severe drought stress on photosynthetic efficiency in tolerant and susceptible barley (Hordeum vulgare L.) genotypes. Journal of Agronomy and Crop Science, 200(4), 403–415. DOI 10.1111/jac.12062. [Google Scholar] [CrossRef]
2. Bangar, P., Chaudhury, A., Tiwari, B., Kumar, S., Kumari, R. et al. (2019). Morphophysiological and biochemical response of mung bean (Vigna radiata (L.) Wilczek) varieties at different developmental stages under drought stress. Turkish Journal of Biology, 43(1), 58–69. DOI 10.3906/biy-1801-64. [Google Scholar] [CrossRef]
3. Kumar, A., Sharma, K. D. (2009). Physiological responses and dry matter partitioning of summer mung bean (Vigna radiata L.) genotypes subjected to drought conditions. Journal of Agronomy and Crop Science, 195(4), 270–277. DOI 10.1111/j.1439-037X.2009.00373.x. [Google Scholar] [CrossRef]
4. Baroowa, B., Gogoi, N. (2015). Changes in plant water status, biochemical attributes and seed quality of black gram and green gram genotypes under drought. International Letters of Natural Sciences, 42, 1–12. DOI 10.18052/www.scipress.com/ILNS.42.1. [Google Scholar] [CrossRef]
5. Kumar, A., Omae, H., Egawa, Y., Kashiwaba, K., Shono, M. (2005). Influence of water and high temperature stresses on leaf water status of high temperature tolerant and sensitive cultivars of snap bean (Phaseolus vulgaris L.). Japanese Journal of Tropical Agriculture, 49(2), 109–118. DOI 10.11248/jsta1957.49.109. [Google Scholar] [CrossRef]
6. Islam, M. S., Hasan, K., Sarkar, N. A. A., El Sabagh, A., Rashwan, E. et al. (2017a). Yield and yield contributing characters of mung bean as influenced by zinc and boron. Scientific Journals of Agricultural Advances, 6(1), 391–397. DOI 10.14196/aa.v6i1.2362. [Google Scholar] [CrossRef]
7. Islam, M. S., El Sabagh, A., Hasan, K., Akhter, M., Barutçular, C. et al. (2017b). Growth and yield response of mung bean (Vigna radiata L.) as influenced by sulphur and boron application. Scientific Journal of Crop Science, 6(1), 153–160. DOI 10.14196/sjcs.v6i1.2383. [Google Scholar] [CrossRef]
8. Islam, M. S., Fahad, S., Hossain, A., Chowdhury, M. K., Iqbal, M. et al. (2021). Legumes under drought stress: Plant responses, adaptive mechanisms and management strategies in relation to nitrogen fixation. In: Fahad, S., Sönmez, O., Saud, S., Wang, D., Wu, C. et al. (Eds.Engineering tolerance in crop plants against abiotic stress, pp. 178–208. Boca Raton, USA: CRC Press. [Google Scholar]
9. Thomas, Robertson, M. J., Fukaic, S., Peoples, M. B. (2004). The effect of timing and severity of water deficit on growth, development, yield accumulation and nitrogen fixation of mung bean. Field Crops Research, 86(1), 67–80. DOI 10.1016/S0378-4290(03)00120-5. [Google Scholar] [CrossRef]
10. Ashraf, M., Harris, P. J. C. (2004). Potential biochemical indicators of salinity tolerance in plants. Plant Science, 166(1), 3–16. DOI 10.1016/j.plantsci.2003.10.024. [Google Scholar] [CrossRef]
11. Serraj, R., Sinclair, T. R. (2002). Osmolyte accumulation: Can it really help increase crop yield under drought conditions? Plant, Cell & Environment, 25(2), 333–341. DOI 10.1046/j.1365-3040.2002.00754.x. [Google Scholar] [CrossRef]
12. Ashraf, M. (2004). Some important physiological selection criteria for salt tolerance in plants. Flora, 199(5), 361–376. DOI 10.1078/0367-2530-00165. [Google Scholar] [CrossRef]
13. Irigoyen, J. J., Emerich, D. W., Sanchez-Diaz, M. (1992). Water stress induced changes in concentrations of proline and total soluble sugars in nodulated alfalfa (Medicago sativa) plants. Physiologia Plantarum, 84(1), 55–60. DOI 10.1111/j.1399-3054.1992.tb08764.x. [Google Scholar] [CrossRef]
14. Kohl, D. H., Kennelly, E. J., Zhu, Y., Schubert, K. R., Shearer, G. (1991). Proline accumulation, nitrogenase activity and activities of enzymes related to proline metabolism in drought-stressed soybean nodules. Journal of Experimental Botany, 42(7), 831–837. DOI 10.1093/jxb/42.7.831. [Google Scholar] [CrossRef]
15. Hooda, A., Nandwal, A. S., Kuhad, M. S., Dutta, D. (1999). Plant water status and C, N and K distribution in potassium fertilised mung bean under drought and during recovery. In: Faroda, A. S., Joshi, N. L., Kathju, S., Karj, A. (Eds.Recent advances in management of arid ecosystem, pp. 207–214. Jodhpur, India: Arid Zone Research Association of India. [Google Scholar]
16. Garg, B. K., Vyas, S. P., Kathyu, S., Lahiri, A. N. (1998). Influence of water deficit stress at various growth stages on some enzymes of nitrogen metabolism and yield in cluster bean genotypes. Indian Journal of Plant Physiology, 3(3), 214–218. [Google Scholar]
17. Day, W. (1981). Water stress and crop growth. In: Johnson, C. B. (Ed.Physiological processes limiting productivity, pp. 199–215. London: Butterworths. [Google Scholar]
18. Sayed, O. H. (2003). Chlorophyll fluorescence as a tool in cereal crop research. Photosynthetica, 41(3), 321–330. DOI 10.1023/B:PHOT.0000015454.36367.e2. [Google Scholar] [CrossRef]
19. Nejadsahebi, M., Moallemi, N., Landi, A. (2010). Effects of cycocel and irrigation regimes on some physiological parameters of three olive cultivars. American Journal of Applied Science, 7(4), 459–465. DOI 10.3844/ajassp.2010.459.465. [Google Scholar] [CrossRef]
20. Hussain, S., Hussain, S., Qadir, T., Khaliq, A., Ashraf, U. et al. (2019). Drought stress in plants: An overview on implications, tolerance mechanisms and agronomic mitigation strategies. Plant Science Today, 6(4), 389–402. DOI 10.14719/pst.2019.6.4.578. [Google Scholar] [CrossRef]
21. Raza, M. A. S., Shahid, A. M., Saleem, M. F., Khan, I. H., Ahmad, S. et al. (2017). Effects and management strategies to mitigate drought stress in oilseed rape (Brassica napus L.A review. Zemdirbyste-Agriculture, 104(1), 85–94. DOI 10.13080/z-a.2017.104.012. [Google Scholar] [CrossRef]
22. Islam, M. R. (2020). Improving mung bean productivity under drought stress through screening of genotypes and potassium application (Ph.D. Thesis). Department of Agronomy, Hajee Mohammad Danesh Science and Technology University (HSTUDinajpur, Bangladesh. [Google Scholar]
23. Azad, A. K., Gosshami, B. K., Rahman, M. L., Malaker, P. K., Hasan, M. S. et al. (2017). Krishi ProjuktiHatboi (Handbook on agro-technology). 7th edition. Gazipur, Bangladesh: Bangladesh Agricultural Research Institute. [Google Scholar]
24. Swathi, L., Reddy, D. M., Sudhakar, P., Vineela, V. (2017). Screening of mung bean (Vigna radiata L. Wilczek) genotypes against water stress mediated through polyethylene glycol. International Journal of Current Microbiology and Applied Sciences, 6(10), 2524–2531. DOI 10.20546/ijcmas.2017.610.296. [Google Scholar] [CrossRef]
25. Dutta, P., Bera, A. K. (2008). Screening of mung bean genotypes for drought tolerance. Legume Research, 31(2), 145–148. [Google Scholar]
26. Saminathan, B. (2013). Effect of chromium studies on germination and biochemical content of black gram (Vigna mungo (L.) Hepper.). International Journal of Advanced Research, 1(7), 216–222. [Google Scholar]
27. Michael, A. M. (1996). Irrigation theory and practice, pp. 512. New Delhi, India: Vikas Publishing House Pvt. Ltd. [Google Scholar]
28. Bates, L. S., Waldren, R. P., Teare, I. D. (1973). Rapid determination of free proline for water stress studies. Plant & Soil, 39(1), 205–208. DOI 10.1007/BF00018060. [Google Scholar] [CrossRef]
29. Witham, H., Blades, D. F., Devin, R. H. (1986). Exercise in plant physiology. 2nd edition, pp. 128–131. Boston, USA: PWS Publishers. [Google Scholar]
30. Sairam, R. K. (1994). Effect of moisture stress on physiological activities of two contrasting wheat genotypes. Indian Journal of Experimental Biology, 32, 594–597. [Google Scholar]
31. Barr, H. D., Weatherley, P. E. (1962). A re-examination of the relative turgidity technique for estimating water deficit in leaves. Australian Journal of Biological Science, 15(3), 413–428. DOI 10.1071/BI9620413. [Google Scholar] [CrossRef]
32. Saneoka, H., Hamid, A., Hashem, A. (1995). Soil and plant water relations. IPSA-JICA Project Reports. Salna, Gazipur, Bangladesh. [Google Scholar]
33. Baque, M. A., Karim, M. A., Hamia, A. (2002). Role of potassium on water relation behavior of Triticum aestivum L. under water stress conditions. Progressive Agriculture, 13(1&2), 71–75. [Google Scholar]
34. Hasan, M. K., Islam, M. S., Islam, M. R., Ismaan, H. N., Sabagh, A. E. L. et al. (2019). Water relations and dry matter accumulation of black gram and mung bean as affected by salinity. Thai Journal of Agriculture Science, 52(1), 54–67. [Google Scholar]
35. Asana, R. D., Williams, R. F. (1965). The effect of temperature stress on grain development in wheat. Australian Journal of Agricultural Research, 16(1), 1–3. DOI 10.1071/AR9650001. [Google Scholar] [CrossRef]
36. Fischer, R. A., Maurer, R. (1978). Drought resistance in spring wheat cultivars. Australian Journal of Agricultural Research, 29(5), 897–912. DOI 10.1071/AR9780897. [Google Scholar] [CrossRef]
37. Hossain, A. B. S., Sears, A. G., Cox, T. S., Paulsen, G. M. (1990). Desiccation tolerance and its relationship to assimilate partitioning in winter wheat. Crop Science, 30(3), 622–627. DOI 10.2135/cropsci1990.0011183X003000030030x. [Google Scholar] [CrossRef]
38. Fernandez, G. C. J. (1992). Effective selection criteria for assessing stress tolerance. Proceedings of the International Symposium on Adaptation of Vegetables and Other Food Crops in Temperature and Water Stress, pp. 257–270. Taiwan: AVRDC Publication. [Google Scholar]
39. Team, R. C. (2016). R: A language and environment for statistical computing. R Foundation for Statistical Computing, Vienna, Austria. https://www.r-project.org. [Google Scholar]
40. Gomez, K. A., Gomez, A. A. (1984). Statistical procedures for agricultural research. 2nd edition, pp. 302–307. Singapore: A Wiley Interscience Publication, John Wiley and Sons. [Google Scholar]
41. Mendiburu, F. D. (2015). Agricolae: Statistical procedures for agricultural research. R Package Version 1.2-3. http://CRAN.R-project.org/package=agricolae. [Google Scholar]
42. Sanchez, F. J., Manzanarcs, M., Andres, R. F., Tcrnorio, J. L., de Ayerbe, L. et al. (1998). Turgor maintenance, osmotic adjustment and soluble sugar and proline accumulation in 49 pea cultivars in response to water stress. Field Crop Research, 59(3), 225–235. DOI 10.1016/S0378-4290(98)00125-7. [Google Scholar] [CrossRef]
43. Stoyanov, Z. Z. (2005). Effect of water stress on leaf water relations of young bean plants. Journal of Central European Agriculture, 6(1), 5–14. [Google Scholar]
44. Ludlow, M. M., Muchow, R. C. (1990). A critical evaluation of traits for improving crop yields in water limited environments. Advances in Agronomy, 43, 107–153. DOI 10.1016/S0065-2113(08)60477-0. [Google Scholar] [CrossRef]
45. Singh, D. K., Sale, P. W. G., Pallaghy, C. K., Singh, V. (2000). Role of proline and leaf expansion rate in the recovery of stressed white clover leaves with increased phosphorus concentration. The New Phytologist, 146(2), 261–269. DOI 10.1046/j.1469-8137.2000.00643.x. [Google Scholar] [CrossRef]
46. Kavi-Kishor, P. B., Sangam, S., Amrutha, R. N., Sri-Laxmi, P., Naidu, K. R. et al. (2005). Regulation of proline biosynthesis, degradation, uptake and transport in higher plants: Its implications in plant growth and abiotic stress tolerance. Current Science, 88(3), 424–438. [Google Scholar]
47. Bayoumi, T. Y., Eid, M. H., Metwali, E. M. (2008). Application of physiological and biochemical indices as a screening technique for drought tolerance in wheat genotypes. African Journal of Biotechnology, 7(14), 2341–2352. DOI 10.5897/AJB2008.000-5059. [Google Scholar] [CrossRef]
48. Watanabe, S., Kojima, K., Ide, Y., Sasaki, S. (2000). Effects of saline and osmotic stress on proline and sugar accumulation in Populus euphratica in vitro. Plant Cell, Tissue and Organ Culture, 63(3), 199–206. DOI 10.1023/A:1010619503680. [Google Scholar] [CrossRef]
49. Saruhan, N., Terzi, R., Kadioglu, A. (2006). The effects of exogenous polyamines on some biochemical changes during drought stress in Ctenanthe setosa (Rosc.) Eichler. Acta Biologica Hungarica, 57(2), 221–229. DOI 10.1556/ABiol.57.2006.2.9. [Google Scholar] [CrossRef]
50. Mousa, H. R., Abdel-Aziz, S. M. (2008). Comparative response of drought tolerant and drought sensitive maize genotypes to water stress. Australian Journal of Crop Science, 1(1), 31–36. [Google Scholar]
51. Silverira, J., Viegas, R., da Rocha, I., Moreira, A., Moreira, R. et al. (2003). Proline accumulation and glutamine synthetase activity are increased by salt induced proteolysis in cashew leaves. Journal of Plant Physiology, 160(2), 115–123. DOI 10.1078/0176-1617-00890. [Google Scholar] [CrossRef]
52. Jaleel, C. A., Gopi, R., Sankar, B., Manivannan, P., Kishorekumar, A. et al. (2007). Studies on germination, seedling vigour, lipid peroxidation and proline metabolism in Catharanthus roseus seedlings under salt stress. South African Journal of Botany, 73(2), 190–195. DOI 10.1016/j.sajb.2006.11.001. [Google Scholar] [CrossRef]
53. Carter, G. A., Knapp, A. K. (2001). Leaf optical properties in higher plants: Linking spectral characteristics to stress and chlorophyll concentration. American Journal of Botany, 88(4), 677–684. DOI 10.2307/2657068. [Google Scholar] [CrossRef]
54. Islam, M. R., Kamal, M. M., Alam, M. A., Hossain, J., Soufan, W. et al. (2021). Physiochemical changes of mung bean [Vigna radiata (L.) R. Wilczek] in responses to varying irrigation regimes. Horticulturae, 7(12), 565. DOI 10.3390/horticulturae7120565. [Google Scholar] [CrossRef]
55. Abass, S. M., Mohamed, H. I. (2011). Alleviation of adverse effects of drought stress on common bean (Phaseolus vulgaris L.) by exogenous application of hydrogen peroxide. Bangladesh Journal of Botany, 40(1), 75–83. DOI 10.3329/bjb.v40i1.8001. [Google Scholar] [CrossRef]
56. Baroowa, B., Gogoi, N., Paul, S., Baruah, K. K. (2015). Response of leaf water status, stomatal characteristics, photosynthesis and yield in black gram and green gram genotypes to soil water deficit. Functional Plant Biology, 42(10), 1010–1018. DOI 10.1071/FP15135. [Google Scholar] [CrossRef]
57. Batra, N. G., Vinay, S., Nilima, K. (2014). Drought-induced changes in chlorophyll fluorescence, photosynthetic pigments, and thylakoid membrane proteins of Vigna radiata. Journal of Plant Interactions, 9(1), 712–721. DOI 10.1080/17429145.2014.905801. [Google Scholar] [CrossRef]
58. Reddy, M. P., Vora, A. B. (1986). Salinity induced changes in pigment composition and chlorophyllase activity of wheat. Indian Journal of Plant Physiology, 29(4), 331–334. [Google Scholar]
59. Chowdhury, M. K., Hasan, M. A., Bahadur, M. M., Islam, M. R., Hakim, A. A. et al. (2021). Evaluation of drought tolerance of some wheat (Triticum aestivum L.) genotypes through phenology, growth, and physiological indices. Agronomy, 11(9), 1792. DOI 10.3390/agronomy11091792. [Google Scholar] [CrossRef]
60. Allahmoradi, P., Mokhtar, G., Shayesteh, T., Shahrbanu, T. (2011). Physiological aspects of mung bean (Vigna radiata L.) in response to drought stress. International Conference on Food Engineering and Biotechnology. IPCBEE, vol. 9, pp. 272–275. Jurong West, Singapore, IACSIT Press. [Google Scholar]
61. Lalinia, A. A., MajnonHoseini, N., Galostian, M., EsmaeilzadehBahabadi, S., MarefatzadehKhameneh, M. (2012). Echophysiological impact of water stress on growth and development of mung bean. International Journal of Agronomy and Plant Production, 3(12), 599–607. [Google Scholar]
62. Thaltooth, A. T., Tawfik, M. M., Mohamed, H. M. (2006). A comparative study on the effect of foliar application of zinc, potassium and magnesium on growth, yield and some chemical constituents of mung bean plants grown under water stress conditions. World Journal of Agricultural Sciences, 2(1), 37–46. [Google Scholar]
63. Dutta, P., Bera, A. K. (2014). Effect of NaCl salinity on seed germination and seedling growth of mung bean cultivars. Legume Research, 37(2), 161–164. DOI 10.5958/j.0976-0571.37.2.024. [Google Scholar] [CrossRef]
64. Anjum, S. A., Xie, X., Wang, L., Saleem, M. F., Man, C. et al. (2011). Morphological, physiological and biochemical responses of plants to drought stress. African Journal of Agricultural Research, 6(9), 2026–2032. DOI 10.5897/AJAR10.027. [Google Scholar] [CrossRef]
65. Nayyar, H., Gupta, D. (2006). Differential sensitivity of C3and C4 plants to water deficit stress: Association with oxidative stress and antioxidants. Environmental and Experimental Botany, 58(1–3), 106–113. DOI 10.1016/j.envexpbot.2005.06.021. [Google Scholar] [CrossRef]
66. Naidu, T. C. M., Raju, N., Narayanan, A. (2001). Screening of drought tolerance in green gram (Vigna radiata L. Wilczek) genotypes under receding soil moisture. Indian Journal of Plant Physiology, 6(2), 197–201. [Google Scholar]
67. Ratnasekera, D., Subhashi, A. P. T. (2015). Morpho-physiological response of selected mung bean (Vigna radiata L.) Sri Lankan genotypes to drought stress. Journal of AgriSearch, 2(3), 162–166. [Google Scholar]
68. Rahbarian, R., Ramazanali, K., Ali, G., Abdolreza, B., Farzaneh, N. (2011). Drought stress effects on photosynthesis, chlorophyll fluorescence and water relations in tolerant and susceptible chickpea (Cicer arietinum L.) genotypes. Acta Biologica Cracoviensia Series Botanica, 53(1), 47–56. DOI 10.2478/v10182-011-0007-2. [Google Scholar] [CrossRef]
69. Khan, M. S. A., Karim, M. A., Abullah-Al-Mahmud., Parveen, S., Bazzaz, M. M. et al. (2015). Plant water relations and proline accumulations in soybean under salt and water stress environment. Journal of Plant Sciences, 3(5), 272–278. DOI 10.11648/j.jps.20150305.15. [Google Scholar] [CrossRef]
70. Islam, M. S., Akhter, M. M., El Sabagh, A., Liu, L. Y., Nguyen, N. T. et al. (2011). Comparative studies on growth and physiological responses to saline and alkaline stresses of foxtail millet (Setaria italica L.) and proso millet (Panicum miliaceum L.). Australian Journal Crop Science, 5(10), 1269–1277. [Google Scholar]
71. Islam, M. S. (2001). Morpho-physiology of blackgram and mung bean as influenced by salinity (M.S. Thesis). Department of Agronomy, Bangabandhu Sheikh Mujibur Rahman Agricultural University, Salna, Gazipur, Bangladesh. [Google Scholar]
72. Bazzaz, M. M. (2013). Morpho-physiology and yield response of wheat to variable water regimes (Ph.D. Thesis). Department of Agronomy, Bangabandhu Sheikh Mujibur Rahman Agricultural University, Salna, Gazipur, Bangladesh. [Google Scholar]
73. Islam, M. S. (2008). Water stress tolerance of mung bean [Vigna radiata (L.) Wilczek] genotypes as influenced by plant growth regulators (Ph.D. Thesis). Department of Agronomy, Bangabandhu Sheikh Mujibur Rahman Agricultural University, Salna, Gazipur, Bangladesh. [Google Scholar]
74. Choudhury, A. K. (2009). Water stress tolerance of French bean (Phaseolus vulgaris L.) (Ph.D. Thesis). Department of Agronomy, Bangabandhu Sheikh Mujibur Rahman Agricultural University, Salna, Gazipur, Bangladesh. [Google Scholar]
75. Sangakkara, U. R., Hartwig, U. A., Nosberger, J. (1996). Response of root branching and shoot water potentials of French bean (Phaseolus vulgaris L.) to soil moisture and fertilizer potassium. Journal of Agronomy and Crop Science, 177(3), 165–173. DOI 10.1111/j.1439-037X.1996.tb00234.x. [Google Scholar] [CrossRef]
76. Rahman, M. A. (2015). Enhancement of drought tolerance in mung bean through osmo and hydro priming (M.S. Thesis). Department of Agronomy, Sher-e-Bangla Agricultural University, Sher-e-Bangla Nagar, Dhaka, Bangladesh. [Google Scholar]
77. Martinez, J. P., Silva, H., Ledent, J. F., Pinto, M. (2007). Effect of drought stress on the smotic adjustment, cell wall elasticity and cell volume of six cultivars of common beans (Phaseolus vulgaris L.). European Journal of Agronomy, 26(1), 30–38. DOI 10.1016/j.eja.2006.08.003. [Google Scholar] [CrossRef]
78. Tardieu, F., Reymond, M., Hamard, P., Granier, C., Muller, B. (2000). Spatial distribution of expansion rate, cell division rate and cell size in maize leaves: A synthesis of the effects of soil water status, evaporative demand and temperature. Journal of Experimental Botany, 51(350), 1505–1514. DOI 10.1093/jexbot/51.350.1505. [Google Scholar] [CrossRef]
79. Wakabayashi, H., Abe, H., Sakamoto, M. (1997). Wood preservatives containing troponoid metal complexes. Japanese Patent No. JP 09175916, Tokyo, Japan: MitsubishiGas Chemical Co. Inc. Chiyoda-ku. [Google Scholar]
80. Baque, M. A. (2003). Potassium induced changes in the physiology of wheat plants (Triticum aestivum L.) under water stress conditions (M.S. Thesis). Department of Agronomy, Bangabandhu Sheikh Mujibur Rahman Agricultural University, Salna, Gazipur, Bangladesh. [Google Scholar]
81. Omae, H., Kumar, A., Egawa, Y., Kashiwaba, K., Shono, M. (2005). Midday drop of leaf water content to drought tolerance in snap bean (Phaseolus vulgaris L.). Plant Production Science, 8(4), 465–467. DOI 10.1626/pps.8.465. [Google Scholar] [CrossRef]
82. Nunez-Barrios, A., Hoogenboom, G., Nesmith, D. C. (2005). Drought stress and the distribution of vegetative and reproductive traits of bean cultivar. Scientia Agricola, 62(1), 18–22. DOI 10.1590/S0103-90162005000100004. [Google Scholar] [CrossRef]
83. Kokubun, M., Shimada, S., Takahashi, M. (2001). Flower abortion caused by preanthesis water deficit is not attributed to impairment of pollen in soybean. Crop Science, 41(5), 1517–1521. DOI 10.2135/cropsci2001.4151517x. [Google Scholar] [CrossRef]
84. Liu, F., Andersen, M. N., Jensen, C. R. (2004). Root signal controls pod growth in drought-stressed soybean during the critical, abortion-sensitive phase of pod development. Field Crop Research, 85(2), 159–166. DOI 10.1016/S0378-4290(03)00164-3. [Google Scholar] [CrossRef]
85. Turk, K. J., Hall, A. E. (1980). Drought adaptation of cowpea. Influence of drought on plant water status and relation with seed yield. Agronomy Journal, 72(3), 421–427. DOI 10.2134/agronj1980.00021962007200030005x. [Google Scholar] [CrossRef]
86. Lopez, F. B., Johansen, C., Chauhan, Y. S. (1996). Effect of timing of drought stress on phenology, yield and yield components of a short-duration pigeonpea. Journal of Agronomy and Crop Science, 177(5), 311–320. DOI 10.1111/j.1439-037X.1996.tb00251.x. [Google Scholar] [CrossRef]
87. Ricciardi, L., Polignano, G. B., de Giovanni, C. (2001). Genotypic response of faba bean to water stress. Euphytica, 118(1), 39–46. DOI 10.1023/A:1004078017159. [Google Scholar] [CrossRef]
88. Simsek, M., Comlekcioglu, N., Ozturk, I. (2011). The effects of the regulated deficit irrigation on yield and some yield components of common bean (Phaseolus vulgaris L.) under semi-arid conditions. African Journal of Biotechnology, 10(20), 4057–4064. DOI 10.4314/AJB.V10I20. [Google Scholar] [CrossRef]
89. Sharma, L., Priya, M., Bindumadhava, H., Nair, R. M., Nayyar, H. (2016). Influence of high temperature stress on growth, phenology and yield performance of mungbean [Vigna radiate (L.) Wilczek] under managed growth conditions. Scientia Horticulturae, 213(6), 379–391. DOI 10.1016/j.scienta.2016.10.033. [Google Scholar] [CrossRef]
90. Westgate, M. E., Peterson, C. M. (1993). Flower and pod development in water deficient soybean (Glycine max L. Merr.). Journal of Experimental Botany, 44(1), 109–117. DOI 10.1093/jxb/44.1.109. [Google Scholar] [CrossRef]
91. Pannu, R. K., Singh, D. P. (1988). Influence of water deficits on morpho-physiological and yield behaviour of mung bean (Vigna radiata (L.) Wilczek). Proceeding of the Second International Symposium, pp. 252–259. Taiwan. [Google Scholar]
92. Reddy, S. R. (2007). Irrigation agronomy. New Delhi, India: Kalayani Publishers. [Google Scholar]
93. Ambachew, S., Tena, A., Assefa, M. (2014). Performance of mung bean under deficit irrigation application in the semi-arid highlands of Ethiopia. Agricultural Water Management, 136(C), 68–74. DOI 10.1016/j.agwat.2014.01.012. [Google Scholar] [CrossRef]
94. de Costa, W. A. J. M., Shanmugathasan, K. N. (1999). Effects of irrigation at different growth stages on vegetative growth of mung bean, Vigna radiata (L.) Wilczek, in dry and intermediate zones of Sri Lanka. Journal of Agronomy and Crop Science, 183(2), 137–143. DOI 10.1046/J.1439-037X.1999.00328.X. [Google Scholar] [CrossRef]
95. Graham, P. H., Ranalli, P. (1997). Common bean (Phaseolus vulgaris L.). Field Crops Research, 53(1–3), 131–146. DOI 10.1016/S0378-4290(97)00112-3. [Google Scholar] [CrossRef]
96. Teran, M., Singh, S. P. (2002). Comparison of sources and lines selected for drought resistance in common bean. Crop Science, 42(1), 64–70. DOI 10.2135/cropsci2002.6400. [Google Scholar] [CrossRef]
97. Liu, F., Andersen, M. N., Jensen, C. R. (2003). Loss of pod set caused by drought stress is associated with water status and ABA content of reproductive structures in soybean. Functional Plant Biology, 30(3), 171–280. DOI 10.1071/FP02185. [Google Scholar] [CrossRef]
98. Kumar, P., Pravesh, K., Tarkeshwar, S., Anil, K. S., Ram, I. Y. (2014). Effect of different potassium levels on mung bean under custard apple based agri-horti system. African Journal of Agricultural Research, 9(8), 728–734. DOI 10.5897/AJAR2013.7883. [Google Scholar] [CrossRef]
Cite This Article
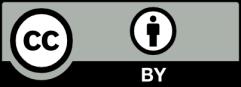