Open Access
ARTICLE
Metabolites Composition of Bacillus subtilis HussainT-AMU Determined by LC-MS and Their Effect on Fusarium Dry Rot of Potato Seed Tuber
1
Plant Pathology and Nematology Section, Department of Botany, Aligarh Muslim University, Aligarh, India
2
Biological and Geological Sciences Department, Faculty of Education, Ain Shams University, Cairo, Egypt
* Corresponding Authors: Touseef Hussain. Email: ; Heba I. Mohamed. Email:
(This article belongs to the Special Issue: Role of Biostimulants in the Alleviation of Biotic and Abiotic Stress)
Phyton-International Journal of Experimental Botany 2023, 92(3), 783-799. https://doi.org/10.32604/phyton.2022.026045
Received 12 August 2022; Accepted 16 September 2022; Issue published 29 November 2022
Abstract
Fusarium dry rot is considered to be the most critical soilborne and postharvest disease that damages potato tubers worldwide when they are stored for a long time. This study was performed to demonstrate the effect of crude extract, culture filtrate, and cell suspension obtained from the bacterium Bacillus subtilis HussainT-AMU, on the net house and field. From oil-contaminated soil, through the serial dilution method, biosurfactant bacteria were isolated on nutrient agar medium. To isolate and screen the prospective biosurfactant strain, various biosurfactant screening methods were used. Standard protocols were carried out for morphological, molecular, and chemical characterization. The disease incidences were significantly decreased under net house and field conditions after treatment with biosurfactant extract (20%, 70%), culture filtrate (23%, 49%), and bacterial cell suspension (46%, 27%), respectively. These results indicate a significant effect of B. subtilis HussainT-AMU against Fusarium oxysporum. The characterization of the partially purified extract by gas-chromatography indicated the existence of a high amount of methyl ester fatty acids. The quantitative analysis determined the presence of lipopeptide-surfactin, fengycin, and iturin in the biosurfactant extract. Scanning electron microscopy revealed that morphological variation was revealed in the structure of stomata size in leaves and in the mycelia of the pathogen that was investigated. This promising biocontrol agent can be a plausible option for further research and can also be used for the production of green biomolecules at an additional industrial level.Keywords
The potato (Solanum tuberosum L.) is considered one of the essential crops in every human diet. Potatoes are currently grown on 19 million hectares of land, with a global yearly production of 388 million tonnes [1]. Around 1.3 billion people in India and China consume fresh potatoes as a basic diet (50 kg per person per year). Potato breeders’ major goal is to increase yield and nutrient quality while limiting field losses due to pests’ infection, diseases, and harsh environmental conditions. Potato production losses and decline in nutritional value are caused by a variety of factors, including pre- and post-harvest handling, frost, heavy rainfall fields, and storage disease [2]. More than 40 diseases, including bacteria, viruses, fungi, nematodes, and pests cause harm to potato foliar portions and tubers [3]. As a result, it is critical to manage and prevent diseases that cause substantial crop losses and a fall in farmer revenue [4]. In the Indian plains, various Fusarium species, including F. oxysporum Schlechtend, produce dry rot disease [5]. Dry rot affects crop stand by reducing potato sprout production, resulting in losses of up to 25% to 60% during storage [6]. Although dry rot is a severe issue for tubers in storage, also its negative impacts on potato plants appear in the field as wilting and root degeneration [7]. The wounds formed on the tubers throughout harvesting, handling, and shipping serve as entrance points for fungal spores, and infested tubers induce dry rot in storage. Only when the tuber skin ruptures can the fungus cause infection. The infectious hypha develops in intercellular spaces in living cells and then becomes intracellular only after the cell dies [8].
Moreover, Fusarium spp. also produces toxins like mycotoxins which due to their effects lead to mycotoxicosis. Therefore, infected tubers are harmful to human beings and animals [9]. Dry rot symptoms, which are characterized by sunken and wrinkled dark to black tissue patches on tubers, typically appear about 1–2 months after tuber storage. The wrinkled patches produce cottony white, purple, pink, or brick orange spores and mycelial masses that remain in the soil or rotting tuber debris during long-term storage [10]. If the affected tuber is utilized as seed material, it causes Fusarium wilt in fields. The pathogen inoculum is derived from infected seed tubers, which cause soil infestation [6]. The infections by Fusarium species depend on the storage conditions and cultivar species [10].
Chemical management strategies against dry rot in potatoes are not very effective and economically viable. Furthermore, the frequent use of synthetic agrochemical products leads to harmful residual effects on plants, the natural microflora of the soil and humans [11]. In the last decade, there has been a significant increase in knowledge and attention to using environmentally friendly strategies such as biocontrol techniques [3]. Nowadays, bio-control agents are gaining ground in the latest agricultural farming and practice as an alternative to chemical-based fungicides [12–17]. The Indian government is focusing on the manufacture of these eco-friendly bio fungicides for sustainable agricultural production. For long-term stability, effective biological approaches to control soil-borne pathogens and reduce disease, are the best-recommended solutions [17]. Due to its vast applications, biosurfactant is one of the main groups of microbial solutions with well-recognized biochemical properties [18–20]. Microbial surfactants that are a mixture of lipids, phospholipids, polysaccharides, lipoproteins, and other biological macromolecules are formed by several types of bacteria that encompass many functional groups, such as carboxyl, amino, and phosphate groups [21]. In this regard, lipopeptides produced by Bacillus subtilis are especially intriguing because of their high surface activity and therapeutic effects in comparison to many other forms of biosurfactants [22–23]. However, producing biosurfactants on a wider scale remains difficult and poses a considerable barrier to their commercialization [24].
Early and late blight were successfully overcome by the isolates of B. subtilis and Trichoderma harzianum [25]. Shafi et al. [26] reported that Bacillus species exhibited direct antibiosis and induced resistance against Fusarium spp., Pythium spp., and Rhizoctonai solani. Gliocladium catenulatum, an active element of a bio-fungicide, has exhibited good biocontrol efficacy against both seeds and soilborne diseases caused by Phytophthora spp., Pythium spp., Alternaria spp., Cladosporium spp., Rhizoctonia spp., Verticillium spp. in horticultural crops, and storage diseases caused by Penicillum spp., Helminthosporium solani and R. solani in potatoes [19–20]. The late blight disease in potatoes was constrained by the application of Chaetomium globosum [27]. Rhamnolipids, glycolipids, and lipopeptide biosurfactants are produced by the genera Pseudomonas, Candida and Bacillus that make up most of the reported biofilms [28–29].
The genus Bacillus sp. can tolerate extreme conditions of pH, temperature, and osmotic pressure [30]. These bioagents not only cause the lysis of many fungal pathogens mycelium but also colonize surfaces and promote plant growth activities [31]. The use of biological control approaches to combat soil-borne pathogens is related to soil health and pathogens [32]. Bacillus sp. is also used against postharvest pathogens [33–34]. Three novel cyclic lipopeptides (surfactin homologues) were produced by Bacillus sp. SY27F using liquid chromatography–tandem mass spectrometry (LC–MS–MS) and gas chromatography–mass spectrometry (GC–MS) [35].
For example, lipopeptides are commonly competent against Colletotrichum dematium [36], R. solani, Macrophomina phaseolina (Tassi) Goid [37], Pythium ultimum [38], Fusarium head blight [39] and root-knot nematode caused by Meloidogyne incognita [18]. Several studies used lipopeptides/rhamnolipids as viable fungicides in order to preserve numerous economically important crops [40]. Diversification in stomatal structure is commonly observed in all plant species [40] and it is proposed that reduced substomatal cavities induce less efficient absorption of CO2, leading to further unnecessary water loss during stomata opening and regulating gas exchange [41].
Therefore, we conducted this experiment to demonstrate the effect of the culture filtrate produced by B. subtilis HussainT-AMU strain in both pot studies at Net House and farmer fields. We also detected and characterized the fatty acid components of the biosurfactant using chromatography techniques.
2.1 Isolation of Biosurfactant Bacterial and Fungal Pathogen
The residual oil-contaminated soil sample was collected from Paradwip port in Odisha (India) at a depth of 5–10 cm for the isolation of bacteria using the serial dilution method [42]. The isolated bacteria were characterized according to their morphological and bio-chemical parameters by following the standard Bergey’s Manual of Determinative Bacteriology. For molecular characterization, 16S rRNA gene sequence was performed for sequence analysis that was outsourced through a private company.
Dry rot tubers, infected with Fusarium sp., were collected from the Haathras region, Uttar Pradesh (India), before the crop planting season in 2018. Isolation of fungi was performed based on the protocol of Hussien et al. [17]. The bacteria colonies were then purified by repeated striking, picking up a single colony and maintaining them on nutrient agar slants at 4°C.
2.2 Extraction of Biosurfactant Production
Bacterial cells were removed by centrifugation at 12,000 rpm for 10 min from 1 L of nutrient broth medium (0.1% petrol mixed), and the extract was obtained from the culture filtrate. The pH of the culture filtrate was brought to 2N with HCl and kept overnight at 4°C, with an equal volume of chloroform: Methanol (2:1) was mixed with the overnight culture filtrate, vigorously stirred for several minutes, and allowed to set until the phase separated. The chloroform was evaporated at 50°C using a rotary evaporator, and the biosurfactant extract was concentrated. The resulting honey-coloured material was considered a crude extract. The experiment was carried out in triplicate.
The bio-efficacy of B. subtilis HussainT-AMU strain was tested against Fusarium dry rot using the dual culture method. Sterile cork-borer from a seven-day-old new culture of Fusarium sp. mycelial discs (5 mm) was set at the periphery of the Petri-dishes containing sterilized potato dextrose agar media (P.D.A.). The new bioagent was streaked on the opposite side of the Petri dish at an identical distance. Three replicates were maintained. All the Petri dishes were placed at 26°C ± 2°C in a dark Biological Oxygen Demand (B.O.D.) incubator until the radial growth of pathogens (control-without-treatment) Petri-dishes were completely full. Aneja [43] method was used to calculate the percentage (%) suppression of test pathogens at various time periods (24, 48, 72, and 96 h). The study was repeated three times.
2.4 Experimental Procedure and Treatments
Antagonistic activity of B. subtilis HussainT-AMU was investigated using seed tubers of cv. Kufri Bahar consisting of five treatments viz; T1-Healthy seed tubers, T2-Infected control (Positive), T3-Culture filtrate of B. subtilis HussainT-AMU, T4-B. subtilis HussainT-AMU cell suspension 1–109 CFU/mL, and T5-Carbendazim 50% WP (0.3%) treated 24 h before plantation. Under the net house, the treated tubers were planted in 30 cm diameter earthen pots. The number of tubers and yield (g/plant) were recorded at harvest time. The prevalence of the disease, its severity, and its excess over control were assessed [44]. The field trial was conducted in an agricultural field owned by a farmer in Haathras, Uttar Pradesh, during the cropping season (2019–2020). A 3 × 3 m plot size was used to be laid out in an (randomized block designs) RBD. All agronomic work was carried out in accordance with the region’s recommendations, with seedings spaced 60 × 20 cm apart. The three replications of the study’s treatments were used as the standard. To show severe infection, the potato tubers were treated with different formulations 24 h prior to planting. Untreated black scurf-infected tubers and seed tubers that appeared healthy were kept as a source of control.
According to the pot study, observations on disease incidence and severity were made at the time of harvest. Crops were harvested two weeks after dehaulming, and haulms were cut more than 100 days after planting. At harvest, 230 tubers (roughly 75 tubers per replication) were randomly selected from each treatment to track the incidence and severity of the disease. On a scale of 0 to 4, the severity of the disease was determined (0 being no disease, 1 being 1% to 25% of the surface area covered, 2 being 26% to 50%, 3 being 51% to 75% covered, and 4 being >75% covered). According to the study, observations on the prevalence and severity of the diseases were made at the time of harvest.
2.5 Gas Chromatography-Mass Spectroscopy (GC-MS)
Fatty acid methyl esters were carried out as per standard protocol followed at ARIF Center at the J.N.U., New Delhi according to the method of Somani [45]. The biosurfactant extract was filtered using 0.45 µm micron filters under laminar flow, dissolved in methanol. Fatty acid methyl esters analysis was carried out on an Agilent Model 7890A gas chromatograph fitted to a 5975C mass detector. Helium (≥99.99%) as a carrier gas was applied with a flow rate of 1 mL/min. A total of 10 μL of the sample was injected into the system. The oven temperature was kept within the range of 60°C–260°C. To evaluate the fatty acid component in the extract, major fatty acids were studied by comparing them to the retention times of authentic standards (Wiley 8) and the National Institute of Standards and Technology (NIST) mass spectrum library database.
2.6 Qualitative Analysis through Liquid Chromatography-Mass Spectrometry (LC-MS)
The partially purified collected biosurfactant, which was solubilized in methanol, was included in the qualitative approach, which was performed using LC-MS, electrospray ionization mass spectrometry (ESI-MS) at the Sophisticated Analytical Instrumental Facility (SAIF) center, CSIR-Central Drug Research Institute, Lucknow, Uttar Pradesh, according to their standard procedures. The filter-purified lipopeptide extract was immediately injected into the Mass Spectrometer (LTQ XL Linear Ion Trap Mass Spectrometer) using a direct insertion pump equipped with an E.S.I. source (Thermo Scientific, USA) at a flow rate of 10 L min−l in the sample. The sheath gas flow (N2) and capillary temperature were tuned to 350°C and 30 arbitrary units, respectively, in both scan modes. The results of a complete ion scan with a mass scan range of m/z 50 ± 2000 were negative.
2.7 Scanning Electron Microscopy (S.E.M.)
Scanning electron microscopy was used to show the influence of the new bioagent’s biosurfactant extract, culture filtrate, and bacterial cell suspension on the morphological structure of the stomata and the mycelia of the pathogen. Leaf and mycelia samples were gathered for each treatment; 55 mm leaf samples were cut and randomly set. The samples were fixed for 24 h at 4°C in 3% glutaraldehyde (100 mM, pH 6.8). The specimens were then washed in phosphate buffer (pH 6.8) for 2–3 h before being dehydrated in a graded ethanol-acetone series. Dehydration started with a series of ethanol concentrations (30%–90%), then two variations through absolute ethanol and twice through pure acetone, with the sample staying 30 s at each step. The specimens were then dried using CO2 as a transition fluid, and the dry samples were placed using double-stick tape over copper stubs before being covered in a sputter coater with roughly 20 nm gold particles. Specimens were examined using an electron microscope with a scanning attachment set to 20 kV. To confirm the findings, at least three randomly selected samples were maintained from each batch. Changes in the pathogen mycelia and stomata morphology seen in the S.E.M. were caught and recorded as an image.
IRRISTAT software (version 4.4.20030719) (India) was used to evaluate the experimental data.
3.1 Isolation and Production of Biosurfactant Bacterial and Fungal Pathogen
A new biosurfactant strain of Bacillus was isolated from Pradwip, Odisha. The 16S rRNA gene sequence alignment (Table 1) confirmed that the ODI-02 strain had the highest similarity index (99%) to Bacillus subtilis, and the sequence was named B. subtilis HussainT-AMU with accession No. LC385950 and deposited in the NCBI database (Fig. 1). Identification was confirmed based on morphological characteristics of conidia. Conidiophores develop from mycelium and form 1–4 celled sickle-shaped conidia of varying sizes. Every two months, the pathogen’s pure fungal culture was kept on P.D.A. at 4°C.
Figure 1: Phylogenetic tree showing the relationship between B. subtilis HussainT-AMU and other Bacillus spp.
3.2 The Bio-Efficacy of B. subtilis HussainT-AMU Strain Using the Dual Culture Method
Under the dual culture study, the new bioagent B. subtilis HussainT-AMU reduced the growth of the pathogen mycelium. A significant level of pathogen growth inhibition was noted (Fig. 2). The new Bacillus strain produced antifungal peptide antibiotic compounds, that significantly reduced the phytotoxicity of Fusarium oxysporum by Bacillus.
Figure 2: Dual culture study with the new bioagent B. subtilis HussainT-AMU strain
3.3 Effect of B. subtilis HussainT-AMU against Fusarium under Pot and Field Conditions
Biosurfactant extract, culture filtrate, and bacterial cell suspension significantly reduced the disease incidence by (20%, 70%), (23%, 49%) and (46%, 27%) respectively under net house and field conditions, in comparison to 97% of infected control (Table 2). The disease incidence was greater in the untreated tuber plants (controls) when compared to healthy plants and this incidence boosted the duration of infection time. The maximum number of tubers per plant was noticed with Carbendazim 50% W.P. treated seed tubers, followed by biosurfactant extract (26.88 t/ha), cultural filtrate (25.60 t/ha), and bacterial cell suspension (23.20 t/ha). Tuber yield was obtained from extract and culture filtrate treatment after harvesting.
3.4 Fatty Acids Composition by GC-MS
To detect the effects of possible changes and structural adaptation of membrane lipids in lipopeptide producer B. subtilis HussainT-AMU, fatty acid analysis was carried out through GC-MS (Fig. 3). (1) 9,12-Octadienoyl chloride, (Z, Z)-cis-9 (Retention Time: 24.90, 35.70%) was present in high amounts in comparison to other fatty acids, (2) 9-Octadecenoic acid,1,2,3-propanetriyl ester, (E, E, E) (R.T.: 44.01, 7.14%), (3) 9,12-Octadecadienoic acid, methyl ester (R.T.: 24.30, 5.905), (4) 8,11, 14-Docosatrienoic acid, methyl ester (R.T.: 24.380, 5.00%), (5) n-Hexadecanoic acid, methyl ester (R.T.: 22.75, 3.71%), (6) 9,12-Octadecadienoyl chloride, (Z, Z) (R.T.: 28.634, 3.455%), and (7) n-Hexadecanoic acid, methyl ester (R.T.: 22.28, 3.28%) were the leading fatty acids we studied in the B. subtilis HussainT-AMU strain (Table 3).
Figure 3: GC-MS of biosurfactant collected from B. subtilis HussainT-AMU
Based on the gas chromatogram investigation, the hydrocarbon ranges, between C10–C29, which may be due to petrol oil, were used as a significant carbon source. More specifically, the novel biosurfactant strain completely wrecked several low molecular weight molecules ranging from C10 to C14. Molecules with C15–C19 ranges were about 22% degraded; other high molecules were around 78% degraded, indicating that B. subtilis HussainT-AMU has an alkane hydroxylase enzyme to destroy the several alkane ranges.
3.5 Lipopeptide from B. subtilis HussainT-AMU Strain Using LC-MS
Lipopeptides from the B. subtilis HussainT-AMU strain were analyzed using liquid chromatography-mass spectrometry. It indicated the appearance of surfactin and fengycin peaks, which were more intense, and the peak of iturin, which was minor ample (Figs. 4 and 5). The electrospray ionization mass spectrometry (ESI-MS) study revealed that the first set of signal peaks corresponded to the surfactin family at m/z 780, 934, 960, and 1,068 and that fragile [M+H]+ signals of these surfactin variations existed at m/z 934, 960, 1,068, and 1,069. These peaks indicated that isomers were near to C12, C13, C14, C16, and C17 acyl chains. There was also a scarcity of iturin peaks, which were detected as 1,070, 1,085, and 1,091 closeness of isomers to C16, C17 acyl chains. The fengycin (antifungal agent) family is represented by the third set of peaks. The molecular weights of these molecules correlate with those of fengycin A and B, and with C14 and C18 acyl chains (Table 4).
Figure 4: MS-ESI scan of lipopeptides produced by B. subtilis HussainT-AMU strain
Figure 5: Chemical structures of antibiotics
3.6 The Effect of Treatments on the Morphology of Stomata Using SEM
The morphology of the stomata size changed when treated with the culture filtrate-dipped potato leaves in comparison to the cell culture suspension treatment. The stomata in the untreated potato tubers were small and diminished in size (Fig. 6).
Figure 6: Scanning electron microscopy of stomata morphology observed in potato leaves. (A) Seed tubers treated with culture filtrate, (B) Seed tubers treated with bacterial cell suspension, (C) Crude biosurfactant extract treated tubers (D) Fusarium sp. infected tubers without any treatment as positive control
3.7 The Effect of Treatments on the Morphology of Mycelium of Fusarium Using SEM
In many asexual reproductions and diseases, evolution mycelium is the vegetative structure of a fungus that plays a crucial role. Untreated mycelium of Fusarium sp. displayed general/simple morphology with identical thickness and shape, whereas mycelium treated with crude extract exhibited an irregular shape with a rough surface and ruptured. Treated mycelium was severely reduced in thickness compared to the untreated (Fig. 7).
Figure 7: Scanning electron microscopy images of (A) untreated mycelial of Fusarium sp., and (B) mycelia treated with bio surfactant extract
Pathogens of potatoes such as Rhizoctonia spp., Fusarium spp., Verticillium spp., Sclerotinia spp., Pythium spp. and Phytophthora spp. can reduce crop performance and decrease yield, thus adding higher production costs. Furthermore, pathogens persist in the soil for a long time and attack tubers, the most economically vital part of the plant [32]. In recent years, research and development for production through fermentation and its heterogenous utilization in numerous fields, such as safeguarding the environment, has steadily increased the interest in the biosurfactant sector. For use in multiple environmental applications, specificity with remarkable structural diversity, the potential for profitable ex-situ production and its biodegradability are some of the features that make biosurfactant a promising option [46].
A new biosurfactant strain of Bacillus was isolated from Pradwip, Odisha [47]. Multiple species of the genus Fusarium cause rotting of potato seed tubers worldwide [48]. F. solani, F. graminearum and F. oxysporum Schlechtend are the most observed in the plains of India. On P.D.A. medium, it produced morphological characteristics that are similar to those described by Srivastava et al. [49].
The reduction in pathogen growth could be due to the production of antifungal antibiotic compounds secreted by the bacteria. Upadhyay et al. [50] reported that bioagents like B. cereus, B. licheniformis and B. subtilis produced some antibiotics or other inhibitory compounds against several post-crop pathogens. Similarly, the in vitro antagonistic ability of the bacterial isolates like B. subtilis, Pseudomonas fluorescens was studied using a dual culture [51]. Biocontrol agents reduce soilborne plant infections by parasitism, the generation of aggressive compounds, competition for the host and nutrients, and the development of disease-causing pathogen defense in plants [52]. Our results are consistent with these findings.
The disease severity decreased significantly after treatment with biosurfactant extract, culture filtrate, and bacterial cell suspension. Our results agree with previous findings reported by Hussain et al. [3] where similar evidence was noticed in other isolates of Fusarium spp. It was evaluated with some Bacillus isolates when it was included in P.D.A. and its efficacy was higher than Thiabendazole. B. subtilis develops adherent biofilms on inert surfaces, which are influenced by a number of conditions, such as the release of lipopeptides that have antimicrobial characteristics [53] which was also observed in our study. Biofilms are structured communities of cells that cling to a surface and are surrounded by an extracellular polymeric matrix [54]. The biocontrol technique employing B. subtilis is based on the creation of protective biofilms [55], with its potential to produce structurally varied secondary metabolites with a wide range of antibiotic action [56]. The current research revealed the existence of many interrelationships, in vitro and in vivo, between Fusarium and antifungal action by the new biosurfactant bioagent. Bacillus sp. is characterized not only by a decrease in vitro growth, but also by many other mechanisms, including morphological changes, severe lymphatic, mycelia cable, and reduced mycelial density, all unusual events observed under stress in untreated controls and Fusarium sp.
Branch chains, as well as iso- and anteiso-fatty acids, were the primary components of lipids. The branched-chain fatty acid is the predominant cellular fatty acid found in abundance in Bacillus species [57]. A similar experience was observed with B. subtilis HussainT-AMU strain, examined in our present findings as well as summarizing lipopeptide nature. This variance in lipopeptide production could be due to variations in fatty acid compounds that depend not only on the generating bacteria but also on the culture conditions and substrate employed.
The lipopeptide-based biosurfactant consists of fatty acids like octadecanoic acid and 9-octadecenoic acid as significant components as reported by Ibrahim et al. [58], this result is consistent with our findings. The GC-MS analysis performed in our present study detected that the biosurfactant compounds were lipopeptides and hexadecenoic acid was also found in a small amount. These results are in accordance with Parthipan et al. [24]. In addition, Bezza et al. [59] detected that gram-positive B. subtilis A1 can synthesize lipopeptide biosurfactants that efficiently absorb hydrocarbons in crude oil. Ibrahim et al. [58] found several bacterial species associated with oil-degrading biosurfactants, such as Achromobacter sp., Bacillus sp., Serratia sp., Sphingomonas sp., and Micrococcus sp. Moreover, much research has demonstrated that most hydrocarbon-degrading bacteria can readily use alkanes in the C14–C20 range as an energy source [24]. Several reports have also described a combination of b-hydroxy fatty acids of dodecanoic, tetradecanoic, pentadecanoic, hexadecenoic, octadecanoic, (9)-octadecenoic and (9,12)-octadecadienoic in the lipopeptide biosurfactant [60], which was confirmed with our study. Saturated fatty acids have been reported to exert inhibitory activity towards some fungal species; hydrophobic groups of SFA seem to play a role in the antimicrobial activity. However, long chain fatty acids have a reduced solubility in aqueous environments, due to the high number of hydrophobic groups, while shorter chain fatty acids may not have enough hydrophobic groups to interact with the cell membrane. Events leading to fungi sensitivity to this fatty acid were, sequentially: (i) partitioning of the fatty acid into fungal membranes, (ii) increase in membrane fluidity, dependence of sterol content, leading to higher membrane disorder, causing conformational changes in membrane proteins, and (iii) ultimately, cytoplasmatic disintegration [61].
Furthermore, biosurfactants boost the bioavailability of hydrophobic compounds in soil, which can function as nutrients and aid plant growth and development [62]. The plant’s resistance to pathogens can be improved by the application of different biological and/or green-chemical agents. In addition, the application of biocontrol agents with chemical inducers can significantly improve plants resistance, enhance growth, resistance to pathogen infections, and increase yield [62].
Fengycin is a strong antifungal active molecule compared to surfactin and finding this lipopeptide corroborates our results. These results are in accordance with those of Desmyttere et al. [63] who found that fengycin, surfactin, and mycosubtilin are synthesized by Bacillus subtilis and all lipopeptides exhibited antifungal potential against Venturia inaequalis that causes apple scab. These LCMS-ESI findings are similar to the previous studies reported by Ali et al. [64], Sarwar et al. [65].
Because different Bacillus strains biosynthesized different lipopeptides, their biocontrol effectiveness vary against different phytopathogens [66]. As a result, surfactants (both synthetic and biological) play a distinct role in plant-pathogen destruction, either directly or indirectly, and at a variety of agricultural stages [67]. All of the biofilm-forming bacteria were also biosurfactant active. Surfactin synthesis was discovered to be crucial for biofilm formation and may be a key characteristic for efficient B. subtilis bio-control strains. This finding implies that biofilm development may help B. subtilis HussainT-AMU to colonize plant roots more effectively [68].
The overall boost in stomatal size will also result in enhanced CO2 uptake, improved water usage efficiency, and result in an enhancement in tuber yield or biomass [69]. These results imply that B. subtilis HussainT-AMU crude extract, and culture filtrate function may have an effect on stomatal function. Therefore, any damage to the integrity of the mycelium can adversely affect the pathogenesis of a fungus. In this sense, SEM was performed to evaluate the effect of crude extract on the mycelium of F. oxysporium. The supply of surfactant and, therefore, lipopeptide significantly changed the morphology of fungus mycelia which agrees with the previous studies by Park et al. [70] who found that the mycelium surface of Colletotrichum gloeosporioides treated with surfactin was rugged and unusually swollen and rough, implying that the peptide directly impacts the fungal cell wall components (chitin, glucans, and glycoproteins) to exert antifungal action. This damage leads to the leakage of electrolytes, proteins, and DNA due to the surfactant activity caused by the blockage of the phospholipid bilayer of the membrane cell [70].
To our knowledge, the present study reports for the first-time essential findings based on the application of biosurfactant bioagent for the management of Fusarium dry rot of potato tubers. Following treatment with biosurfactant extract, culture filtrate, and bacterial cell suspension, the disease incidences were significantly reduced in both the net house and the field. These findings show that B. subtilis HussainT-AMU significantly inhibits F. oxysporum growth. Gas chromatography analysis of the partially purified extract revealed a significant presence of methyl ester fatty acids. The biosurfactant extract contained iturin, fengycin, and lipopeptide-surfactin, according to the quantitative analysis. The pathogen under investigation exhibits morphological variation in the structure of stomata size in leaves and in its mycelia, according to SEM.
Authorship: The authors confirm contribution to the paper as follows: study conception and design: T.H; data collection: T.H; analysis and interpretation of results: T.H; draft manuscript preparation: T.H, A.A.K, H.I.M. All authors reviewed the results and approved the final version of the manuscript.
Acknowledgement: First Author is very thankful to the SERB-Department of Science and Technology, Government of India, New Delhi, for providing the financial start-up grants received under File No. DST-SERB-NPDF/2016/001409 and Department of Botany, Aligarh Muslim University, Aligarh, U.P., for providing all the facilities and, infrastructure. The author is also thankful to ARIF Centre at J.N.U., New Delhi for providing GC-MS facility, UISEF, at A.M.U., Aligarh for S.E.M. facility, SAIF Center for LC-MS, at CSIR-CDRI, Lucknow, U.P.
Funding Statement: Science and Engineering Research Board (SERB) Govt. of India, New Delhi, under File No. SERB-DST-NPDF/2016/001409.
Conflicts of Interest: The authors declare that they have no conflicts of interest to report regarding the present study.
References
1. FAOSTAT (2019). Food and agriculture data. https://www.fao.org/faostat/en/#data/Qcinfo. [Google Scholar]
2. Tiwari, R. K., Kumar, R., Sharma, S., Naga, K. C., Subhash, S. et al. (2021). Continuous and emerging challenges of silver scurf disease in potato. International Journal of Pest Management, 68(1), 89–101. DOI 10.1080/09670874.2020.1795302. [Google Scholar] [CrossRef]
3. Hussain, T., Khan, A. A., Mohamed, H. I. (2022). Potential efficacy of biofilm-forming biosurfactant Bacillus firmus HussainT-Lab.66 against Rhizoctonia solani and mass spectrometry analysis of their metabolites. International Journal of Peptide Research & Therapeutics, 28(1), 3. DOI 10.1007/s10989-021-10318-5. [Google Scholar] [CrossRef]
4. Lastochkina, O., Baymiev, A., Shayahmetova, A., Garshina, D., Koryakov, I. et al. (2020). Effects of endophytic Bacillus subtilis and salicylic acid on postharvest diseases (Phytophthora infestans, Fusarium oxysporum) development in stored potato tubers. Plants, 9(1), 76. DOI 10.3390/plants9010076. [Google Scholar] [CrossRef]
5. Tiwari, R. K., Kumar, R., Sharma, S., Sagar, V., Aggarwal, R. et al. (2020). Potato dry rot disease: Current status, pathogenomics and management. 3 Biotech, 10(11), 1–8. DOI 10.1007/s13205-020-02496-8. [Google Scholar] [CrossRef]
6. Heltoft, P., Brierley, J. L., Lees, A. K., Sullivan, L., Lynott, J. et al. (2016). The relationship between soil inoculum and the development of Fusarium dry rot in potato cultivars Asterix and Saturna. European Journal of Plant Pathology, 146(3), 711–714. DOI 10.1007/s10658-016-0946-2. [Google Scholar] [CrossRef]
7. Bojanowski, A., Avis, T. J., Pelletier, S., Tweddell, R. J. (2013). Management of potato dry rot. Postharvest Biology Technology, 84, 99–109. DOI 10.1016/j.postharvbio.2013.04.008. [Google Scholar] [CrossRef]
8. Xue, H., Yang, Z. (2021). Potato dry rot caused by Fusarium spp. and mycotoxins accumulation and management. In: Fusarium–An overview of the genus. UK, London: IntechOpen. DOI 10.5772/intechopen.100651. [Google Scholar] [CrossRef]
9. Chen, D., Nahar, K., Bizimungu, B., Soucy, S., Peters, R. D. et al. (2020). A Simple and efficient inoculation method for fusarium dry rot evaluations in potatoes. American Journal of Potato Research, 97(3), 265–271. DOI 10.1007/s12230-020-09774-4. [Google Scholar] [CrossRef]
10. Vatankhah, M., Saberi Riseh, R., Moradzadeh Eskandari, M., Sedaghati, E., Alaie, H. et al. (2019). Biological control of Fusarium dry rot of potato using some probiotic bacteria. Journal of Agricultural Science & Technology, 21(5), 1301–1312. [Google Scholar]
11. Fothergill, A., Abdelghani, A. (2013). A review of pesticide residue levels and their related health exposure risks. Food & Environment, 170, 1–11. DOI 10.2495/FENV130181. [Google Scholar] [CrossRef]
12. Lahlali, R., Ezrari, S., Radouane, N., Kenfaoui, J., Esmaeel, Q. et al. (2022). Biological control of plant pathogens: A global perspective. Microorganisms, 10(3), 596. DOI 10.3390/microorganisms10030596. [Google Scholar] [CrossRef]
13. Sofy, M. R., Mohamed, H. I., Dawood, M. F. A., Abu-Elsaoud, A. M., Soliman, M. H. (2021). Integrated usage of Trichoderma harzianum and biochar to ameliorate salt stress on spinach plants. Achieves of Agronomy & Soil Science, 17, 1–22. DOI 10.1080/03650340.2021.1949709. [Google Scholar] [CrossRef]
14. Youssef, K., Roberto, S. R., Tiepo, A. N., Constantino, L. V., de Resende, J. T. V. et al. (2020). Salt solution treatments trigger antioxidant defense response against gray mold disease in table grapes. Journal of Fungi, 6(3), 179. DOI 10.3390/jof6030179. [Google Scholar] [CrossRef]
15. Youssef, K., Hashim, A. F. (2020). Inhibitory effect of clay/chitosan nanocomposite against Penicillium digitatum on citrus and its possible mode of action. Jordan Journal of Biological Sciences, 13(3), 349–355. [Google Scholar]
16. Lachhab, N., Sanzani, S. M., Fallanaj, F., Youssef, K., Nigro, F. et al. (2015). Protein hydrolysates as resistance inducers for controlling green mould of citrus fruit. Acta Horticulturae, 1065, 1593–1598. DOI 10.17660/ActaHortic.2015.1065.203. [Google Scholar] [CrossRef]
17. Hussien, A., Ahmed, Y., Al-Essawy, A. H., Youssef, K. (2018). Evaluation of different salt-amended electrolysed water to control postharvest moulds of citrus. Tropical Plant Pathology, 43(1), 10–20. DOI 10.1007/s40858-017-0179-8. [Google Scholar] [CrossRef]
18. Hussain, T., Khan, A. A., Tomar, S. (2019). Biocontrol of dry rot of potato seed tubers caused by Fusarium sp. by using novel biosurfactant bacteria Bacillus firmus HussainT-AMU: Lab.66 producing cyclic lipopeptides. Indian Plant Science Congress, pp. 23–25. Kattankulathur, TN, India. [Google Scholar]
19. Hussain, T., Haris, M., Shakeel, A., Khan, A. A., Khan, M. A. (2020). Bio-nematicidal activities by culture filtrate of Bacillus subtilis HussainT-AMU: New promising biosurfactant bioagent for the management of root galling caused by Meloidogyne incognita. Vegetos, 33(2), 229–238. DOI 10.1007/s42535-020-00099-5. [Google Scholar] [CrossRef]
20. Hussain, T., Khan, A. A. (2020a). Determining the antifungal activity and characterization of Bacillus siamensis AMU03 against Macrophomina phaseolina (Tassi) goid. Indian Phytopathology, 73(3), 507–516. DOI 10.1007/s42360-020-00239-6. [Google Scholar] [CrossRef]
21. Hussain, T., Khan, A. A. (2020b). Bacillus firmus Hussain T: Lab. 66, a new biosurfactant bioagent having potential bio-nematicidal activity against root knot nematode Meloidogyne incognita. National Conference on Recent Advances in Biological Science (NCRABS-2020), pp. 42. India, New Delhi. [Google Scholar]
22. Vijayakumar, S., Saravanan, V. (2015). Biosurfactants-types, sources and applications. Research Journal of Microbiology, 10(5), 181–192. DOI 10.17311/jm.2015.181.192. [Google Scholar] [CrossRef]
23. Koo, H., Allan, R. N., Howlin, R. P., Stoodley, P., Hall-Stoodley, L. (2017). Targeting microbial biofilms: Current and prospective therapeutic strategies. Nature Reviews Microbiology, 15(12), 740–755. DOI 10.1038/nrmicro.2017.99. [Google Scholar] [CrossRef]
24. Parthipan, P., Preetham, E., Machuca, L. L., Rahman, P. K. S. M., Murugan, K. et al. (2017). Biosurfactant and degradative enzymes mediated crude oil degradation by bacterium Bacillus subtilis A1. Frontiers in Microbiology, 8, 193. [Google Scholar]
25. Chowdappa, P., Mohan, K. S. P., Jyothi, L. M., Upreti, K. K. (2013). Growth stimulation and induction of systemic resistance in tomato against early and late blight by Bacillus subtilis OTPB1 or Trichoderma harzianum OTPB3. Biological Control, 65(1), 109–117. [Google Scholar]
26. Shafi, J., Tian, H., Ji, M. (2017). Bacillus species as versatile weapons for plant pathogens: A review. Biotechnology & Biotechnological Equipment, 31(3), 446–459. DOI 10.1080/13102818.2017.1286950. [Google Scholar] [CrossRef]
27. Shanthiyaa, V., Saravanakumar, D., Rajendran, L., Karthikeyan, G., Prabakar, K. et al. (2013). Use of Chaetomium globosum for biocontrol of potato late blight disease. Crop Protection, 52, 33–38. [Google Scholar]
28. Mnif, I., Ellouz-Chaabouni, S., Ghribi, D. (2018). Glycolipid biosurfactants, main classes, functional properties and related potential applications in environmental biotechnology. Journal of Polymers and the Environment, 26(5), 2192–2206. DOI 10.1007/s10924-017-1076-4. [Google Scholar] [CrossRef]
29. Aleksic, I., Petkovic, M., Jovanovic, M., Milivojevic, D., Vasiljevic, B. et al. (2017). Anti-biofilm properties of bacterial di-rhamnolipids and their semi-synthetic amide derivatives. Frontiers in Microbiology, 8, 2454. [Google Scholar]
30. Dricks, A. (2004). The Bacillus spore coat. Phytopathology, 94(11), 1249–1251. [Google Scholar]
31. Jha, Y., Mohamed, H. I. (2022). Inoculation with Lysinibacillus fusiformis strain YJ4 and Lysinibacillus sphaericus strain YJ5 alleviates the effects of cold stress in maize plants. Gesunde Pflanzen. DOI 10.1007/s10343-022-00666-7. [Google Scholar] [CrossRef]
32. Panth, M., Hassler, S. C., Baysal-Gurel, F. (2020). Methods for management of soilborne diseases in crop production. Agriculture, 10(1), 16. DOI 10.3390/agriculture10010016. [Google Scholar] [CrossRef]
33. Lastochkina, O., Seifikalhor, M., Aliniaeifard, S., Baymiev, A., Pusenkova, L. et al. (2019). Bacillus spp.: Efficient biotic strategy to control postharvest diseases of fruits and vegetables. Plants, 8(4), 97. DOI 10.3390/plants8040097. [Google Scholar] [CrossRef]
34. Gao, H., Xu, X., Dai, Y., He, H. (2016). Isolation, identification and characterization of Bacillus subtilis CF-3, a bacterium from fermented bean curd for controlling postharvest diseases of peach fruit. Food Science & Technology Research, 22(3), 377–385. DOI 10.3136/fstr.22.377. [Google Scholar] [CrossRef]
35. Zhou, H., Cong, B., Tian, Y., He, Y., Yang, H. (2019). Characterization of novel cyclic lipopeptides produced by Bacillus sp. SY27F. Process Biochemistry, 83, 206–213. [Google Scholar]
36. Hiradate, S., Yoshida, S., Sugie, H., Yada, H., Fuji, Y. (2002). Mulberry anthracnose antagonists (iturins) produced by Bacillus amyloliquefaciens RC-2. Phytochemistry, 61(6), 693–698. [Google Scholar]
37. Hussain, T., Khan, A. A. (2018). Biological control of black scurf of potato caused by Rhizoctonia solani using lipopeptides produced by novel biosurfactant bacteria: Bacillus subtilis HussainT-AMU isolated from Paradwip port, India. National Conference on Biointensive Approaches in Plant Protection Strategies and Their Socio-Economic Impacts. Department of Plant Protection, Aligarh Muslim University, Aligarh, India under Indian Phytopathological Society, pp. 31. Aligarh, India. [Google Scholar]
38. Ongena, M., Jacques, P., Toure, Y., Destain, J., Jabrane, A. et al. (2005). Involvement of fengycin-type lipopeptidesin the multifaceted biocontrol potential of Bacillus subtilis. Applied Microbiology & Biotechnology, 69, 29–38. [Google Scholar]
39. Dunlap, C. A., Bowman, M. J., Schisler, D. A. (2013). Genomic analysis and secondary metabolite production in Bacillus amyloliquefaciens AS 43.3: A biocontrol antagonist of Fusarium head blight. Biological Control, 64(2), 166–175. [Google Scholar]
40. Goswami, D., Handique, P. J., Deka, S. (2014). Rhamnolipid biosurfactant against Fusarium sacchari—The causal organism of pokkah boeng disease of sugarcane. Journal of Basic Microbiology, 54(6), 548–557. [Google Scholar]
41. Patel, M., Siddiqui, A. J., Hamadou, W. S., Surti, M., Awadelkareem, A. M. et al. (2021). Inhibition of bacterial adhesion and antibiofilm activities of a glycolipid biosurfactant from Lactobacillus rhamnosus with its physicochemical and functional properties. Antibiotics, 10(12), 1546. [Google Scholar]
42. Lawson, T., Vialet-Chabrand, S. (2019). Speedy stomata, photosynthesis and plant water use efficiency. New Phytologist, 221(1), 93–98. DOI 10.1111/nph.15330. [Google Scholar] [CrossRef]
43. Aneja, K. R. (2003Experiments in microbiology, plant pathology and biotechnology, 4th edition, pp. 632. India: New Age International (P) Ltd. [Google Scholar]
44. Aly, A. A., El-Mahdy, O. M., Habeb, M. M., Elhakem, A., Asran, A. A. et al. (2022). Pathogenicity of Bacillus strains to cotton seedlings and their effects on some biochemical components of the infected seedlings. The Plant Pathology Journal, 38(2), 90–101. [Google Scholar]
45. Somani, A. K. (1986). Non-hazardous chemical control of black scurf of potato. Indian Journal of Agriculture Science, 56(5), 366–369. [Google Scholar]
46. Ongena, M., Jacques, P. (2008). Bacillus lipopeptides: Versatile weapons for plant disease biocontrol. Trends Microbiology, 16(3), 115–125. [Google Scholar]
47. Hussain, T., Khan, A. A. (2020c). Bacillus subtilis HussainT-AMU and its antifungal activity against Potato Black scurf caused by Rhizoctonia solani. Biocatalysis and Agricultural Biotechnology, 9, 2363. DOI 10.3389/fmicb.2018.02363. [Google Scholar] [CrossRef]
48. Lucas, J. A. (2017). Fungi, food crops, and biosecurity: Advances and challenges. Advances in Food Security and Sustainability, 2(1), 1–40. DOI 10.1016/bs.af2s.2017.09.007. [Google Scholar] [CrossRef]
49. Srivastava, S., Kadooka, C., Uchida, J. Y. (2018). Fusarium species as pathogen on orchids. Microbiological Research, 207, 188–195. [Google Scholar]
50. Upadhyay, R. S., Jayaswal, R. K. (1992). Pseudomonas cepacia causes mycelial deformities and inhibition of conidiation in phytopathogenic fungi. Current Microbiology, 24, 181–187. [Google Scholar]
51. Basco, M. J., Bisen, K., Keswani, C., Singh, H. B. (2017). Biological management of fusarium wilt of tomato using biofortified vermicompost. Mycosphere, 8(3), 467–483. [Google Scholar]
52. Shafique, H. A., Sultana, V., Ehteshamul-Haque, S., Athar, M. (2017). Management of soilborne diseases of organic vegetables. Journal of Plant Protection Research, 56(3), 221–230. [Google Scholar]
53. Stanley, N. R., Britton, R. A., Grossman, A. D., Lazazzera, B. A. (2003). Identification of catabolite repression as a physiological regulator of biofilm formation by Bacillus subtilis by use of D.N.A. microarrays. Journal of Bacteriology, 185(6), 1951–1957. [Google Scholar]
54. Annous, B. A., Fratamico, P. M., Smith, J. L. (2009). Quorum sensing in biofilms: Why bacteria behave the way they do. Journal of Food Science, 74(1), R24–R37. [Google Scholar]
55. Cairns, L. S., Hobley, L., Stanley-Wall, N. R. (2014). Biofilm formation by Bacillus subtilis: New insights into regulatory strategies and assembly mechanisms. Molecular Microbiology, 93(4), 587–598. [Google Scholar]
56. Liu, B., Qiao, H., Huang, L., Buchenauer, H., Han, Q. et al. (2009). Biological control of take-all in wheat by endophytic Bacillus subtilis E1R-j and potential mode of action. Biological Control, 49(3), 277–285. [Google Scholar]
57. Diomande, S. E., Guinebretière, M. H., Broussolle, V., Brillard, J. (2015). Role of fatty acids in Bacillus environmental adaptation. Frontiers in Microbiology, 6, 813. [Google Scholar]
58. Ibrahim, M. L., Ijah, U. J. J., Manga, S. B., Bilbis, L. S., Umar, S. (2013). Production and partial characterization of biosurfactant produced by crude oil degrading bacteria. International Biodeterioration and Biodegradation, 81, 28–34. [Google Scholar]
59. Bezza, F. A., Chirwa, E. M. N. (2015). Production and applications of lipopeptide biosurfactant for bioremediation and oil recovery by Bacillus subtilis CN2. Biochemical Engineering Journal, 101, 168–178. [Google Scholar]
60. Guimarães, A., Venâncio, A. (2022). The potential of fatty acids and their derivatives as antifungal agents: A review. Toxins, 14(3), 188. DOI 10.3390/toxins14030188. [Google Scholar] [CrossRef]
61. Sachdev, D. P., Cameotra, S. S. (2013). Biosurfactants in agriculture. Applied Microbiology & Biotechnology, 97, 1005–1016. [Google Scholar]
62. Abd El-Rahman, S. S., Mohamed, H. I. (2014). Application of benzothiadiazole and Trichoderma harzianum to control faba bean chocolate spot disease and their effect on some physiological and biochemical traits. Acta Physiologia Plantarum, 36(2), 343–354. [Google Scholar]
63. Desmyttere, H., Deweer, C., Muchembled, J., Sahmer, K., Jacquin, J. et al. (2019). Antifungal activities of Bacillus subtilis lipopeptides to two Venturia inaequalis strains possessing different tebuconazole sensitivity. Frontiers of Microbiology, 10, 2327. DOI 10.3389/fmicb.2019.02327. [Google Scholar] [CrossRef]
64. Ali, S., Hameed, S., Imran, A., Iqbal, M., Lazerovits, G. (2014). Genetic, physiological and biochemical characterization of Bacillus sp. strain RMB7 exhibiting plant growth promoting and broad-spectrum antifungal activities. Microbial Cell Factories, 13, 144. [Google Scholar]
65. Sarwar, A., Brader, G., Corretto, E., Aleti, G., Abaidullah, M. et al. (2018). Qualitative analysis of biosurfactants from Bacillus species exhibiting antifungal activity. PLoS One, 13(6), e0198107. [Google Scholar]
66. Mihalache, G., Balaes, T., Gostin, I., Stefan, M., Coutte, F. et al. (2017). Lipopeptides produced by Bacillus subtilis as new biocontrol products against fusariosis in ornamental plants. Environmental Science Pollution Research, 25, 29784–29793. [Google Scholar]
67. Gajbhiye, A., Rai, A. R., Meshram, S. U., Dongre, A. B. (2010). Isolation, evaluation and characterization of Bacillus subtilis from cotton rhizospheric soil with biocontrol activity against Fusarium oxysporum. World Journal of Microbiology Biotechnology, 26, 1187–1194. [Google Scholar]
68. Mousivand, M., Jouzani, G. S., Monazah, M., Kowsari, M. (2012). Characterization and antagonistic potential of some native biofilm-forming and surfactant-producing Bacillus subtilis strains against six pathotypes of Rhizoctonia solani. Journal of Plant Pathology, 94(1), 171–180. [Google Scholar]
69. Helmi, A., Mohamed, H. I. (2016). Biochemical and ulturasturctural changes of some tomato cultivars to infestation with Aphis gossypii Glover (Hemiptera: Aphididae) at Qalyubiya, Egypt. Gesunde Pflanzen, 68, 41–50. [Google Scholar]
70. Park, G., Nam, J., Kim, J., Song, J., Kim, P. I. et al. (2019). Structure and mechanism of surfactin peptide from Bacillus velezensis antagonistic to fungi plant pathogens. Bulletin of the Korean Chemical Society, 40(7), 704–709. DOI 10.1002/bkcs.11757. [Google Scholar] [CrossRef]
Cite This Article
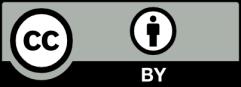
This work is licensed under a Creative Commons Attribution 4.0 International License , which permits unrestricted use, distribution, and reproduction in any medium, provided the original work is properly cited.