Open Access
ARTICLE
Effects of Different Chinese Hickory Husk Returning Modes on Soil Nutrition and Microbial Community in Acid Forest Soil
1
State Key Laboratory of Subtropical Silviculture, Zhejiang A&F University, Hangzhou, 311300, China
2
Lingfeng Temple Forest Farm, Anji, 313300, China
* Corresponding Author: Chenfei Liang. Email:
(This article belongs to the Special Issue: The Effect of Soil Quality Degradation on the Plant Growth, Quality and Food Safety in Subtropical Agroforestry Ecosystems)
Phyton-International Journal of Experimental Botany 2023, 92(3), 943-954. https://doi.org/10.32604/phyton.2022.023225
Received 20 April 2022; Accepted 23 May 2022; Issue published 29 November 2022
Abstract
Chinese hickory (Carya cathayensis Sarg.) is an important economic forest in Southeastern China. A large amount of hickory husk waste is generated every year but with a low proportion of returning. Meanwhile, intensive management has resulted in soil degradation of Chinese hickory plantations. This study aims to investigate the effects of three Chinese hickory husk returning modes on soil amendment, including soil acidity, soil nutrition, and microbial community. The field experiment carried out four treatments: control (CK), hickory husk mulching (HM), hickory husk biochar (BC), and hickory husk organic fertilizer (OF). The phospholipid fatty acid (PLFA) biomarker method was employed to determine the soil microbial community. After one year of treatment, the results showed that: (i) HM and BC significantly increased soil pH by 0.33 and 1.71 units, respectively; (ii) HM, BC and OF treatments significantly increased the soil organic carbon, alkaline nitrogen, available phosphorous, and available potassium. The OF treatment demonstrated the most significant improvement in the soil nutrient; (iii) The soil microbial biomass significantly increased in the HM, BC and OF treatments, and all microbial groups showed an increasing trend. HM treatment increased the fungal/bacterial ratio (F/B). The OF treatment significantly decreased the Shannon-Wiener diversity (H’) and evenness index (J) of the microbial community (P < 0.05). Considering the treatments effects, costs, and ease of operation, our recommended returning modes of Chinese hickory husk are mulching and organic fertilizer produced by composting with manure.Keywords
Chinese hickory (Carya cathayensis Sarg.) is an important economic forest and nut tree widely grown in Southeastern China [1,2]. Chinese hickory is well-liked by consumers for its unique taste and nutritional value. Currently, the Chinese hickory planting area is more than 86,000 ha, with an annual production of 40,000 t [3]. However, to increase production and maximize economic benefits, a large amount of chemical fertilizer, pesticide, and herbicide is applied annually. Long-term intensive management has caused soil degradation including soil erosion, soil acidification, nutrient imbalance, and reduction in soil microbial activities and diversity in Chinese hickory forests [4–7]. These problems can lead to a decline in the quality and yield of Chinese hickory plants [8], thereby causing adverse impacts on economic and ecological benefits.
Agroforestry residues returning to fields have been widely recognized [9]. The rational utilization of wastes is beneficial to proven to increase soil fertility and have positive effects on soil microbial communities. Organic mulching can suppress weeds, reduces soil temperature fluctuations, reduce erosion, and increases total soil porosity and soil moisture [10–12], but mulches composed of organic materials offer an additional range of potential benefits including enhanced soil nutrients and improved soil microbial diversity [13,14]. Quan et al. [15] found that straw return was effective in reducing the loss of soil organic carbon. Zhou et al. [16] found that bacteria were the main driving force of straw degradation, and mulching straw increased soil bacterial biomass; Soil amendment with biochar can adjust soil acidity [17,18], and its adsorption capacity property is conducive to reducing nutrient loss, improving soil water holding capacity, and prevent leaching of plant nutrients [19,20], which also provides a favorable living environment for microorganisms to survive, reproduce and inhabit [21–23]; Organic fertilizers, such as compost and manure, are biodegradable and environmentally friendly, which makes better nutrient sources to increase crop yields. The main advantage of organic fertilizer is to increase the organic matter content of the soil. Several studies reported that the application of organic fertilizers can improve fertility and promote the formation of soil macroaggregates, in addition to increasing the water holding capacity of the soil [24,25]. Modification of soil physicochemical properties had positive effects on soil microbial biomass and activity [26].
Research based on agricultural waste resources can find a better growth environment that can provide nutrients to crops and improve yield and quality. On the other hand, it is of great importance to save resources and protect the environment. Chinese hickory husk accounts for about 60% of its total weight [27]. With the increase of hickory production, a large number of hickory husks are produced every year. The output of Chinese hickory is around 15,600 t in Lin’an City in 2020, and the husk production is about 9360 t (dry weight about 2800 t). Chinese hickory husk contains a wide variety of chemical components such as amino acids, quinones, alkaloids, and contains a large amount of nitrogen, phosphorus, and potassium [28,29]. Unique compared to other agricultural waste resources, Chinese hickory husk has a high carbon content, of which approximately 60% are lignocellulose. Lignocellulosic mulch can improve tree growth, increase soil moisture and soil carbon, as well as promote the formation and stabilization of soil aggregate [30]. The application of carbon-rich crops or organic fertilizers can increase soil fertility and also enhance the diversity of soil microorganisms [31]. Currently, Chinese hickory husks have been developed as raw materials for edible mushroom cultivation, organic fertilizer, or bioinhibitor [27,32], but a large number of hickory husks are still discarded in mountains and roadside endangering soil and water [33,34]. With the current use of hickory husks, hickory forests will lose a significant nutrient each year in the form of husks.
In this study, we selected three common modes of returning Chinese hickory husk to forests, including mulching, biochar, and organic fertilizer. The main objective of this study is to test the effects of the selected three methods on soil acidification, nutrient imbalance, and microbial community in Chinese hickory forests, resolving the contradiction between agricultural waste resources and continuous soil degradation in the forest. We hypothesized that (1) mulching and biochar can alleviate soil acidification; (2) the increase of soil C content is beneficial to improving soil microbial community structure and microbial diversity.
The experimental sample site was located in a hickory forest in Tuankou Town (118°59’51” E, 30°4’26” N), Lin’an City, Western Zhejiang Province. The area has a subtropical monsoon climate with an average annual temperature of 18.2°C and average annual precipitation of 1448.7 mm. The altitude of the area is 340 m and the average annual daylight time is 1781.4 h [35]. Soil types are mainly yellow clay [36]. Chinese hickory tree age of this forest was 20 years and has been under intensive management for 10 years (including the long-term application of chemical fertilizers and herbicides). For fertilizer application, compound fertilizer (N:P2O5:K2O, 15:15:15) with a dosage of about 350 kg hm−2 were used in early May and October, respectively. For the herbicide application, 20% glyphosate with a dosage of about 300 kg hm−2 was applied at the end of April, June, and August, respectively.
The experiment was established on 14 December 2018, set up in a randomized block design with 16 plots (2 m × 2 m) in 4 blocks (20 m × 20 m each). Blocks were separated by at least 20 m. Four treatments were imposed: (1) control (CK); (2) hickory husk biochar (BC); (3) hickory husk mulching (HM); (4) hickory husk organic fertilizer (OF). Each treatment was randomly arranged in the block. The plots were located 5 m from each other and separated by a 30 cm deep sheet of 1 mm thick polyethylene film (20 cm deep belowground and 10 cm aboveground), in order to prevent the exchange of water and nutrients among the plots.
Each treatment was applied as 1.42 kg m−2 C. The application of hickory husk, organic fertilizer, and biochar were 2.92 kg m−2, 6.13 kg m−2 and 2.2 kg m−2 (dry matter), respectively. In this experiment, for one tree, the cost of mulching was close to zero, the cost of organic fertilizer was roughly $3.7 and the cost of biochar was roughly $13. The organic fertilizer was a mixture of hickory husks and sheep manure in the ratio of 4:6 by mass, with total nitrogen of 1.36%, total phosphorus of 0.55%, total potassium of 3.03%, and total carbon of 23.28%. The hickory husks contained 0.96% total nitrogen, 0.45% total phosphorus, 3.26% total potassium, and 48.86% total carbon. The biochar was produced by anaerobic pyrolysis at 500°C for 4 h. Before application, the biochar was ground through a 2 mm sieve. The basic physicochemical properties of biochar with total carbon of 64.85%, total nitrogen of 0.44%, C/N ratio of 147.39, ash of 13.26%, and pH value of 10.02.
On 10 December 2019, soil samples were collected from the topsoil (0–20 cm) after excluding surface litter and mixed well. Soil samples were sieved through a 2 mm sieve and removed debris, fine roots, and stones. Soil samples were divided into two parts, one part was air-dried and used to determine soil organic carbon, total nitrogen, total phosphorus, and available potassium. The other part was freeze-dried and stored at −70°C for PLFA determination.
2.4 Physical and Chemical Properties of Soils
Soil physical and chemical property analysis was carried out with reference to “Soil Agrochemical Analysis” [37]. Soil pH (ratio of 1:2.5, w/v) was measured by the composite electrode method, and soil organic carbon (SOC) was determined following wet digestion with sulfuric acid (H2SO4) and potassium dichromate (K2CrO7). Alkaline nitrogen (AN) was measured by the diffusion absorption method, available phosphorous (AP) was measured by hydrochloric acid-ammonium fluoride method, and available potassium (AK) was measured by ammonium acetate extraction-flame photometer method.
2.5 Microbial Community Indices of Soils
The fatty acids in phospholipids are present only in living microbial cells and are rapidly decomposed upon the death of living cells; Therefore, PLFAs can provide direct information about the living microbial community [38]. Soil microbial community composition was determined by the phospholipid fatty acids (PLFAs) method [39]. The individual fatty acid species are indicated by nmol g−1 dry soil. Among each component, 14:00, 15:00, 16:00, 17:00, 18:00, i14:0, a15:0, i15:0, i15:0 3OH, 15:1ω6c, i16:0, 16:1ω7c, a17:0, cy17:0, i17:0, i17:0 3OH, 18:1ω5c, 18:1ω7c cy19:0 indicate bacteria; 17:00, i14:0, a15:0, i15:0, i16:0, a17:0, i17:0 indicate Gram-positive bacteria; cy17:0, cy19:0, 18:1ω7c indicate Gram-negative bacteria; 10Me16:0, 10Me17:0, 10Me18:0 indicate actinomycetes; 18:1ω9c, 18:2ω6, 9c indicate fungi; 16:1ω5c indicate arbuscular mycorrhizal fungi [40,41].
The soil microbial community diversity was assessed by the Richness (SR), Shannon-Wiener index (H′), and evenness index (J) of PLFA biomarkers. These ecological parameters were calculated as follows:
Richness (SR):
Shannon-Wiener index (H′):
Evenness index (J):
where S is the number of phospholipid fatty acid species occurring in each sample, Pi is the individual as a proportion of the total, Ni is the phospholipid fatty acid content, and N is the sum of all phospholipid fatty acid contents in each sample.
Data were analyzed using SPSS 25.0 and R 3.5.3 software. One-way analysis of variance (ANOVA) was used to compare the differences in soil physicochemical and PLFA indices between treatments (P < 0.05). Principal component analysis (PCA) was used to compare the changes in soil physicochemical and microbial communities between treatments. Redundancy analysis (RDA) was used to analyze the effects of changes in environmental factors on microbial communities, and both PCA and RDA were performed using the vegan package in R software.
3.1 Physical and Chemical Properties of Soils
The results showed that hickory husk returning modes affected the physicochemical properties of the soil (Table 1). The soil pH of BC and HM treatments were significantly higher than the other two treatments (P < 0.05), by 1.71 and 0.33 units, respectively, but OF treatment had no significant effect on pH (P > 0.05). The SOC, AN, AP, and AK contents of OF treatment were the highest among the four treatments. HM, OF and BC treatments increased the soil organic carbon content (P < 0.05). Compared to the CK, the OF treatment had the most significant effect with an increase of 165.7%. The alkaline nitrogen content in the HM and OF treatments were higher than CK, with an increase of 22% and 62%, respectively, and the BC treatment improvement was not significant (P > 0.05). Compared to CK, the available phosphorous of OF treatment was 63.24 mg kg−1, which significantly increased AP content by 333.74%. The BC treatment was increased by 73.04% (P < 0.05), and there was no significant difference between HM and CK (P > 0.05). The three treatments increased the available potassium content in the soil (P < 0.05), the enhancement effect showed that OF > BC > HM > CK, and the pairwise comparisons between these groups revealed a significant difference (P < 0.05).
3.2 Microbial Community Structure of Soils
The total microbial biomass of BC, HM, and OF treatments were significantly higher than CK, increasing by 54.89%, 25.41%, and 22.13%, respectively (P < 0.05) (Fig. 1). The bacterial biomass of the four treatments were ranked as BC > OF > HM > CK, and the fungal biomass of the three treatments were higher than CK (P > 0.05). The BC treatment arbuscular mycorrhizal fungi (AMF) biomass was 0.80 nmol g−1, which was higher than the other three treatments (P < 0.05), and the OF treatment of 0.21 nmol g−1 was the lowest among the four treatments. The BC treatment had the highest biomass of actinomycetes among the four treatments (P < 0.05). Three treatments had significantly higher Gram-positive bacteria and BC had the largest increase by 54.9%. Gram-positive bacteria was higher in BC and OF treatments than in CK (P < 0.05), and slightly increased in HM treatment (P > 0.05). Compared to CK, the ratio of Gram-positive bacteria/Gram-negative bacteria PLFAs (G+/G−) in OF and HM treatments were significantly different (P < 0.05), with a decrease of 0.32 in OF treatment. The HM treatment fungal/bacterial ratio (F/B) of 0.2 was higher than in OF (P < 0.05), and there was no significant difference between BC and CK (P > 0.05).
Figure 1: Comparisons of soil microbial PLFAs, and the ratios fungi/bacteria and G+/G- in different treatments
Error bars represent the standard error of the mean (n = 4). Different letters indicate significant differences at P < 0.05. B: bacterial PLFAs; F: fungal PLFAs; F/B: the ratio of fungal to bacterial PLFAs; G+: gram-positive bacterial PLFAs; G-: gram-negative bacterial PLFAs; G+/G−: the ratio of gram-positive bacterial to gram-negative bacterial PLFAs; AMF: arbuscular mycorrhizal fungal PLFAs; ACT: actinomycests PLFAs; Total: total PLFAs.
There was no significant difference in the richness (SR) among the four treatments (Table 2). Compared to the CK, the evenness index (J) was significantly lower in OF and BC treatments (P < 0.05), and there was no significant difference between HM and CK treatments (P > 0.05). The Shannon-Wiener diversity index (H′) in OF was the lowest among the four treatments (P < 0.05).
3.3 Relationships between Soil Microbial Community Changes and Environmental Factors
Combined with Pearson’s correlation analysis (Fig. 2), pH was found to be positively correlated (P < 0.01) with soil mycorrhizal fungi, fungi, actinomycetes, Gram-positive bacteria, Gram-negative bacteria, G+/G−, and total biomass, except for F/B. The SOC, AN, and AP showed a negative correlation (P < 0.05) with mycorrhizal fungi and actinomycetes, and a positive correlation (P < 0.05) with Gram-negative bacteria. The AK was significantly and positively correlated with Gram-negative bacteria and G+/G− (P < 0.05).
Figure 2: Correlation coefficients between physicochemical properties and soil microbial PLFAs
Values are correlation coefficients, and symbols represent the significance of the correlation coefficient. * P < 0.05; ** P < 0.01; *** P < 0.001.
The relationship between environmental factors and soil microbial communities was explained by redundancy analysis (RDA) (Fig. 3). The first ordination RDA axis (RDA axis 1, horizontal) explained 49.35% of the variation in the sample and the second ordination RDA axis (RDA axis 2, vertical) explained 22.15% of the total variation in the sample, both cumulatively explained 71.5% of the variation in the microbial community. On RDA 1, BC was significantly different from HM (P < 0.05), and HM was significantly different from OF and CK (P < 0.05). On RDA 2, OF was significantly different from BC (P < 0.05), and BC was significantly different from HM and CK (P < 0.05). The direction of transformation of CK compared with OF is mainly in the positive direction of RDA 2, and all the physicochemical properties are the same except for the pH value. The direction of transformation of CK compared with HM and BC was mainly in the positive direction of RDA 1. All microbiological indicators were identical to them except for the F/B ratio.
Figure 3: Redundancy analysis (RDA) of soil microbial PLFAs and physicochemical properties
Red arrows connect the soil microbial PLFAs, blue arrows connect the physical and chemical properties.
4.1 Physical and Chemical Properties of Soils
Hickory husk mulching and biochar can significantly improve soil pH. BC and HM treatments contain large amounts of exchangeable cations [38] and abundant alkaloids [28,29], respectively, both of which can significantly improve soil pH. However, the hickory husks in OF treatment are decomposed after composting and the alkaloids are degraded [42]. Moreover, various organic acids have been produced during the decomposition and transformation of organic matter in sheep manure [43], resulting in OF treatment not changing soil acidity.
Soil organic carbon was significantly increased by different hickory husk returning modes. The OF treatment had the highest organic carbon, with an increase of 165.70% (Table 2). In OF treatment, the hickory husk and sheep manure has been decomposed into organic matter or humus [44], thus increasing the input of soil organic carbon. In BC treatment, biochar can provide a stable source of soil organic carbon due to its stable aromatic hydrocarbon structure [45], moreover, its unique microporous structure that can adsorb organic small molecules from the soil [46]. The enhancement effect of HM treatment on soil organic carbon was close to BC treatment, and the contribution of hickory husks to soil nutrients was closely related to the rate of hickory husks decomposition, which required further experiments on the rate of hickory husks decomposition [47,48]. Chinese hickory trees are mostly planted in mountainous areas. Many studies reported that increasing the soil organic carbon is conducive to reducing soil loss [49]. Mulching directly improves soil stability and soil moisture [10,11].
Compared to CK, three hickory husk returning modes can increase the content of AN, AP, and AK on the soil. In general, OF and BC treatments were more effective in enhancing nutrients than HM treatment. This may be due to the fact that hickory husk is more easily decomposed after high temperatures compost, thus more conducive to nutrient release. Han et al. [50] found that the decay and nutrient release rate of applied rotting straw was faster than the direct application of straw. Biochar, as a pyrolysis product, with a unique microporous structure and nutrient availability can lead to higher nutrient retention and nutrient availability [51]. Compared to BC and OF treatments, hickory husk mulching was slightly effective in improving soil nutrients because of the high content of hard-to-decompose substances in the hickory husk [28], which is not conducive to the release of nutrient elements from hickory husks. Significantly, hickory husk had low N and P content but high K content of about 3.25%, so the three treatments had a significant promotion of available potassium content. In addition, we also observed that the OF treatment showed the most significant increase in AN, AP and AK due to the addition of sheep manure.
4.2 Microbial Community Structure of Soils
In previous studies, other agroforestry wastes (straw, peanut shells, sheep manure, etc.) applied to soil in a similar utilization manner all significantly increased the soil microbial biomass [14,52,53]. The changes in soil pH, SOC, AN, AK, and AP by the three ways of hickory husk returning modes were closely related to the structural variation of the soil microbial community by RDA analysis (Fig. 3).
The BC treatment was the most effective in increasing soil bacterial biomass among different methods of hickory husk incorporation. This can be explained by the fact that the BC treatment contains a wide variety of organic carbon, salt-based ions and has a unique microporous structure [54,55], which improves the acidity and nutrient deficiency of hickory woodland soils and creates a soil environment conducive to bacterial growth. The arbuscular mycorrhizal fungi and actinomycetes prefer to grow in neutral or alkaline soils [56] and prefer to feed on difficult to decompose materials in the soil [57], and the biomass of both were also found to be significantly positively correlated with soil pH and negatively correlated with soil organic carbon in Pearson correlation analysis (Fig. 2). Therefore, the biomass of arbuscular mycorrhizal fungi and actinomycetes in BC and HM treatments were significantly higher than in OF treatment. In the correlation analysis, G+/G− was found to be positively and significantly correlated with pH and negatively and significantly correlated with nutrient indicators (Fig. 2), so the ratio of G+/G− in OF treatment was significantly decreased. Meanwhile, the G+/G− ratio characterized the degree of soil nutrient stress [58], which shows that OF treatment was the most effective among the four treatments in improving the soil nutrient status.
Soil fungal biomass did not vary significantly among the different methods of hickory husks incorporation, with pairwise comparisons between these treatments were not significant (P > 0.05), but HM treatment fungal biomass was the highest among the four treatments (Fig. 1). The RDA analysis yielded a significant positive correlation between soil pH and fungal biomass (Fig. 3). The HM treatment significantly improved soil pH and the fungus is the microorganism with the most ability to decompose lignin and cellulose [59], the high content of indecomposable substances in the hickory husk. Therefore, the hickory husk mulch is conducive to fungal colonization.
Both the evenness index (J) and the Shannon-Wiener index (H’) decreased significantly in OF treatment, and the evenness index (J) decreased significantly in BC treatment, with only HM showing no significant change. Compared to OF and BC treatments, HM treatment is similar to no-tillage and has a lower level of disturbance to the system. In addition, HM treatment has the highest F/B ratio, which also indicates that the hickory husk mulching method improves the low efficiency of resource conversion under the original intensive management and improves the system below disturbance [60,61].
In this study, hickory husk organic fertilizer has the most significant promotion effect on soil available nutrients without a decrease in pH. The hickory husk biochar and hickory husk mulching are conducive to reducing soil acidity and both the diversity and stability of soil microbial communities. It is necessary to comprehensively consider factors such as soil acidification status, material cost, and ease of operation in the management process. Our recommended returning modes of Chinese hickory husk are mulching and organic fertilizer produced by composting with manure.
Acknowledgement: We thank Wei Fang, Shanshan Wang, Chi Zhang, Ziyi Zhang and Yien Ma for help with fieldwork and laboratory analysis.
Authorship: The authors confirm contribution to the paper as follows: study conception and design: Chenfei Liang and Xinru Ma; data collection: Yongqian Tang and Xinyu Shen; analysis and interpretation of results: Qian Liu and Sayikal DUYXANALE; draft manuscript preparation: Qian Liu; reviewed the draft: Chenfei Liang and Shuai Shao. All authors reviewed the results and approved the final version of the manuscript.
Funding Statement: This work was financially supported by Natural Science Foundation of Zhejiang Province (LY20C160003) and the National College Students’ Innovation and Entrepreneurship Training Program (202110341063).
Conflicts of Interest: The authors declare that they have no conflicts of interest to report regarding the present study.
References
1. Lv, H. J. (2005). The natural stands canditions of Cara cathayensis Sarg. in Lin’an Country of Zhejiang Province. Forest Engineering, 21(1), 1–3. [Google Scholar]
2. Ai, C. X., Li, C. X., Chen, X. Y., Liu, Q. Z. (2006). Resources of Carya Nutt. in China. Deciduous Fruits, 20(4), 23–24. [Google Scholar]
3. Yang, J. M. (2018). The survey and analysis on cultivation status, economic benefits, input-output ratioand development strategies of Ningguo Carya cathayensis. Anhui Agricultural Science Bulletin, 24(21), 118–119. [Google Scholar]
4. Gao, S. C., Guan, D. W., Ma, M. C., Zhang, W., Li, J. et al. (2017). Effects of fertilization on bacterial community under the condition of continuous soybean monoculture in black soil in Northeast China. Scientia Agricultura Sinica, 50(7), 1271–1281. [Google Scholar]
5. Zhang, L. L., Jia, G., Ye, M., F, J., Ma, L. J. (2013). Frequency of Carya cathayensis canker disease in Lin’an City, Zhejiang Province. Journal of Zhejiang A&F University, 30(1), 148–152. [Google Scholar]
6. Fu, W. J., Dong, J. Q., Ding, L. Z., Yang, H. S., Ye, Z. Q. et al. (2022). Spatial correlation of nutrients in a typical soil-hickory system of Southeastern China and its implication for site-specific fertilizer application. Soil & Tillage Research, 217, 105265. DOI 10.1016/j.still.2021.105265. [Google Scholar] [CrossRef]
7. Jin, J., Wang, L. Q., Müller, K., Wu, J. S., Wang, H. L. et al. (2021). A 10-year monitoring of soil properties dynamics and soil fertility evaluation in Chinese hickory plantation regions of Southeastern China. Scientific Reports, 11(1), 23531. DOI 10.1038/s41598-021-02947-z. [Google Scholar] [CrossRef]
8. Wu, J. S., Zhang, J. C., Qian, J. F., Huang, J. Q. (2013). Intercropping grasses improve soil organic carbon content and microbial community functional diversities in Chinese hickory stands. Transactions of the Chinese Society of Agricultural Engineering, 29(20), 111–117. [Google Scholar]
9. Cao, G., Zhang, X., Zheng, F. (2006). Inventory of black carbon and organic carbon emissions from China. Atmospheric Environment, 40(34), 6516–6527. DOI 10.1016/j.atmosenv.2006.05.070. [Google Scholar] [CrossRef]
10. Snapp, S. S., Swinton, S. M., Labarta, R., Mutch, D., O’Neil, K. (2005). Evaluating cover crops for benefits, costs and performance within cropping system niches. Agronomy Journal, 97(1), 322–332. DOI 10.2134/agronj2005.0322a. [Google Scholar] [CrossRef]
11. Wang, Y., Xie, Z., Malhi, S. S., Vera, C. L., Zhang, Y. et al. (2009). Effects of rainfall harvesting and mulching technologies on water use efficiency and crop yield in the semi-arid loess plateau, China. Agricultural Water Management, 96(3), 374–382. DOI 10.1016/j.agwat.2008.09.012. [Google Scholar] [CrossRef]
12. Tu, A. G., Xie, S. H., Zheng, H. J., Li, H. G., Li, Y. et al. (2021). Long-term effects of living grass mulching on soil and water conservation and fruit yield of citrus orchard in South China. Agricultural Water Management, 252, 106897. DOI 10.1016/j.agwat.2021.106897. [Google Scholar] [CrossRef]
13. Dong, Q. G., Yang, Y. C., Yu, K., Feng, H. (2018). Effects of straw mulching and plastic film mulching on improving soil organic carbon and nitrogen fractions, crop yield and water use efficiency in the loess plateau, China. Agricultural Water Management, 201, 133–143. DOI 10.1016/j.agwat.2018.01.021. [Google Scholar] [CrossRef]
14. Zhang, S. N., Wang, Y., Sun, L. T., Qiu, C., Ding, Y. Q. et al. (2020). Organic mulching positively regulates the soil microbial communities and ecosystem functions in tea plantation. BMC Microbiology, 20(5), 374–382. DOI 10.1186/s12866-020-01794-8. [Google Scholar] [CrossRef]
15. Quan, Z., Li, S. L., Zhu, F. F., Zhang, L. M., He, J. Z. et al. (2018). Fates of 15N-labeled fertilizer in a black soil-maize system and the response to straw incorporation in Northeast China. Journal of Soils & Sediments, 18(4), 1441–1452. DOI 10.1007/s11368-017-1857-3. [Google Scholar] [CrossRef]
16. Zhou, Z. J., Guo, S., Chen, K., Zeng, X. Z., Shangguan, Y. X. et al. (2021). Long-term straw mulching affects rice and wheat yields, soil nitrogen fractions, and microbial community under a no-till system. Acta Pedologica Sinica, 7, 1–13. [Google Scholar]
17. Chintala, R., Mollinedo, J., Schumacher, T. E., Malo, D. D., Julson, J. L. (2014). Effect of biochar on chemical properties of acidic soil. Archives of Agronomy and Soil Science, 60(3), 393–404. DOI 10.1080/03650340.2013.789870. [Google Scholar] [CrossRef]
18. Shetty, R., Prakash, N. B. (2020). Effect of different biochars on acid soil and growth parameters of rice plants under aluminium toxicity. Scientific Reports, 10(1), 12249. DOI 10.1038/s41598-020-69262-x. [Google Scholar] [CrossRef]
19. Major, J., Rondon, M., Molina, D., Riha, S. J., Lehmann, J. (2012). Nutrient leaching in a Colombian savanna oxisol amended with biochar. Journal of Environmental Quality, 41(4), 1076–1086. DOI 10.2134/jeq2011.0128. [Google Scholar] [CrossRef]
20. Hossain, M. Z., Bahar, M. M., Sarkar, B., Donne, S. W., Ok, Y. S. et al. (2020). Biochar and its importance on nutrient dynamics in soil and plant. Biochar, 2(4), 379–420. DOI 10.1007/s42773-020-00065-z. [Google Scholar] [CrossRef]
21. Gu, M. Y., Tang, G. M., Liu, H. L., Li, Z. Q., Liu, X. W. et al. (2016). Effects of cotton stalk biochar on microbial community structure and function of continuous cropping cotton rhizosphere soil in Xinjiang, China. Chinese Journal of Applied Ecology, 27(1), 173–181. [Google Scholar]
22. Wang, D. D., Zhang, N., Tang, H. Q., Adams, J. M., Sun, B. et al. (2019). Straw biochar strengthens the life strategies and network of rhizosphere fungi in manure fertilized soils. Soil Ecology Letters, 1(1–2), 72–84. DOI 10.1007/s42832-019-0008-8. [Google Scholar] [CrossRef]
23. Gomez, J. D., Denef, K., Stewart, C. E., Zheng, J., Cotrufo, M. F. (2014). Biochar addition rate influences soil microbial abundance and activity in temperate soils. European Journal of Soil Science, 65(1), 28–39. DOI 10.1111/ejss.12097. [Google Scholar] [CrossRef]
24. Zhang, J. M., Chi, F. Q., Wei, D., Zhou, B. K., Cai, S. S. et al. (2019). Impacts of long-term fertilization on the molecular structure of humic acid and organic carbon content in soil aggregates in black soil. Scientific Reports, 9(1), 1–7. DOI 10.1038/s41598-019-48406-8. [Google Scholar] [CrossRef]
25. Subaedah, S., Aladin, A., Nirwana (2016). Fertilization of nitrogen, phosphor and application of green manure of Crotalaria juncea in increasing yield of maize in marginal dry land. Agriculture and Agricultural Science Procedia, 9, 20–25. DOI 10.1016/j.aaspro.2016.02.114. [Google Scholar] [CrossRef]
26. Cai, Y. J., Shen, J. P., Di, H. J., Zhang, L. M., Zhang, C. J. et al. (2020). Variation of soil nitrate and bacterial diversity along soil profiles in manure disposal maize field and adjacent woodland. Journal of Soils and Sediments, 20, 3557–3568. DOI 10.1007/s11368-020-02679-z. [Google Scholar] [CrossRef]
27. Yan, F. (2013). Advance in resource utilization of agricultural and forestry waste hickorg epicarp. Journal of Anhui Agricultural Sciences, 41(14), 6329–6330. [Google Scholar]
28. Chen, X. M., Yu, Z. M., Jin, J., Liu, M. X. (2007). Studies on the inorganic composition of hickory hull. Chinese Journal of Analysis Laboratory, 8, 45–47. [Google Scholar]
29. Jiang, Z. Y., Xuan, G. D., Li, L. L. (2009). Study on qualitative identification of chemical constituents in husks of Carya cathayensis sarg and extraction techniques of total alkaloids. Journal of Zhejiang University (Science Edition), 36(4), 442–449. [Google Scholar]
30. Tian, G., Li, Y. (2022). Lignocellulose mulch increases the economic benefit of Chinese chestnut by suppressing weed and ameliorating soil properties. Scientia Horticulturae, 291, 110576. DOI 10.1016/j.scienta.2021.110576. [Google Scholar] [CrossRef]
31. Liu, T., Chen, X. Y., Hu, F., Ran, W., Shen, Q. R. et al. (2016). Carbon-rich organic fertilizers to increase soil biodiversity: Evidence from a meta-analysis of nematode communities. Agriculture, Ecosystems and Environment, 232, 199–207. DOI 10.1016/j.agee.2016.07.015. [Google Scholar] [CrossRef]
32. Shen, X. Y., Mao, J. W., Cai, C. G. (2013). Research progress of ingredients and function of hickory hell. Food Research and Development, 34(21), 128–130. [Google Scholar]
33. Wang, G. P., W, G. U. O. Z. (2006). Comprehensive control and effect of hickory shell pollution. Modern Agricultural Science and Technology, (23), 72–73. [Google Scholar]
34. Liu, Y. H., Lv, J. L., Yuan, K., Yang, P. (2011). Antioxidant activities of the extracts of Carya cathayensis sarg. Asian Journal of Chemistry, 23(2), 676–680. [Google Scholar]
35. Bureau, Z. M. (2017). Yearbook of zhejiang meteorological stations. Hangzhou: Zhejiang Science and Technology Publishing House. [Google Scholar]
36. Qian, X. Y., Huang, J. Q., Shuai, X. B., Wu, J. S. (2013). Comparison of soil physiochemical properties at Carya cathayensis stands in Lin’an. Journal of Zhejiang Forestry Science and Technology, 33(1), 7–11. [Google Scholar]
37. Lu, R. K. (2000). Soil and agro-chemistry analytical methods. Beijing, China: China Agricultural Science and Technology Press. [Google Scholar]
38. Bossio, D. A., Scow, K. M. (1998). Impacts of carbon and flooding on soil microbial communities: Phospholipid fatty acid profiles and substrate utilization patterns. Microbial Ecology, 35, 265–278. DOI 10.1007/s002489900082. [Google Scholar] [CrossRef]
39. Bligh, E. G., Dyer, W. J. (1959). A rapid method of total lipid extraction and purification. Canadian Journal of Biochemistry and Physiology, 37(8), 911–917. DOI 10.1139/o59-099. [Google Scholar] [CrossRef]
40. Zak, D. R., Ringelberg, D. B., Pregitzer, K. S., Randlett, D. L., White, D. C. et al. (1996). Soil microbial communities beneath populus grandidentata grown under elevated atmospheric CO2. Ecological Applications, 6(1), 257–262. DOI 10.2307/2269568. [Google Scholar] [CrossRef]
41. Bååth, E., Anderson, T. H. (2003). Comparison of soil fungal/bacterial ratios in a pH gradient using physiological and PLFA-based techniques. Soil Biology and Biochemistry, 35(7), 955–963. DOI 10.1016/S0038-0717(03)00154-8. [Google Scholar] [CrossRef]
42. Wang, Y. P., Xu, B. B., Li, S. W., Xu, X., Ma, J. H. (2013). The research advance of comprehensive utilization of the husks of Carya cathayensis sarg. Guangzhou Chemical Industry, 41(23), 20–22. [Google Scholar]
43. Rinki, N. N., Salam, A. B. A., Khan, M. Z. (2019). Effects of coconut oil cakes on the growth performance of gima kalmi (Ipomoea aquatica). Asian Journal of Advances in Agricultural Research, 8(4), 2456–8864. DOI 10.9734/AJAAR. [Google Scholar] [CrossRef]
44. During, C., Weeda, W. C., Dorofaeff, F. D. (2012). Some effects of cattle dung on soil properties, pasture production, and nutrient uptake. New Zealand Journal of Agricultural Research, 16(3), 431–438. DOI 10.1080/00288233.1973.10421126. [Google Scholar] [CrossRef]
45. Schmidt, M. W. I., Noack, A. G. (2000). Black carbon in soils and sediments: Analysis, distribution, implications, and current challenges. Global Biogeochemical Cycles, 14(3), 777–793. DOI 10.1029/1999GB001208. [Google Scholar] [CrossRef]
46. Johannes, H., Martin, K. H., Stefanie, S., Reiner, R., Markus, F. et al. (2014). Linking N2O emissions from biochar-amended soil to the structure and function of the N-cycling microbial community. The ISME Journal, 8(3), 660–674. DOI 10.1038/ismej.2013.160. [Google Scholar] [CrossRef]
47. Shure, D. J., Gottschalk, M. R., Parsons, K. A. (1986). Litter decomposition processes in a floodplain forest. The American Midland Naturalist, 115(2), 314–327. DOI 10.2307/2425868. [Google Scholar] [CrossRef]
48. Coleman, D. C., Crossley, D. A., Hendrix, P. F. (2004). Fundamentals of soil ecology. Burlington: Elsevier Academic Press. [Google Scholar]
49. Remus, P., IonAndrei, N., Cristian, P., Mihai, N., Victor, B. M. et al. (2021). Global changes in soil organic carbon and implications for land degradation neutrality and climate stability. Environmental Research, 201, 111580. DOI 10.1016/j.envres.2021.111580. [Google Scholar] [CrossRef]
50. Han, X. J., Zhang, X. Z. (2013). Effects of treating different crop straw returning to field with decomposition on the physico chemical properties of soil and crop yield. Anhui Agricultural Science Bulletin, 19(16), 80–82. [Google Scholar]
51. Saito, M., Marumoto, T. (2002). Inoculation with arbuscular mycorrhizal fungi: The status quo in Japan and the future prospects. Plant and Soil, 244(1–2), 273–279. [Google Scholar]
52. Wang, C., Chen, D., Shen, J. L., Yuan, Q., Fan, F. L. et al. (2021). Biochar alters soil microbial communities and potential functions 3–4 years after amendment in a double rice cropping system. Agriculture, Ecosystems and Environment, 311(4), 107291. DOI 10.1016/j.agee.2020.107291. [Google Scholar] [CrossRef]
53. Jiang, Y. H., Arafat, Y., Letuma, P., Ali, L., Tayyab, M. et al. (2019). Restoration of long-term monoculture degraded tea orchard by green and goat manures applications system. Sustainability, 11(4), 1011. DOI 10.3390/su11041011. [Google Scholar] [CrossRef]
54. Chen, Z., Wang, Y. P., Xia, D., Jiang, X. L., Fu, D. et al. (2016). Enhanced bioreduction of iron and arsenic in sediment by biochar amendment influencing microbial community composition and dissolved organic matter content and composition. Journal of Hazardous Materials, 311, 20–29. DOI 10.1016/j.jhazmat.2016.02.069. [Google Scholar] [CrossRef]
55. Ippolito, J. A., Stromberger, M. E., Lentz, R. D., Dungan, R. S. (2014). Hardwood biochar influences calcareous soil physicochemical and microbiological status. Journal of Environmental Quality, 43(2), 681–689. DOI 10.2134/jeq2013.08.0324. [Google Scholar] [CrossRef]
56. Zhang, Y. L., Li, T. T., Wu, H. H. (2019). Effect of different fertilization practices on soil microbial community in a wheat-maize rotation system. Sustainability, 11(15), 4088. DOI 10.3390/su11154088. [Google Scholar] [CrossRef]
57. Asadu, C. O., Aneke, N. G., Egbuna, S. O., Agulanna, A. C. (2018). Comparative studies on the impact of bio-fertilizer produced from agro-wastes using thermo-tolerant actinomycetes on the growth performance of maize (Zea-mays) and okro (Abelmoschus esculentus). Environmental Technology & Innovation, 12, 55–71. DOI 10.1016/j.eti.2018.07.005. [Google Scholar] [CrossRef]
58. Garcia-S-nchez, M., Garcia-Romera, I., Cajthaml, T., Tlusto, P., Sz-ková, J. (2015). Changes in soil microbial community functionality and structure in a metal-polluted site: The effect of digestate and fly ash applications. Journal of Environmental Management, 162, 63–73. DOI 10.1016/j.jenvman.2015.07.042. [Google Scholar] [CrossRef]
59. Putri, P. I. P. A., Mangunwardoyo, W., Sudiana, I. M., Idris Ramadhani, I. (2021). Isolation, screening, and molecular identification of lignin-degrading fungi isolates from Cibinong Science Centre, Indonesia Institute of Sciences, Cibinong, West Java. Journal of Physics: Conference Series, 1725(1), 012068. IOP Publishing. DOI 10.1088/1742-6596/1725/1/012068. [Google Scholar] [CrossRef]
60. Morriën, E., Hannula, S. E., Snoek, L. B., Helmsing, N. R., Zweers, H. et al. (2017). Soil networks become more connected and take up more carbon as nature restoration progresses. Nature Communications, 8(2), 1–10. DOI 10.1038/ncomms14349. [Google Scholar] [CrossRef]
61. Vries, F. T. D., Hoffland, E., Eekeren, N. V., Brussaard, L., Bloem, J. (2006). Fungal/bacterial ratios in grasslands with contrasting nitrogen management. Soil Biology and Biochemistry, 38(8), 2092–2103. DOI 10.1016/j.soilbio.2006.01.008. [Google Scholar] [CrossRef]
Cite This Article
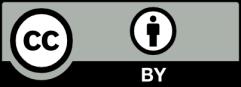
This work is licensed under a Creative Commons Attribution 4.0 International License , which permits unrestricted use, distribution, and reproduction in any medium, provided the original work is properly cited.