Open Access
ARTICLE
Comparative Analysis of the Photosynthetic Characteristics and Active Compounds of Semiliquidambar cathayensis Chang Heteromorphic Leaves
Hunan Botanical Garden, Changsha, 410116, China
* Corresponding Author: Xiaoming Tian. Email:
(This article belongs to the Special Issue: Identification of Genetic/Epigenetic Components Responding to Biotic and Abiotic Stresses in Crops)
Phyton-International Journal of Experimental Botany 2023, 92(3), 837-850. https://doi.org/10.32604/phyton.2023.024408
Received 26 July 2022; Accepted 14 September 2022; Issue published 29 November 2022
Abstract
In the present study, the variation patterns of leaf shape in different populations of individual Semiliquidambar cathayensis plants were analyzed to investigate the relationship among leaf shape variation, photosynthetic properties, and active compounds to understand the genetic characteristics of S. cathayensis and screen elite germplasms. The leaf shape of 18 offspring from three natural S. cathayensis populations was analyzed to investigate the level of diversity and variation patterns of leaf shape. Furthermore, photosynthetic pigment content, physiological parameters of photosynthesis, and the active compounds in leaves of different shapes were determined. Statistical analysis showed that the leaf shape variation in S. cathayensis indicated a high level of genetic diversity among and within the populations. Cluster analysis showed that the three natural populations formed two clusters, one whose offspring was dominated by entire leaves and another characterized by palmately trifoliate leaves. The differences in photosynthetic characteristics and active compounds of leaves of three different shapes were comprehensively evaluated using principal component analysis. Two principal components with a cumulative contribution rate of 92.768% were extracted, of which the highest comprehensive score was for asymmetrically lobed leaves. The leaf shape in different S. cathayensis germplasms exhibited distinct patterns, and there were some correlations between the photosynthetic properties and active compounds in leaves of different shapes. Thus, the leaf shape can be used to predict active compound content, and in turn, select varieties based on that purpose; it also provides a simple and effective method to classify S. cathayensis germplasms.Keywords
Semiliquidambar cathayensis belongs to the family Altingiaceae and is endemic to China. It is monoecious and pollinated mainly by wind. It has the following characteristics: straight trunk, attractive tree shape, and variable leaf shape. Its leaves are purplish red in early spring and green, yellow, and purplish red to dark red in late autumn. It is of high value for landscape development. Its roots, branches, and leaves are used as Traditional Chinese Medicine [1,2]. One active ingredient of the root of S. cathayensis is oleanolic acid [3], which has anti-hepatitis effect. Various active compounds in the leaves of S. cathayensis can be used to treat rheumatism, bruise, swelling and pain, and postpartum paralysis [4]. As harvesting the branches, roots, and nectar of S. cathayensis is difficult, people in China often boil the leaves of S. cathayensis to prepare a medicinal solution for bathing, topical application, or ingestion to dispel wind, cold, and dampness, strengthen the body, and achieve reinforcement against the harsh environment in high mountain areas [5].
Mature S. cathayensis leaves are clustered at the top of branches and have traits that are a combination of traits of two genera Liquidambar and Altingia—as single leaves similar to those of Altingia, as palmately trifoliate leaves similar to those of Acer buergerianum, or asymmetrically lobed leaves known as “evolutionary heterophylly” [4]. For example, the long branches and leaves of young Populus euphratica resemble those of Salix, but the short branches and leaves of mature plants resemble those of Populus, which reflects the relationship between the genera Populus and Salix [6].
Owing to the harsh growth environment and scarcity of viable seeds, S. cathayensis is often a non-dominant species in an ecological community and is often in an unfavorable position when competing for light and thermal resources. Besides over-harvesting, the above factors have contributed to a major loss of the genetic resources of S. cathayensis, placing the plant on the verge of extinction. Currently, S. cathayensis populations remain only in the mountainous regions of Southern and Southeastern China [7] and thus, artificial breeding and cultivation are urgently required to preserve the species. Using SRAP (Sequence-related amplified polymorphism) marker technology, studies have found that S. cathayensis has high genetic diversity [8,9]; therefore, it is important to elucidate the differences among germplasms and screen elite germplasms. The identification and description of plant phenotypic traits are the most appropriate methods for research on germplasm resources. In this study, we analyzed the variation patterns of leaf shape in different populations of S. cathayensis and the relationship between leaf shape variation and photosynthetic properties and active compounds. Our results will serve as an important reference and scientific basis for understanding the genetic characteristics of S. cathayensis and for screening elite germplasms.
Seeds from individual adult S. cathayensis plants in each region were collected between October and November 2018 from naturally distributed populations in Jianghua and Jingzhou Counties, Hunan Province, and Dayu County, Jiangxi Province. In addition, the naturally distributed S. cathayensis populations in these three counties were identified as S. cathayensis Chang by botanical expert Yan Lihong from Hunan Botanical Garden Plant Conservation Research Institute through morphological identification.
The number of individual plants in each site from which the seeds were collected was determined by the size of the population in that area. The seeds of S. cathayensis were collected from 18 individuals and numbered as JH01-JH08 (Jianghua County), JZ01-JZ06 (Jingzhou County), and DY01-DY04 (Dayu County). Detailed geographic distribution data are shown in Suppl. Table S1. In January 2019, the collected seeds were sown in a planting trough at Hunan Botanical Garden nursery and in May, when most of the seedlings had more than four true leaves, 60 young offspring from each parent were randomly selected and transferred to 26 cm × 21 cm plastic nutrient cups with tillage soil as the substrate. The seedling cultures were weeded regularly, and pests and diseases were controlled throughout the experiment.
Ten offspring seedlings from a single JZ01 parent plant were randomly selected for leaf photosynthetic characteristic and active compound analyses. For the analyses, we collected three fully expanded leaves of each of the three leaf shapes from the top to bottom of the second round of healthy and mature branches from each seedling.
2.2.1 Semiliquidambar Cathayensis Leaf Shape Diversity
In May 2021, 30 offspring of each parent plant were randomly selected and the leaf shape of each offspring seedling was recorded.
2.2.2 Determination of Photosynthetic Pigment Content in Semiliquidambar Cathayensis Leaves
Photosynthetic pigment content in leaves of different shapes was determined as previously described [10] with slight modifications. Several S. cathayensis leaves of different shapes were collected and any dirt on the surface of the leaves was washed off. The midvein was removed and the remaining leaf tissue was cut to ensure that 0.25 g of each sample was obtained; this step was performed for three biological samples. Thereafter, the samples were added into 50 mL centrifuge tubes with 25 mL acetone and absolute ethanol mixture (V:V = 1:1) and placed away from light for 48 h to fully dissolve the pigment. The solution was then added to a quartz colorimeter and the absorbance was measured at 470, 663 and 645 nm using an ultraviolet spectrophotometer (Epoch 2; Biotek, Winooski, VT, USA). Eqs. (1)–(4) were used to calculate photosynthetic pigment content.
Chla is the content of chlorophyll a, Chlb is the content of chlorophyll b, Chl (a + b) is the content of total chlorophyll, Car is the content of carotenoid, V is the volume of extraction solution (mL), and W is the mass of each sample (g).
2.2.3 Measurement of Photosynthetic Parameters in Semiliquidambar Cathayensis Leaves
Gas exchange parameters were measured using a LI-6400 portable photosynthesis system (LI-COR, Lincoln, NE, USA). Photosynthetic rate–light intensity response curves were plotted as previously described [11]. The tested leaves were induced at a light intensity of 800 μmol·m−2s−1 for 30 min before each measurement. Next, red/blue light was used to deliver photosynthetic photon flux density of different intensities and the CO2 concentration was stabilized at 400 ± 0.5 μmol·mol−1 at a gas flow rate of 500 ± 0.5 mmol·s−1. The light response curves of different S. cathayensis leaf shapes were fitted with a right-angled hyperbolic correction model. The induction curves of the three replicates were averaged from data collected in triplicate between 08:00 and 10:00 h.
2.2.4 Determination of Active Compound Content in Semiliquidambar Cathayensis Leaves
The fresh leaves of S. cathayensis were collected, washed, and dried in shade. The dried samples were powdered and filtered through a 60 mesh sieve. The powder was stored in a sealed bag for subsequent tests.
Determination of Total Flavonoid Content
Total flavonoid content was determined using colorimetric assay according to the method described by Zhang et al. [12] with some modifications. The leaf powder (2 g) was extracted with 50% ethanol (25 mL) for 5 h at 25°C. Thereafter, ultrasonic assisted extraction was performed for 20 min. The extraction process was repeated twice. The extract was passed through filter paper. The filtrate was cooled for 20 min, and then dried using a rotary evaporator. The residue was suspended with methanol (50 mL) and passed through a 0.45-μM membrane (Millipore, USA) for filtration. The extract (5 mL) was added to a 10-mL flask, and then 5% NaNO2 solution (0.3 mL) was added thereto. The samples were mixed well and placed at room temperature for 6 min, and 5% Al(NO3)3 solution (0.3 mL) was added to the flask. The samples were mixed well and placed at room temperature for 6 min, and 4% NaOH solution (4.4 mL) was added. The samples were mixed well and placed at room temperature for 12 min. Thereafter, the absorbance of the samples was read at 510 nm, and rutin was used as the standard for the calibration curve.
Determination of Total Polysaccharide Content
Polysaccharide content was determined using the phenol-sulfuric acid colorimetric method [13] with some modifications. The leaf powder (0.1 g) was extracted with distilled water (20 mL) at 100°C for 4 h. The aqueous extract was concentrated and then mixed with five volumes of cold absolute ethanol to precipitate the polysaccharides. The precipitate was cooled at 4°C for 12 h before being collected via centrifugation (4000 g, 25 min). The residue was then dried to obtain the desired polysaccharides. The polysaccharides were dissolved in water and diluted to 50 mL in a volumetric flask. The polysaccharide solution (1 mL) was mixed with 5% phenol (1 mL) and concentrated sulfuric acid (5 mL). The mixture was shaken and incubated at 100°C for 15 min. After cooling, absorbance of the samples was read at 490 nm, and D-glucose was used as the standard for the calibration curve.
Determination of Total Polyphenol Content
Total polyphenol content was determined using the Folin-Ciocalteu method [14] with some modifications. The leaf powder (10 g) was added into a 100-mL conical flask; 80% ethanol (50 mL) was into the flask, which was sealed for 3 days. The conical flask was placed in an ultrasonic cleaner for extraction three times. The combined filtrate was concentrated on a rotary evaporator (40°C), alcohol-free. The alcohol-free extract was transferred into a 100-mL volumetric flask, and the volume was made up with distilled water. Thereafter, 1 mL of the solution was transferred to a 10-mL volumetric flask, and the volume was made up with distilled water to prepare the sample solution. The sample solution (1.0 mL) was added into a 10-mL volumetric flask, to which distilled water (3 mL) and FC chromogenic agent (0.5 mL) were added. After shaking, 20% NaCO3 (1.5 mL) was added within 8 min. The volume was made up with distilled water, and the flask was placed in a water bath at 75°C for 10 min. Absorbance of the sample was read at 760 nm, and gallic acid was used as the standard for a calibration curve.
Determination of Total Saponin Content
Total saponin content was determined using the colorimetric assay according to the method described by Xu et al. [15] with some modifications. The leaf powder (2 g) was placed in a Soxhlet extractor, diethyl ether (30 mL) was added to degrease until colorless, and then volatilized the diethyl ether. Thereafter, methanol (30 mL) was used for reflux extraction for 6 h. The methanol solution was recovered at a small amount; the volume of the sample in the volumetric flask was maintained at 10 mL with methanol. The supernatant was centrifuged as the sample solution. The sample solution (0.5 mL) was transferred to a tube with a stopper, the solvent was evaporated, and 50 g·L−1 vanillin glacial-acetic acid (0.4 mL) and perchloric acid (1.6 mL) were added. The sample was mixed well, heated in a 70°C water bath for 20 min, and cooled in an ice bath for 2 min, glacial acetic acid (5 mL) was added, and shaken well. Absorbance of the sample was read at 545 nm, and ginsenoside Re was used as the standard for the calibration curve.
Determination of Total Triterpenoidic Acid Content
Total triterpenoidic acid content was determined using the colorimetric assay according to the method described by Xia et al. [16] with some modifications. The leaf powder (10 g) was ultrasonically extracted with methanol (50 mL) for 40 min; the solution was cooled and weighed again. Thereafter, the mass loss was compensated with methanol to obtain the sample solution. The sample solution (0.3 mL) was evaporated methanol in a water bath, 5% vanillin glacial-acetic acid solution (0.3 mL) and perchloric acid (1.0 mL) were added, sealed, and mixed well. The sample was allowed to develop color in a 60°C water bath for 45 min and cooled in an ice bath; thereafter, glacial acetic acid was added to a constant volume of 5.0 mL. Absorbance of the sample was read at 550 nm, and ursolic acid was used as the standard for the calibration curve.
MS Excel was used for the statistical analysis of leaf shape diversity. Differences in photosynthetic pigment content, photosynthetic properties, and active compound content were analyzed using the one-way ANOVA function in SPSS 26.0 software (IBM, USA) (p < 0.05). Principal component analysis of photosynthetic characteristics and active compound content of heteromorphic leaves was carried out using SPSS 26.0 software.
3.1 Variation in Semiliquidambar Cathayensis Leaf Shape
The variation in the leaf shape of the offspring of 18 S. cathayensis individuals from three populations was statistically analyzed (Fig. 1, Table 1). The three leaf shapes accounted for different proportions in the offspring of the three populations; the offspring of the JH population was dominated by entire leaves at an average of 50.88%, whereas the proportion of entire leaves observed in the JZ and DY populations was 42.61% and 26.00%, respectively. The offspring of the DY population was dominated by palmately trifoliate leaves, with an average of 56.76%. The differences in leaf shape among the populations were statistically significant (p < 0.05), indicating that leaf shape of S. cathayensis had a high degree of dispersion at the species level and that geographic population differentiation was either extremely variable or had already occurred. The coefficient of variation (CV) represents the variability of phenotypic traits within the population, with a higher CV indicating greater dispersion of phenotypic traits. In the three populations, the CVs increased as follows: JZ < JH < DY for entire leaves, JZ < DY < JH for asymmetrically lobed leaves, and JZ < DY < JH palmately trifoliate leaves. The UPGMA (unweighted pair-group method with arithmetic means, UPGMA) clustering map (Fig. 2) showed that the 18 single offspring groups of the three natural S. cathayensis populations were divided into two clusters, among which four single offspring groups from the DY population (DY) were clustered in Cluster II and the other 14 single offspring groups were clustered in Cluster I. Cluster I was subdivided into two subgroups; most of the sample plants from the JH population were clustered in subgroup Ib, whereas JH01, JH05, JH08, and the JZ population were clustered in subgroup Ia.
Figure 1: Semiliquidambar cathayensis leaf traits found in southern China. (a) The leaves of S. cathayensis clustered at the top of a branch. The (b) entire leaf; (c) asymmetrically lobed leaf; and (d) palmately trifoliate leaf traits observed within the tested populations
Figure 2: Clustering relationship of different individual Semiliquidambar cathayensis plants based on leaf shape traits
3.2 Analysis of Photosynthetic Pigment Content in the Leaves
The photosynthetic pigment content in S. cathayensis leaves differed significantly (p < 0.05) among the different leaf shapes. The Chla, Chlb, and carotenoid content in the entire leaves were significantly lower than those in the two other leaf shapes. When the palmately trifoliate leaves were used as the control group, the Chl (a+b) content in the entire leaves was only 71.44% of that in the palmately trifoliate leaves, and the carotenoid content was 77.57% that of the palmately trifoliate leaves (Table 2).
3.3 Analysis of Physiological Parameters of Photosynthesis in Semiliquidambar Cathayensis Leaves
A right-angled hyperbolic correction model was used to fit the light response curves of different S. cathayensis leaf shapes to a high degree (Fig. 3), with coefficients of determination (R2) of 0.9993, 0.9986, and 0.9989 (entire leaves, asymmetrically lobed leaves and palmately trifoliate leaves). In general, the net photosynthetic rate of leaves of different shapes increased with increasing chlorophyll content. When we compared the three fitted light response curves, there were significant differences (p < 0.05) in the apparent quantum yield (AQY), maximal net photosynthetic rate (Pn-max), light saturation point (LSP), and dark respiration rate (Rd); whereas, the difference in light compensation point (LCP) was not statistically significant (p > 0.05). The AQY, Pn-max, LSP, and Rd increased with increasing chlorophyll content in each leaf shape, and the LSP of entire leaves, asymmetrically lobed leaves, and palmately trifoliate leaves were 985.374, 1037.858, and 1245.467 μmol·m−2s−1, respectively. As the light intensity increased, photoinhibition was induced and the net photosynthetic rate decreased (Table 3).
Figure 3: Light response of photosynthesis in heteromorphic Semiliquidambar cathayensis leaves
3.4 Analysis of Active Compounds in Semiliquidambar Cathayensis Leaves
The content of active compounds, including total flavonoids, polysaccharides, polyphenol, triterpenoidic acids, and saponins, varied considerably among different S. cathayensis leaf shapes with some differences in the level of statistical significance (Table 4). The total flavonoid content did not differ significantly among the three leaf shapes, whereas the total polysaccharide, polyphenol, and saponin content in the entire leaves were significantly lower than those in the other two leaf shapes. The total polysaccharide content in the entire leaves was 77.05% of that in the asymmetrically lobed leaves, and 74.53% of that in the palmately trifoliate leaves. The total polyphenol content in the entire leaves was 67.79% of that in the asymmetrically lobed leaves, and 78.25% of that in the palmately trifoliate leaves. The palmately trifoliate leaves had the lowest total triterpenoidic acids content (13.538 mg·g−1), which was 67.42% and 68.23% of that in the entire leaves and the asymmetrically lobed leaves, respectively.
3.5 Relationship among Photosynthetic Pigments, Photosynthetic Properties, and Active Compounds in Semiliquidambar Cathayensis Leaves
SPSS 26.0 software was used to conduct principal component analysis on the photosynthetic characteristics and active components of three different leaf shapes, and two principal component factors were extracted. The results are shown in Table 5. The contribution rate of the first principal component (Y1) was 74.158%, which mainly reflected the photosynthetic pigment content, photosynthetic efficiency, total triterpene acid, total polysaccharide, and total saponin content of leaves. The contribution rate of the second principal component (Y2) was 18.610%, which mainly reflected the content of total flavonoids and total polyphenol in the leaves. The cumulative contribution value of the first two principal components was 92.768%, which indicates that the first two principal components extracted can represent the nine indexes including the photosynthetic characteristics and active ingredient characteristics of leaves. According to the eigenvalues and contribution rates of the two principal components, the scores of each principal component and the comprehensive scores are shown in Table 6. The high score of principal component Y1 for the three different leaf shapes indicates that it has the advantage of photosynthetic characteristics and the content of total triterpenoidic acids, total polysaccharides and saponins are high. The order of Y1 score was as follows: palmately trifoliate leaves > asymmetrically fallen leaves > entire leaves. The leaf shape with a high score of principal component Y2 indicates that the content of total flavonoids and total polyphenol was high. The order of Y2 score was as follows: asymmetrically lobbed leaves > palmately trifoliate leaves > entire leaves. The comprehensive score (y) was sorted as follows: asymmetrically lost leaves > palmately trifoliate leaves > entire leaves.
Analysis of the genetic diversity in leaf shape traits can be used to obtain a preliminary assessment of the potential characteristics of germplasm resources [17]. Leaf shape is a structural trait that can remain stable under relatively unstable environmental conditions [18]. In the present study, statistical analysis of leaf shape in the offspring of three natural S. cathayensis populations showed that the proportion of the three leaf shapes varied among these populations, and the variation in leaf shape was maintained at a high level reflecting genetic diversity among and within the populations. In general, the phenotypic diversity observed in populations is related to the number of samples, as populations with a higher number of samples tend to have higher phenotypic diversity [19]. When we compared the sample size and diversity proportions of these S. cathayensis populations, we found that the JH population had the highest number of samples and a large CV for the three leaf shapes. Although the DY population had the smallest number of samples, the CV of the asymmetrically lobed and palmately trifoliate leaves was higher than that of the JZ population. In addition, the UPGMA cluster mapping showed that a single offspring of the DY population clustered only in Cluster II, whereas the offspring of the JH and JZ populations were clustered in Cluster I. This indicated that the DY population had relatively high phenotypic diversity and variation. Genetic diversity is the basis of the evolutionary potential of a species to adapt to changes in its external environment [20]. S. cathayensis is a natural hybrid between the genera Populus and Salix with a complex genetic background and high heterozygosity [21,22]. Altingiaceae is the basal taxon of the superrosids, and the high genetic diversity of leaf shape traits in S. cathayensis may be the result of the Altingiaceae phylogeny as an ancient tree species, or it may be related to S. cathayensis being a natural hybrid. The results of this study provide a scientific basis for the taxonomic conservation of different germplasm resources of S. cathayensis.
As a basic structural and functional unit, the leaf is the principal organ for photosynthesis and the energy converter for primary producers in an ecosystem [23]. The study of the photosynthetic properties of different S. cathayensis leaf shapes is essential for its introduction, cultivation, and processing. Photosynthetic pigments are the foundation of photosynthesis in S. cathayensis and are an important indicator of photosynthetic activity [24]. Higher chlorophyll content in plant leaves results in an improved ability of plants to capture light energy at low light intensities, and this facilitates photosynthesis and increases organic matter accumulation. In the light response curve model, the AQY indicates not only increased net photosynthetic rate at low light intensities, but also light energy utilization at low light intensities [25]. The palmately trifoliate leaves had a higher total chlorophyll and carotenoid content and the AQY values were significantly higher than those of entire and asymmetrically lobed leaves, indicating that the light use efficiency (LUE) of palmately trifoliate leaves was significantly higher than that of entire and asymmetrically lobed leaves at low light intensities. The LCP and LSP reflect the light condition requirements and degree of adaptation, whereas Pn-max is a characterization of LUE and photosynthetic capacity in plants [26]. Here, the palmately trifoliate leaves of S. cathayensis had significantly higher AQY, Pn-max, LSP, and LCP than the other leaf shapes, although this difference was not significant. However, these results suggest that the palmately trifoliate leaves may have higher photosynthetic activity and increased light energy utilization that could improve light energy conversion efficiency and dry matter accumulation. The total chlorophyll and carotenoid content were the lowest and Pn-max, LSP, and LCP were significantly lower in entire leaves than in the palmately trifoliate leaves, but not in asymmetrically lobed leaves, indicating there was no significant difference in photosynthetic efficiency between entire and asymmetrically lobed leaves. Hence, palmately trifoliate leaves are an indicator of higher photosynthetic efficiency in this species.
The quality of S. cathayensis, which is not included in Chinese Pharmacopoeia, has not yet been evaluated using uniform standards [27]. From current research on the active compounds in S. cathayensis roots and leaves, the known active compounds primarily include flavonoids, polysaccharides, alkaloids, saponins, triterpenoids, and sterols [28]; however, this has not been systematically evaluated. Yang et al. [29] identified 85 chemical constituents from the roots of S. cathayensis, including 35 alkaloids, 12 flavonoids, 7 terpenoids, 5 phenylpropanoids, 9 fatty acids, 7 cyclic peptides, and 10 other compounds. Tian et al. [30] identified 38 significantly different metabolites among S. cathayensis leaves, stems, and roots. These metabolites were differentially expressed in these tissues; the terpenoids were primarily triterpenoids and most of the flavonoids were significantly enriched in the leaves. In the present study, the analysis of active compounds in S. cathayensis leaves of different shapes revealed that the total polysaccharide, phenol, and saponin content in palmately trifoliate leaves were significantly higher than those in the other two leaf shapes, but the total triterpene content was lower than that in the entire leaves and asymmetrically lobed leaves. The utilization of light energy by plants reflects their ability to convert this energy into organic matter [31,32], and the active compounds that determine their use as medicinal herbs are derived directly or indirectly from photosynthesis during plant growth and development [33]. By comparing and analyzing the differences in photosynthetic characteristics and active components among the three leaf shapes of S. cathayensis, the principal component analysis was carried out on each characteristic index, and two principal components with a cumulative contribution rate of 92.768% were extracted to comprehensively evaluate the photosynthetic characteristics and active components of the three leaf shapes. The photosynthetic efficiency and active component content of asymmetrically lobed leaves were the highest, followed by those of palmately trifoliate leaves and entire leaves.
In this study, we found that the leaf shape of different S. cathayensis germplasm resources exhibited some correlation between photosynthetic properties and active compounds. The total proportion of asymmetrically lobed leaves and palmately trifoliate leaves in the DY population was higher than that of the other two populations, suggesting the total active compound content in S. cathayensis leaves was higher in the DY population than in the JZ and JH populations. In actual production, we can predict active compound content based on S. cathayensis leaf shape to select and breed the required varieties based on medicinal purposes; thus, the use of leaf shape provides a simple and effective method for classifying S. cathayensis germplasms and for screening elite germplasms.
Supplementary Materials: Table S1: Geographic distribution information of three natural populations of Semiliquidambar cathayensis.
Authorship: The authors confirm their contribution to the paper as follows: Conceptualization: Xiaoming Tian; Methodology: Xiaoming Tian, Guangfeng Xiang; Software: Xiaoming Tian; Investigation: Peng Jing; Resources: Guangfeng Xiang, Lu Zhu; Data curation: Lu Zhu; writing original draft preparation: Xiaoming Tian. All authors have read and agreed to the published version of the manuscript.
Funding Statement: This research was funded by Changsha Natural Science Foundation (Grant No. kq2202356) and Hunan Forestry Science and Technology Innovation Plan Project (Grant No. XLK202106-2).
Conflicts of Interest: The authors declare that they have no conflicts of interest to report regarding the present study.
References
1. Tang, X., Fan, H., Zhang, J., Huang, Y. (2020). The complete chloroplast genome of Semiliquidambar cathayensis HT Chang ‘T5’ (Hamamelidaceae). Mitochondrial DNA Part B: Resources, 5(2), 1267–1268. [Google Scholar]
2. Tian, X., Yan, L., Jiang, L., Li, G., Wu, J. (2022). Comparative transcriptome analysis of leaf, stem, and root tissues of Semiliquidambar cathayensis reveals candidate genes involved in terpenoid biosynthesis. Molecular Biology Reports. DOI 10.21203/rs.3.rs-1249220/v1. [Google Scholar] [CrossRef]
3. Liu, Z., Kaliaperumal, K., Chen, H., Zhang, J. (2022). Anti-inflammatory activity of the water extract of Semiliquidambar cathayensis leaf. Natural Product Research, 43(5), 1–7. DOI 10.1080/14786419.2022.2056892. [Google Scholar] [CrossRef]
4. Zhang, J. Y., Kaliaperumal, K., Liu, Z. Y., Zhang, J. (2022). Chemical constituents from Semiliquidambar chingii and their chemotaxonomic significance. Biochemical Systematics and Ecology, 100, 10436. [Google Scholar]
5. Lu, H. X., Wu, Z. L., Liang, W. J., Chen, M. L., Huang, B. B. et al. (2015). Chemical constituents from Semiliquidambar cathayensis roots. Journal of Chinese Medicinal Materials, 38(12), 2543–2546. [Google Scholar]
6. Wang, H. Z., Han, L., Xu, Y. L., Liu, Y. P., Wang, L. (2019). Chlorophyll fluorescence characteristics of photosystem II of Populus euphratica heteromorphic leaves. Acta Botanica Boreali-Occidentalia Sinica, 39(10), 1795–1804. [Google Scholar]
7. Zhuang, Q., Wu, S., Chen, K., Zhao, G., Qian, F. (2015). Preliminary researches on influence of several kinds of plants including Semiliquidambar cathayensis Chang on activity of cholinesterase from pig serum. International Conference on Energy Equipment Science and Engineering (ICEESE), pp. 357–360. Guangzhou, China. [Google Scholar]
8. Ye, X. Z., Zhao, G. H., Zhang, M. Z., Cui, X. Y., Fan, H. H. et al. (2020). Distribution pattern of endangered plant Semiliquidambar cathayensis (Hamamelidaceae) in response to climate change after the last interglacial period. Forests, 11(4), 434. [Google Scholar]
9. Ye, X. Z., Wen, G. W., Zhang, M. Z., Liu, Y. P., Fan, H. H. et al. (2021). Genetic diversity and genetic structure of a rare and endangered species Semiliquidambar cathayensis Hung T. Chang. Plant Science Journal, 39(4), 415–423. [Google Scholar]
10. Lichtenthaler, H. K., Buschmann, C., Rinderie, U., Schmuck, G. (1986). Application of chlorophyll fluorescence in ecophysiology. Radiation and Environmental Biophysics, 25(4), 297–308. [Google Scholar]
11. Deng, G. C., Hou, S. M., Tian, D. M., Zhang, Y. Y., Zhang, X. et al. (2006). Separation and determination of selenium polysaccharide in enriched-Se Cordyceps militaris. Spectroscopy and Spectral Analysis, 26(3), 522–525. [Google Scholar]
12. Zhang, M., Cao, J., Dai, X., Chen, X., Wang, Q. (2012). Flavonoid contents and free radical scavenging activity of extracts from leaves, stems, rachis and roots of Dryopteris erythrosora. Iranian Journal of Pharmaceutical Research, 11, 991–997. [Google Scholar]
13. Zhang, A., Shen, Y., Cen, M., Hong, X., Shao, Q. et al. (2019). Polysaccharide and crocin contents, and antioxidant activity of saffron from different origins. Industrial Crops and Products, 133, 111–117. [Google Scholar]
14. Chen, G. L., Hu, K., Zhong, N. J., Guo, J., Gong, Y. S. et al. (2013). Antioxidant capacities and total polyphenol content of nine commercially available tea juices measured by an in vitro digestion model. European Food Research and Technology, 236(2), 303–310. [Google Scholar]
15. Xu, H., Xu, Z., Zhu, D. (2003). Resources investigation and determination of total saponin content in Polygala fallax from Guangxi. Journal of Plant Resources and Environment, 12(1), 47–49. [Google Scholar]
16. Xia, L., Chen, Y., Feng, Y., Jiao, Z., Liu, H. et al. (2016). Changes in flavonoids, total phenolics, triterpenoidic acids and antioxidant capacity during fruit development of different cultivars of apricot. Journal of Fruit Science, 33(4), 425–435. [Google Scholar]
17. Koyuncu, F., Koyuncu, M. A., Yıldırım, F., Vural, E. (2004). Evaluation of black mulberry (Morus nigra L.) genotypes from Lakes region, Turkey. European Journal of Horticultural Science, 69(3), 125–131. [Google Scholar]
18. Cui, D., Cui, G., Yang, R., She, W., Liu, Y. et al. (2021). Phenotypic characteristics of ramie (Boehmeria nivea L.) germplasm resources based on UAV remote sensing. Genetic Resources and Crop Evolution, 68(2), 551–566. [Google Scholar]
19. Livanios, I., Lazaridi, E., Bebeli, P. J. (2018). Assessment of phenotypic diversity in bitter vetch (Vicia ervilia (L.) Willd.) populations. Genetic Resources and Crop Evolution, 65(1), 355–371. [Google Scholar]
20. Przybylski, P., Mohytych, V., Rutkowski, P., Tereba, A., Tyburski, Ł. et al. (2021). Relationships between some biodiversity indicators and crown damage of Pinus sylvestris L. in natural old growth pine forests. Sustainability, 13(3), 1239. [Google Scholar]
21. Wu, W., Zhou, R., Huang, Y., Boufford, D. E., Shi, S. (2010). Molecular evidence for natural intergeneric hybridization between Liquidambar and Altingia. Journal of Plant Research, 123(2), 231–239. [Google Scholar]
22. Zhang, M., Jiang, Y., Ye, X., Chen, S., Fan, H. et al. (2020). The complete chloroplast genome of Semiliquidambar cathayensis (Hamamelidaceae). Mitochondrial DNA Part B: Resources, 5(1), 695–696. [Google Scholar]
23. Henry, R. J., Furtado, A., Rangan, P. (2020). Pathways of photosynthesis in non-leaf tissues. Biology, 9(12), 438. [Google Scholar]
24. Shi, S. B., Zhu, W. Y., Li, H. M., Zhou, D. W., Han, F. et al. (2004). Photosynthesis of Saussurea superba and Gentiana straminea is not reduced after long-term enhancement of UV-B radiation. Environmental and Experimental Botany, 51(1), 75–83. [Google Scholar]
25. Cao, Y., Zhou, B., Chen, S., Xiao, J., Wang, X. (2011). The photosynthetic physiological properties of Illicium lanceolatum plants growing under different light intensity conditions. African Journal of Agricultural Research, 6(26), 5736–5741. [Google Scholar]
26. Zhao, M., Ding, Z., Lafitte, R., Sacks, E., Dimayuga, G. et al. (2010). Photosynthetic characteristics in Oryza species. Photosynthetica, 48(2), 234–240. [Google Scholar]
27. Shi, Y., Duan, N., Liu, B. (2019). Complete chloroplast genome sequence of Semiliquidambar cathayensis (hamamelidaceaea rare and endangered species endemic to China. Mitochondrial DNA Part B: Resources, 4(2), 3252–3253. [Google Scholar]
28. Zhou, G. X., Yang, Y. C., Shi, J. G., Yang, W. L. (2002). Studies on chemical constituents from Semiliquidambar cathayensis. Chinese Traditional and Herbal Drugs, 33(7), 16–18. [Google Scholar]
29. Yang, L., Liu, R. H., He, J. W. (2019). Rapid analysis of the chemical compositions in Semiliquidambar cathayensis roots by ultra high-performance liquid chromatography and quadrupole time-of-flight tandem mass spectrometry. Molecules, 24(22), 4098. [Google Scholar]
30. Tian, X. M., Yan, L. H., Jiang, L., Xiang, G. (2021). Chemical comparison of different tissues of Semiliquidambar cathayensis Chang by UHPLC-QTOF/MS-based metabolomics. Plant Physiology Journal, 57, 1311–1318. [Google Scholar]
31. Ku, M. S., Wu, J., Dai, Z., Scott, R. A., Chu, C. et al. (1991). Photosynthetic and photorespiratory characteristics of flaveria species. Plant physiology, 96(2), 518–528. [Google Scholar]
32. Wang, Q., Zhang, Q. D., Jiang, G., Lu, C. M., Kuang, T. Y. et al. (2000). Photosynthetic characteristics of two superhigh-yield hybrid rice. Acta Botanica Boreali-Occidentalia Sinica, 42(12), 1285–1288. [Google Scholar]
33. Yang, L., Zhao, Y., Zhang, Q., Cheng, L., Han, M. et al. (2019). Effects of drought-re-watering-drought on the photosynthesis physiology and secondary metabolite production of Bupleurum chinense DC. Plant Cell Reports, 38(9), 1181–1197. DOI 10.1007/s00299-019-02436-8. [Google Scholar] [CrossRef]
Cite This Article
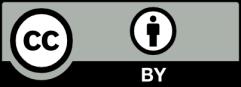
This work is licensed under a Creative Commons Attribution 4.0 International License , which permits unrestricted use, distribution, and reproduction in any medium, provided the original work is properly cited.