Open Access
ARTICLE
Leaf Wettability Difference Among Tea Leaf Ages and Analysis Based on Microscopic Surface Features
1
School of Agricultural Engineering, Jiangsu University, Zhenjiang, 212013, China
2 National Institute of Measurement and Testing Technology, Chengdu, 610021, China
* Corresponding Author: Yongguang Hu. Email:
(This article belongs to the Special Issue: Plant–Environment Interactions)
Phyton-International Journal of Experimental Botany 2023, 92(2), 411-421. https://doi.org/10.32604/phyton.2022.023437
Received 26 April 2022; Accepted 06 June 2022; Issue published 12 October 2022
Abstract
The wettability of leaf surface, commonly represented by contact angle (CA), affects various physiological and physical processes. The present study aims to better understand the wettability of tea leaves and elucidate its influence on the energy barrier of the droplet condensation process. The CA values of different leaf ages (young, mature and old) of five famous tea cultivars (Maolu, longjing 43, Huangjinya, Zhongcha 108 and Anji Baicha) were measured via the sessile drop method, and the micro-morphology of two cultivars leaves (Maolu, Zhongcha 108) was investigated by a 3D super depth-of-field digital microscope. Specifically, two radically distinctive types of CA trends were observed, one was the decreased firstly and then increased slightly with the increase of leaf age, while the other stayed constant. The valley depth or maximum height (RZ) of Maolu leaf surface increased with the leaf age while the RZ of Zhongcha 108 leaf remained unchanged by comparing the microscopic features. The Maolu mature leaf CA decline attributed to the young leaf was hydrophilic (θ < 90°), and it was considered that surface structures like folds and pits on old leaf played a crucial role in making CA increased. Small deviation in CA can lead to significant error in calculation of the contact angle function of energy barrier in phase change. It will have great significant for simulating and better understanding the formation of frost on tea leaves.Keywords
Tea (Camellia sinensis [L.] O. Kuntze) is one of the high-value cash crops in hilly areas of China, and tea products has become the most consumed beverage worldwide [1]. Frost happening frequently in late spring causes serious damages on tea leaves, resulting in huge quality reduction and economic losses [2,3]. Generally, before frost forms on the surface, there will be visible condensation droplets on it [4–6]. After the liquid droplet changes to a solid state, frost crystals and branches grow on the frozen droplets [7]. Wettability of surface directly affects the droplet condensation and solidification in frosting [8]. In addition, leaf wettability is also an important characteristic related to canopy water retention, gas exchange [9] and bacterial transmission [10], which affects the quality of tea.
Wettability is most often assessed on the basis of contact angle (CA) value, measured between a horizontal solid surface and fluid interface [11]. The CA is usually a constant quantity when a droplet statically spread on a horizontal surface [12]. The classical Young’s model [13] was employed to describe this ideal wetting phenomenon (Fig. 1a). However, absolutely smooth leaf surfaces are nonexistent in nature, which most typical example is ‘lotus effect’ [14]. Wenzel [15] considered the bottom liquid of the droplet infiltrate the micro-structure and modified Young’s model by taking the surface roughness into consideration (Fig. 1b). Afterwards, Cassie et al. [16] proposed a new theoretical model by considering the proportion of different components on the surface (Fig. 1c). In this state, composite interface underneath the liquid was formed by air pockets and play a key role to help us to understand the wetting behavior.
Figure 1: Three classic wetting models, where γSL, γSA and γLA are the interfacial tensions of solid-liquid, solid-air, liquid-air, respectively; θ is contact angle (CA)
Generally, the wetting traits of a solid surface can be classified into three types. First one is the superhydrophobic surface, when the CA of droplet is over 160°, the surface is considered to be water repellency. The second type is a hydrophobic surface with a CA above 90°. The last one is the hydrophilic surface which can be easily wetted by water with CA lower than 90° [17]. Leaf CA is usually measured by the sessile droplet method using a contact angle meter even though it is not an artificial solid surface [18]. Droplet will roll off and without water spread if leaf surface has hydrophobic property. Conversely, the leaf surface shows hydrophilic property when droplets spread or adhere to it [19].
The wetting behavior of plant surfaces is much more complicated with limited researches than that of abiotic rigid surfaces. Hanba et al. [20] investigated the influence of leaf wettability on photosynthesis in bean and pea. The CO2 assimilation of bean leaves (hydrophilic) differs from that of pea leaves (hydrophobic) and will be reduced after subjecting them to water mist. Papierowska et al. [21] pointed that leaf wettability has effect on droplet splashing phenomenon, which also influence diseases spreading [22]. The largest dimensionless diameter of droplet on leaves decreased with hydrophobic property increased. Tea leaf, Zhu et al. [23] analyzed the influence of wettability on surfactant droplet maximum retention. Several factors affecting the leaf wettability have also taken into account and discussed. Holder [24] measured the CA of 36 species of tree leaves and found that leaf wettability varied greatly between regions. Zhu et al. [25] observed that rice leaf CA increased with growth and there were no distinct difference between cultivars. Papierowska et al. [18] indicated that the wettability of potato leaf was closely related to trichome types and density. Moreover, wax mass and structure also influence leaf wettability [26].
Interestingly, there are some intrinsically different conclusions about the effect of leaf age on leaf wettability. Holm oak leaf CA decrease with the increase of leaf age whether on the adaxial or abaxial surface [27]. This indicates that the CA of young holm oak leaf is maximum. However, not all plant leaf CA values are distributed so regularly. For example, abaxial surface CA of European beech young leaf at top canopy is minimal in October [28]. In addition, young leaf of rice is more easily wetted compared to leaves with other ages, which also means that young leaf CA is minimal [25]. It can be seen that leaf age has different effects on leaf wettability of different plants. Meanwhile, the effect on the leaf age of tea leaf is still poorly studied.
Therefore, the study aims to quantitatively describe the influence of tea leaf ages on its wettability by measuring CA, and a microscopic analysis was conducted to interpret the differences in wettability. It can provide a theoretical basis for simulating and better understanding the formation of frost on tea leaf.
2.1 Leaf Material and Preparation
Five cultivars of famous tea in Jiangsu Province, China were selected for this research. These cultivars were Maolu, longjing 43, Anji Baicha, Zhongcha 108 and Huangjinya. Six fresh leaves of different age (young, mature and old) of each cultivar were collected and transported to the laboratory in a cool chamber on December 02, 2021. Those leaves were rinsed using deionized water to remove surface contaminants and then dried at ambient temperature. Both left and right sides of the midrib were cut off and 12 test samples of each cultivar were made. To obtain a flat surface, each part of abaxial surface was carefully fixed to glass slide with double sided adhesive tape.
In this study, young leaves were from the second or third fully unfolded leaf below the terminal bud; leaves that were 5 to 7 leaves away from the bud were considered mature leaves and those having many years of age on the bottom canopy were selected as old leaves. All leaves were guaranteed to be intact without any injury.
2.2 Contact Angle (CA) Measurements
The wettability of tea leaves of various ages of each cultivar was obtained by measuring a distilled water droplet CA with a device (CAM 200, KSV, Finland, Fig. 2a). It was connected to a computer with built-in software for data processing. The measurement was conducted in the laboratory via the sessile drop method, given the ambient temperature of 293 K and relative humidity of 60%. The critical volume of the pure water droplet for measuring the leaf CA was about 10 μL [9]. The droplet with the volume of 10 μL was generated through a syringe, shown in Fig. 2b, and photographed roughly 20 s after dropping. The CA of the adaxial leaf surface was measured 10 times and the average value was taken as the final result.
Figure 2: Experimental device and measuring method. (a) Schematic diagram of contact angle meter; (b) water droplet of 10 μL was measured via the sessile drop method
An analysis of variance (ANOVA) was performed on the differences in the measured data using Origin. Tukey test was used for mean comparison and significance levels were taken as 0.05 [9]. There was a significant difference between the two sets of data if the P-value was less than 0.05.
2.3 Microscopic Leaf Observation
A 3D super depth-of-field digital microscope (VHX-200, KEYENCE, Japan) was used for observing the surface structure of the examined leaves by photographing at the same magnification (×500). The microscope allowed easy observation of the three-dimensional leaf surface morphology as well as measurement of the desired parameters. It was found that the center light source at the lens made the images clearer. Three photographs of each sample were chosen from a three times repeated experiment.
2.4 Calculation of Energy Barrier
Water vapor will condense into liquid cores if it touches a cooler surface. It can spontaneously condense vapor molecules to a droplet when the liquid core was larger than a certain radius. The Gibbs free energy of the system caused by the formation of a critical liquid core is called the energy barrier. According to the phase transformation dynamics, the energy barrier was considered as:
where ϕ is a single molecule volume; ∆g is the energy change of a single molecule. Assuming that the droplets condensed on a leaf surface are spheroidal as shown in Fig. 1a, then its volume was:
The contact areas between the different phases will be:
where SLA and SSL are the contact area of liquid-air and solid-liquid, respectively; SSA is the reduced area of solid-air; θ is CA and R is the sphere radius .
So the ∆Gc can be derived as [29]:
In particular, f(θ) is the contact angle function:
3.1 Contact Angle Measurements
The different letters above the columns of Fig. 3 represent that the P-value between any two data sets was lower than 0.05 and there was a significant difference. Maolu leaves CA values were significantly different (Fig. 3a). The CA values showed the obvious trend that firstly decreased and then increased and had no varied gradient with the leaf age. The young leaf CA was 86.33° which was 13.39° and 6.32° higher than that on mature and old leaf CA, respectively. Meanwhile, the CA of old leaf increased 7.07° vs. mature leaf CA.
Figure 3: The leaf CA of 5 cultivars
A similar distribution of leaf CA values was also observed for longjing 43 and Huangjinya leaves. The CA of young leaf was maximum while the mature leaf CA firstly decreased and then old leaf CA increased slightly, as shown in Figs. 3b and 3c. However, Zhongcha 108 and Anji Baicha leaves CA values kept nearly constant with the various leaf ages (Figs. 3d and 3e).
Leaves of different ages had CA values less than 90° and were considered hydrophilic surface according to accepted classification criteria [17–19]. The CA of Huangjinya was the largest among young leaves, but it was still below 90°. For mature leaves, it was found that the CA of Huangjinya decreased compared with that of the young leaf. Mature leaf CA of Zhongcha 108 was the largest, while the CA of Maolu was the smallest which was around 70°. Remarkably, Huangjinya old leaf CA was the largest value (87.21°). The maximum and minimum average CAs were on Huangjinya and Maolu with values of 85.02° and 79.76°, respectively.
3.2 Microscopic Features of Leaves
The θ of Maolu, longjing 43 and Huangjinya had the trend of first decreased and then increased. However, the CA of Maolu’s leaf had the most obvious differences among leaf ages, while those of Zhongcha 108 and Anji Baicha leaves of different ages were similar. Maolu and Zhongcha 108 leaf surfaces had different CA trends and were selected for microscopic observation. The 3D morphology images of different leaves of Maolu and Zhongcha 108 are shown in Fig. 4.
Figure 4: 3D images of leaf surface morphology. (a), (c) and (e) are young, mature and old leaves of Maolu; (b), (d) and (f) are young, mature and old leaves of Zhongcha 108, respectively, with magnification of 500 times
Fig. 4a shows that the young leaf surface of Maolu was tender, and the valley depth or maximum height (RZ) was only 11.3 μm. On the contrary, the young leaf of Zhongcha 108 had obvious surface folds with a higher RZ of 23.4 μm (Fig. 4b). Figs. 4c and 4d show the morphology of mature leaves. The color of Maolu mature leaf was darker and the RZ increased to 19.6 μm. The surface morphology of Zhongcha 108 mature leaf was similar to the young leaf, RZ had a slight increase to 26.2 μm. In Fig. 4e, the surface color of Maolu old leaf turned to black green and the gaps were clear. The RZ of old leaf of Zhongcha 108 was 25.5 μm which had almost no change compared with that on young and mature leaves.
The schematic diagrams of Maolu leaf and CA variation are in Fig. 5. The young leaf was relatively smooth as the morphological observation results shown which can be simplified as an ideal flat surface (Fig. 5a). The classic Young’s model was used to describe this ideal wetness phenomenon:
Figure 5: Schematic diagrams of tea CA change (not to scale). The young leaf was considered as an ideal flat surface and CA was θ1; folds were increased on mature leaf and CA was θ2 which was lower than θ1; folds and pits of the old leaves were further deepened and CA was θ3 which was larger than θ2
where γSL, γSA and γLV are solid and liquid, solid and air, liquid and air interface tensions.
Revisiting the 3D morphology and structure results in Fig. 4c, obvious folds appeared on the surface with the growth of leaves, and the RZ of mature leaf increased. Inspired by classical wetting theories, the CA decreased can be reasonably interpreted by the Wenzel’s model:
where θW and θ are the Wenzel’s CA and the Young’s CA, respectively, r is the ratio of the actual area of droplet contact to the projected area on the horizontal plane, known as the roughness factor. A hydrophilic surface (
In nature, surface structure of plant leaves can significantly influence the CA. For instance, the homogeneous distribution of papillae structures on the superhydrophobic surface of lotus leaf [30], effectively support the bottom of the droplet. Anisotropic groove shape on the surface also make superhydrophobic the reed [31] and ginkgo leaves [32]. Machining similar surface structures is one method of increasing surface CA in industry. Those microscopic structures on leaves allow liquid to impregnate or partially penetrate into them. What’s more, the eggbeater shaped structure of salvinia leaf can form a thin air layer that prevents water from contacting the leaf surface further [33]. Similarly, the bounce of droplets falling at low velocity on other stationary droplet is also thought to be generated by the air between the surfaces [34].
A slight increase in CA of Maolu old leaves was observed instead of a decrease. We considered that the main cause of this result was that microscopic surface features like folds and pits developed further on the old leaf, and let droplets more difficult to intrude into the gaps, as shown in Fig. 5c. The effect of surface defects distribution and size on leaf CA needs further study.
3.3 Influence of CA on Energy Barrier
The
Figure 6: The variation of
The
Figure 7:
The CA values of tea leaf surfaces of different cultivars and various leaf ages were measured in this research which were all less than 90°, and belonged to hydrophilic surfaces. But two variation trends of CA were observed with increasing of leaf age. One was the trend of firstly descend and then slightly ascend with the leaf age. There was a gradual increase in the RZ of the leaf surface, the hydrophilic surface contributed to the CA decreases firstly. For the old tea leaf surface, there were obvious defects on it and these small folds or pits structures hinder the spreading of droplets which made the CA increases slightly. Another trend in CA is that it remained essentially constant with leaf age. The accurate CA of the target leaf should be measured when calculating the energy barrier. The CA error of a dozen degrees can cause the deviation in
Authorship: The authors confirm contribution to the paper as follows: study conception and design: Qingmin Pan, Yongguang Hu; data collection: Yongzong Lu; analysis and interpretation of results: Qingmin Pan, Liang Xue, Yongguang Hu; draft manuscript preparation: Qingmin Pan, Yongzong Lu. All authors reviewed the results and approved the final version of the manuscript.
Funding Statement: This research was funded by the Key Research and Development Program of Jiangsu Province (BE2021340), the Natural Science Foundation of the Jiangsu Higher Education Institutions of China (21KJB210019), the Jiangsu Postdoctoral Science Foundation (2021K614C), the Key Laboratory of Modern Agricultural Equipment and Technology, Jiangsu University (MAET202119), the Project of Postgraduate Innovation of Jiangsu Province (KYCX22_3683) and the Priority Academic Program Development of Jiangsu Higher Education Institutions (PAPD-2018-87).
Conflicts of Interest: The authors declare that they have no conflicts of interest to report regarding the present study.
References
1. Feng, L., Gong, Z. M., Liu, G. F., Liu, Y. L. (2020). Do strigolactones regulate bud winter dormancy and charactrisitc secondary metabolism in tea? Phyton-International Journal of Experimental Botany, 90(1), 65–73. DOI 10.32604/phyton.2020.012303. [Google Scholar] [CrossRef]
2. Hu, Y. G., Zhao, C., Liu, P. F., Asante, E. A., Li, P. P. (2016). Sprinkler irrigation system for tea frost protection and the application effect. International Journal of Agricultural and Biological Engineering, 9(5), 17–23. DOI 10.3965/j.ijabe.20160905.1315. [Google Scholar] [CrossRef]
3. Hu, Y. G., Asante, E. A., Lu, Y. Z., Mahmood, A., Buttar, N. A. et al. (2018). A review of air disturbance technology for plant frost protection. International Journal of Agricultural and Biological Engineering, 11(3), 21–28. DOI 10.25165/j.ijabe.20181103.3172. [Google Scholar] [CrossRef]
4. Wang, F., Liang, C. H., Yang, M. T., Zhang, X. S. (2015). Effects of surface characteristics on liquid behaviors on fin surfaces during frosting and defrosting processes. Experimental Thermal and Fluid Science, 61(4), 113–120. DOI 10.1016/j.expthermflusci.2014.10.022. [Google Scholar] [CrossRef]
5. Wang, F., Liang, C. H., Zhang, X. S. (2016). Visualization study of the effect of surface contact angle on frost melting process under different frosting conditions. International Journal of Refrigeration, 64(2), 143–151. DOI 10.1016/j.ijrefrig.2016.01.008. [Google Scholar] [CrossRef]
6. Kim, H., Kim, D., Jang, H., Dong, R. K., Lee, K. S. (2016). Microscopic observation of frost behaviors at the early stage of frost formation on hydrophobic surfaces. International Journal of Heat and Mass Transfer, 97(13–14), 861–867. DOI 10.1016/j.ijheatmasstransfer.2016.02.020. [Google Scholar] [CrossRef]
7. Song, M. J., Dang, C. B. (2018). Review on the measurement and calculation of frost characteristics. International Journal of Heat and Mass Transfer, 124(2), 586–614. DOI 10.1016/j.ijheatmasstransfer.2018.03.094. [Google Scholar] [CrossRef]
8. Chaudhary, G., Li, R. (2014). Freezing of water droplets on solid surfaces: An experimental and numerical study. Experimental Thermal and Fluid Science, 57(4), 86–93. DOI 10.1016/j.expthermflusci.2014.04.007. [Google Scholar] [CrossRef]
9. Matos, I. S., Rosado, B. H. P. (2016). Retain or repel? Droplet volume does matter when measuring leaf wetness traits. Annals of Botany, 117(6), 1045–1052. DOI 10.1093/aob/mcw025. [Google Scholar] [CrossRef]
10. Dong, X., Zhu, H. P., Yang, X. J. (2015). Characterization of droplet impact and deposit formation on leaf surfaces. Pest Management Science, 71(2), 302–308. DOI 10.1002/ps.3806. [Google Scholar] [CrossRef]
11. Rabbani, H. S., Zhao, B. Z., Juanes, R., Shokri, N. (2018). Pore geometry control of apparent wetting in porous media. Scientific Reports, 8(1), 15729–15737. DOI 10.1038/s41598-018-34146-8. [Google Scholar] [CrossRef]
12. Barman, J., Shao, W., Tang, B., Yuan, D., Groenewold, J. et al. (2019). Wettability manipulation by interface-localized liquid dielectrophoresis: Fundamentals and applications. Micromachines, 10(5), 329–346. DOI 10.3390/mi10050329. [Google Scholar] [CrossRef]
13. Tadmor, R. (2004). Line energy and the relation between advancing receding, and young contact angles. Langmuir, 20(18), 7659–7664. DOI 10.1021/la049410h. [Google Scholar] [CrossRef]
14. Neinhuis, W. B. (1997). Purity of the sacred lotus, or escape from contamination in biological surfaces. Planta, 202(1), 1–8. DOI 10.1007/s004250050096. [Google Scholar] [CrossRef]
15. Wenzel, R. N. (1936). Resistance of solid surfaces to wetting by water. Transactions of the Faraday Society, 28(8), 988–994. DOI 10.1021/ie50320a024. [Google Scholar] [CrossRef]
16. Cassie, A. B. D., Baxter, S. (1944). Wettability of porous surface. Transactions of the Faraday Society, 40, 546–551. DOI 10.1039/tf9444000546. [Google Scholar] [CrossRef]
17. Wang, G. Y., Guo, Z. G., Liu, W. M. (2014). Interfacial effects of superhydrophobic plant surfaces: A review. Journal of Bionic Engineering, 11(3), 325–345. DOI 10.1016/S1672-6529(14)60047-0. [Google Scholar] [CrossRef]
18. Papierowska, E., Szatyłowicz, J., Samborski, S., Szewińska, J., Rózańska, E. et al. (2020). The leaf wettability of various potato cultivars. Plants, 9(4), 504–517. DOI 10.3390/plants9040504. [Google Scholar] [CrossRef]
19. Holder, C. D. (2013). Effects of leaf hydrophobicity and water droplet retention on canopy storage capacity. Ecohydrol, 6(3), 483–490. DOI 10.1002/eco.1278. [Google Scholar] [CrossRef]
20. Hanba, Y. T., Moriya, A., Kimura, K. (2004). Effect of leaf surface wetness and wettability on photosynthesis in bean and pea. Plant, Cell & Environment, 27(4), 413–421. DOI 10.1046/j.1365-3040.2004.01154.x. [Google Scholar] [CrossRef]
21. Papierowska, E., Mazur, R., Stańczyk, T., Beczek, M., Szewińska, J. et al. (2019). Influence of leaf surface wettability on the drop splash phenomenon. Agricultural and Forest Meteorology, 279(2), 107762–107773. DOI 10.1016/j.agrformet.2019.107762. [Google Scholar] [CrossRef]
22. Gilet, T., Bourouiba, L. (2015). Fluid fragmentation shapes rain-induced foliar disease transmission. Journal of the Royal Society Interface, 12(104), 1092–1104. DOI 10.1098/rsif.2014.1092. [Google Scholar] [CrossRef]
23. Zhu, F., Cao, C., Cao, L. D., Li, F. M., Du, F. P. et al. (2019). Wetting behavior and maximum retention of aqueous surfactant solutions on tea leaves. Molecules, 24(11), 2094–2110. DOI 10.3390/molecules24112094. [Google Scholar] [CrossRef]
24. Holder, C. D. (2006). Leaf water repellency of species in Guatemala and Colorado (USA) and its significance to forest hydrology studies. Journal of Hydrology, 336(1), 147–154. DOI 10.1016/j.jhydrol.2006.12.018. [Google Scholar] [CrossRef]
25. Zhu, Y. Q., Yu, C. X., Li, Y., Zhu, Q. Q., Zhou, L. et al. (2014). Research on the changes in wettability of rice (Oryza sativa.) leaf surfaces at different development stages using the OWRK method. Pest Management Science, 70(3), 462–469. DOI 10.1002/ps.3594. [Google Scholar] [CrossRef]
26. Chachalis, D., Reddy, K. N., Elmore, C. D., Steele, M. L. (2001). Herbicide efficacy, leaf structure, and spray droplet contact angle among Ipomoea species and smallflower morningglory. Weed Science, 49(5), 628–643. DOI 10.1614/0043-1745(2001)049[0628:HELSAS]2.0.CO;2. [Google Scholar] [CrossRef]
27. Fernández, V., Sancho-Knapik, D., Guzmán, P., Peguero-Pina, J. J., Gil, L. et al. (2014). Wettability, polarity, and water absorption of holm oak leaves: Effect of leaf side and age. Plant Physiology, 166(168), 168–180. DOI 10.1104/pp.114.242040. [Google Scholar] [CrossRef]
28. Wittenberghe, S. V., Adriaenssens, S., Staelens, J., Verheyen, K., Samson, R. (2012). Variability of stomatal conductance, leaf anatomy, and seasonal leaf wettability of young and adult European beech leaves along a vertical canopy gradient. Trees, 26(5), 1427–1438. DOI 10.1007/s00468-012-0714-7. [Google Scholar] [CrossRef]
29. Wang, F., Liang, C. H., Yang, M. T., Zhang, X. S. (2015). Effects of surface characteristics on liquid behaviors on fin surfaces during frosting and defrosting processes. Experimental Thermal and Fluid Science, 61(4), 113–120. DOI 10.1016/j.expthermflusci.2014.10.022. [Google Scholar] [CrossRef]
30. Guo, Z. G., Liu, W. M. (2007). Biomimic from the superhydrophobic plant leaves in nature: Binary structure and unitary structure. Plant Science, 172(6), 1103–1112. DOI 10.1016/j.plantsci.2007.03.005. [Google Scholar] [CrossRef]
31. Liang, Y. H., Peng, J., Li, X. J., Xu, J. K., Zhang, Z. H. et al. (2016). From natural to biomimetic: The superhydrophobicity and the contact time. Microscopy Research and Technique, 172(6), 1103–1112. DOI 10.1002/jemt.22689. [Google Scholar] [CrossRef]
32. Pan, S. J., Guo, R., Xu, W. J. (2014). Investigating and biomimicking the surface wetting behaviors of ginkgo leaf. Soft Matter, 10(44), 8800–8803. DOI 10.1039/C4SM01844C. [Google Scholar] [CrossRef]
33. Barthlott, W., Schimmel, T., Wiersch, S. (2010). The Salvinia paradox: Superhydrophobic surfaces with hydrophilic pins for air retention under water. Advanced Materials, 22(21), 2325–2328. DOI 10.1002/adma.200904411. [Google Scholar] [CrossRef]
34. Joo, H. M., Chang, K. C., Jeffrey, S. A. (2015). A rebounding droplet impacting on a static droplet. Journal of Heat Transfer, 137(8), 80909. DOI 10.1115/1.4030473. [Google Scholar] [CrossRef]
Cite This Article
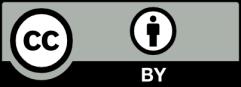