Open Access
ARTICLE
Do Geographically Isolated Grasslands Follow the Principle of Island Biogeography in a Landscape Scale? Taking Poyang Lake Grassland as an Example
1
College of Land Resources and Environment, Jiangxi Agricultural University, Nanchang, 330045, China
2
East China University of Technology, Nanchang, 330032, China
3
The Key Laboratory of Landscape and Environment, Jiangxi Agricultural University, Nanchang, 330045, China
4
School of Tourism and Urban Management, Jiangxi University of Finance and Economics, Nanchang, 330013, China
* Corresponding Author: Wenbo Chen. Email:
(This article belongs to the Special Issue: Plant–Environment Interactions)
Phyton-International Journal of Experimental Botany 2023, 92(2), 455-470. https://doi.org/10.32604/phyton.2022.022466
Received 11 March 2022; Accepted 13 June 2022; Issue published 12 October 2022
Abstract
As one of the basic theories of biodiversity conservation, island biogeography has been widely accepted in the past decades. Originally, island biogeography was put forward and applied in oceanic environments. But later on, it was found out that the application was not only limited to oceanic islands, but also in terrestrial environments with relatively isolated conditions. In terms of biodiversity level, island biogeography generally focuses on a small scale, such as species diversity and genetic diversity. The studies of biodiversity on a large-scale based on island biogeography, such as ecosystem and landscape scales, were seldomly conducted. Taking Poyang Lake, the largest fresh water lake in China as case study area, 30 grasslands were randomly selected to study whether island biogeography can be applied to grasslands at a landscape level from three island attributes (area, distance and shape), and the most important ecological variable (flooding) in Poyang Lake. The results showed that in general, grasslands have the property of an island, and follow the basic principle of island biogeography. We found the area and flooding duration were the two most important determinants of landscape diversity. There was a significant positive correlation between the grassland area and the landscape diversity, which could be well expressed by logarithmic function model (R2 = 0.73). There was a negative correlation between flooding duration and landscape diversity, which could be described by an inverse model (R2 = 0.206). The distance to mainland and the shape of grassland were correlated with landscape diversity, but the fitting result of the models was not as good as expected. The possible reason could be that Poyang Lake is a seasonal lake, the water level varies with hydrological conditions, so that the grasslands are not strongly isolated and their shape is not stable enough required by island biogeography. Furthermore, it indicates that besides area, distance and shape attributes, flooding strongly affects the biodiversity of grassland vegetation, and should not be ignored when applying island biogeography theory to Poyang Lake. This study is expected to be a supplement for island biogeography in terrestrial environments, and the results are expected to benefit for the biodiversity conservation in Poyang Lake.Keywords
Biodiversity is an important factor that affects the functioning of ecosystems [1], and the vegetation monitoring, classification, and restoration, especially protection, which have always been hot spots for many scholars [2,3]. Four levels of biodiversity, i.e., species diversity, genetic diversity, ecosystem diversity and landscape diversity are commonly identified [4]. As one of the basic theories of biodiversity conservation, island biogeography has been widely recognized due to its simplicity [5], and it is essential to promote people’s understanding of the geographical distribution and dynamic pattern of biodiversity.
Although island biogeography was put forward and applied in oceanic environments, it was found out that the application was not only limited to oceanic islands, but also in terrestrial environments with relatively isolated conditions. These later include remnant forest patches after fragmentation [6], ponds [7,8] and lakes [9,10], and even oasis in deserts or urban green roofs [11]. In terms of biodiversity level, island biogeography generally focuses on a small scale, such as species diversity. The classic island biogeography proposes that species richness is determined by the rates of immigration and extinction [12]. Recently, genetic diversity among islands using island biogeography theory was also studied [13]. The results show that there is a significant relationship between biodiversity and island area [14–17], distance to the mainland [18,19], shape of the island [20] and some other ecological variables such as soil type [21] and hydrological change [22]. Both genetic diversity and species diversity belong to small-scale biodiversity. Studies of biodiversity on a large-scale based on island biogeography, such as ecosystem and landscape scales, were seldomly conducted [23].
Poyang Lake, located in the north of Jiangxi Province, is the largest freshwater lake in China, and known for its ecological importance of biodiversity conservation [22]. The main component of the ecosystem of Poyang Lake is grassland. The vegetation of the grasslands in Poyang Lake plays an important ecological role in providing habitat for benthic animals and migratory birds. Therefore, grasslands have become a research hot spot for many scholars. Since Poyang Lake is connected to the Yangtze River, the longest river in China, the water volume is affected by the Yangtze River and five main tributaries. Seasonal variation of the water level, with the typical hydrological characteristics of “like an ocean in flood season while a stream in dry season”, was observed in the Poyang Lake. Several studies show that the seasonal water level fluctuation of Poyang Lake is the main factor influencing vegetation diversity in grasslands [24]. Water level fluctuation can not only affect the vegetation of grasslands directly through mechanical damage [25,26], but also affect vegetation indirectly through the effect of other physical and chemical characteristics, such as illumination and soil physical and chemical properties [27–29]. The mean elevation of grasslands varies from 10 m to 18 m at normal water level. The grasslands with lower elevation are submerged while the ones with higher elevation are isolated by water and present themselves as islands (Fig. 1). Due to different ecological habitats, the vegetation on grasslands gradually forms a relatively stable landscape pattern under the influence of hydrology.
Figure 1: The isolated grasslands of Poyang Lake at normal water level (view in July 2018)
In this study, we put forward a scientific question: at a landscape scale, does island biogeography theory can be applied to the grasslands of Poyang Lake? The past researches on Poyang Lake rarely focused on this question. This study aims to analyze vegetation biodiversity at a landscape scale from the perspectives of three island attributes and the most important ecological variable, i.e., flooding. In order to fulfil this task, thirty isolated grasslands were randomly chosen, and landscape diversity index of each chosen grassland was used as the dependent variable while grassland area, isolation, shape, and flooding duration experienced by the grassland were used as independent variables for statistical analysis and mathematical modelling. The optional model was established to discover in what form are presented the biogeographical island effects. This research can provide new ideas for the sake of biodiversity conservation at a landscape scale in Poyang Lake from the perspective of island biogeography.
Poyang Lake is one of the main tributaries in the middle and lower reaches of the Yangtze River, and plays an important role in regulating the water level, conserving biodiversity, adjusting the local climate and maintaining the ecological balance of the surrounding areas [30]. Due to the lack of topographic rain, the average annual precipitation in most areas varies from 1400 mm to 1600 mm. It there exists obvious flood and dry seasons yearly in Poyang Lake. The flood season ranges from April to September, while the dry season ranges from October to the next year in March [31]. Accordingly, the area of the lake varies from 4,125 km2 or above at high water levels (20 m), 3,150 km2 at medium water levels (14∼15 m), to only 500 km2 at low water levels (12 m) (from https://baike.so.com/doc/4565817-4776776.html). As a typical landscape, grasslands change frequently due to the influence of the hydrological processes [32]. The vegetation of grasslands can be divided into three ecological associations according to semi-aquatic, aquatic and phytoplankton environments. Their main species are reed (Phragmites australis (Cav.) Trin. ex Steud.), sedge (Carex spp.), etc. [31].
The selected grasslands for this study are mainly located in The Nanji Wetland National Nature Reserve (NWNNR), south of Poyang Lake. This is because human disturbances in this area are strictly forbidden by national nature reserve regulations and the wetland landscape is well protected (Fig. 2). It provided an ideal place for this study.
Figure 2: Location of the Poyang Lake
2.2 Image Data and Grasslands Selection
Because the images required for the study should be taken during the normal water level period of Poyang Lake, the selection of data is quite limited. After screening the data available, the Landsat 8 data (water level 14.07 m) imaged on September 14, 2017 and the GF-1 PMS data (water level 13.8 m) imaged on November 08, 2017 were the closest to the normal water level, and thus were used in this study. The landsat-8 remote sensing image having multispectral bands with a spatial resolution of 30 m, and a panchromatic band with a spatial resolution of 15 m on September 14, 2017, was downloaded from the geospatial data cloud (http://www.gscloud.cn). The GF-1 PMS data possessing multispectral bands with a spatial resolution of 8 m, and a panchromatic band with a spatial resolution of 2 m, was obtained from the Natural Resources Department of Jiangxi Province.
The grasslands and water area of the Poyang Lake were identified visually from the Landsat 8 data since they were easily differentiated in the band composition of 432 (Fig. 3a). There were a total of 233 grasslands identified by visual interpretation in the Nanji Wetland National Nature Reserve (Fig. 3b). We numbered them one by one for random selection, and more than 30 samples were selected to satisfy qualitative data required by statistics. The selected grasslands should expose during the normal water level period of Poyang Lake, so that they were located in areas with higher altitudes. Furthermore, since the water level of Poyang Lake is fluctuating, the selected grasslands might be connected together when the water level decreases. By the expertise of hydrology on the Poyang Lake, the grasslands are not easy to join each other if their distance is further than 1 km at normal water level. The grassland whose distance to other selected grasslands was less than 1 km was abandoned for maintaining enough independence, thus ensuring that the randomly selected grasslands will not connect each other due to slight water level changes. Finally, nearly 13% of all grasslands (i.e., 30 samples) were selected for this study (Fig. 3b).
Figure 3: Grasslands’ location and interpretation results (samples 5, 21 as examples). Grasslands No. 1 to 13 are nearest to Kangshan station. Grasslands No. 14 to 30 are nearest to Tangyin station
2.3 Vegetation Classification and Accuracy Verification
After identifying and selecting the grasslands from the Landsat 8 data, the GF-1 PMS data with higher resolution were used for vegetation classification. The maximum-likelihood method has been commonly used in vegetation detection in Poyang Lake [24,31]. Firstly, the preprocessing of GF-1 PMS data such as radiation calibration, atmospheric correction, geometric correction, and ortho-rectification was conducted. Then we fused the panchromatic band with multispectral bands to improve the spatial resolution of GF-1 to 2 m. Samples were taken through field work in July 2018, and vegetation classification was conducted by using the maximum likelihood method. Finally, the results were fine-tuned by visual identification referring to multi-source data such as the Poyang lake vegetation distribution map and previous classification results [31]. The confusion matrix was used to evaluate the classification accuracy. Furthermore, the accuracy evaluation was conducted by the Kappa coefficient [33] to express the accuracy of image classification used to produce a thematic map [11].
There were 6 vegetation types of identified grasslands (i.e., reed (Phragmites australis (Cav.) Trin. ex Steud), sedge (Carex spp.), Bermudagrass (Cynodon dactylon (L.) Pers.), Phalaris arundinacea (Phalaris arundinacea L.), Polygonum criopolitanum (Polygonum criopolitanum Hance) and weeds). The confusion matrix is shown in Table 1. It indicates that the overall accuracy was 88.3%, and the kappa coefficient was 0.86. It also indicates that the classification accuracy was satisfied, and the results obtained from it can be used for further studies (Figs. 3c and 3d).
2.4 Variable Definition and Calculation
The Shannon diversity index (SHDI) was used to express biodiversity on a landscape scale in this study. It reflects the number of different patch types and the proportional area distribution among patch types.
where Pi is the percentage of type i patch area to total area, and m is the total number of patch types in the landscape.
The area (ha) and perimeter (m) of each grassland were determined by map calculation of the interpreted image data. The distance in island biogeography originally refers to the spatial distance between an island and its closest mainland in oceanic environments, correspondingly, the meaning of which should be transferred and broadened in terrestrial environments. As a sign of island isolation on land, distance could be expressed as the distance to the mainland, to other island matrix, or to the stepping stone [34]. Therefore, both the distances from the chosen grasslands to the nearest mainland (G-M) (Fig. 4a), and to the nearest grassland network (G-G) were applied in the study to obtain the most suitable distance indicator. The nearest distance calculation method was applied to determine G-M and G-G, which was carried out by ArcGIS 10.2 software. The results were shown in Fig. 4.
Figure 4: (a) The shortest distance between grassland and the mainland (G-M). (b) The shortest distance between grassland and grassland network (G-G)
The path shape index (PSI) of each grassland was defined as
where P is the patch perimeter and A is the patch area. The larger the PSI is, the more deviated the grassland is, the more complex the shape is.
Previous studies found that the flooding duration is the main factor affecting vegetation distribution [35]. Therefore, the study calculated the flooding duration of each grassland in one year. Firstly, monthly the water level of Poyang Lake from November 08, 2016 to November 08, 2017 was simulated by spline interpolation. The elevation and the simulated water level of each grassland were records, and the relationship between the simulated water level and the nearest station water level was established (Fig. 3). The water level of the nearest station to each grassland was also recorded. Finally, according to the daily water level data of the station, the flooding duration of each grassland in one year was counted.
The area, distance/isolation, shape index and flooding duration were used as explanatory variables for this study. The variable attributes of the 30 grasslands are shown in Table 2.
We took the area, G-M and G-G isolation, and the PSI of the chosen grasslands as explanatory variables, and the SHDI of the chosen grasslands as dependent variables. Ten models such as linear, logarithmic, inverse, quadratic, cubic, compound, power, S-type, growth curve and exponential functions were fitted by regression (Table 3). By comparing the goodness of fitting, the island effects of grassland were verified and the optical model was established.
3.1 Area Attribute and Optimal Model Selection
Ten commonly used equations were applied to model the correlation between SHDI and area. The fitting results are shown in Table 3. It indicates that the area of grassland is significantly correlated with SHDI in any selected model (p < 0.01). Consequently, grassland area had a strong effects on vegetation biodiversity in Poyang Lake as it was supposed to be. Moreover, there were two models (i.e., the logarithmic and cubic equation models) with a R2 of more than 0.7. This indicates that these models have the power to explain more than 70% of variance and have the potential for better prediction. The fitting curves of the two models are shown in Fig. 5 for comparison. It was found that cubic equation had a negative correlation with SHDI when the area of grassland ranged from 35.97 ha to 45.52 ha. It is not a reasonable and practical relationship between area and SHDI. Consequently, the logarithmic function with a R2 of 0.73 was regarded as the optimal model from the perspective of island biogeography on a landscape scale.
Figure 5: Two better fitting model curves. (a) Logarithmic. (b) Cubic
3.2 Distance Attribute and Optimal Model Selection
Selecting different distance indexes is conducive to the study of the distance attribute [36]. The distance from each chosen grassland to the nearest mainland and to nearest grassland network were both applied in this study. Similarly, ten equations were used for modelling as area attribute did. The results are shown in Table 4. It can be concluded that G-M is better than G-G because p values of the selected equations using G-G were obviously larger than those using G-M. All selected equations using G-G as distance were not significant at the p level of 0.05. As for the G-M, only the inverse equation (Fig. 6) can be accepted for modelling at a significant level of 0.05, in which SHDI was negatively correlated with G-M, having the power to explain more than 12% (R2 = 0.12) of variance.
Figure 6: Inverse model curve of SHDI and distance (G-M)
Generally speaking, the distance attribute of grassland landscape in Poyang Lake is relatively weaker than that of area. The distance to mainland rather than to grassland network had a better explanation. Distance means isolation from the perspective of island biogeography. Since Poyang Lake has the typical hydrological characteristics of “an ocean in flood season while a stream in dry season”, grasslands isolation can be frequently changed during the flooding seasons. The possible reason for the weak distance attribute could be that there was no enough isolation among grassland landscapes required by island biogeography in terrestrial environment.
3.3 Shape Attribute and Optimal Model Selection
Ten models were also selected for shape attribute modelling. The fitting results are shown in Table 5. It appears there did not exist a weak shape attribute. According to the p values, no function can model well the relationship between SHDI and the shape of chosen grasslands. Consequently, it cannot be concluded that there exists a reasonable shape attribute of grassland in Poyang Lake. Moreover, Poyang Lake is a seasonal lake, and the shape index of grassland was very sensitive to the water level frequently changed. The instability of the shape and thus the errors of modelling should be taken into consideration in this study.
3.4 Flooding Duration and Optimal Model Selection
The fitting results of SHDI and flooding duration are shown in Table 6. According to the R2 and p values, the inverse functions can well model the relationship between SHDI and flooding duration. According to the model curve (Fig. 7), SHDI was negatively correlated with flooding duration. That’s because, flooding duration can change the availability of soil nutrients and deposition process, which directly or indirectly affects the growth and development of vegetation [36]. It also indicates that besides area and distance, the ecological variable of flooding duration can strongly affect the biodiversity and distribution of vegetation in Poyang Lake, as was found by many other studies [22].
Figure 7: Inverse model curve of SHDI and flooding duration
Island biogeography believes that the number of species on islands is the result of two major processes. One is island size affecting species richness, the other is island isolation directly reducing species diversity. The results of this study showed that the diversity of isolated grasslands in Poyang Lake on landscape scale has a strong positive correlation with the area, and a weak negative correlation with the distance from grasslands to the main land. This shows that the geographically isolated grassland in Poyang Lake generally follows the principle of island biogeography at a landscape scale from the perspective of area and distance.
SHDI of grassland had a strong positive correlation with area, since area is the strongest predictor of island diversity on a landscape scale [5]. For the isolated grasslands in Poyang Lake, larger area can increase niche diversity and population size [37], and it thus can improve ecosystem diversity on a landscape scale. Moreover, it has been found that elevation is a key factor affecting vegetation distribution due to frequent flood disturbances [38,39]. The habitat quality of grasslands of different elevations is significantly different. Therefore, larger areas mean that the grasslands have more diverse habitats related with elevation, creating more environments suitable for ecosystem succession [8,40]. According to the results of model fitting, a logarithmic function was the optimal model to express the SHDI and area relationship. It is consistent with the studies of Dengler [41], in which the power and logarithmic function were regarded as the best-fitting species-area relation models [42].
In island biogeography, longer distance to mainland means more isolation limiting the ability of species to migrate, especially for the vegetation with low diffusion capacity [43–45]. We chose two distance indexes (i.e., G-M and G-G) for a comparative study. It is concluded that G-M was better than G-G, because continental landmasses harbor large species pools for potential island colonization [46]. The results showed that there existed a weak distance attribute, similar to the results of other studies [34]. One reason for this is that study area was of a limited spatial extent [47]. Compared with the islands in the ocean, the distance among grasslands in Poyang Lake was closer, and the distribution extent was limited. The other reason for this is related to the seasonal changes of water levels in Poyang Lake [48,49]. In the dry season, with the decline of water level, the area of grasslands gradually expanded, and connection among grasslands was strengthened. Thus, the isolation among grassland may be not enough required by island biogeography in oceanic environments. Finally, species of oceanic islands are strongly influenced by such ecological processes as prevailing winds and ocean currents [50,51], while vegetation on grasslands in Poyang Lake is mainly affected by flooding and migratory birds disturbance [52,53]. The different disturbances between continental and oceanic environments could be another reason for a weak distance attribute.
Shape attributes are expected to exist for the grasslands of Poyang Lake at a landscape scale. According to landscape ecology, shape affects the ecological flow between landscape elements, which can promote the propagation and growth of organisms [54,55]. The complexity of the patch’s shape affects the area of its core and edge, and thus the population size that can remain in irregularly shaped, fragment areas [56]. But in our study, though SHDI appeared to have no correlation with PSI, the best-fitted models were not consistent with the idea that the diversity will be improved with the increase of shape complexity at a landscape scale. Since the shape index of grassland was very sensitive to the frequent change of water level in Poyang Lake, it is recommended that the instability of shape and thus the errors should be taken into consideration in the process of shape attribute verification.
Besides area, distance and shape attributes, there are many ecological variables strongly affecting the spatial distribution and diversity of grassland vegetation. Flooding is the most important one of them. In this study, the flooding duration was selected as a variable because flooding duration was a comprehensive expression of the elevation of the grassland and the water level change, both of which were regarded as decisive factors for vegetation biodiversity and distribution in Poyang Lake. We found a significantly negative effect of flooding duration on the landscape diversity of the grassland. The sensitivity to the flooding duration determines the growth status and distribution pattern of grassland vegetation [35]. Moreover, the growth period of most vegetation is around in summer, and the flooding season of Poyang Lake usually occurs during summer and autumn. If the flooding duration is too long, it will limit the growth of vegetation, thereby reducing the bio-diversity of grasslands.
This paper aimed to apply the basic idea of island biogeography to the grasslands in Poyang Lake from the perspective of area, distance and shape attributes while taking the most important flooding ecological variable into consideration. It made some conclusions beneficial for the application of island biogeography in terrestrial environments. However, there were some shortcomings in our research. For example, is only one-year-data were applied in this study. We try to project the grassland temporal variation into a spatial sequence by randomly selecting 30 samples. However, it cannot replace the long-time positioning observation. Thus, more investigations on grassland habitats are required to be conducted for our deeper studies in the future.
By using geographic information technology and statistical modelling, this paper attempted to study whether the landscape diversity of isolated grasslands of Poyang Lake follow the basic principles of island biogeography from the aspects of area, distance, and shape attributes and flooding ecological variable. The results showed as follows: 1) It there exists a strong area attribute which can be well expressed by a logarithmic function model with a high R2 of 0.73. 2) The SHDI was negatively correlated with the distance between grassland and its nearest mainland (G-M), but the R2 of the fitting was low. Weak isolation caused by seasonal flooding in Poyang Lake can be the main reasons. 3) The SHDI showed a weak correlation with PSI, but the tendency was not consistent with the idea that biodiversity will be improved with the increase of shape complexity at a landscape scale. 4) There was a significantly negative effect of flooding duration on the SHDI because prolonged flooding limits vegetation growth. It indicates that besides area and distance attributes, the flooding ecological variable strongly affects the biodiversity of grassland vegetation, and should not be ignored when applying island biogeography theory to Poyang Lake.
The study indicated that in general the island biogeography theory can apply to the vegetation biodiversity of isolated grasslands in Poyang Lake at a landscape scale. Due to the specific hydrological process of Poyang Lake, it is recommended that the errors caused by the seasonal water level fluctuation, and the resulting instability of the grasslands, should be considered when island biogeography theory is applied.
Authorship: Shiqi Luo: Conceptualization, Data curation, Investigation, Methodology, Software, Writing–original draft; Wenbo Chen: Conceptualization, Funding acquisition, Investigation, Supervision, Writing–review & editing; Lei He: Investigation, Writing–review & editing; Qiongbing Xiong: Data curation, Investigation, Software.
Funding Statement: This study was supported by the National Natural Science Foundation of China (Nos. 41961036, 41901130).
Conflicts of Interest: The authors declare that they have no conflicts of interest to report regarding the present study.
References
1. Gamfeldt, L., Hillebrand, H., Jonsson, P. R. (2008). Multiple functions increase the importance of biodiversity for overall ecosystem functioning. Ecology, 89(5), 1223–1231. [Google Scholar]
2. Cretois, B., Linnell, J. D. C., Grainger, M., Nilsen, E. B., Rød, J. K. (2020). Hunters as citizen scientists: Contributions to biodiversity monitoring in Europe. Global Ecology and Conservation, 23, e1077. [Google Scholar]
3. Etter, A., Andrade, A., Nelson, C. R., Cortés, J., Saavedra, K. (2020). Assessing restoration priorities for high-risk ecosystems: An application of the IUCN red list of ecosystems. Land Use Policy, 99, 104874. [Google Scholar]
4. West, N. (1993). Biodiversity of rangelands. Rangeland Ecology & Management/Journal of Range Management Archives, 46(1), 2–13. [Google Scholar]
5. MacArthur, R. H., Wilson, E. O. (1963). An equilibrium theory of insular zoogeography. Evolution, 17(4), 373–387. [Google Scholar]
6. Glassman, S. I., Lubetkin, K. C., Chung, J. A., Bruns, T. D. (2017). The theory of island biogeography applies to ectomycorrhizal fungi in subalpine tree “islands” at a fine scale. Ecosphere, 8(2), e1677. [Google Scholar]
7. Uchida, Y., Inoue, M. (2010). Fish species richness in spring-fed ponds: Effects of habitat size versus isolation in temporally variable environments. Freshwater Biology, 55(5), 983–994. [Google Scholar]
8. O’Dwyer, J. P., Green, J. L. (2010). Field theory for biogeography: A spatially explicit model for predicting patterns of biodiversity. Ecology Letters, 13(1), 87–95. [Google Scholar]
9. Wang, Y., Bao, Y., Yu, M., Xu, G., Ding, P. (2010). Nestedness for different reasons: The distributions of birds, lizards and small mammals on islands of an inundated lake. Diversity and Distributions, 16(5), 862–873. [Google Scholar]
10. Si, X., Pimm, S. L., Russell, G. J., Ding, P. (2014). Turnover of breeding bird communities on islands in an inundated lake. Journal of Biogeography, 41(12), 2283–2292. [Google Scholar]
11. Blank, L., Vasl, A., Schindler, B. Y., Kadas, G. J., Blaustein, L. (2017). Horizontal and vertical island biogeography of arthropods on green roofs: A review. Urban Ecosystems, 20(4), 911–917. [Google Scholar]
12. Patiño, J., Guilhaumon, F., Whittaker, R. J., Triantis, K. A., Gradstein, S. R. et al. (2013). Accounting for data heterogeneity in patterns of biodiversity: An application of linear mixed effect models to the oceanic island biogeography of spore-producing plants. Ecography, 36(8), 904–913. [Google Scholar]
13. Costanzi, J. M., Steifetten, O. (2019). Island biogeography theory explains the genetic diversity of a fragmented rock ptarmigan (Lagopus muta) population. Ecology and Evolution, 9(7), 3837–3849. [Google Scholar]
14. Magura, T., Ködöböcz, V., Tóthmérész, B. (2001). Effects of habitat fragmentation on carabids in forest patches. Journal of Biogeography, 28(1), 129–138. [Google Scholar]
15. Wang, S., Zhu, W., Gao, X., Li, X., Yan, S. et al. (2014). Population size and time since island isolation determine genetic diversity loss in insular frog populations. Molecular Ecology, 23(3), 637–648. [Google Scholar]
16. Hill, R., Loxterman, J. L., Aho, K. (2017). Insular biogeography and population genetics of dwarf mistletoe (Arceuthobium americanum) in the central Rocky Mountains. Ecosphere, 8(5), e1810. [Google Scholar]
17. Sato, J. J., Tasaka, Y., Tasaka, R., Gunji, K., Yamamoto, Y. et al. (2017). Effects of isolation by continental islands in the seto inland sea, Japan, on genetic diversity of the large Japanese field mouse, Apodemus speciosus (Rodentia: Muridaeinferred from the mitochondrial dloop region. Zoological Science, 34(2), 112–121. [Google Scholar]
18. Garcia-Verdugo, C., Sajeva, M., La Mantia, T., Harrouni, C., Msanda, F. et al. (2015). Do island plant populations really have lower genetic variation than mainland populations? Effects of selection and distribution range on genetic diversity estimates. Molecular Ecology, 24(4), 726–741. [Google Scholar]
19. Francisco, F. O., Santiago, L. R., Mizusawa, Y. M., Oldroyd, B. P., Arias, M. C. (2016). Genetic structure of island and mainland populations of a neotropical bumble bee species. Journal of Insect Conservation, 20(3), 383–394. [Google Scholar]
20. Cousins, S. A. O., Aggemyr, E. (2008). The influence of field shape, area and surrounding landscape on plant species richness in grazed ex-fields. Biological Conservation, 141(1), 126–135. [Google Scholar]
21. Johnson, M. P., Simberloff, D. S. (1974). Environmental determinants of island species numbers in the British Isles. Journal of Biogeography, 1(3), 149–154. [Google Scholar]
22. Han, X., Chen, X., Feng, L. (2015). Four decades of winter wetland changes in Poyang Lake based on Landsat observations between 1973 and 2013. Remote Sensing of Environment, 156, 426–437. [Google Scholar]
23. Prevedello, J. A., Gotelli, N. J., Metzger, J. P. (2016). A stochastic model for landscape patterns of biodiversity. Ecological Monographs, 86(4), 462–479. [Google Scholar]
24. Dai, X., Wan, R., Yang, G., Wang, X., Xu, L. et al. (2019). Impact of seasonal water-level fluctuations on autumn vegetation in Poyang Lake wetland, China. Frontiers of Earth Science, 13(2), 398–409. [Google Scholar]
25. Zhang, Q., Li, L., Wang, Y. G., Werner, A. D., Xin, P. et al. (2012). Has the three-Gorges Dam made the Poyang Lake wetlands wetter and drier? Geophysical Research Letters, 39(20). [Google Scholar]
26. Mei, X., Dai, Z., Du, J., Chen, J. (2015). Linkage between three gorges Dam impacts and the dramatic recessions in China’s largest freshwater lake, Poyang Lake. Scientific Reports, 5(1), 18197. [Google Scholar]
27. Dronova, I., Gong, P., Wang, L. (2011). Object-based analysis and change detection of major wetland cover types and their classification uncertainty during the low water period at Poyang Lake, China. Remote Sensing of Environment, 115(12), 3220–3236. [Google Scholar]
28. Chen, L., Jin, Z., Michishita, R., Cai, J., Yue, T. (2014). Dynamic monitoring of wetland cover changes using time-series remote sensing imagery. Ecological Informatics, 24, 17–26. [Google Scholar]
29. You, H., Xu, L., Liu, G., Wang, X., Wu, Y. et al. (2015). Effects of inter-annual water level fluctuations on vegetation evolution in typical wetlands of Poyang Lake, China. Wetlands, 35(5), 931–943. [Google Scholar]
30. Chen, B., Chen, L., Huang, B., Michishita, R., Xu, B. (2018). Dynamic monitoring of the Poyang Lake wetland by integrating landsat and MODIS observations. ISPRS Journal of Photogrammetry and Remote Sensing, 139, 75–87. [Google Scholar]
31. Wan, R., Dai, X., Shankman, D. (2019). Vegetation response to hydrological changes in Poyang Lake, China. Wetlands, 39(S1), 99–112. [Google Scholar]
32. Lan, Z., Chen, Y., Li, L., Li, F., Jin, B. et al. (2019). Testing mechanisms underlying elevational patterns of lakeshore plant community assembly in Poyang Lake, China. Journal of Plant Ecology, 12(3), 438–447. [Google Scholar]
33. Foody, G. M. (2020). Explaining the unsuitability of the kappa coefficient in the assessment and comparison of the accuracy of thematic maps obtained by image classification. Remote Sensing of Environment, 239, 111630. [Google Scholar]
34. Weigelt, P., Kreft, H. (2013). Quantifying island isolation-insights from global patterns of insular plant species richness. Ecography, 36(4), 417–429. [Google Scholar]
35. Tan, Z., Jiang, J. (2016). Spatial-temporal dynamics of wetland vegetation related to water level fluctuations in Poyang Lake, China. Water, 8(9), 397. [Google Scholar]
36. Turchi, G. M., Kennedy, P. L., Urban, D., Hein, D. (1995). Bird species richness in relation to isolation of aspen habitats. The Wilson Bulletin, 107(3), 463–474. [Google Scholar]
37. Peay, K. G., Bruns, T. D., Kennedy, P. G., Bergemann, S. E., Garbelotto, M. (2007). A strong species-area relationship for eukaryotic soil microbes: Island size matters for ectomycorrhizal fungi. Ecology Letters, 10(6), 470–480. [Google Scholar]
38. Mata-González, R., Pieper, R., Cardenas, M. M. (2002). Vegetation patterns as affected by aspect and elevation in small desert mountains. The Southwestern Naturalist, 47(3), 440–448. [Google Scholar]
39. Campos-Cerqueira, M., Arendt, W. J., Wunderle, J. M., Aide, T. M. (2017). Have bird distributions shifted along an elevational gradient on a tropical mountain? Ecology and Evolution, 7(23), 9914–9924. [Google Scholar]
40. He, F., Legendre, P. (2002). Species diversity patterns derived from species-area models. Ecology, 83(5), 1185–1198. [Google Scholar]
41. Dengler, J. (2009). Which function describes the species-area relationship best? A review and empirical evaluation. Journal of Biogeography, 36(4), 728–744. [Google Scholar]
42. Matthews, T. J., Guilhaumon, F., Triantis, K. A., Borregaard, M. K., Whittaker, R. J. (2016). On the form of species-area relationships in habitat islands and true islands. Global Ecology and Biogeography, 25(7), 847–858. [Google Scholar]
43. Van Ruremonde, R. H. A. C., Kalkhoven, J. T. R. (1991). Effects of woodlot isolation on the dispersion of plants with fleshy fruits. Journal of Vegetation Science, 2(3), 377–384. [Google Scholar]
44. Warren, B. H., Simberloff, D., Ricklefs, R. E., Aguilee, R., Condamine, F. L. et al. (2015). Islands as model systems in ecology and evolution: Prospects fifty years after MacArthur-wilson. Ecology Letters, 18(2), 200–217. [Google Scholar]
45. Portillo, J. T. D. M., Ouchi Melo, L. S., Crivellari, L. B., Oliveira, T. A. L., Sawaya, R. J. et al. (2019). Area and distance from mainland affect in different ways richness and phylogenetic diversity of snakes in atlantic forest coastal islands. Ecology and Evolution, 9(7), 3909–3917. [Google Scholar]
46. Chown, S., Gremmen, N., Gaston, K. (1998). Ecological biogeography of southern ocean islands-species-area relationships, human impacts, and conservation. American Naturalist, 152(4), 562–575. [Google Scholar]
47. Whittaker, R., Willis, K., Field, R. (2001). Scale and species richness: Towards a general, hierarchical theory of species diversity. Journal of Biogeography, 28(4), 453–470. [Google Scholar]
48. Deng, P. X., Bing, J. P., Jia, J. W., Liu, X. (2019). Impact of the three gorges reservoir operation on the hydrological situation of Poyang Lake. IOP Conference Series: Earth and Environmental Science, 344, 12088. [Google Scholar]
49. Ning, L., Zhou, Y., Cheng, C., Ye, S., Shen, S. (2019). Using a complex network to analyze the effects of the three gorges Dam on water level fluctuation in Poyang Lake. Isprs International Journal of Geo-Information, 8(11), 470. [Google Scholar]
50. Munoz, J., Felicisimo, A. M., Cabezas, F., Burgaz, A. R., Martinez, I. (2004). Wind as a long-distance dispersal vehicle in the southern hemisphere. Science, 304(5674), 1144–1147. [Google Scholar]
51. Cook, L. G., Crisp, M. D. (2005). Directional asymmetry of long-distance dispersal and colonization could mislead reconstructions of biogeography. Journal of Biogeography, 32(5), 741–754. [Google Scholar]
52. Prather, C. M., Huynh, A., Pennings, S. C. (2017). Woody structure facilitates invasion of woody plants by providing perches for birds. Ecology and Evolution, 7(19), 8032–8039. [Google Scholar]
53. Parnikoza, I., Rozhok, A., Convey, P., Veselski, M., Esefeld, J. et al. (2018). Spread of antarctic vegetation by the kelp gull: Comparison of two maritime antarctic regions. Polar Biology, 41(6), 1143–1155. [Google Scholar]
54. Hill, J. L., Curran, P. J. (2003). Area, shape and isolation of tropical forest fragments: Effects on tree species diversity and implications for conservation. Journal of Biogeography, 30(9), 1391–1403. [Google Scholar]
55. Ewers, R. M., Didham, R. K. (2007). The effect of fragment shape and species’ sensitivity to habitat edges on animal population size. Conservation Biology, 21(4), 926–936. [Google Scholar]
56. Sisk, T. D., Haddad, N. M., Ehrlich, P. R. (1997). Bird assemblages in patchy woodlands: Modeling the effects of edge and matrix habitats. Ecological Applications, 7(4), 1170–1180. [Google Scholar]
Cite This Article
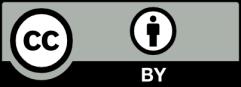
This work is licensed under a Creative Commons Attribution 4.0 International License , which permits unrestricted use, distribution, and reproduction in any medium, provided the original work is properly cited.