Open Access
ARTICLE
Effect of Water Stress Induced by Polyethylene Glycol on Growth, Proline Accumulation in Agave americana L.
1 Laboratorio de Biotecnología vegetal, Instituto Tecnológico de Tuxtla Gutiérrez, Tuxtla Gutiérrez, 29050, México
2 Centro de Investigación Científica de Yucatán, Unidad de Bioquímica y Biología Molecular de Plantas, Mérida, 97205, México
3 Insituto Nacional de Investigaciones Forestales, Agrícolas y Pecuarias, Huimanguillo, Tabasco, 86400, México
4 CONACYT-Tecnológico Nacional de México/Instituto Tecnológico de Tuxtla Gutiérrez, Tuxtla Gutiérrez, 29050, México
* Corresponding Authors: Ruiz-Lau Nancy. Email: ,
# These authors contributed equally to this work
(This article belongs to the Special Issue: Plant–Environment Interactions)
Phyton-International Journal of Experimental Botany 2023, 92(2), 629-643. https://doi.org/10.32604/phyton.2022.023970
Received 19 May 2022; Accepted 01 August 2022; Issue published 12 October 2022
Abstract
The effect of water deficit was determined on both in vitro and soil seedling as well as in cells in suspension of Agave americana L. In order to do the establishment of cells, the formation of callus was induced; for it two auxins were evaluated: 2,4-dichlorophenoxyacetic acid (2,4-D) and 4-mino-3,5,6-trichloropicolinic acid (picloram) at three concentrations (0.25, 0.5 and 0.75 mg L−1) in three explants (leaf, root and meristems) cultured in MS semisolid medium. The callogenesis response was related to the type and section of the explant, as well as the regulator used, and a cell suspension was established using 0.5 mg L−1 naphthaleneacetic acid (NAA) + 0.5 mg L−1 Benzylaminopurine (BAP). Seedlings were exposed to polyethyleneglycol (15% and 30% w/v) with a water potential of −0.87 and −2.67 MPa, respectively, under soil conditions. Water stress was applied through restricted irrigation. Fresh weight, root system growth, and chlorophyll concentration were some of the parameters that were affected by the effect of water deficit on A. americana L. Chlorophyll concentration values were significantly decreased by 15 at 30% PEG (19.6 SPAD units) compared to the control treatment. In in vitro plants, the highest concentration of proline was found in the roots, being the treatment with 30% polyethylene glycol where the highest concentration of this osmoregulator was obtained (62.5 mg g−1 DW). Under restricted irrigation conditions, an increase in proline concentration was observed both in the aerial part (2.2 µg 100 g−1 DW) and in the root system (1.8 µg 100 g−1 DW). However, the concentrations found were approximately ten times greater, less than those found under in vitro conditions. Therefore, the accumulation of proline can be considered an indicator of stress in Agave Americana L. growth in vitro.Keywords
“Stress” in plants can be defined as any external factor that negatively influences the growth, productivity, reproductive capacity, or survival of an organism. Those factors are divided into two categories: the biotic ones, caused by different living organisms such as fungi, bacteria, viruses, and insects, and, the abiotic ones which are caused by environmental factors such as drought, waterlogging, high and low temperatures and salinity, among others [1]. Between the abiotic factors, drought is one of the most important because it negatively affects the growth and development of plants, manifesting its effects from a cell to the entire organism [2]. The most common symptoms that plants present to water deficit or drought stress can be rolled and chlorotic leaves, loss of cell turgor, senescence and, to a lesser extent, necrosis, growth retardation, and plant thinning [3,4].
Exist certain plants that can survive in conditions of severe water stress. The best known among them, are the crassulacean acid metabolism plants (CAM), which are characterized by opening their stomata during night, when temperatures are low and relative humidity is higher, reducing evapotranspiration and increasing water use efficiency [5]. Although CAM-type plant species have great potential in the production of food, fiber, fuel, and fructan polymers (inulin), the area dedicated to their cultivation is still insignificant (0.002%) [6,7]. However, there is little interest for studying this type of plant. Within the CAM plants are those of the Agave genus, which are endemic to America since they are distributed from Florida, United States, to the north of South America, including the Caribbean islands. This genus contains approximately 210 species, of which 159 (75%) are present in Mexico, where 129 are endemic [8,9].
One of the main tools for the study of tolerance to abiotic stress in plants is through cell suspension culture since its studies mean a direct uniform population of cells (mainly in liquid form) instead of a multicellular tissue that has a higher degree of organization, which differs according to the location of the tissue or organ [10]. Some studies in Agave under in vitro or soil conditions have shown that in the presence of water stress induced by PEG, an increase in root length occurs [11] and the suspension of prolonged irrigation does not affect the dry weight of the root and the aerial part [12]. In Agave potatorum Zucc plants, cultivated in perlite substrate and fertigated with Hoagland and Aron nutrient solution after five months of suspension of irrigation accumulated more fructans in the head [13]. However, it is not surprising that physiological research is mainly focused on water stress in model plants with the C3 and C4 metabolism types. The objective of this study was to evaluate the effect of water deficit on A. americana L. on growth and proline accumulation during in vitro culture using osmolytes as stress inducer (polyethylene glycol) and grown in soil employing restricted irrigation.
Agave americana L. shoots was cultivated under aseptic conditions in MS medium (in vitro plantlets) for eight months [14]. For their rooting, they were reseeded in MS medium supplemented with 1 mg L−1 of indole butyric acid (IBA).
Two synthetic auxins were evaluated: 2,4-Dichlorophenoxyacetic acid (2,4-D) and 4-mino-3,5,6-Trichloropicolinic acid (picloram) at different concentrations (0.25, 0.5, and 0.75 mg L−1) in three different explants [leaf, root, and apical meristems (undifferentiated cells responsible for the apical growth located in the center of the stem) in MS semisolid medium]. For the leaf and the meristem three different sections of the explant (base, middle and apical) were used with the thin cell layer (TCL) technique proposed by Monja-Mio et al. [15]. The response variables were the percentage of callus formation, type, and coloration.
2.3 Induction of Cell Suspension of Agave americana L.
For callus growth in a liquid medium, two strategies were used. The first strategy consisted of growing the selected callus in the same callus induction treatment, and the second strategy consisted of using combinations of hormones: the first treatment was using the combination of 2,4-D + 6-Benzylaminopurine (BAP) (0.5 + 9 mg L−1) [16], and the second treatment was using the combination of naphthaleneacetic acid (NAA) + BAP (0.5 + 0.5 mg L−1) [17]. Cell viability was determined using the Trypan blue technique [18].
2.4 Water Stress in Cell Suspensions of Agave americana L.
To determine the effect of water stress on the cell suspension, three treatments with four replicates each were used: polyethylene glycol 8000 (PEG) (0%, 15%, 30% w/v) in MS liquid medium added with NAA + BAP (0.5 + 0.5 mg L−1). The experimental unit consisted of a 500 mL flask with a volume of 50 mL of liquid medium + 1 g of fresh weight (FW) of the cell suspension, which was kept for 30 days.
2.5 Water Stress Induced with PEG in Vitro Seedlings of Agave americana L.
Eight-month-old seedlings cultivated in vitro were used, which were transplanted into a semi-solid MS medium with different concentrations of PEG (15% and 30% w/v) with 10 repetitions each so that the experimental unit consisted of a flask with a seedling. The seedlings were exposed to stress for 60 days under controlled conditions of 18 h light/6 h darkness and at 25°C. The water potential in the medium was measured using a Dewpoint PotentiaMeter model WP4.
2.6 Water Stress by Suspension of Irrigation in Seedlings Agave americana L.
Plants from in vitro culture were planted in 307 mL expanded polystyrene cups with a mixture of agrolite:peat moss (1:3) at 25°C and with a photoperiod of 16 h light/8 h darkness and one irrigation every third day for 90 days. The water deficit consisted of stress through irrigation once a week and as a control one every third day. The volume of water used was 30 mL, calculated with the field capacity of the substrate.
For the chlorophyll content, the Minolta Spad 502 plus meter was used. The method of Arnon [19] was used to determine the chlorophyll content (a, b, and total). The concentration of chlorophyll a, b and total was determined using the following formulas proposed by Inskeep and Bloom [20].
where: (A664) and (A647) represent the absorbance values read at 664 and 647 nm wavelength wave, respectively. Chl a: chlorophyll a; Chl b: chlorophyll b; Chl total: total chlorophyll.
2.8 Determination of Proline Content
To determine the proline content, the protocol described by Bates et al. [21] was used and its concentration was determined from a standard curve as follows:
A 3 × 2 × 3 factorial design (three types of explants, two hormones, and three hormone concentrations) was performed with a comparison of Tukey means (P-value ≤ 0.05) for callus induction. To see the effect of water deficit during in vitro conditions, a completely randomized design was carried out, four repetitions were used for cells in suspension and 10 repetitions for seedlings. For growing in soil ex vitro conditions, 12 replicates were used using an Analysis of Variance (ANOVA) and a Tukey mean comparison test (P-value ≤ 0.05). In all cases, the Statgraphics Centurión XV program was used.
All three types of explants presented different responses to the concentrations of the regulators evaluated (Fig. 1). In leaf explants, no morphogenic response was observed (Fig. 1A). Regarding the meristem (undifferentiated cells responsible for the apical growth of plants), all treatments presented callus formation (Figs. 1B and 1C).
Figure 1: Response of Agave americana L. explants to various auxin concentrations in the culture medium. (A) Leaf oxidation. (B) Callus in meristem culture in MS + 2,4-D (0.75 mg L−1), and (C) Callus in meristem culture in MS + picloram (0.5 mg L−1) at 60 days of induction
During the evaluation of the influence of the sections of the meristem as an explant, the results showed that when using the base of the meristem, the best treatment was with 2,4-D at 0.5 and 0.75 mg L−1. For the middle part of the meristem, picloram showed better results, presenting callus formation percentages of 70% and 50% at 0.5 and 0.75 mg L−1, respectively (Table 1). According to the Pareto diagram (Fig. 2), it was determined that the factors that exert a significant effect (P < 0.05) were the explant section (Factor C) and the combination of auxin-explant section (Factors AC) for callus production from the meristem.
Figure 2: Pareto chart of the standardized effects of different types of auxin, the auxin concentration, and the section of the explant on callus formation. P-value ≤ 0.05 represents statistically significant
Table 2 reports the characteristics of the callus obtained from the sections of the meristem evaluated. Friable callus was found in all treatments, which made them suitable for the culture of cells in suspension since they maintained a constant growth. In addition, they showed a greater capacity to disintegrate.
3.2 Induction of Cell Suspension of Agave americana L.
For the induction of the cell suspension, only the callus originating from the meristem was used (Fig. 3A). As mentioned above, the first strategy used was to grow the callus in the same concentrations as those of the induction, which were, the concentrations of 0.5 and 0.75 mg L−1 for both auxins (2,4-D and picloram). At these concentrations somatic embryos were presented after four weeks in the liquid medium, while at the concentration of 0.25 mg L−1 there was no embryogenic response or biomass increase. The second strategy used for induction, the treatment with the combination of 2,4-D + BAP (0.5 + 9 mg L−1) did not present biomass growth; however, the combination of 2,4-D + BAP (0.5 + 0.5 mg L−1) (Fig. 3B) showed viable cells (Fig. 3D) coupled with biomass generation without cell regeneration even after six weeks of subcultures, although callus darkening was also present (Fig. 3C).
Figure 3: Cell suspension cultures from meristematic callus in liquid MS medium with 0.5 mg L−1 2,4-D + 0.5 mg L−1 BAP. (A) Callus induction from meristematic callus (0.75 mg L−1 2,4-D). (B) Establishment of cell suspension in a liquid medium. (C) Oxidized callus in liquid medium after 15 d. (D) Cell not stained by trypan blue dye after six weeks
3.3 Effect of Polyethylene Glycol (PEG) on Cells Suspension of Agave americana L.
Polyethylene glycol had an effect on the fresh weight of Agave cells at both concentrations evaluated (15% and 30%), observing a significant decrease of 35% and 79%, respectively, compared to the control (Table 3).
3.4 Effect of Polyethylene Glycol (PEG) on in Vitro Seedlings of Agave americana L.
The water potential values showed that the treatments were found in a range of light (−0.5 to −0.8 MPa) to severe stress (>−1.5 MPa) (Table 4).
Different responses were observed in A. americana L. seedlings that were exposed to PEG treatments (Fig. 4). A significant reduction of 62% and 75% was observed in the Fresh Weight (FW) of the aerial part and the Dry Weight (DW) of the root system respect to the control. In this last parameter, a reduction was observed at the formation of roots in the treated seedlings with different concentrations of PEG evaluated with respect to the control treatment (Table 5). The number of roots formed was significantly reduced up to 77% in the presence of PEG. However, no significant effect was observed on the root length as well as the DW of the aerial part and FW of the root system as the PEG concentration increased.
Figure 4: Effect of Polyethylene glycol (PEG) on Agave americana L. seedlings after 60 d. (A) Control; (B) 15% PEG; (C) 30% PEG. Bar = 1 cm
3.5 Water Deficit in Soil Grown Plants of Agave americana L.
Agave americana L. plants under water deficit conditions (Fig. 5) showed a decrease in FW, and DW (40% and 30%, respectively) in the aerial part in comparison to the control (Table 6). This same response was presented in the root system, observing a decrease in FW, DW and length (75%, 60%, and 20%, respectively) when plants were exposed to water stress.
Figure 5: Effect of irrigation on Agave americana L. after 60 d. (A) Constant irrigation (B) Restricted irrigation. Bar = 1 cm
Chlorophyll content values were significantly decreased by 15% at 30% PEG (19.6 SPAD units) compared to the control treatment (29.3 SPAD units) (Table 7). When grown under soil conditions, plants did not present a significant difference (P ≤ 0.05) between treatments (Table 8). However, the concentrations of chlorophyll a, b, and total showed an increase of 45%, 60%, and 50%, respectively, compared to control when soil-grown plant grew under water deficit conditions.
3.7 Proline Accumulation in Agave americana L. Seedlings Exposed to Water Stress
In vitro seedlings showed a significant increase in proline accumulation in both the aerial (60%) and root parts when exposed to 30% PEG (Figs. 6A and 6B) compared to the control treatment. In the in vitro grown plants, the proline accumulation was much higher in the roots than in the aerial parts (Figs. 6A and 6B). Similarly, in plants grown in soil, the proline accumulation increased 50% in leaves (Fig. 6C) and 100% in roots (Fig. 6D) under restricted irrigation conditions.
Figure 6: Proline concentration. Plants grown in vitro with PEG (A) Leaf and (B) Root; plants grown in soil (C) Leaf and (D) Root. n = 5. Values with different letters are significantly different (P-value ≤ 0.05)
It was only possible to induce calluses from meristem explants with both 2,4-D and Picloram, this response could not be obtained in leaf explants, perhaps due to the oxidation, phenolization and necrosis that occurred, which suggests the presence of phenols in the leaves of A. americana L. [22,23]. Phenols are produced by reactive oxygen species (ROS), during the explants cutting stress. These molecules damage lipids, proteins, enzymes and DNA [24] causing the cell to be unable to react with phytohormones, reducing the induction and callus formation. Regarding embryogenesis in the root, the only work with Agave where root tissues were used for a morphogenic process is that of Portillo et al. [25]. They found a low formation of embryos (7.25) using 3 mg L−1 of 2,4-D in Agave tequilana. Therefore, this type of explant can be considered feasible for the use of embryogenesis in this genus.
The meristem are undifferentiated cells responsible for the apical growth of plants. Due to their cell division capacity, they are considered a good source of explants. Our results are in the range reported in other works of A. Americana, regarding the percentage of callus where the meristem was used [20,26], finding percentages ranging from 80% to 100%. However, the callus obtained in those works was of the organogenic type, which is not desirable to generate a cell suspension.
There are few reports on the response of the meristem section and its effect on callus generation. One of them is that of Monja-Mio et al. [15] who evaluated different sections of stems of Agave fourcroydes using the thin cell sheet technique. They found the best results in the apical part, due to the amount of meristematic tissue present in it. However, the studies reported are based specifically on the study of the meristematic zone of the apical part of the meristem in Arabidopsis thaliana. This is because these cells give rise to the generation of organs and cell division processes.
Alternatively, it has been observed that auxins affect the dedifferentiation and redifferentiation of tissues in different ways, depending on the studied Agave species. Cancino-García et al. [27] evaluated the effect of two auxins (indole acetic acid “IAA” and 2,4-D) on the growth of three different species of Agave (A. angustifolia, A. fourcroydes and A. tequilana). They found that in the absence of auxins A. angustifolia developed roots while in the presence of 0.5 µM IAA and 2,4-D, these plants developed shoots and calluses, respectively. The other two species only generated roots in the absence of auxin. In the same study, where 32 ARF (Auxin Response Factor) genes related to the growth of lateral roots, embryogenesis, leaf expansion, and fruit development, the results showed different expression patterns in the three Agave species in the presence and absence of auxins. Consequently, it was concluded that A. angustifolia has a different mechanism for the callus initiation process compared to A. fourcroydes and A. tequilana. The coloration of the callus is what allows to elucidate whether it is an organogenic or non-organogenic callus. Studies have been carried out in this regard in cocoa [28], coffee [29,30], rice [31] and banana [32]. In the case of Agave, no literature indicates the type of response that can be generated concerning the coloration of the calluses in the species.
Cell suspension cultures have been developed in species such as A. tequila [33] and A. amaniensis [34]. The results in this study would be the first for A. americana L. The use of cells in suspension to analyze abiotic stress responses is based on the fact that in vitro cultured cells behave similarly to intact plant cells subjected to water deficit conditions [35]. The addition of PEG to the medium produces osmotic stress [36] and decreases the water potential [37–39] which negatively affects growth. Studies have reported a lower growth rate in the presence of PEG in date palm [40], cane [41], tomato [42], wheat [43], and soybean [44].
In our study, the use of PEG in A. americana L. shoot cultivated under aseptic conditions (in vitro plants) showed a similar response to that of root length. Anyhow, there was a tendency to decreased root growth as reported by Puente-Garza et al. [11]. These authors reported that in vitro seedlings of Agave salmiana subjected to stress with PEG for 60 days showed a significant decrease in root length compared to control plants (2.63 cm) concerning treatments with PEG at 10%, 20% and 30% (0.6, 0.3 and 0.1 cm respectively). In A. americana plants the number of roots formed was significantly reduced in the presence of PEG at 15% and 30%, but the length was not affected. The presence of PEG in a liquid medium could cause a flooding stress, which could explain why the plants did not generate the same number of roots as the control did.
The decrease in biomass has been seen in other CAM-type plants such as Aloe vera, Silva et al. [45] mentioned that, in this type of plant, the most important amount of biomass is presented mainly in the leaves. The loss of biomass may be related rather to dehydration of the seedlings caused by the high concentrations of PEG in the medium. This causes an increase in the water potential (more negative) in the culture medium compared to the internal potential of the plant so there is an outflow of water from the plant to the medium to maintain an osmotic adjustment. Therefore, the biomass production of the aerial part depends on the availability of water in the environment [46]. This is because A. americana is not so dependent on stomatal closure as a measure of tolerance to water deficit, since it can use other mechanisms such as: the accumulation of osmolytes such as proline and fructans, that provide membrane stability and osmotic adjustment. In the presence of PEG, no significant differences were found in the different photosynthetic pigments using the acetone method. Otherwise with the chlorophyllometer, the highest concentrations of PEG were found to have the lowest values of chlorophyll. The decrease of pigments only present at the highest concentrations could be whereas at these concentrations the Agave americana L. plants may be under water stress. This could induce the production and accumulation of ROS, which induce lipid peroxidation in membranes and that ultimately, leads to chlorophyll degradation and loss of photosynthetic activity [47,48].
The response to the decrease in biomass in A. americana L. plants under conditions of water deficit due to decreased irrigation was similar to that found in vitro using PEG. Langlé-Argüello et al. [13] demonstrated a decrease in the number, thickness, and FW of the leaves in Agave potatorum Zucc. after 5 months of drought. On the other hand, Bergsten et al. [49] found a decrease in root FW, height and total FW in Agave weberi plants with water deficit after 80 days. Ramirez-Tobias et al. [12] showed that the DW of the root and leaf of A. americana L. is not affected under conditions of water deficit (−3.5 MPa) even after 14 months under greenhouse conditions in plants obtained from seeds. This evidence that the response to water deficit in the soil through root growth to increase water absorption seems unnecessary; conserving the water stored in its leaves and stems are a more efficient response. However, in Agave species such as A. angustifolia subsp. tequilana, A. asperrima, A. lechuguilla, and A. striata increased root biomass (−3.5 MPa) demonstrated the heterogeneity between Agave species.
Furthermore, it was observed that after 60 days A. americana plants with water deficit did not decrease the concentration of photosynthetic activity. Ramírez-Tobias et al. [50] evaluated two species of Agave (A. striata and A. salmiana) under water deficit, finding a reduction of chlorophyll-a compared to the control of one third and two thirds for A. striata and A. Salmiana, respectively, this demonstrates the heterogeneity of the species studied.
Altogether, few works are based on the effect of water stress in Agave. Peña-Valdivia et al. [51] found a significant increase in proline accumulation from 1.6 to 2.1 mmol mg−1 DW in the roots of Agave salmiana when they were under stress conditions (−2.5 MPa). Langlé-Argüello et al. [13] also studied the effect of water deficit and the physiological and morphological characteristics, and the generation of proline in roots. They evaluated the effect of restricted irrigation (−3.5 MPa) in eight species of Agave, having an average of 0.52 μmol 100 mg−1 of DW under frequent irrigation (−0.7 MPa). The only exception was on A. americana where there was 0.31 μmol 100 mg−1, under conditions of humidity restriction (−3.5 MPa) the proline content increased to an average of 1 μmol 100 mg−1. Riaz et al. [52] evaluated the morphological characteristics and proline accumulation in Agave sisalana leaves (from 1.23 to 4.26 μmol proline g−1 FW) when the plants were under restricted irrigation (2% irrigation). Delatorre-Herrera et al. [53] evaluated the effect of restricted irrigation on Aloe vera, finding a significant increase in proline accumulation in plants at 25% irrigation (0.8 mg g−1 DW) compared to the control (0.4 mg g−1 DW).
Water deficit both in vitro and grown in soil decreased the growth of the aerial part, as well as the root system in A. americana L. plants. Proline was an indicator of stress in both conditions.
The highest concentration of this osmolyte was found in the root system, mainly on in vitro plants. Therefore, the increase in proline constitutes a protective response in Agave americana L. favoring cellular homeostasis under stress conditions, although this accumulation will depend on the species, the physiological age of the plant and the time it was subjected to stress.
Authorship: The authors confirm contribution to the paper as follows: study conception and design: R-L N.; data collection: A-C AE., Y L-G C.; analysis and interpretation of results and draft manuscript preparation: A-C AE., R-L N., Y L-G C.; analysis and technical support for proline extraction: M-E M. and M-L MF.; supervision: L-G C., R-L N., G-M FA., S-G MC. All authors reviewed the results and approved the final version of the manuscript.
Acknowledgement: To CONACYT for the Master’s scholarship of Arnoldo Enrique Alfaro Corres (Scholarship No. 621617).
Funding Statement: The authors received no specific funding for this study.
Conflicts of Interest: The authors declare that they have no conflicts of interest to report regarding the present study.
References
1. Ahmad, M., Ali, Q., Hafeez, M. M., Malik, A. (2021). Improvement for biotic and abiotic stress tolerance in crop plants. Biological and Clinical Sciences Research Journal, 2021, e004. DOI 10.47264/bcsrj0201004. [Google Scholar] [CrossRef]
2. Đurić, M., Subotić, A., Prokić, L., Trifunović-Momčilov, M., Cingel, A. et al. (2020). Morpho-physiological and molecular evaluation of drought and recovery in Impatiens walleriana grown ex vitro. Plants, 9(11), 1559. DOI 10.3390/plants9111559. [Google Scholar] [CrossRef]
3. Toscano, S., Ferrante, A., Romano, D. (2019). Response of Mediterranean ornamental plants to drought stress. Horticulturae, 5(1), 6. DOI 10.3390/horticulturae5010006. [Google Scholar] [CrossRef]
4. Corso, D., Delzon, S., Lamarque, L. J., Cochard, H., Torres-Ruiz, J. M. et al. (2020). Neither xylem collapse, cavitation, or changing leaf conductance drive stomatal closure in wheat. Plant Cell and Enviromrnt, 43(4), 854–865. DOI 10.1111/pce.13722. [Google Scholar] [CrossRef]
5. Heyduk, K., Ray, J. N., Leebens-Mack, J. (2021). Leaf anatomy is not correlated to CAM function in a C3+CAM hybrid species, Yucca gloriosa. Annals of Botany, 127(4), 437–449. DOI 10.1093/aob/mcaa036. [Google Scholar] [CrossRef]
6. USDA NASS (2018). Census of agriculture statistics. Washington DC, USA: United States Department of Agriculture National Agricultural Statistics Service. https://www.nass.usda.gov/. [Google Scholar]
7. Davis, S. C., Simpson, J., Gil-Vega, K. C., Niechayev, N. A., van Tongerlo, E. et al. (2019). Undervalued potential of crassulacean acid metabolism (CAM) for current and future agricultural production. Journal of Experimental Botany, 70(22), 6521–6537. DOI 10.1093/jxb/erz223. [Google Scholar] [CrossRef]
8. García-Mendoza, A. (2002). Distribution of Agave (Agavaceae) in Mexico. Cactus and Succulent Journal, 74(4), 177–187. [Google Scholar]
9. García-Mendoza, A. J., Franco-Martínez, I., Sandoval-Gutiérrez, D. (2019). Cuatro especies nuevas de Agave (Asparagaceae, Agavoideae) del sur de México. Acta Botánica Mexicana, (126), e1461. DOI 10.21829/abm126.2019.1461. [Google Scholar] [CrossRef]
10. Alqurashi, M., Chiapello, M., Bianchet, C., Paolocci, F., Lilley, K. S. et al. (2018). Early responses to severe drought stress in the Arabidopsis thaliana cell suspension culture proteome. Proteomes, 6(4), 38. DOI 10.3390/proteomes6040038. [Google Scholar] [CrossRef]
11. Puente-Garza, C. A., Meza-Miranda, C., Ochoa-Martínez, D., García-Lara, S. (2017). Effect of in vitro drought stress on phenolic acids, flavonols, saponins, and antioxidant activity in Agave salmiana. PPB, 115(5), 400–407. DOI 10.1016/j.plaphy.2017.04.012. [Google Scholar] [CrossRef]
12. Ramírez-Tobías, H. M., Peña-Valdivia, C. B., Aguirre, J. R. (2014). Biochemical-physiological response of Agave species to moisture restriction. Botanical Sciences, 92(1), 131–139. DOI 10.17129/botsci.156. [Google Scholar] [CrossRef]
13. Langle-Arguello, L. A., Martínez-Gutierrez, G. A., Santiago-García, P. A., Escamirosa-Tinoco, C., Morales, I. (2019). Nutrient solutions and drought in plant growth and fructans content of Agave potatorum Zucc. HortScience, 54(9), 1581–1584. DOI 10.21273/HORTSCI14129-19. [Google Scholar] [CrossRef]
14. Murashige, T., Skoog, F. (1962). A revised medium for rapid growth and bioassays with tobacco tissue cultures. Physiologia Plantarum, 15(3), 473–497. DOI 10.1111/j.1399-3054.1962.tb08052.x. [Google Scholar] [CrossRef]
15. Monja-Mio, K. M., Robert, M. L. (2013). Direct somatic embryogenesis of Agave fourcroydes Lem. through thin cell layer culture. In Vitro Cellular & Developmental Biology—Plant, 49(5), 541–549. [Google Scholar]
16. Lecona-Guzmán, C. A., Reyes-Zambrano, S., Barredo-Pool, F. A., Abud-Archila, M., Montes-Molina, J. A. et al. (2017). In vitro propagation of Agave americana by indirect organogenesis. HortScience, 52(7), 996– 999. DOI 10.21273/HORTSCI10498-16. [Google Scholar] [CrossRef]
17. Jin, C. S., keng, C. L. (2013). Factors affecting the selection of callus cell lines and the preparation of the cell suspension culture of Artemisia annua L. Plant Tissue Culture and Biotechnology, 23(2), 157–163. DOI 10.3329/ptcb.v23i2.17507. [Google Scholar] [CrossRef]
18. Martínez-Torres, A. C., Uscanga-Palomeque, A. C., Rodríguez-Padilla, C. (2016). In vitro cytotoxic activity against tumor cells. In: Rivas-Morales, C., Oranday-Cardenas, M. A., Verde-Star, M. J. (Eds.Research on medically important plants, pp. 269–312. Barcelona, España: OmniaScience. [Google Scholar]
19. Arnon, A. N. (1967). Method of extraction of chlorophyll in the plants. Agronomy Journal, 23, 112–121. [Google Scholar]
20. Inskeep, W. P., Bloom, P. R. (1985). Extinction coefficients of chlorophyll a and b in N, N-Dimethylformamide and 80% acetone. Plant Physiology, 7(2), 483–485. DOI 10.1104/pp.77.2.483. [Google Scholar] [CrossRef]
21. Bates, L. S., Waldren, R. P., Teare, I. D. (1973). Rapid determination of free proline for water-stress studies. Plant and Soil, 39(1), 205–207. DOI 10.1007/BF00018060. [Google Scholar] [CrossRef]
22. Hamissa, A. M. B., Seffen, M., Aliakbarian, B., Casazza, A. A., Perego, P. et al. (2012). Phenolics extraction from Agave americana (L.) leaves using high-temperature, high-pressure reactor. Food and Bioproducts Processing, 90(1), 17–21. DOI 10.1016/j.fbp.2010.11.008. [Google Scholar] [CrossRef]
23. Maazoun, A. M., Hamdi, S. H., Belhadj, F., Jemâa, J. M. B., Messaoud, C. et al. (2019). Phytochemical profile and insecticidal activity of Agave americana leaf extract towards Sitophilus oryzae (L.) (Coleoptera: Curculionidae). Environmental Science and Pollution Research, 26(19), 19468–19480. DOI 10.1007/s11356-019-05316-6. [Google Scholar] [CrossRef]
24. Dumanović, J., Nepovimova, E., Natić, M., Kuča, K., Jaćević, V. (2020). The significance of reactive oxygen species and antioxidant defense system in plants: A concise overview. Frontiers in Plant Science, 11, 552969. DOI 10.3389/fpls.2020.552969. [Google Scholar] [CrossRef]
25. Portillo, L., Santacruz-Ruvalcaba, F. (2006). Obtaining Agave tequilana Weber embryoids from root explant. Zonas Áridas, 10(1), 11–19. DOI 10.21704/za.v10i1.549. [Google Scholar] [CrossRef]
26. Reyes-Zambrano, S., Lecona-Guzmán, C., Ambrosio-Calderón, J., Abud-Archila, M., Rincón-Rosales, R. et al. (2016). Plant growth regulators optimization for maximize shoots number in Agave americana L. by indirect organogénesis. Gayana Botánica, 73(1), 124–131. DOI 10.4067/S0717-66432016000100014. [Google Scholar] [CrossRef]
27. Cancino-García, V. J., Ramírez-Prado, J. H., De-la-Peña, C. (2020). Auxin perception in Agave is dependent on the species’ auxin response factors. Scientific Reports, 10(1), 3860. DOI 10.1038/s41598-020-60865-y. [Google Scholar] [CrossRef]
28. Gallego-Rúa, A. M., Henao-Ramírez, A. M., Urrea-Trujillo, A. I., Atehortúa-Garcés, L. (2016). Polyphenols distribution and reserve substances analysis in cacao somatic embryogenesis. Acta Biologica Colombiana, 22(2), 335–345. DOI 10.15446/abc.v21n2.50196. [Google Scholar] [CrossRef]
29. Pádua, M. S., Paiva, L. V., da Silva, L., Do Livramento, K. G., Alves, E. et al. (2014). Morphological characteristics and cell viability of coffee plants calli. Ciência Rural, 44(4), 660–665. DOI 10.1590/S0103-84782014000400014. [Google Scholar] [CrossRef]
30. Irene, W. M., Asava, K. K., Anami, S. E. (2019). Effects of genotype and plant growth regulators on callus induction in leaf cultures of Coffea arabica L. F1 hybrid. Journal of Plant Biochemistry and Physiology, 7(2), DOI 10.35248/2329-9029.19.7.236. [Google Scholar] [CrossRef]
31. Ijaz, B., Sudiro, C., Hyder, M. Z., Malik, S. I., Farrakh, S. et al. (2019). Histo-morphological analysis of rice callus cultures reveals differential regeneration response with varying media combinations. In Vitro Cellular & Developmental Biology—Plant, 55, 569–580. DOI 10.1007/s11627-019-09974-6. [Google Scholar] [CrossRef]
32. Ribeiro, L. O., Paiva, L. C., Pádua, M. S., Santos, B. R., Alves, E. et al. (2012). Morphological and ultrastructural analysis of various types of banana callus, cv. Prata anã. Acta Scientiarum. Agronomy, 34(4), 423–429. DOI 10.4025/actasciagron.v34i4.14501. [Google Scholar] [CrossRef]
33. Santacruz-Ruvalcaba, F., Portillo, L. (2009). Thin cell suspension layer as a new methodology for somatic embryogenesis in Agave tequilana Weber cultivar azul. Industrial Crops and Products, 29(2–3), 609–614. DOI 10.1016/j.indcrop.2008.12.001. [Google Scholar] [CrossRef]
34. Kartosentono, S., Indrayanto, G., Zaini, N. C. (2002). The uptake of copper ions by cell suspension cultures of Agave amaniensis, and its effect on the growth, amino acids and hecogenin content. Plant Cell, Tissue and Organ Culture, 68(3), 287–292. DOI 10.1023/a:1013920919889. [Google Scholar] [CrossRef]
35. Attree, S. M., Moore, D., Sawhney, V. K., Fowke, L. C. (1991). Enhanced maturation and desiccation tolerance of white spruce [Picea glauca (Moench) Voss] somatic embryos: Effects of a non-plasmolysing water stress and abscisic acid. Annals of Botany, 68(6), 519–552. DOI 10.1093/oxfordjournals.aob.a088291. [Google Scholar] [CrossRef]
36. Hernández-Pérez, C. A., Gómez-Merino, F. C., Spinoso-Castillo, J. L., Bello-Bello, J. J. (2021). In vitro screening of sugarcane cultivars (Saccharum spp. Hybrids) for tolerance to polyethylene glycol-induced water stress. Agronomy, 11(3), 598. DOI 10.3390/agronomy11030598. [Google Scholar] [CrossRef]
37. Aazami, M. A., Torabi, M., Jalili, E. (2010). In vitro response of promising tomato genotypes for tolerance to osmotic stress. African Journal Biotechnology, 9(26), 4014–4017. [Google Scholar]
38. Khodarahmpour, Z. (2011). Effect of drought stress induced by polyethylene glicol (PEG) on germination in dices in corn (Zea mays L.) hybrids. African Journal Biotechnology, 10(79), 18222–18227. DOI 10.5897/AJB11.2639. [Google Scholar] [CrossRef]
39. Saepudin, A., Khumaida, N., Sopandie, D., Sintho, W. A. (2017). In vitro selection of four soybean genotypes using PEG for drought tolerance. Journal Agronomi Indonesia, 45(1), 14–22. DOI 10.24831/jai.v45i1.13749. [Google Scholar] [CrossRef]
40. Helaly, M. N., El-Hosieny, H. A. R., El-Sarkassy, N. M., Fuller, M. P. (2017). Growth, lipid peroxidation, organic solutes, and anti-oxidative enzyme content in drought-stressed date palm embryogenic callus suspension induced by polyethylene glycol. In Vitro Cellular & Developmental Biology, 53(2), 133–141. DOI 10.1007/s11627-017-9815-8. [Google Scholar] [CrossRef]
41. Masoabi, M., Lloyd, J., Kossmann, J., van der Vyver, C. (2017). Ethyl methanesulfonate mutagenesis and in vitro polyethylene glycol selection for drought tolerance in sugarcane (Saccharum spp.). Sugar Tech, 20(1), 50–59. DOI 10.1007/s12355-017-0524-8. [Google Scholar] [CrossRef]
42. Kareem, T., Karrar, A. (2018). Biochemical and physiological changes of callus growth and lycopene pigment production from tomato (Lycopersicon esculentum Mill.) under drought stress. Himalayan Journal of Health Sciences, 3(1), 7–21. DOI 10.22270/ijist.v3i1.9. [Google Scholar] [CrossRef]
43. Haruna, M. K., Aguoru, C. U., Iheuwumere, C. C., Ogbonna, C. I. C., Salisu, I. D. (2019). In vitro callus induction potentials of wheat genotypes using mature embryo as ex-plant source under different levels of Polyethylene glycol (PEG). Journal of Biological Sciences and Bioconservation, 11(2), 34–56. [Google Scholar]
44. Mishra, N., Tripathi, M. K., Tiwari, S., Tripathi, N., Sapre, S. et al. (2021). Cell suspension culture and in vitro screening for drought tolerance in soybean using poly-ethylene glicol. Plants, 10(3), 517. DOI 10.3390/plants10030517. [Google Scholar] [CrossRef]
45. Silva, H., Sagardia, S., Ortíz, M., Franck, N., Opazo, M. et al. (2014). Relationships between leaf anatomy, morphology, and water use efficiency in Aloe vera (L.) Burm f. as a function of water availability. Revista Chilena de Historia Natural, 87, 13. DOI 10.1186/s40693-014-0013-3. [Google Scholar] [CrossRef]
46. Silva, H., Sagardia, S., Seguel, O., Torres, C., Franck, N. et al. (2010). Effect of water availability on growth and water use efficiency for biomass and gel production in aloe vera (Aloe barbadensis M.). Industrial Crop Production, 31(1), 20–27. DOI 10.1016/j.indcrop.2009.08.001. [Google Scholar] [CrossRef]
47. Anjum, S. A., Ashraf, U., Tanveer, M., Khan, I., Hussain, S. et al. (2017). Drought induced changes in growth, osmolyte accumulation and antioxidant metabolism of three maize hybrids. Frontier ins Plant Science, 8(69), 19. DOI 10.3389/fpls.2017.00069. [Google Scholar] [CrossRef]
48. Shivakrishna, P., Reddy, K. A., Rao, D. M. (2018). Effect of PEG-6000 imposed drought stress on RNA content, Relative water content (RWCand Chlorophyll content in peanut leaves and roots. Saudi Journal of Biological Sciences, 25(2), 285–289. DOI 10.1016/j.sjbs.2017.04.008. [Google Scholar] [CrossRef]
49. Bergsten, S. J., Stewart, J. R. (2014). Measurement of the influence of low water availability on the productivity of Agave weberi cultivated under controlled irrigation. Canidian Journal Plant Science, 94(2), 439–444. DOI 10.4141/cjps2013-256. [Google Scholar] [CrossRef]
50. Ramírez-Tobías, H. M., Cedillo-de-la-Rosa, R. A., Flores, J., López-Palacios, C. (2021). Seedlings from two Agave species differing in microhabitat evolve different tolerance mechanisms to drought and shade under nurse plants. Flora, 274, 151729. DOI 10.1016/j.flora.2020.151729. [Google Scholar] [CrossRef]
51. Peña-Valdivia, C. B., Sánchez-Urdaneta, A. B. (2009). Effects of substrate water potential in root growth of Agave salmiana Otto ex Salm-Dyck seedlings. Biological Research, 42(2), 239–248. DOI 10.4067/S0716-97602009000200013. [Google Scholar] [CrossRef]
52. Riaz, S., Aftab, B., Sarwar, M. B., Batool, F., Iqbal, F. et al. (2016). Adaptations of plant responses in Agave sisalana under drought stress conditions. Journal of Biodiversity and Environmental Sciences, 9(4), 114–123. [Google Scholar]
53. Delatorre-Herrera, J., Delfino, I., Salinas, C., Silva, H., Cardemil, L. (2010). Irrigation restriction effects on water use efficiency and osmotic adjustment in Aloe Vera plants (Aloe barbadensis Miller). Agricultural Water Management, 97(10), 1564–1570. DOI 10.1016/j.agwat.2010.05.008. [Google Scholar] [CrossRef]
Cite This Article
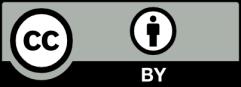
This work is licensed under a Creative Commons Attribution 4.0 International License , which permits unrestricted use, distribution, and reproduction in any medium, provided the original work is properly cited.