Open Access
ARTICLE
A Rapid Determination of 30 Prohibited Pesticide Residues in Lycii Fructus by UPLC-MS/MS
1 KMHD, Shenzhen, 518128, China
2 Shenzhen Key Laboratory of Edible and Medicinal Bioresources, HKUST Shenzhen Research Institute, Shenzhen, 518057, China
3 Kangmei Pharmaceutical Co., Ltd., Jieyang, 515300, China
* Corresponding Authors: Ran Duan. Email: ; Ning Li. Email:
# These two authors contributed equally to this work
Phyton-International Journal of Experimental Botany 2023, 92(2), 423-437. https://doi.org/10.32604/phyton.2022.023763
Received 13 May 2022; Accepted 06 June 2022; Issue published 12 October 2022
Abstract
Prohibited pesticide residues have become one of the main factors affecting the quality and safety of Lycii Fructus, However, rarely studies focus on the rapid determination of these residues. Here, a total of 30 kinds of prohibited pesticide residues were determined by ultra-performance liquid chromatography tandem triple quadrupole mass spectrometry (UPLC-MS/MS) in five different process ways. Pretreatment methods, chromatographic separation and detection conditions in mass spectrometry were all optimized accordingly. Among the five different pretreatment methods, the first and third solid phase extraction failed to provide high recoveries of sulfosulfuron compounds (both lower than 60%). Recovery of chlorphenamidine by the Quick Easy Cheap Effective Rugged and Safe multiresidue method (QuEChERS) was lower than 60%, which did not meet the requirements of trace determination. The concentrations of 30 prohibited pesticides residues treated by straightforward and solid phase extraction showed good linearity in their corresponding ranges, with correlation coefficients over 0.99. The average recoveries of straightforward ranged from 78.13% to 110.9%, while RSD ranged from 1.3% to 16.9%, albeit poor purification was observed. The recovery yield from solid phase extraction was between 67.75% and 103.08% with RSD value from 0.8% to 14.0%, which met the requirements of trace determination, this method has good precision and stability. These results could be employed to other Traditional Chinese Medicines (TCMs) in detecting prohibited pesticide residues.Keywords
Lycii Fructus is a “berry-type” fruit of the plant Lycium barbarum, which is a deciduous shrub belonging to the family Solanaceae. It is a valuable traditional herbal medicine, as well as one of the homologous varieties of medicine and food in China [1]. In recent years, Lycii Fructus has become an important economic crop in the northwest China and planting area is expanding rapidly [2]. With the increase of planting years, as well as abnormal changes in climate, Lycii Fructus pests and diseases are becoming more and more serious, which makes our prevention and control extremely difficult. At present, chemical control is the main way to control pests and diseases in the production of Lycii Fructus [3]. While due to the farmers’ unregulated use of pesticide, it brings hidden dangers to the quality and safety of Lycii Fructus [4,5]. Although the government enacted of regulations in 2014 stipulating 33 banned and prohibited pesticides and 17 pesticides restricted to plant crops, incidents of non-standard use of pesticides by farmers or recessive addition of prohibited ingredients to pesticides still occur from time to time. The prohibited pesticide residues have become a great issue to the Lycii Fructus, which is of great concern to the society and regulatory authorities. Therefore, the determination of prohibited pesticide residues in Lycii Fructus has become a necessity in view of the toxicity and stability of these xenobiotics [3,6]. Some laboratories determining prohibited pesticide residues are still using procedures developed 30 years ago, when analytical and legal requirements were less rigorous and technology was not as advanced as it is today. Traditional procedures are time-consuming, labour-intensive, complicated and expensive; moreover, they produce considerable quantities of wastes, and frequently, a sufficiently low limit of detection is unobtainable [7–9].
In this study, based on the 2020 edition of “Pharmacopoeia of the people’s Republic of China” (hereinafter referred to as “Chinese Pharmacopoeia”) contains 33 kinds of prohibited pesticides in plants and 5 kinds of sample pretreatment methods [10]. A quick and inexpensive procedure has been described for determining pesticide residues in Lycii Fructus providing reliable results whilst reducing the number of essential analytical steps, as well as quantities of reagents and laboratory glassware. Acetonitrile was used as extraction solvent, multiple reaction monitoring (MRM) mode of UPLC-MS/MS was used to detect, no less than two groups of characteristic ion pairs were used for qualitative analysis, and the pesticide residues of Lycii Fructus were detected quantitatively by external standard calibration curve method. Based on the strong specificity of traditional Chinese medicine, we optimized the UPLC-MS detection methods in pharmacopoeia, selected the best pretreatment method according to the characteristics of sample matrix, and improved the liquid quality analysis conditions [11]. In addition, in order to provide reference for the edible and medicinal safety of Lycii Fructus, 30 prohibited pesticide residues were detected and assessed on the collected food-grade and pharmaceutical-grade Lycii Fructus. Through verification, this methodology simplifies the extraction of analytes and extract cleanup without adversely affecting the magnitude of analyte recoveries, and can be used for rapid screening and determination of prohibited pesticide residues in Lycii Fructus.
Pesticide standards with purity greater than 98% or higher were purchased from Shanghai Ambrosia Pharmaceutical Co., Ltd. (Shanghai, China). The HPLC grade acetonitrile, ethanol and formic acid were from Merck (Darmstadt, Germany). Ultrapure water was supplied with a Direct-Q water purification system (Millipore, Milford, MA, USA).
The blank samples of Lycii Fructus were provided by Shenzhen Key Laboratory of Edible and Medicinal Bioresources at Hong Kong University of Science and Technology, and have been screened without the pesticides measured in this study. The experiment samples in a total of 22 batches were collected from Ningxia, China, in which except F1–F4 for food grade, the other 18 samples (P1–P18) were all pharmaceutical grades, they were cut into small pieces and ground into powder before sieving through a 100# mesh sieve. Details of batches and origin could be found in Appendix A. All the Lycii Fructus mentioned above were identified by Ning Li.
2.2.1 Straightforward Extraction Method
5.0 g samples and 1.0 g NaCl were homogenized (speed not less than 12,000 revolutions per minute), and then 50 mL mL acetonitrile were added to it. With standing at ambient temperature for 2 min, the mixture was centrifuged for 5 min at 4,000 rpm at 4°C. After collecting the supernatant, another 50 mL acetonitrile was added to the residues, repeat the above steps to extract once again, combine the supernatant and evaporated to 3~5 mL and diluted to 10 mL by acetonitrile for UPLC-MS/MS analysis.
2.2.2 QuEChERS Multiresidue Method
Extraction was carried out following modified version of the QuEChERS multi residue method [12]. Briefly, 3.0 g homogenised samples were weighed in to a 50 mL Teflon centrifuge tube and soaked with 15 mL 1% acetic acid at ambient temperature for half an hour, another 15 mL acetonitrile was added to the samples, and the mixture was shaken for 5 min. Afterward, 1.5 g NaCl and 6.0 g MgSO4 were added. The mixture was shaken immediately for 3 min by a vortex mixer to prevent the formation of coagulated MgSO4 and was centrifuged for 5 min at 4,000 rpm at 4°C, and clean-up using 0.9 g MgSO4, 0.3 g primary secondary amine (PSA), 0.3 g of octadecyl silane bonded silica gel, 0.3 g silica gel and 0.2 g graphitized carbon black (GCB), the tubes were shaken vigorously for 5 min and then centrifuged for 5 min at 4,000 rpm at 4°C. After centrifugation, 5 mL of the cleaned extracts was transferred into test tubes and evaporated to dryness under a stream of nitrogen. The residues were immediately reconstituted in 1 mL acetonitrile for UPLC-MS/MS analysis.
2.2.3 Solid-Phase Extraction Method
Mode I: 4 mL of the test solution prepared by straightforward method was added to a purification tube with dispersion-type purification material (1.2 g MgSO4, 0.3 g PSA,0.1 g Octadecyl silane bonded silica gel), The tubes were shaken vigorously for 5 min and then centrifuged for 5 min at 4,000 rpm at 4°C. After centrifugation, transferred the cleaned extracts into test tubes and evaporated to dryness under a stream of nitrogen. The residues were immediately reconstituted in 4 mL acetonitrile for UPLC-MS/MS analysis.
Mode II: 4 mL of the test solution prepared by straightforward method was added to a solid phase extraction column (200 mg, 6 mL) with hydrophilic-lipophilic equilibrium material (HLBSPE), collected all the purification solution and then centrifuged for 5 min at 4,000 rpm at 4°C. After centrifugation, transferred the cleaned extracts into test tubes and evaporated to dryness under a stream of nitrogen. The residues were immediately reconstituted in 4 mL acetonitrile for UPLC-MS/MS analysis
Mode III: Extraction was carried out following a modified version of the published study [13]. 2 mL of the test solution prepared by straightforward method was added to a solid phase extraction column (500 mg/500 mg, 6 mL) with graphitized carbon black amino composite, pre-wash with 10 mL acetonitrile-toluene mixed solution (3:1) before use. Eluate with 20 mL acetonitrile-toluene mixed solution (3:1), collected all the purification solution and then centrifuged for 5 min at 4,000 rpm at 4°C. After centrifugation, transferred the cleaned extracts into test tubes and evaporated to dryness under a stream of nitrogen. The residues were immediately reconstituted in 2 mL acetonitrile for UPLC-MS/MS analysis.
2.3 Sample Preparation for UPLC-MS/MS
100 μL of various standard products were mixed, and the volume was set to 20 mL by acetonitrile to obtain the mixed standard solutions. The blank matrix solutions of Lycii Fructus were prepared according to the method of “2.2”, 1 mL of the solutions were added to 10 centrifuge tubes separately, and blow dry under nitrogen. After that 5, 10, 20, 50, 100, 150, 200, 250, 300, 350 μL of the mixed standard solutions was added to it, respectively, and then added up to 1.0 mL with acetonitrile, followed by 0.3 mL ultrapure water. The resulted solutions were used as matrix calibration samples. All the 22 test matrix solutions of Lycii Fructus were prepared according to the method of “2.2”, 1 mL of the solutions were added to tubes separately and blow dry under nitrogen. After that 1.0 mL acetonitrile was added to it, followed by 0.3 mL ultrapure water. The resulted solutions were used as test samples. Each sample was vortexed for 1 min and centrifuged at 12,000 rpm for 10 min. A volume of 2 µL aliquot of each supernatant was injected into UPLC-MS/MS for analysis.
Chromatographic analysis was performed on an A30 Altus™ UPLC system (PerkinElmer, Waltham, MA, USA) [14]. The autosampler was kept at 4°C. Sample separation was achieved on a Waters CORTECS UPLC T3 (100 mm × 2.1 mm, 1.6 μm) (Waters, Milford, MA, USA) column with a constant flow rate of 0.2 mL/min at 30°C. The mobile phase was composed of water (0.1% formic acid, 2 mmol/L ammonium formate, A) and acetonitrile (0.1% formic acid, B), using a gradient elution of 0~5 min, 5% B; 0.5~10 min, 5%~90% B; 10~12 min, 90% B; 12~12.5 min, 90%~5% B; 12.5~17 min, 5% B and maintained at 5% mobile phase B for an additional 2 min for re-equilibration. The injected volume was set at 2 µL. Detection was performed by Qsight™ 220triple quadrupole mass spectrometer (PerkinElmer, Waltham, MA, USA), using multiple reaction monitoring (MRM) in the positive ion mode. The acquired parameters were optimized as follows: drying gas value, 100 μL/min; nebulizer gas value, 200 μL/min; electrospray voltage, 5 kV; HSID temperature, 250°C.
Simplicity 3QTMsoftware platform (PerkinElmer, Waltham, MA, USA) was used for data acquisition and processing. The concentrations of each pesticide residues were calculated according to the calibration curve. Data were expressed as mean ± standard deviation (SD) (n = 5). The significance of differences in data between the groups was determined by one-way ANOVA with Duncan’s test.
3.1 Establishment of UPLC-MS/MS Analysis
In order to obtain better resolution and peak shape, the effects of different buffer salts (ammonium formate, ammonium acetate) and different proportional flow equal conditions on the analysis were investigated. The results showed that with water (containing 0.1% formic acid, 2 mmol/L ammonium formate, A)-acetonitrile (containing 0.1% formic acid, B) as the mobile phase, 95% aqueous phase as the initial ratio, the injection volume was 2 μL, and the flow rate was 0.2 mL/min, the solvent effect of UPLC was significantly reduced, the resolution was significantly improved, and a better peak shape were obtained. The optimized total ion flow chromatogram of 30 prohibited pesticides was shown in Fig. 1.
Figure 1: Optimized total ion flow chromatogram of 30 prohibited pesticides. 1, Methamidophos; 2, Aldicarb-sulfoxide; 3, Aldicarb-sulfone; 4, Monocrotophos; 5, Chlordimeform; 6, Carbofuran-3-hydroxy; 7, Phosfolanh; 8, Fenamiphos-sulfoxide; 9, Aldicarb; 10, Phosphamidon; 11, Fenamiphos-sulfone; 12, Metsulfuron-methyl; 13, Carbofuran; 14, Chlorsulfuron; 15, Phorate-sulfoxide; 16, Ethametsulfuron-methyl; 17, Demeton; 18, Terbufos-sulfoxide; 19, Phorate-sulfone; 20, Isocarbophos; 21, Fenamiphos; 22, Ethoprophos; 23, Terbufos-sulfone; 24, Isazofos; 25, Cadusafos; 26, Isofenphos-methyl; 27, Coumaphos; 28, Fonofos; 29, Sulfotep; 30, Phorate
The multiple reaction monitoring (MRM) in positive ion mode was identified for each pesticide, considering sensitivity and reproducibility of dominant ions in full-scan mass spectra, as reported previously [15,16]. The 30 pesticides were firstly characterized based on their mass spectra from syringe pump infusion analysis, as to determine their precursor ions and selected product ions for MRM. Then, the parameters included capillary voltage, entrance voltage, collision energy and dwell time were optimized, based on the peak areas of analytes to minimize the matrix effect, as well as to increase overall sensitivity. The optimized parameters of these 30 pesticides are shown in Table 1.
The blank matrix samples were added with different concentrations of mixed reference substance solution, and the recovery tests were carried out according to the above five pretreatment methods and the established detection methods. The examination points were quantitative limit, twice quantitative limit and quadruple quantitative limit (QuEChERS multiresidue method was quantitative limit, 1.5x quantitative limit and double quantitative limit). The measured results are substituted into the standard curve calculation. The average sample recovery rate of five pretreatment methods and RSD (n = 3) are shown in Table 2.
The recovery rates of straightforward extraction method, QuEChERS multiresidue method, and solid-phase extraction method (I, II, III) ranged from 78.13%~110.09%, 48.04%~99.44%, 0%~98.44%, 67.75%~103.08% and 0%~91.33%, respectively. The results showed that only the straightforward extraction method and solid-phase extraction method (mode II) met the requirements of trace determination and pharmacopoeia (70%~120%).
To examine the matrix effect (ME) for the five pre-treatment methods and the blank solvent, the responses of matrix-matched standards with that of the blank solvent was compared. ME < 0 indicates that the matrix suppresses the response value of the substance to be measured, while ME > 0 indicates that the matrix enhances the response of the substance to be measured. The matrix effect is divided into three grades according to its absolute value: 0 < |ME| ≤ 20%, which is weak matrix effect, which is negligible; 20% < |ME| ≤ 50%, medium intensity matrix effect; |ME| > 50%, strong matrix effect [17]. As shown in the Appendix A, there was a significant ion enhancement effect in all tested matrixes, and a higher substrate effect was often apparent at low pesticide concentrations (usually near the LOQs). On the basis of these results, matrix-matched calibration was used to compensate for signal-enhancement effects and to ensure precision of the analysis. For the analysis, using a high-sensitivity instrument whenever possible is important. To obtain reliable test results, the samples should be diluted sufficiently to greatly reduce the matrix effects of the extraction solvent [18]. Combining the results for recovery, matrix effect, treatment conditions, background color and stability, solid-phase extraction method (mode II) would be the best extraction and clean-up method for Lycii Fructus.
The linearity, sensitivity, precision, accuracy, stability, matrix effect and extraction efficiency were determined to evaluate the integrity of the developed method according to the criteria described in the FDA guidelines for bioanalytical method validation [19]. As shown in Table 3, the calibration was conducted based on the analysis of ten-level pesticides calibration standards. Linearity was assessed by establishing the calibration curves by plotting the peak response areas (Y) against their corresponding concentrations (X, ng/mL), and were fitted by linear regression with a weight factor of 1/X. Acceptable linear correlations under these conditions were confirmed by correlation coefficients (R2) of no less than 0.9972. Analytical sensitivity was determined as limit of detection (LOD) of each pesticide, which were estimated at signal-to-noise (s/n) ratios of 3:1 and found to be in the range of 2~100 ng/mL, the accuracy was also assessed by the value of RSD of no more than 5.1%. Indicating that this method is appropriate for the quantitative determination of the 30 prohibited pesticides.
In the specificity determination, the following three conditions must be met simultaneously: retention time, simultaneous detection of qualitative and quantitative ion pairs, and consistent peak area ratio of qualitative and quantitative ion pairs. The matrix solution of Lycii Fructus blank sample was prepared by solid phase extraction, and the results showed that there was no significant interference, and the matrix mixed control solution composed of mixed control solution and Lycii Fructus blank matrix solution was used to locate and confirm each color peak (Appendix B).
Autosampler storage stability of all pesticides was evaluated by analysis of three different concentration levels (low, medium and high) of the mixed reference standards at 8 intervals over 12 h (4°C) and calculated by coefficient of variation (CV). The results showed that 93.3% of the compounds in the mixed reference solution were stable within 12 h at 4°C. The spiked recovery was used to evaluate the accuracy of the method. Three different concentration levels (low, medium and high) of the mixed reference standards were added to the known amounts of sample, and then, prepared and analyzed as described in solid-phase extraction method (mode II). Triplicate replicates at each level were analyzed to calculate the recovery according to the following equation: (found amount − original amount) × 100%/spiked amount. The RSD was used to evaluate the results. As shown in Table 3, the developed analytical method was accurate, with recoveries of 67.75%~103.08% (RSD ≤ 14%). Thus, the developed method exhibited good specificity, stability and accuracy.
The developed UPLC-MS/MS analytical method was applied to the quantitative analysis of 30 prohibited pesticide residues in 22 batches of Lycii Fructus. As shown in Table 4, only 8 pesticides were detected in these samples, including methamidophos, chlordimeform, carbofuran-3-hydroxy, carbofuran, phorate-sulfone, isocarbophos, cadusafos and coumaphos, the content of these compounds ranged from 0.1~2.2, 0.1~0.3, 0.2~12.7, 0.3~23.2, 0.5~1.4, 1.2~26.3, 0.1~1.2 and 0.8~1.7 µg/kg, respectively. Among the 8 detected pesticides, carbofuran had the highest detection rate of 90.9%, followed by carbofuran-3-hydroxy (86.4%) and cadusafos (68.2%). This figure for other 5 pesticides were from 22.7% to 45.5%. The contents of the 8 components varied greatly among the 22 batches samples (ANOVA, P < 0.05).
Based on the statistical analysis of the determination results of pesticide residues, these 22 batches of Lycii Fructus samples were divided into two categories according to food grade and pharmaceutical grade, and the sample categories were taken as grouping variables, and each pesticide residue in 22 batches of samples was taken as test variables. A new variable was the sum of carbofuran and carbofuran-3-hydroxy pesticide residues (i.e., carbofuran pesticides), and the normal distribution test was carried out on the above 9 test variables. The variables that do not conform to the normal distribution are transformed by the square root, and the converted variables are all in accordance with the normal distribution. The independent sample T-test is used to analyze the test variables. The results showed that only carbofuran pesticide residues in different grades of Lycii Fructus were significantly different (P < 0.05).
Pigments, sugars and proteins were three main interference matrices in Lycii Fructus. The matrix interference contaminated the instrument by accumulating at the ion source, resulting in unstable MS response and increasing the cost of instrument maintenance. The removal of some strong polar impurities through sample purification was helpful to reduce the sensitivity of the instrument and the impact on instrument maintenance. From the results of sample recovery, only straightforward and solid phase extraction mode II met the requirements of trace determination. The recoveries of metsulfuron-methyl, chlorosulfuron and ethametsulfuron-methyl by one and three solid phase extraction methods were all less than 1%, it was consistent with the relevant reports. It might because sulfuron pesticides contain amide bonds and form hydrogen bonds with the amino groups of N-propyl ethylenediamine and hard to elute, result in N-propyl ethylenediamine in the purification material had great adsorption to sulfuron pesticides. In terms of purification effect, compared with the straightforward method, the solid phase extraction mode II method could better remove the interference components such as pigment and reduced the pollution of the sample to the equipment, so the solid phase extraction method mode II was finally selected as the pretreatment method of Lycii Fructus. This method effectively reduced the interference of the main impurities in Lycii Fructus to the determination and met the needs of quantitative analysis as well.
The matrix effect during validation of analytical methods in biological fluids may be best examined by comparing the MS/MS response (peak areas or peak heights) of an analyte at any given concentration spiked post extraction into a biological fluid extract to the MS/MS response of the same analyte present in the “neat” mobile phase [20]. The presence of matrix effects affects the accuracy and reproducibility of the results. Matrix effects include matrix enhancement effects and matrix inhibition effects, which must be effectively overcome in trace analysis to ensure accurate determination results [21].
The results showed that in the UPLC-MS/MS analysis if the matrix was not purified (i.e., the pretreatment method was straightforward), most of the 30 pesticides showed matrix inhibition effect, and the components with shorter chromatographic retention time were more significant. The most likely reason was that the matrix components and target compounds compete with each other during the ionization of ESI, which will affect the sensitivity and repeatability of the instrument, affect the accuracy of qualitative and quantitative analysis through the decrease of sensitivity. The final treatment method of the sample selected in this experiment was solid phase extraction mode II. In the previous treatment method, only 40% of the pesticides showed matrix inhibition effect and 86.7% of pesticides showed weak matrix effect. Accurate quantitative analysis could be carried out by using blank matrix matching, and at the same time, the pollution of matrix interference to the instrument was greatly reduced. If the blank matrix was not easy to obtained, there were two suggestions: The first one is to choose single compound solution or mixed compound solution with great enhancement effect of the matrix as the analytical protective agent, such as triphenyl phosphate, or a combination of analytical protective agents, mix the prepared analytical protective agent solution with a series of volume mixed reference solution, and prepare the working curve for the determination of sample content. The second is to investigate the matrix effect of the sample solution obtained by different pretreatment methods, and select the method with weak matrix effect as a whole to investigate the sample recovery rate of different concentrations. when the repeatability is satisfied, the working curve can be prepared with the mixed reference solution, and the recovery rate can be used to correct the quantitative results.
In this study, pesticide residues in 22 batches of Lycii Fructus were screened and a total of 8 prohibited pesticides were detected, which were methamidophos, chlordimeform, carbofuran (sum of carbofuran and 3-hydroxycarbofuran), phorate-sulfone, isocarbophos, cadusafos and coumaphos, all of which were broad-spectrum pesticides. Among them, the residues of carbofuran in 4 batches of samples exceeded the relevant provisions of the maximum residue limits (MRL) of pesticides in China (GB2763-2016). It can be concluded that the prohibited pesticide residues of Lycii Fructus were as follows: Firstly, the composition of the prohibited pesticide residues in Lycii Fructus was complex and involved a wide variety of species. In particular, cadusafos and carbofuran, which were common insecticides, had high residue rates. Secondly, there was a significant difference between food grade and pharmaceutical grade carbofuran pesticide residues, the over-standard rate of food grade Lycii Fructus carbofuran pesticide was 75%, but the figure for pharmaceutical grade was at a very low level with low risk, indicating that the planting process was relatively safety. It is suggested that the relevant departments should strengthen to guide the agricultural growers of Lycii Fructus to standardize the use of pesticides and advocate the use of low-toxic pesticides, especially strengthening the regulation of insecticides.
The food-grade Lycii Fructus collected in this study were all purchased and sold in the market, and most of the pharmaceutical-grade samples were provided by traditional Chinese medicine enterprises. The former was simply dried by growers, while the latter was treated by disinfection and sterilization in the production process. Whether there were effects of different processing methods on pesticide residues remained to be evaluated. On the other hand, the sample sizes of food-grade Lycii Fructus were less than that of pharmaceutical-grade, it might lack of representativeness and comprehensiveness.
In this study, a highly automatic UPLC-MS/MS approach was developed to characterize and compare the compositional difference of 30 prohibited pesticide residues from 22 different batches Lycii Fructus. The method has been improved on the basis of the 2020 edition of Chinese Pharmacopoeia, and the final detection method has the advantages of simple pretreatment, few influencing factors, accuracy and high sensitivity. It can be used for the routine determination of multi-pesticides in TCMs.
Acknowledgement: We thank the anonymous reviewers for their helpful comments.
Authorship: The authors confirm contribution to the paper as follows: study conception and design: Kesheng Lin, Ran Duan, Ning Li; data collection: Jiawen Zhou, Li Wang; analysis and interpretation of results: Kesheng Lin, Jingjing Wen, Tingxia Dong; draft manuscript preparation: Zhengqi Wang. All authors reviewed the results and approved the final version of the manuscript.
Funding Statement: The authors received no specific funding for this study.
Conflicts of Interest: The authors declare that they have no conflicts of interest to report regarding the present study.
References
1. Zhang, X. F., Chen, J., Yang, J. L., Shi, Y. P. (2018). UPLC-MS/MS analysis for antioxidant components of Lycii Fructus based on spectrum-effect relationship. Talanta, 180, 389–395. DOI 10.1016/j.talanta.2017.12.078. [Google Scholar] [CrossRef]
2. Neelam, K., Dey, S., Sim, R., Lee, J., Au Eong, K. G. (2021). Lycii Fructus: A natural dietary supplement for amelioration of retinal diseases. Nutrients, 13(1), 246. DOI 10.3390/nu13010246. [Google Scholar] [CrossRef]
3. Wang, Y., Jin, H. Y., Sui, H. X., Zuo, T. T., Wang, Z. et al. (2018). Analysis of pesticide R esidues in wolfberry and dietary exposure risk assessment. Chinese Pharmaceutical Journal, 53(3), 182–186. DOI 10.11669/cpj.2018.03.005. [Google Scholar] [CrossRef]
4. Xue, J., Hao, L., Peng, F. (2008). Residues of 18 organochlorine pesticides in 30 traditional Chinese medicines. Chemosphere, 71(6), 1051–1055. DOI 10.1016/j.chemosphere.2007.11.014. [Google Scholar] [CrossRef]
5. Xu, W., Saiki, S., Myojin, T., Liu, Y., Zhu, B. et al. (2018). Lycii fructus extract ameliorates hydrogen peroxide-induced cytotoxicity through indirect antioxidant action. Bioscience, Biotechnology, and Biochemistry, 82(10), 1812–1820. DOI 10.1080/09168451.2018.1487274. [Google Scholar] [CrossRef]
6. Xiao, J., Xu, X., Wang, F., Ma, J., Liao, M. et al. (2019). Analysis of exposure to pesticide residues from Traditional Chinese Medicine. Journal of Hazardous Materials, 365(8), 857–867. DOI 10.1016/j.jhazmat.2018.11.075. [Google Scholar] [CrossRef]
7. Abbas, K. A., Mohamed, A., Abdulamir, A. S., Abas, H. A. (2008). A review on supercritical fluid extraction as new analytical method. American Journal of Biochemistry and Biotechnology, 4(4), 345–353. DOI 10.3844/ajbbsp.2008.345.353. [Google Scholar] [CrossRef]
8. Wilkowska, A., Biziuk, M. (2011). Determination of pesticide residues in food matrices using the QuEChERS methodology. Food Chemistry, 125(3), 803–812. DOI 10.1016/j.foodchem.2010.09.094. [Google Scholar] [CrossRef]
9. Bauer, A., Luetjohann, J., Rohn, S., Jantzen, E., Kuballa, J. (2018). Development of a suspect screening strategy for pesticide metabolites in fruit and vegetables by UPLC-Q-Tof-MS. Food Analytical Methods, 11(6), 1591–1607. DOI 10.1007/s12161-017-1143-4. [Google Scholar] [CrossRef]
10. Chinese Pharmacopoeia Commission (2020). Pharmacopoeia of People’s Republic of China, pp. 268–269. China: China Medical Science Press. [Google Scholar]
11. Masia, A., Suarez-Varela, M. M., Llopis-Gonzalez, A., Pico, Y. (2016). Determination of pesticides and veterinary drug residues in food by liquid chromatography-mass spectrometry: A review. Analytica Chimica Acta, 936, 40–61. DOI 10.1016/j.aca.2016.07.023. [Google Scholar] [CrossRef]
12. Gwon, J. H., Kim, T. K., Seo, E. K., Hong, S. M., Kwon, H. Y. et al. (2014). Multiresidue analysis of 124 pesticides in soils with QuEChERS extraction and LC-MS/MS. The Korean Journal of Pesticide Science, 18(6), 296–313. DOI 10.7585/kjps.2014.18.4.296. [Google Scholar] [CrossRef]
13. Wang, Y., Jin, H. Y., Wei, H., Sun, L., Ma, S. C. et al. (2016). Determination of forbidden and restrictted pesticides in flowers and fruits of Traditional Chinese Medicines. Chinese Pharmaceutical Journal, 51(5), 404–412. DOI 10.11669/cpj.2016.05.014. [Google Scholar] [CrossRef]
14. Wang, H. Y., Wang, C., Guo, S. C., Chen, Z. C., Peng, Z. T. et al. (2019). Polysaccharide deriving from Ophiopogonis Radix promotes metabolism of ginsenosides in the present of human gut microbiota based on UPLC-MS/MS assay. Journal of Pharmaceutical and Biomedical Analysis, 175, 112779. DOI 10.1016/j.jpba.2019.112779. [Google Scholar] [CrossRef]
15. Wang, H. Y., Guo, S. C., Peng, Z. T., Wang, C., Duan, R. et al. (2019). Ophiopogon polysaccharide promotes the in vitro metabolism of ophiopogonins by human gut microbiota. Molecules, 24(16), 2886. DOI 10.3390/molecules24162886. [Google Scholar] [CrossRef]
16. Jiang, P., Lu, Y., Chen, D. (2017). Qualitative and quantitative analysis of multiple components for quality control of Deng-Zhan-Sheng-Mai capsules by ultra high performance liquid chromatography tandem mass spectrometry method coupled with chemometrics. Journal of Separation Science, 40(3), 612–624. DOI 10.1002/jssc.201600744. [Google Scholar] [CrossRef]
17. Kmellar, B., Fodor, P., Pareja, L., Ferrer, C., Martinez-Uroz, M. A. et al. (2008). Validation and uncertainty study of a comprehensive list of 160 pesticide residues in multi-class vegetables by liquid chromatography-tandem mass spectrometry. Journal of Chromatography A, 1215(1–2), 37–50. DOI 10.1016/j.chroma.2008.10.121. [Google Scholar] [CrossRef]
18. Lin, X. Y., Mou, R. X., Cao, Z. Y., Cao, Z. Z., Chen, M. X. (2018). Analysis of pyrethroid pesticides in Chinese vegetables and fruits by GC-MS/MS. Chemical Papers, 72(8), 1953–1962. DOI 10.1007/s11696-018-0447-1. [Google Scholar] [CrossRef]
19. U.S. Department of Health and Human Services, Food and Drug Administration, CDER (2018). Guidance for industry: Bioanalytical method validation. USA: Rockville, MD. [Google Scholar]
20. Matuszewski, B. K., Constanzer, M. L., Chavez-Eng, C. M. (2003). Strategies for the assessment of matrix effect in quantitative bioanalytical methods based on HPLC-MS/MS. Analytical Chemistry, 75(13), 3019–3030. DOI 10.1021/ac020361s. [Google Scholar] [CrossRef]
21. Gosetti, F., Mazzucco, E., Zampieri, D., Gennaro, M. C. (2010). Signal suppression/enhancement in high-performance liquid chromatography tandem mass spectrometry. Journal of Chromatography A, 1217(25), 3929–3937. DOI 10.1016/j.chroma.2009.11.060. [Google Scholar] [CrossRef]
Appendix
Appendix B: Total ion flow chromatogram of blank matrix of Lycii Fructus (−), mixed standards (−) and blank matrix + mixed standards (−)
Cite This Article
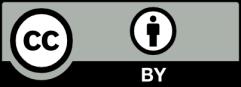
This work is licensed under a Creative Commons Attribution 4.0 International License , which permits unrestricted use, distribution, and reproduction in any medium, provided the original work is properly cited.