Open Access
ARTICLE
Types of Irrigation Water and Soil Amendment Affect the Growth and Flowering of Petunia x alkinsiana ‘Bravo Pinc’
1 Department of Plant Production, College of Food and Agriculture Sciences, King Saud University, Riyadh, 11671, Saudi Arabia
2 Department of Vegetable and Floriculture, Faculty of Agriculture, Mansoura University, Mansoura, 35516, Egypt
* Corresponding Author: Khalid M. Elhindi. Email:
(This article belongs to the Special Issue: Symbiotic Associations for Nutrients Management and Complexes Formation for Better Agricultural Crops Productivity under Biotic and Abiotic Stresses)
Phyton-International Journal of Experimental Botany 2023, 92(2), 487-499. https://doi.org/10.32604/phyton.2022.022850
Received 29 March 2022; Accepted 14 June 2022; Issue published 12 October 2022
Abstract
Water insufficiency is the hampering feature of crop sustainability, especially in arid and semi-arid regions. So, the effectual usage of all water resources especially underground brackish water represents the core priority in Saudi Arabia. The present study aimed to recognize the influence of different types of water irrigation (tap water as a control, salinized well water, and magnetized salinized well water) with or without soil amendments (soil without any amendment as a control, peat-moss, ferrous sulfate, and peat-moss plus ferrous sulfate) on petunia plant growth and flowering as well as ion content. Irrigating Petunia plants with saline well water adversely affected growth and flowering as compared to tap water and magnetized saline well water. Additionally, plants irrigated with magnetized water showed a significant enhancement in all the studied vegetative and flowering growth parameters as compared to those irrigated with salinized well water. Furthermore, mineral contents and survival of Petunia plants irrigated with magnetized well water were higher than those irrigated with tap water. Irrigation with magnetized well water significantly reduced levels of Na+ and Cl− ions in leaves of Petunia plants indicating the role of magnetization in alleviating harmful effects of salinity. In conclusion, we recommend the utilization of magnetized saline well water for irrigating Petunia plants either alone or in combination with soil amendments (peat moss plus ferrous sulfate).Keywords
Water scarcity is the restricting factor for crop sustainability and agricultural development worldwide. The agricultural usage in the Kingdom of Saudi Arabia (KSA) had the biggest water consumption (about 88% of all water utilized); therefore, the efficient use of all water resources is the core priority, especially for floriculture and ornamental plants [1]. Prominent saline water has provoked a desertification phenomenon and saline soil development that disturbs critical plant growth and production [2]. The drastic effects of salinity on plants are coupled with ionic, osmotic, and oxidative stress [3–6]. Therefore, recent agriculture endeavors are currently in search of a competent, eco-friendly approach to sustain the properties of irrigation water, and develop valid irrigation practices to avoid contributing to disaster in arid and semi-arid regions, like KSA, without any harmful effects on plant productivity. Recently, different approaches have been employed to counteract the stress effect among crops, including nutritional management strategies, application of chemical and biological amendments, and utilizing biosensors [7–9] and magnetic water treatments [10].
Water exposed to a magnetic field or bypassed via a magnetic device is identified as magnetic water (MW) that changes its properties, thus it can be utilized for diverse agriculture purposes. The MW treatment has been recorded to alter several physic-chemical properties of water such as dropping the surface tension, increasing nutrient dissolvability for plant growth [11], and decreasing water viscosity that will increase the ability to enter root cells [12], it can also alter refractive index, melting temperature, conductivity and pH [13]. Furthermore, magnetic treatment re-structures the water molecules into extremely tiny clusters with stronger inter-cluster hydrogen bonds [14]. Each one is a small and identical hexagonal shape, so it can simply penetrate the passageway in plants [15]. There are debatable issues about the utilization of MW in crop production because the stated outcome has small reproducibility and slight reliability and it was rarely established by physicists. The influence of MW depends on the crop varieties, magnetic field pathway length, and flow rate [16]. The MW enhanced crop growth and productivity [10,17,18]. Over the years numerous studies demonstrating an encouraging boost in plant production, a decline in plant diseases, enhancement of crop quality, and increases in fertilizers’ efficiency [10,19]. Likewise, MW improved some plant metabolic processes, enhanced photosynthetic pigment, and improved photosynthetic rate and translocation of photoassimilates [8]. These results propose that MW can be used as the most novel eco-friendly treatment to improve plant productivity with saline water. Additionally application of MW boosts the proficient exploitation of the arable land utilizing the available water spring in crop production globally [15]. The cost of the application of MW to enhance crop production is justifiable on a long-term basis and eco-friendly in particular in-country that suffers spatially and temporally from water shortage, in the context of atmosphere alteration linked with salinity injury.
Soil amendments with peat-moss (PM) and ferrous sulfate (FS) might be utilized to moderate the depressed impacts of soil salinity as a result of irrigation with saline water [10]. Iron (Fe) is a vital micronutrient for all biota, it contributes to numerous metabolic pathways such as nucleic acid assimilation, chlorophyll accumulation, activation of numerous enzymes, and redox homeostasis [20]. Cakmak [21] stated that Fe deficiency is prevalent in about 30%–50% of cultivated soils worldwide, so its deficiency might a confusion in plant metabolic processes. The application of Fe fertilization with FS represents the most applicable and efficient approach to recover crop Fe efficiency [22].
Petunia, which originated from South America, is a genus of the Solanaceae family. Petunia hybrid is one of the most popular ornamental plant species worldwide due to its various shapes, and colors [23]. It is widely cultivated as a potting and bedding flowering plant to decorate the landscape. However, Petunia plants have been reported as sensitive to salinity levels [24]. In the present study, we evaluated the effects of irrigation water type (tap water, salinized well water, and magnetized salinized well water) with or without soil supplementation on Petunia plant growth and flowering to exploit underground brackish water. The experimental results may participate in reducing the utilization of fresh water for irrigation of lawns and public gardens in KSA that suffers from scarcity of fresh water and high costs of desalinization seawater. Additionally, the outcomes may be introducing an innovative strategy for utilizing salinized well water and maintaining water sustainability.
2.1 Plant Material, Experimental Site, and Seedling Establishment
The present study was done at the Sustainability and Environmental Development Department Nursery, King Saud University, KSA (Coordinates: 24.722°N 46.627°E). For the seedling establishment, P. x alkinsiana ‘Bravo Pinc’ seeds were sown in germination foam trays with PM for 30 days in a semi-controlled plastic greenhouse (11–13 h photoperiod, 24/20°C day/night temperature, and 70% relative humidity).
2.2 Soil and Irrigation Water Analyses
To assess, the physic-chemical characteristics of the experimental soil (sandy soil), soil samples at 0–30 and 30–50 cm depth were collected and its pH, electrical conductivity, cation exchange capacity (CEC), and the percentage of organic components, calcium carbonate, nitrogen (N+), phosphorous (P−), and potassium (K+) were determined following Page et al. [25] protocol (Table 1).
Additionally, the irrigation water samples, i.e., tap water (TW) as a control, magnetized salinized well water (MW), and salinized well water (WW) were analyzed for their pH, electrical conductivity, sodium adsorption ratio (SAR), K+, calcium (Ca2+), magnesium (Mg2+), sodium (Na+), chloride (Cl−), sulfate (SO42−), carbonate (CO32−), and bicarbonate (HCO3−) as indicated in Table 2.
2.3 Experimental Layout and Irrigation Water Treatments
The experiment was designed following the split-plot in a completely randomized block design with three replicates in an open field, each plot was 12 m2, containing 24 plants (Fig. 1c). Irrigation water type (TW as a control, MW, and WW) represent the main factor, meanwhile, soil supplementation (sandy soil with organic manure as a control for soil amendments, PM, FS, and PM + FS) represent the sub-factor. The experimental area was separated into 36 plots and the treatments were randomly scattered. PM was added to the sandy soil in selected plots at 3:1 (v:v) ratio, meanwhile, FS was used at 100 g/selected plots following the appearance of the 3rd leaf of P. x alkinsiana plants (83.3 kg FS hectar−1). For PM addition, the experimental plots were dug, then the resulting soil was mixed with PM at 3:1 (v:v), then added to the digging plots. After that, the experimental plots were surface leveling. Fertilization with Sangral NPK 20:20:20 was done twice, at cultivation and two months from cultivation, at the rate of 0.907 kg/30 m2.
Figure 1: Preparation and experimental design at Sustainability and Environmental Development Department, Nursery, King Saud University, KSA. (a) soil digging, (b) tanks with different water types and (c) overview of the experiment
Drip irrigation systems with drippers that produced 8 L/ha flow rate were used in this study. The system (Fig. 1b) had three separate tanks (5000 L) for each irrigation water type (TW, MW, and WW). The tank for MW was connected with a three-unit of 8000 GMX magnetic fluid conditioner (GMX Corporation, USA), that gave 1500 Gouss for each unit, to magnetize the water before irrigation. The amount of irrigation water delivered for sufficient irrigation levels was planned centered on the reference evapotranspiration (ETo). The experimental site’s ETo was assessed with the FAO-CROPWAT model and the monthly average climatic data over 5 years before the experiment.
2.4 Measurements of Growth and Flowering Parameters
At 90 days from planting, plant samples (n = 6) were harvested (two from each plot) to estimate their growth and flowering attributes including, the height of the plants, leaf, and branches number/plant, stem diameter, leaf area/plant, shoot dry matter, flower number/plant, flower dry matter and survival percentage (survival% = survival plants at the end of experiment/number of planted plants). Moreover, the number of days to flowering was recorded in each treatment.
2.5 Determination of Mineral Contents
Grounded dried plant materials were wet digested by perchloric and sulfuric acid mixtures and diluted to a known volume. N, P, Ca2+, Mg2+, Fe, K+, Na+, and Cl− were estimated in the resultant digested solution following the protocols presented in AOAC [26], using micro-kjeldahl (N); phosphor-molybdic (P), versenate (Ca2+, and Mg2+), atomic absorption (Fe), flame photometer (K+ and Na+) and silver nitrate (Cl−) protocols.
The data were analyzed by two-way analysis of variance using the SAS v9.4 software (Carey, USA) and significant treatment means were compared using the least significance difference (LSD) test at 0.05 probability level.
Irrigation with WW significantly decreased Petunia plant growth attributes such as the plant height (19.39%), stem diameter (24.59%), number of branches (29.73%) and leaves (25.57%), leaf area (28.67%), and shoot dry matter (20.02%) as compared with TW, nevertheless, this inhibition was mitigated by magnetic treatment of WW that increased all growth parameters relative to WW (Table 3, Fig. 2A).
Figure 2: Morphological responses of P. x alkinsiana plants to (A) irrigation with different water types and (B) addition of different soil amendments
Soil amendment with either PM or FS and their interaction significantly increased plant growth parameters of Petunia plants. Common FS supplementation considerably improved P. x alkinsiana plant growth as compared to PM addition or cultivating plants in sandy soil. The greatest values of growth parameters were recorded under the PM + FS treatment with increased plant height, stem diameter, branches and leaves/plant, leaf area and shoots dry matter by 22.92%, 44.20%, 54.95%, 6.61%, 17.76%, and 10.59% respectively over non-treated soil (Table 3, Fig. 2B).
As for the interaction treatments the addition of either PM and/or FS with any irrigation water types significantly (P ≤ 0.05) increased plant growth attributes relative non-amended sandy soil in each irrigation water treatment (Table 3). The greatest values of plant growth attributes was recorded under soil supplementation with PM + FS and irrigated with TW. In addition, the data showed that soil supplementation with PM and/or FS mitigate the harmful effects of WW on plant growth.
3.2 Flowering and Survival Petunia Plants
Irrigation with MW and WW significantly decreased the number of flowers per plant, flower dry matter, and survival% of Petunia plants relative to TW irrigation. Conversely, the application of MW had a lesser declines than those irrigated with WW (Fig. 3). In addition, the results revealed that irrigation with TW resulted in a delay in flowering (55.56 days) relative to irrigation with MW (47.02 days) and WW (41.82 days).
Figure 3: Effects of irrigation water type and different soil amendments on A) days of flowering of P. x alkinsiana, B) number of flowers per plant, C) flower dry matter (FDM), and D) survival percentage. TW: tap water, MW: magnetized salinized well water, and WW: salinized well water. bars represent the mean values of three independent experiments (two plants per replicate) with standard errors. Means followed by different letters are significantly different according to Duncan’s multiple range test at the 5% level
Soil amendment with PM and/or FS significantly increased the number of days to flowering, the number of flower/plant, flower dry matter, and survival%, compared to non-amended sandy soil. The greatest values were obtained by adding PM + FS relative to other treatments or sandy soil.
Regarding the interaction effect, the data indicate that in general, soil amendment with either PM and/or FS under all irrigation water types significantly increased flowering attributes and survival% relative to non-amended sandy soil under such irrigation types. The most effective treatment in this regard was PM + FS (Fig. 3).
Table 4 shows that ion P+, K+, Ca2+, Mg2+, and Fe2+ significantly decreased with WW treatment, meanwhile, the application of MW mitigates the drastic impact of WW on ion content relative to TW treatment. Meanwhile, irrigation with WW or MW non-significantly decreased N% in plant shoot relative to TW treatment. Alternatively, the highest ion content (N, P+, K+, Ca2+, Mg2+, and Fe2+) in the shoot was recorded with TW treatment. Regarding soil amendment treatment, the data in Table 4 show that in general the addition of PM or FS or PM + FS had a considerable effect on ion content relative to non-amended sand soil. The highest values of N, P+, K+, Ca2+, Mg2+, and Fe2+ concentration was recorded in plants amended with PM + FS as compared with application of each one alone or non-amended sandy soil. Regarding the interaction effect between irrigation water and soil amendment, the data showed that application of and soil amendment under irrigation water treatment increased N, P+, K+, Ca2+, Mg2+, and Fe2+ concentration above non-amended sandy soil. Irrigation with TW and PM + FS amendments gave the maximum N+, K+, Ca2+, Mg2+, and Fe2+concentration among other treatments. The application of PM + FS under TW or MW non-significantly changed the potassium concentration in plant tissue (Table 4).
Using WW gave the highest sodium and chloride concentration compared to using TW or MW (Table 4). Application of soil amendments in general significantly decreased Na+% and Cl−% relative to non-amended sand soil. The lowest Na+% and Cl−% was recorded under PM + FS treatment (Table 4). The data in the same Table also indicate that the application of PM and/or FS under all irrigation water types considerably decreased Na+% or Cl−% concentration relative to non-amended sandy soil. The lowest Na+% and Cl−% were obtained with the application of FS + PM under TW treatment.
Shortage and poor quality of water is the most important limiting factor for plant growth. Normally, saline water irrigation negatively affects the several plant species development under open or controlled conditions [5,27]. The injurious effects of salt stress on crop growth may result from osmotic stress, ionic toxicity and imbalance, vital nutrient deficiency, biomembrane dysfunction, and oxidative stress [4,6]. Furthermore, ionic toxicity interrupts enzymes, bursts the biomembrane, and consequently lessens the core metabolic processes [28]. Salinity stress decreases endogenous plant growth regulators assimilation that contributes to cell differentiation leading to a decline in cell turgor. It possibly resulted from the blocking up of tracheids and vessels units; blocking the translocation of nutritional solution [29]. However, irrigation with magnetized well water alleviated the harmful effects of saline water, as evidenced by the higher values of vegetative growth parameters (Table 3). These results may be attributed to the improved water properties resulting from magnetization [30]. Raising the plant’s ability to tolerate a stressful environment is a demanding assignment for plant physiologists. Soil amendment and magnetized irrigation water have provided a boosting effect on Petunia plant development, and it presents a massive prospective in mitigating salinity-induced injuries.
Magnetic treatment of irrigation water is an acknowledged technique for achieving high water use efficiencies due to its effect on some physical and chemical properties of water and soil. These changes result in an increased ability of soil to get rid of salts and consequently better assimilation of nutrients and fertilizers in plants during the vegetative period. It was noticed that anti-salinity substances application increases root length exposed to salinity stress leading to enhanced plant ability in elements aspiration [30]. The stimulatory impact of MW on plant development could result from its effect on key cellular metabolic processes that play an imperative function in cellular biochemical pathways and enzyme activities responsible for plant growth and development [30]. The mechanism of MW in improving plant growth may be associated with: (1) the activation of growth regulators and enzymatic systems [31]; (2) acceleration of cell division and differentiation by activating phenylalanine ammonia-lyase enzyme as well as nucleic acid biosynthesis [18]; (3) increase in cell membrane permeability to ions and water, resulting in the maintenance of the required water status needed for cell turgor and division and metabolic pathway activation [8]; and (4) improvement of the physic-chemical properties of the soil that increased the nutrient and water uptake [32]. Furthermore, Hasan et al. [8] revealed that photosynthetic pigment increased significantly with MW by boosting cytokinin assimilation. They also proved that cytokinin has a vital responsibility on shoot formation and induction of several genes implicated in chloroplast differentiation and nutrient metabolism.
Regarding the irrigation water type, the addition of different soil amendments enhanced the vegetative growth of P. x alkinsiana plants as compared to the control treatment (Table 3). Among all the soil amendment treatments, the combination of Fe2SO4 and peat moss produced the best vegetative growth (Fig. 3). Between Fe2SO4 and peat moss alone, the former produced more vegetative growth in P. x alkinsiana. Soil amendments enhance soil chemical, physical, and even biological characteristics and may provide essential elements required by plants [33]. The addition of Fe2SO4 to soil may reduce SAR by increasing CaCO3 solubility, which in turn replaces Na+ ions [20].
The encouraging impacts of soil amendment on crop development may be due to the increase in K+ absorption, enhances chloroplast number/cell, and leaf area [34]. Presently, the useful effects of soil amendment are linked with the decreased Na+ and increased K+, improving the K+/Na+ ratio, which ultimately recovers Petunia plant growth under all irrigation types. The enhancement of the vegetative growth with PM could be due to their constructive functions in improving soil properties and nutrient availability [34]. Otherwise, Abd Elrahman et al. [35] established that the application of FS boosts the solubility of calcium that will substitute Na+ and diminish Na+ exchanged percentage. The encouraging effects of Fe on plants were possibly owing to their vital role as a co-factor of several enzymes or regulatory for other metabolic pathways [36].
Irrigation with saline water considerably reduced flowering capacity and survival%. This drastic effect may be due to ionic and hormonal imbalance as well as osmotic disorders that may adversely affect plant growth and flowering [10]. Despite the advantages of magnetic treatments in growth attributes, chemical composition, and boosting of flowering attributes, the mechanism of magnetic treatment on plants is still unknown. However, numerous theories had been anticipated to clarify this achievement. Water magnetization significantly enhanced the flowering productivity of P. x alkinsiana. Specifically, irrigation with magnetized or saline water produced P. x alkinsiana plants that flowered earlier than the control plants (Fig. 2A), which may be an avoidance strategy to cope with salinity stress, which is supported by the gradual delay in flowering in plants cultivated in soils supplemented with soil amendments, either individually or in combination. On the other hand, the application of MW generally enhanced cell membrane permeability that induces the higher uptake of ions and water which could stimulate growth and flowering and improve survival rates [8].
Salinity stress disrupted ion homeostasis, increasing the poisonous elements in plants [5,37]. Salt stress increased sodium and chloride accumulation, which is connected with reduced potassium, nitrogen, phosphorus, calcium, and magnesium. Earlier studies show that with salt stress, sodium and chloride increased with a decline in potassium [5,6]. This decline is coupled with an antagonistic phenomenon as sodium uptake by root via non-selective potassium channels and high-affinity potassium transporters are attributable to the physicochemical similarity between sodium and potassium [38]. Moreover, the reduced contents of various nutrients in plants could be a result of root growth inhibition in saline soils [39]. Researchers studied the effects of magnetic water on minerals in the soil and concluded that the concentration of nitrogen, potassium, phosphorus and magnesium + calcium in the soil irrigated with magnetic water is different from that in the soil irrigated with conventional water. They stated that magnetic water by accelerating the processes of crystallization and sedimentation of nutrient dissolved in the soil, reduces the movement of the mineral toward down and as a result greater amount of these elements are adsorbed by plants [38,39].
It was noticeable from the results that the uptake and accumulation of macronutrients were, usually, superior in plants irrigated with MW contrasted to non-magnetized salinized ones. Consistent with [40] irrigation with magnetically treated water accelerates the microbial mass of soils, such as N-fixing bacteria. Such increases will possibly advance the accessibility of nutrients in the soil and therefore, their uptake by plants. Besides, the increase in the accessibility of soil nutrients might be due to soil acidification that resulted from the increase in the released root exudates (organic acids) in the rhizosphere by plants irrigated with MW [31]. The acquired outcomes conform to those of [41], who establish that MW caused a raise in N, P, K, Ca, and Mg in Valencia orange leaves. Noran et al. [42] monitored the differences in N, P, K, Na, Ca and Mg content in soils irrigated with MW relative to normal water. They disputed that MW had more accessible nutrients from soil solution to be absorbed by plants. It was detected that MW irrigation increased all ion concentrations, except for sodium. This was because Na is a paramagnetic ion that has a tiny, positive susceptibility to magnetic fields [43], whereas other ions are diamagnetic that are faintly repelled by a magnetic field [43]. In the same manner, the difference provoked by magnetic fields in ionic currents affected the cell membrane leading to the alteration in osmotic pressure and a significant increase in the rate of water absorption [8]. PM increases the soil’s organic matter content and CEC, leading to enhanced water-holding capacity and increased ion accessibility [10,44].
In conclusion, for improving Petunia plant growth and flowering in the regions irrigated with tap water, we must add peat moss plus ferrous sulfate as soil amendment. Additionally, the present findings have shown the possibility of using magnetized salinized well waters as an eco-friendly, modern technology to enhance Petunia growth and flowering, constantly utilize substandard water, and boost the efficient exploitation of arable land utilizing the accessible water spring in arid and semi-arid regions. We can use the saline well water for irrigation after treating it with a magnetic field, as well as soil supplemented with peat moss and ferrous sulfate.
Authorship: Study conception and design: FAAM, KME; data collection: AMA, KME; analysis and interpretation of results: KME; draft manuscript preparation: AMA, KME; writing–review and editing: KME, FAAM. All authors reviewed the results and approved the final version of the manuscript.
Acknowledgement: We gratefully acknowledge financial support from Abdulaziz City for Science and Technology, Saudi Arabia (Grant Research No. 1-17-04-001-0021).
Funding Statement: This research was funded by Abdulaziz City for Science and Technology, Saudi Arabia (Grant Research No. 1-17-04-001-0021).
Conflicts of Interest: The authors declare that they have no conflicts of interest to report regarding the present study.
References
1. Gabr, S. S., Farg, E. F., Habeebullah, T. M., Arafat, S. M. (2020). Irrigation water consumption and its impact on the groundwater aquifer of wadi uranah, makkah, Saudi Arabia using remote sensing techniques. The Egyptian Journal of Remote Sensing and Space Science, 23(2), 167–180. DOI 10.1016/j.ejrs.2018.10.001. [Google Scholar] [CrossRef]
2. Ayers, R., Westcot, D. (1985). Water quality for agriculture. FAO irrigation and drainage paper 29 review, vol. 1. Food and Agricultural Organization, Rome. [Google Scholar]
3. Farouk, S., Arafa, S. A. (2018). Mitigation of salinity stress in canola plants by sodium nitroprusside application. Spanish Journal of Agricultural Research, 16(3), e0802. DOI 10.5424/sjar/2018163-13252. [Google Scholar] [CrossRef]
4. Farouk, S., Elhindi, K. M., Alotaibi, M. A. (2020). Silicon supplementation mitigates salinity stress on Ocimum basilicum L. via improving water balance, ion homeostasis, and antioxidant defense system. Ecotoxicology and Environmental Safety, 206, 111396. DOI 10.1016/j.ecoenv.2020.111396. [Google Scholar] [CrossRef]
5. Sofy, M. R., Elhindi, K. M., Farouk, S., Alotaibi, M. A. (2020). Zinc and paclobutrazol mediated regulation of growth, upregulating antioxidant aptitude and plant productivity of pea plants under salinity. Plants, 9(9), 1197. DOI 10.3390/plants9091197. [Google Scholar] [CrossRef]
6. Farouk, S., Al-Amri, S. M. (2019). Exogenous zinc forms counteract NaCl-induced damage by regulating the antioxidant system, osmotic adjustment substances, and ions in canola (brassica napus L. cv. pactol) plants. Journal of Soil Science and Plant Nutrition, 19(1), 887–899. DOI 10.1007/s42729-019-00087-y. [Google Scholar] [CrossRef]
7. Penella, C., Calatayud, Á., Melgar, J. C. (2017). Ascorbic acid alleviates water stress in young peach trees and improves their performance after rewatering. Frontiers in Plant Science, 8, 1627. DOI 10.3389/fpls.2017.01627. [Google Scholar] [CrossRef]
8. Hasan, M. M., Alharby, H. F., Hajar, A. S., Hakeem, K. R., Alzahrani, Y. (2019). The effect of magnetized water on the growth and physiological conditions of moringa species under drought stress. Polish Journal of Environmental Studies, 28(3), 1145–1155. DOI 10.15244/pjoes/85879. [Google Scholar] [CrossRef]
9. Casterad, M. A., Herrero, J., Betrán, J. A., Ritchie, G. (2018). Sensor-based assessment of soil salinity during the first years of transition from flood to sprinkler irrigation. Sensors, 18(2), 616. DOI 10.3390/s18020616. [Google Scholar] [CrossRef]
10. Elhindi, K. M., Al-Mana, F. A., Algahtani, A. M., Alotaibi, M. A. (2020). Effect of irrigation with saline magnetized water and different soil amendments on growth and flower production of Calendula officinalis L. plants. Saudi Journal of Biological Sciences, 27(11), 3072–3078. DOI 10.1016/j.sjbs.2020.09.015. [Google Scholar] [CrossRef]
11. Babu, C. (2010). Use of magnetic water and polymer in agriculture. Tropical Research, 08-806-001. [Google Scholar]
12. Ghauria, S. A., Ansari, M. S. (2006). Increase of water viscosity under the influence of magnetic field. Journal of Applied Physics, 100(6), 066101. DOI 10.1063/1.2347702. [Google Scholar] [CrossRef]
13. Pang, X. F., Shen, G. F. (2013). The changes of physical properties of water arising from the magnetic field and its mechanism. Modern Physics Letters B, 27(31), 1350228. DOI 10.1142/S021798491350228X. [Google Scholar] [CrossRef]
14. Wang, Y., Zhang, B., Gong, Z., Gao, K., Ou, Y. et al. (2013). The effect of a static magnetic field on the hydrogen bonding in water using frictional experiments. Journal of Molecular Structure, 1052, 102–104. DOI 10.1016/j.molstruc.2013.08.021. [Google Scholar] [CrossRef]
15. Ali, Y., Samaneh, R., Zohre, R., Mostafa, J. (2014). Magnetic water treatment in environmental management: A review of the recent advances and future perspectives. Current World Environment, 9(3), 1008–1016. DOI 10.12944/CWE.9.3.56. [Google Scholar] [CrossRef]
16. Gabrielli, C., Jaouhari, R., Maurin, G., Keddam, M. (2001). Magnetic water treatment for scale prevention. Water Research, 35(13), 3249–3259. DOI 10.1016/S0043-1354(01)00010-0. [Google Scholar] [CrossRef]
17. Abedinpour, M., Rohani, E. (2017). Effects of magnetized water application on soil and maize growth indices under different amounts of salt in the water. Journal of Water Reuse and Desalination, 7(3), 319–325. DOI 10.2166/wrd.2016.216. [Google Scholar] [CrossRef]
18. Abdul-Qados, A., Hozayn, M. (2010). Response of growth, yield, yield components and some chemical constituents of flax for irrigation with magnetized and tap water. World Applied Sciences Journal, 8, 630–634. [Google Scholar]
19. Massah, J., Dousti, A., Khazaei, J., Vaezzadeh, M. (2019). Effects of water magnetic treatment on seed germination and seedling growth of wheat. Journal of Plant Nutrition, 42(11–12), 1283–1289. DOI 10.1080/01904167.2019.1617309. [Google Scholar] [CrossRef]
20. Rout, G. R., Sahoo, S. (2015). Role of iron in plant growth and metabolism. Reviews in Agricultural Science, 3, 1–24. DOI 10.7831/ras.3.1. [Google Scholar] [CrossRef]
21. Cakmak, I. (2002). Plant nutrition research: Priorities to meet human needs for food in sustainable ways. Plant and Soil, 247, 3–24. DOI 10.1007/978-94-017-2789-1_1. [Google Scholar] [CrossRef]
22. El-Jendoubi, H., Vázquez, S., Calatayud, Á., Vavpetič, P., Vogel-Mikuš, K. et al. (2014). The effects of foliar fertilization with iron sulfate in chlorotic leaves are limited to the treated area. A study with peach trees (Prunus persica L. batsch) grown in the field and sugar beet (Beta vulgaris L.) grown in hydroponics. Front Plant Sciences, 5. DOI 10.3389/fpls.2014.00002. [Google Scholar] [CrossRef]
23. Stehmann, J. R., Lorenz-Lemke, A. P., Freitas, L. B., Semir, J. (2009). The genus petunia. In: Gerats, T., Strommer, J. (Eds.Petunia: Evolutionary, developmental and physiological genetics, pp. 1–28. New York, USA: Springer. DOI 10.1007/978-0-387-84796-2. [Google Scholar] [CrossRef]
24. Aruna, M., Radhakrishnanb, R., Aia, T. N., Nainga, A. H., Leed, I. J. et al. (2016). Nitrogenous compounds enhance the growth of petunia and reprogram biochemical changes against the adverse effect of salinity. The Journal of Horticultural Science and Biotechnology, 91(6), 562–572. DOI 10.1080/14620316.2016.1192961. [Google Scholar] [CrossRef]
25. Page, A. L., Keeney, D. (1982). Methods of soil analysis. Madison: American Society of Agronomy. [Google Scholar]
26. Association of Official Analytical Chemists (1990). Officinal methods of analysis, pp. 62–63. Arlington, Virginia, USA: Pub. A.O.A.C. Inc. DOI 10.1021/jf034268w. [Google Scholar] [CrossRef]
27. Farooq, H., Bashir, M. A., Khalofah, A., Khan, K. A., Ramzan, M. et al. (2021). Interactive effects of saline water irrigation and nitrogen fertilization on tomato growth and yield. Fresenius Environmental Bulletin, 3(4), 3557–3564. [Google Scholar]
28. Ferrante, A., Trivellini, A., Malorgio, F., Carmassi, G., Vernieri, P. et al. (2011). Effect of seawater aerosol on leaves of six plant species potentially useful for ornamental purposes in coastal areas. Scientia Horticulturae, 128(3), 332–341, DOI 10.1016/j.scienta.2011.01.008. [Google Scholar] [CrossRef]
29. Munns, R. (2002). Comparative physiology of salt and water stress. Plant, Cell & Environment, 25(2), 239–250, DOI 10.1046/j.0016-8025.2001.00808.x. [Google Scholar] [CrossRef]
30. Nasher, S. H. (2008). The effect of magnetic water on growth of chick-pea seeds. Engineering & Technology, 26, 4. [Google Scholar]
31. Maheshwari, B. L., Grewal, H. S. (2009). Magnetic treatment of irrigation water: Its effects on vegetable crop yield and water productivity. Agricultural Water Management, 96(8), 1229–1236. DOI 10.1016/j.agwat.2009.03.016. [Google Scholar] [CrossRef]
32. Selim, M. (2008). Application of magnetic technologies in correcting underground brackish water for irrigation in the arid and semi-arid ecosystem. Proceedings of the 3rd International Conference on Water Resources and Arid Environments, pp. 1–11. Cairo, Egypt. Field Crops Research Department, National Research Centre. https://icwrae-psipw.org/papers/2008/Environment/6.pdf. [Google Scholar]
33. Talha Bin Yousaf, M., Farrakh Nawaz, M., Yasin, G., Ahmad, I., Gul, S. et al. (2022). Effect of organic amendments in soil on physiological and biochemical attributes of Vachellia nilotica and Dalbergia sissoo under saline stress. Plants, 11(2), 228. DOI 10.3390/plants11020228. [Google Scholar] [CrossRef]
34. Taiz, L., Zeiger, E. (2010). Plant physiology (5th Ed.). USA: Sinauer Associates. [Google Scholar]
35. Abd Elrahman, S. H., Mostafa, M., Taha, T., Elsharawy, M., Eid, M. (2012). Effect of different amendments on soil chemical characteristics, grain yield and elemental content of wheat plants grown on salt-affected soil irrigated with low quality water. Annals of Agricultural Sciences, 57(2), 175–182. DOI 10.1016/j.aoas.2012.09.001. [Google Scholar] [CrossRef]
36. Abd El-Hady, B. (2007). Effect of zinc application on growth and nutrient uptake of barley plant irrigated with saline water. Research Journal of Applied Sciences, 3, 431–436. [Google Scholar]
37. Amin, I., Rasool, S., Mir, M. A., Wani, W., Masoodi, K. Z. et al. (2021). Ion homeostasis for salinity tolerance in plants: A molecular approach. Physiologia Plantarum, 174(4), 578–594. DOI 10.1111/ppl.13185. [Google Scholar] [CrossRef]
38. de Souza Miranda, R., Mesquita, R. O., Costa, J. H., Alvarez-Pizarro, J. C., Prisco, J. T. et al. (2017). Integrative control between proton pumps and SOS1 antiporters in roots is crucial for maintaining low Na+ accumulation and salt tolerance in ammonium-supplied Sorghum bicolor. Plant Cell Physiology, 58(3), 522–536. DOI 10.1093/pcp/pcw231. [Google Scholar] [CrossRef]
39. Flowers, T. J., Colmer, T. D. (2008). Salinity tolerance in halophytes. New Phytologist, 179(4), 945–963, DOI 10.1111/J.1469-8137.2008.02531.X. [Google Scholar] [CrossRef]
40. Liu, X., Zhu, H., Wang, L., Bi, S., Zhang, Z. et al. (2019). The effects of magnetic treatment on nitrogen absorption and distribution in seedlings of Populus × euramericana ‘Neva’ under NaCl stress. Scientific Reports, 9, 10025. DOI 10.1038/s41598-019-45719-6. [Google Scholar] [CrossRef]
41. Aly, M., Thanaa, M. E., Osman, S., Abdelhamed, A. (2015). Effect of magnetic irrigation water and some anti-salinity substances on the growth and production of valencia orange. Middle East Journal of Agriculture Research, 4, 88–98. [Google Scholar]
42. Noran, R., Shani, U., Lin, I. (1996). The effect of irrigation with magnetically treated water on the translocation of minerals in the soil. Physical Separation in Science and Engineering, 7, 046596. DOI 10.1155/1996/46596. [Google Scholar] [CrossRef]
43. Zlotopolski, V. (2017). Magnetic treatment reduces water usage in irrigation without negatively impacting yield, photosynthesis and nutrient uptake in lettuce. International Journal of Applied Agricultural Sciences, 3(5), 117–122. DOI 10.11648/j.ijaas.20170305.13. [Google Scholar] [CrossRef]
44. Eyras, M. C., Defossé, G. E., Dellatorre, F. (2008). Seaweed compost as an amendment for horticultural soils in Patagonia, Argentina. Compost Science & Utilization, 16(2), 119–124. DOI 10.1080/1065657X.2008.10702366. [Google Scholar] [CrossRef]
Cite This Article
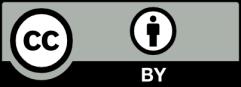
This work is licensed under a Creative Commons Attribution 4.0 International License , which permits unrestricted use, distribution, and reproduction in any medium, provided the original work is properly cited.