Open Access
ARTICLE
Evaluating the Effects of Aquaculture Wastewater Irrigation with Fertilizer Reduction on Greenhouse Tomato Production, Economic Benefits and Soil Nitrogen Characteristics
1 National Key Laboratory of Water Disaster Prevention, Hohai University, Nanjing, 210098, China
2 College of Agricultural Science and Engineering, Hohai University, Nanjing, 210098, China
3 Jiangsu Province Engineering Research Center for Agricultural Soil-Water Efficient Utilization Carbon Sequestration and Emission Reduction, Hohai University, Nanjing, 210098, China
4 Agricultural Technology Extension Station of Ningbo, Ningbo, China
* Corresponding Author: Junzeng Xu. Email:
Phyton-International Journal of Experimental Botany 2023, 92(12), 3291-3304. https://doi.org/10.32604/phyton.2023.044051
Received 19 July 2023; Accepted 19 October 2023; Issue published 28 December 2023
Abstract
The utilization of aquaculture wastewater as irrigation is an effective way to recycle and reuse water and nitrogen fertilizer resources because it contains numerous nutrients. However, it is still unclear that the pattern of substituting aquaculture wastewater irrigation for fertilizer supplementing is conducive to improving the soil nitrogen status, fruit yield and water-fertilizer use efficiency for tomato production. In this context, the experiment was intended to establish the appropriate irrigation regime of aquaculture wastewater in tomato production for freshwater replacement and fertilizer reduction to ensure good yields. Pot experiments were conducted with treatments as farmers accustomed to irrigation and fertilization used as control (CK), 1.75 L aquaculture wastewater with base fertilizer (W1), 2 L aquaculture wastewater with base fertilizer; and 2.25 L aquaculture wastewater with base fertilizer (W3). We examined the effects of aquaculture wastewater irrigation on soil nitrogen distribution, N-related hydrolases, tomato yield, and economic benefits. The results showed that the control treatment had the highest N input, about 24.68% higher than the W3 treatment, while the yield was only about 7.81% higher than W3. This indicated that the overuse of chemical fertilizer was present in the current tomato production. Although the reduction of fertilizer in aquaculture wastewater irrigation caused a decrease in tomato production, this economic loss can be compensated by cost savings in the wastewater disposal. Among aquaculture wastewater treatments, the W3 treatment had the highest overall benefit, achieving 62.63% freshwater savings, 37.50% fertilizer input reduction, and an economic return of approximately 19,466 Yuan per hectare higher than the control. Additionally, increasing the irrigation volume of aquaculture wastewater could provide more available nutrients to the soil, which were more prevalent in the form of organic nitrogen. The lower soil nitrate reductase activities (NR) under aquaculture wastewater treatments after harvesting also proved that this pattern was beneficial to reduce soil nitrate nitrogen residues. Overall, the results demonstrate that aquaculture wastewater irrigation alleviates the soil nitrate residues, improves nutrient availability, and results in more economic returns with water and fertilizer conservation for the greenhouse production of tomatoes.
Keywords
Nomenclature
IWUE | Irrigation water use efficiency |
NFP | Nitrogen partial productivity |
SNR | Soil nitrogen residue amount |
NR | Nitrate reductase |
AN | Soil available nitrogen |
Tomato (Solanum lycopersicum L.), a crop widely grown around the world, is one of the most important vegetables in the global human diet due to its high nutritional value for human health [1–4]. With over one million hectares under cultivation and an annual production of approximately 56 million tons, China is a world leader in tomato production [1]. Open fields and greenhouse environments are the usual choices for tomato cultivation [5]. Because of its ability to withstand climate change and its potential for stable production during the off-season, the solar greenhouse has come to dominate vegetable cultivation in China [6,7]. The Yellow River Basin, for example, the largest greenhouse tomato planting region in China, is vital to the supply of fresh tomatoes [8].
In greenhouse-based production systems, irrigation is considered the only way to provide water to plants [9]. Although different irrigation management strategies are currently employed in the production of greenhouse tomatoes, the majority of practices still rely on the experience of individual farmers [10,11], which can lead to significant soil evaporation and loss to the deep soil with lower crop utilization [12]. Meanwhile, nitrogen is essential to the development of tomatoes, because many physiological metabolic processes and crop conformational structures are related to nitrogen nutrients [13–15]. Over-fertilization has become a common practice in tomato production today in the pursuit of maximum yield, considering the impact of nitrogen availability on tomatoes [1,16]. However, we should be conscious of the resource and environmental problems caused by inappropriate water and fertilizer applications, such as low use efficiency of irrigation water [16], soil nitrate accumulation or leaching, groundwater pollution, and greenhouse gas emissions [17–20]. Consequently, the relationship between crop production and the environment cannot be disregarded for the sustainability of agricultural development.
Recent increases in worldwide population have led to growing fish consumption, which has aided in the ongoing growth and expansion of aquaculture activities [21]. However, in aquaculture practices, an excessive buildup of metabolic waste products and bait remnants from fish can result in an increase in nitrogen concentrations in water, which in turn poses a threat to the aquatic environment [22,23]. Boyd et al. also demonstrated that only 15%–30% of the nitrogen added to the pond was absorbed by the fish or shrimp, while up to 80% of the input nitrogen remained in the water [24,25]. Because of this, aquaculture frequently imports enormous amounts of freshwater to maintain appropriate nitrogen concentrations in the water column [26], but this also generates a massive wastewater disposal task. It is also obvious that depending solely on wastewater disposal systems to solve the issue is impractical because of the high cost of it [27].
Due to the distribution of freshwater’s regional and temporal complexity, water shortages have come to be accepted as a worldwide issue [28]. Unquestionably, it poses a serious threat to food security, which is primarily reliant on freshwater supplies [16]. Therefore, using aquaculture wastewater as a substitute for freshwater in agricultural systems would not only ease the strain on freshwater sustainability but also utilize the nutrients rich in the wastewater [29,30]. This is an effective strategy for recycling resources and avoiding the high expense of wastewater disposal. Undoubtedly, this is also a novel approach to the issue of inadequate water and fertilizer management in current agricultural production. Many scholars have demonstrated that the use of wastewater, which contains many nutrients, for agricultural irrigation can effectively utilize both water and nitrogen. McIntosh et al. have suggested that 20%–31% of the nitrogen needed for wheat production might be provided by aquaculture effluent [31]. Xie et al. found that irrigation of paddy rice using aquaculture effluent may make full use of water and nitrogen, which had practical relevance for easing water-use disputes in the coastal areas of Jiangsu Province and fostering soil desalinization [32]. According to the study of Kolozsvári et al., the utilization of effluent water from an intensive African catfish farm for irrigation boosted the soil’s nitrogen supply and may have a positive impact on soil quality [33]. Qi et al. summarized that aquaculture effluent irrigation might reduce the amount of fertilizer used by 20% and save 5700 m3 ha−1 of freshwater during the rice growing season [34].
Nevertheless, the influence of wastewater irrigation on soil is highly dependent on the source of water, soil type and crops to be grown [35]. It is still necessary to investigate how aquaculture wastewater irrigation affects crop development and soil characteristics. Thus, our objectives were to determine the following: (1) if substituting aquaculture wastewater irrigation for fertilizer supplementing would produce tomato yield equivalent to the farmer accustomed to irrigation and fertilization (2) if this pattern could achieve a win-win situation for tomato production and aquaculture, i.e., high economic returns with water and fertilizer conservation and (3) how the soil nitrogen pool responds to this pattern. The results of this study would provide a reference for economical water and fertilizer management strategies in greenhouse tomato production.
The experiment was carried out in a greenhouse of Hohai University, Nanjing, China (32°06′ N, 118°76′ E). This area is characterized by a humid subtropical climate and is under the influence of the East Asia Monsoon, with an average annual rainfall of 1069.3 mm. The annual mean temperature is 15.6°C and annual sunshine hours are 2017.5 h. The tested soil, taken from the Institute of Vegetable and Flower Science in Nanjing, was loam with a pH of 7.35, organic matter of 14.572 g kg−1, total nitrogen of 0.936 g kg−1, total phosphorus of 0.367 g kg−1, soil alkaline hydrolysis nitrogen of 131.3 mg kg−1 and available phosphorus of 27.2 mg kg−1.
The experiment was conducted in plastic buckets with a height of 36.5 cm and a diameter of 29 cm. In the bucket, we stratified at a height of 5 cm thick and filled the top and bottom three layers with air-dried soil with a capacity of 1.25 and 1.3 g cm−3, respectively. There were four different treatments implemented: (I) 2 L of freshwater irrigation with fertilizer supplements (CK, the control treatment, referred to the farmer accustomed to irrigation and fertilization); (II) 1.75 L of irrigation with aquaculture wastewater without fertilizer supplements; (III) 2 L of irrigation with aquaculture wastewater without fertilizer supplements; and (IV) 2.25 L of irrigation with aquaculture wastewater without fertilizer supplements. Each treatment was repeated three times for a total of 40 test buckets. The tested tomatoes were Jinpeng No. 8, and the seedlings were transplanted on July 13th. Before transplanting, we irrigated the treatments with 4.74 L of freshwater to moisten the soil sufficiently. The seedlings were planted at a depth of 10 cm from the soil surface. Irrigation was accomplished by manual watering in all treatments. During the tomato seedling stage (from July 13th to August 13th), equal amounts of freshwater were irrigated in each treatment based on soil moisture conditions. It should be noted that we started the irrigation schemes with aquaculture wastewater after the seedling stage to ensure the survival of tomato seedlings, with a frequency of about 7 days.
In this experiment, 7.92 g (equivalent to 180 kg ha−1 of N) of compound fertilizer containing 15% N, 15% P2O5, and 15% K2O was applied as a base fertilizer to the 0–15 cm soil layer for each pot before transplantation. In the CK treatment, after dissolving the fertilizer in water, 1.584 g (equivalent to 36 kg ha−1 of N) of compound fertilizer was applied to each pot as a supplemental fertilizer on September 26th, October 4th, and October 14th, respectively. Equal doses of magnesium fertilizer (99% MgSO4, 49.3 kg ha−1) and calciferous fertilizer (25% CaO (chelated calcium), 35 kg ha−1) were applied to each treatment for meeting the needs of tomato growth. Freshwater and aquaculture wastewater for irrigation were taken from residential tap water and a suburban carp farm, respectively. The water qualities for irrigation are shown in Table 1. In particular, it should be noted that during tomato growth, the nitrogen content of aquaculture wastewater fluctuated. Accordingly, we calculated the nitrogen introduced by irrigation water as the product of the total irrigation volume and the highest value of nitrogen in the wastewater during the experiment.
Soil samples were collected at day 45 (August 27th), 60 d (September 11th), day 75 (September 26th), day 90 (October 11th), and day 105 (October 26th) of tomato growth, using a stainless auger. In each pot, soil samples were taken at depths of 0–5 cm, 5–10 cm, and 10–20 cm. To create a composite sample for further analysis, the rocks and remaining plant root fragments were taken out and the samples were sieved (2 mm). The stem diameter of each plant was determined by measuring the circumference with a soft ruler and plant height was measured with a 100 cm stainless steel ruler. After harvest, fruit yields were measured using an electronic platform scale.
Through the extraction with 1M KCl, the concentrations of NH4+-N and NO3−-N in the soils were analyzed by a continuous flow injection analyzer (SKALAR San++ FIA). Inorganic nitrogen content was the sum of NH4+-N and NO3−-N in the soils. The content of soil available nitrogen (AN) was determined using the method described by Roberts et al. [36]. The soil nitrogen residue amount was calculated according to the following formula:
where SNR is soil nitrogen residue amount, kg ha−1; C is the soil nitrogen concentration in each soil layer, mg kg−1; hi is the soil layer depth, cm; γi is the soil bulk density of each layer, g cm−3.
Soil urease activity was measured using the phenol-sodium hypochlorite colorimetry and nitrate reductase activity (NR) was determined with α-naphthylamine-sulfanilic. The irrigation water use efficiency (IWUE) and nitrogen partial productivity (NFP) were calculated according to the following formula:
where
where
To assess the net return of aquaculture wastewater irrigation in greenhouse tomato production, we calculated firstly the saving cost of nitrogen purification using agricultural irrigation for each treatment, which is worked out according to the following formula:
where
The benefit of yield and the cost of fertilizer applied in tomato production were calculated according to the following formula:
where 4 is the average selling price of tomatoes (Yuan kg−1) and 3.5 is the cost of compound fertilizer (Yuan kg−1) [38]. The net return was calculated by using the following formula:
where the labor cost was converted based on Wang et al. [38].
One-way analysis of variance (ANOVA) and Duncan test (p = 0.05) were used to assess the effects of different treatments in soil nitrogen, urease, nitrate reductase activity, and indicators of plant growth by SPSS 25.0 software (IBM Inc., Chicago, IL, USA). Origin 2023 software was used for mapping (Origin Lab Corporation, Northampton, MA, USA).
3.1 Applied Water and Nitrogen
A total of 14 irrigations were applied during the entire growth period of tomatoes (Table 2). To ensure the soil had enough moisture before transplanting, 4.74 L of fresh water in each pot was irrigated until the water stopped seeping down. During the tomato seedling stage, we used freshwater irrigation and maintained the irrigation amount consistently among treatments to ensure that tomato seedlings rose equally. After August 14th, we implemented the irrigation strategy according to the pot experiment designs. At the end of the experiment, a total of 28.74 L of freshwater was irrigated in the CK treatment, and 26.49 L (W1), 28.74 L (W2), and 30.99 L (W3) of wastewater were irrigated in the aquaculture wastewater treatments, respectively. On this basis, we calculated the total N fertilizer input during the experiment for each treatment (Table 3). All treatments were uniformly fertilized with a base fertilizer of 180 kg N ha−1 before transplanting. After entering flowering, we supplemented the nitrogen for the wastewater irrigation treatments with aquaculture wastewater instead of chemical fertilizers, while for the control, we applied three additional fertilizers at the flowering and fruit expansion stages, respectively. Finally, in the CK treatment, the total amount of N fertilization was 288 kg ha−1, while in the aquaculture wastewater treatments, the amount of N application was 223 (W1) kg ha−1, 227 (W2) kg ha−1, and 231 (W3) kg ha−1, respectively.
3.2 Tomato Growth, IWUE, and NFP
The height of tomato plants ranged from 131.7 to 140.40 cm at the end of the experiment. The plant height of tomatoes under irrigation with aquaculture wastewater was lower than that of CK, but no significant differences were seen between treatments. The stem diameter of tomato plants ranged from 12.30 to 13.54 mm under each treatment. When comparing CK and W2, it can be found that irrigation with wastewater can significantly increase the stem diameter under the same amount of irrigation (p < 0.05). By measuring the yield of fresh tomato fruits, the treatments showed: CK (51.74 t ha−1) > W3 (47.99 t ha−1) > W2 (43.60 t ha−1) > W1 (40.50 t ha−1). The average yield of the CK treatment was, respectively, 27.75%, 18.67%, and 7.81% greater than the yields of the W1, W2, and W3 treatments. The tomato yield increased with higher irrigation of wastewater, with W3 treatment yielding about 18.49% and 10.07% higher than W1 and W2 treatments, respectively.
Meanwhile, we estimated IWUE and NFP based on the irrigation and fertilization schemes (Table 4). In the aquaculture wastewater treatments, the IWUE was close to 39 kg m−3, about 15.73% lower than the control, which was related to the higher yield in the CK. Although there were no significant differences in IWUE and NFP among the treatments (p > 0.05), we could still see an increasing trend in NFP with increasing irrigation amount for the aquaculture wastewater treatments. In the W3 treatment, NFP was about 15.65% higher than in the control treatment.
Based on the data on tomato yield and total water and fertilizer inputs, we further investigated the benefits of using aquaculture wastewater for irrigation in terms of water and fertilizer conservation, stabilization of yield, and reduction of wastewater disposal costs (Table 5). Given the higher production, CK treatment can earn 206,963 Yuan ha−1 from selling tomatoes, which was 7.81%–27.74% more than aquaculture wastewater treatments. However, the use of aquaculture wastewater for agricultural irrigation can reduce the investment in nitrogen purification cost of wastewater in the aquaculture industry. In wastewater irrigation treatments, savings of 27,298 Yuan ha−1 (W1), 29,617 Yuan ha−1 (W2), and 31,935 Yuan ha−1 (W3) can be achieved by skipping this step, respectively. After deducting labor costs and fertilizer inputs, the W3 treatment had the highest overall benefit, approximately about 19,466 Yuan ha−1 higher than the control treatment.
3.4 Soil Nitrogen Characteristics
As the tomatoes grew, the available nitrogen (AN) in the soil under each treatment was absorbed by plants (Table 6). The AN content of each treatment gradually declined with the increasing soil depth. At day 45, soil AN ranged from 220.90 to 224.95 mg kg−1 (0–5 cm) and from 203.03 to 218.49 mg kg−1 (5–10 cm), respectively. There was no significant difference between treatments in the 0–5 cm and 5–10 cm soil layers (p > 0.05). In the 10–20 cm soil layer, soil AN of W2 and W3 were 170.20 and 184.13 mg kg−1, respectively, which were significantly higher than W1 and CK (p < 0.05). At day 105, in the 0–5 cm soil layer, the soil AN for CK and W3 were 163.43 and 153.15 mg kg−1, respectively, which were significantly higher than those for W1 and W2 (p < 0.05). In the 5–10 cm soil layer, the AN content of each treatment ranged from 128.6 to 161.13 mg kg−1, with the CK treatment being significantly higher (p < 0.05) than the other treatments (p < 0.05). In the 10–20 cm soil layer, there was no significant difference in soil AN between CK and W3 treatments (p > 0.05), which were 134.30 and 132.86 mg kg−1, respectively. And soil AN content of W1 and W2 were 108.14 and 120.47 mg kg−1, respectively.
Under treatments with aquaculture wastewater, the AN residue in the soil increased with the increase in irrigation amount. Due to the replenishment of soil available nutrients by aquaculture wastewater, the AN residue of each treatment at day 45 was ranked in the following order: W3 (525.66 kg ha−1) > W2 (490.84 kg ha−1) > CK (472.32 kg ha−1) > W1 (469.94 kg ha−1). At this time, the residual of soil AN in the W3 treatment was significantly higher than the other treatments (p < 0.05), while there was no significant difference between the CK, W1, and W2 treatments. At day 105, the residual soil AN for each treatment decreased by 20.80% (CK), 36.21% (W1), 33.83% (W2), and 32.95% (W3), respectively, compared to day 45. The W1, W2, and W3 treatments had AN contents of 299.78, 324.79, and 352.47 kg ha−1, respectively, which were around 19.86%, 13.18%, and 5.78% less than the CK. The highest AN residual occurred in the control treatment (374.09 kg ha−1) due to the additional fertilizer, but it was not significantly different from the W3 treatment (p > 0.05).
At day 45, soil inorganic nitrogen residues ranged from 237.30 to 239.85 kg ha−1, and no significant differences were seen among treatments (p > 0.05). Therefore, we expect that the increased soil AN in the wastewater treatments at day 45 may be less related to inorganic nitrogen because the equivalent amount of base fertilizer was given to each treatment (Fig. 1A). At day 105, inorganic nitrogen residue under the control treatment was significantly higher (p < 0.05) than that under the wastewater treatments (Table 7). Comparing the CK and W2 treatments, it can be seen that irrigation with aquaculture wastewater reduced soil inorganic N residues by 30.58% at the same irrigation volume. In addition, soil inorganic N residues were about 40.38% and 38.78% higher in CK treatment than in W1 and W3 treatments, respectively.
Figure 1: Soil available nitrogen (AN) residue in the 0–20 cm soil profile at day 45 (A) and 105 (B) of the tomato growth periods under different treatments
The main tomato root zone in this experiment was in the 0–10 cm layer of soil, which was also the soil that was fully exposed to exogenous fertilizers and aquaculture wastewater. To evaluate the impact of different irrigation and fertilization management on urease and NR activities, we collected this portion of the soil on day 105 (Fig. 2). The urease activity was 175.33, 173.18, 170.86 and 173.22 μg g−1 d−1 after harvesting under CK, W1, W2 and W3, respectively. There were no statistical differences (p > 0.05) between the four treatments in urease activity. This is indicative of the little effect on urease by aquaculture wastewater treatments. Under irrigation with aquaculture wastewater, the soil NR activities increased with the increase in irrigation amount and were significantly lower (p < 0.05) than the CK. For instance, the NR activity of CK was approximately 65.90%, 60.33%, and 47.75% higher than the W1, W2, and W3 treatments, respectively.
Figure 2: Soil urease (A) and nitrate reductase (B) activities in the 0–10 cm soil profile at day 105 under different treatments
4.1 Potential of Aquaculture Wastewater Irrigation for a Win-Win Situation in Tomato Production and Aquaculture
In addition to environmental issues [2], in some cases, an increase in yield from over-fertilization might result in a decrease in the organoleptic quality of the fruit [39,40]. Therefore, proper management of nitrogen nutrients is beneficial to crop growth and environmental sustainability [41]. According to Hernández et al., reducing nitrogen application after anthesis after flowering did not affect or even improve the nutritional quality of tomatoes [42]. Therefore, we chose to use aquaculture wastewater as a source of irrigation after the tomato flowering period as an alternative to traditional fertilizer supplements.
The actual N inputs under each treatment in Table 3 showed that the control treatment had the highest N input, about 24.68% higher than the W3 treatment, whereas the yield of the W3 treatment was only 7.25% lower than CK. We can infer that there was fertilizer wasted in the control treatment because the high fertilizer input did not get sufficiently high yields. Therefore, considering the optimization of irrigation volume to increase nutrient replenishment to the soil from aquaculture wastewater could help to conserve fresh water and fertilizer while maintaining tomato production. It was also noticed that tomato yields decreased with the utilization of aquaculture wastewater as compared to the pattern of the farmer accustomed to. However, as can be seen in Table 5 using aquaculture wastewater for irrigation can save at least 27,298 Yuan ha−1 of nitrogen purification cost, which might serve as compensation to farmers for reduced returns due to the lower yields under the joint model of aquaculture and tomato cultivation.
4.2 Potential of Aquaculture Wastewater Irrigation for Soil Health Improvement in Tomato Production
As well as trace amounts of water-soluble organic nitrogen, soil AN includes hydrolyzed organic nitrogen (amino acids, amides, and readily hydrolyzed proteins) and inorganic nitrogen [43,44]. At day 45, no significant differences in organic nitrogen residue were seen among treatments but the AN residue was higher in the treatments with aquaculture irrigation than CK (Table 6 and Fig. 1A). It was suggested that the majority of the available nutrients in aquaculture wastewater, particularly nitrogen, was maintained in the form of hydrolytic organic nitrogen. Soil organic nitrogen fractions were correlated with the soil nitrogen supply potential in greenhouse soil, which may serve as key indicators of soil fertility [45]. However, most soil organic nitrogen must be converted to mineral nitrogen before it can be absorbed by the roots of plants [45]. Therefore, irrigation with aquaculture wastewater may provide a constant source of organic nitrogen that can be mineralized, which would help to improve the soil nitrogen supply potential.
NR is one of the key enzymes involved in the nitrogen metabolism pathway, triggered at high nitrate levels [46]. This is supported by the fact that soil nitrate N residues at the end of farm wastewater treatment were significantly lower than the control in this study. Nitrate nitrogen is an important component of the soil inorganic nitrogen pool and is highly susceptible to migration with irrigation water leaching or runoff [47], which may contribute to the risk of groundwater eutrophication and soil nutrient loss [48,49]. However, because we used a pot experiment in this study, we failed to evaluate the characteristics of soil nitrogen leaching under irrigation with farm wastewater, which needs to be improved in future studies.
Furthermore, Delaide et al. [50] have claimed that the abundance of different microorganisms in the aquaculture effluent (bacteria, fungi, protozoa, etc.) and dissolved organic molecules (DOM) may be a chance for increased productivity in tomatoes. However, we should also be aware of the adverse effects of aquaculture wastewater irrigation on soil qualities and crop growth. It has been reported that different types of contaminants (e.g., metals, organic micropollutants) brought by wastewater irrigation may accumulate in soil [51,52]. These contaminants may spread to nearby areas and impede soil fertility, disrupt the soil microbial communities, or even be poisonous to plants [51]. Moreover, synergistic interactions between contaminants (e.g., accumulated metals and antibiotics) can exacerbate their potential effects [53,54]. Therefore, further research is needed to study the soil environment of farmland by long-term aquaculture wastewater irrigation.
Our findings warrant that aquaculture wastewater irrigation has a positive influence on the reduction of freshwater input and fertilizer application for tomato production. Irrigation with 2.25 L aquaculture wastewater (W3) can keep farmers from additional fertilizer during the flowering and fruit expansion periods, saving 62.63% of freshwater and 37.50% of fertilizer. Even though the tomato yield by this pattern decreased by 7.25% when compared to the CK, the 24.68% higher nitrogen use in the CK treatment did not lead to a corresponding increase in yield. This demonstrated the irrationality of fertilizer application under the pattern of farmers accustomed to irrigation and fertilization. Meanwhile, using aquaculture wastewater for irrigation can save at least 27,298 Yuan ha−1 of nitrogen purification cost, which can offset the economic loss from decreased production. Additionally, aquaculture wastewater irrigation can deliver more available nutrients for tomato growth. These nutrients are rich in organic nitrogen content, which is good for the potential of soil nitrogen supply enhancement. Therefore, the application of aquaculture wastewater irrigation with fertilizer reduction is an economic and ecological approach to the tomato production process.
Acknowledgement: We fully appreciate the editors and all anonymous reviewers for their constructive comments and suggestions to improve the manuscript.
Funding Statement: This research was supported by the Ningbo Public Welfare Science and Technology Program (No. 2022S097) and the Fundamental Research Funds for the Central Universities (Nos. 2019B17914, B210206006).
Author Contributions: Study conception and design: Linxian Liao, Hang Guo; investigation and data collection: Hang Guo, Qi Wei, Peng Chen, Kechun Wang; analysis and interpretation of results: Hang Guo, Junzeng Xu, Zhenhao Zheng; draft manuscript preparation: Hang Guo, Junzeng Xu, Linxian Liao. All authors reviewed the results and approved the final version of the manuscript.
Availability of Data and Materials: The data are available upon request from the corresponding author.
Ethics Approval: Not applicable.
Conflicts of Interest: The authors declare that they have no conflicts of interest to report regarding the present study.
References
1. Wu, Y., Si, W., Yan, S. C., Wu, L. F., Zhao, W. J. et al. (2023). Water consumption, soil nitrate-nitrogen residue, and fruit yield of drip-irrigated greenhouse tomatoes under various irrigation levels and fertilization practices. Agricultural Water Management, 277(5), 108092. https://doi.org/10.1016/j.agwat.2022.108092 [Google Scholar] [CrossRef]
2. Cheng, M., Wang, H., Fan, J., Xiang, Y., Tang, Z. et al. (2021). Effects of nitrogen supply on tomato yield, water use efficiency, and fruit quality: A global meta-analysis. Scientia Horticulturae, 290, 110553. https://doi.org/10.1016/j.scienta.2021.110553 [Google Scholar] [CrossRef]
3. Chand, J. B., Hewa, G., Hassanli, A., Myers, B. (2021). Deficit irrigation on tomato production in a greenhouse environment: A review. Journal of Irrigation and Drainage Engineering, 147(2), 295. https://doi.org/10.1061/(ASCE)IR.1943-4774.0001529 [Google Scholar] [CrossRef]
4. Lahoz, I., Pérez-de-Castro, A., Valcárcel, M., Macua, J. I., Beltrán, J. et al. (2016). Effect of water deficit on the agronomical performance and quality of processing tomato. Scientia Horticulturae, 200, 55–65. https://doi.org/10.1016/j.scienta.2015.12.051 [Google Scholar] [CrossRef]
5. Chand, J., Hewa, G., Hassanli, A., Myers, B. (2020). Evaluation of deficit irrigation and water quality on production and water productivity of tomato in greenhouse. Agriculture, 10(7), 297. https://doi.org/10.3390/agriculture10070297 [Google Scholar] [CrossRef]
6. Lv, H., Lin, S., Wang, Y., Lian, X., Zhao, Y. et al. (2019). Drip fertigation significantly reduces nitrogen leaching in solar greenhouse vegetable production system. Environmental Pollution, 245(1), 694–701. https://doi.org/10.1016/j.envpol.2018.11.042 [Google Scholar] [PubMed] [CrossRef]
7. Yao, Z., Yan, G., Wang, R., Zheng, X., Liu, C. et al. (2019). Drip irrigation or reduced N-fertilizer rate can mitigate the high annual N2O+NO fluxes from Chinese intensive greenhouse vegetable systems. Atmospheric Environment, 212, 183–193. https://doi.org/10.1016/j.atmosenv.2019.05.056 [Google Scholar] [CrossRef]
8. Bao, H., Li, Y. (2010). Effect of stage-specific saline irrigation on greenhouse tomato production. Irrigation Science, 28(5), 421–430. https://doi.org/10.1007/s00271-009-0204-x [Google Scholar] [CrossRef]
9. Zhong, F. L., Hou, M. M., He, B. Z., Chen, I. Z. (2017). Assessment on the coupling effects of drip irrigation and organic fertilization based on entropy weight coefficient model. PeerJ, 5, e3855. https://doi.org/10.7717/peerj.3855 [Google Scholar] [PubMed] [CrossRef]
10. Liu, H., Duan, A. W., Li, F. S., Sun, J. S., Wang, Y. C. et al. (2013). Drip irrigation scheduling for tomato grown in solar greenhouse based on pan evaporation in north china plain. Journal of Integrative Agriculture, 12(3), 520–531. https://doi.org/10.1016/s2095-3119(13)60253-1 [Google Scholar] [CrossRef]
11. Zotarelli, L., Scholberg, J. M., Dukes, M. D., Muñoz-Carpena, R., Icerman, J. (2009). Tomato yield, biomass accumulation, root distribution and irrigation water use efficiency on a sandy soil, as affected by nitrogen rate and irrigation scheduling. Agricultural Water Management, 96(1), 23–34. https://doi.org/10.1016/j.agwat.2008.06.007 [Google Scholar] [CrossRef]
12. Al-Ghobari, H. M., Dewidar, A. Z. (2018). Integrating deficit irrigation into surface and subsurface drip irrigation as a strategy to save water in arid regions. Agricultural Water Management, 209(1–2), 55–61. https://doi.org/10.1016/j.agwat.2018.07.010 [Google Scholar] [CrossRef]
13. Cohen, I., Halpern, M., Yermiyahu, U., Bar-Tal, A., Gendler, T. et al. (2019). CO2 and nitrogen interaction alters root anatomy, morphology, nitrogen partitioning and photosynthetic acclimation of tomato plants. Planta, 250(5), 1423–1432. https://doi.org/10.1007/s00425-019-03232-0 [Google Scholar] [PubMed] [CrossRef]
14. Cui, G. C., Zhang, Y., Zhang, W. J., Lang, D. Y., Zhang, X. J. et al. (2019). Response of carbon and nitrogen metabolism and secondary metabolites to drought stress and salt stress in plants. Journal of Plant Biology, 62(6), 387–399. https://doi.org/10.1007/s12374-019-0257-1 [Google Scholar] [CrossRef]
15. Maathuis, F. J. (2009). Physiological functions of mineral macronutrients. Current Opinion in Plant Biology, 12(3), 250–258. https://doi.org/10.1016/j.pbi.2009.04.003 [Google Scholar] [PubMed] [CrossRef]
16. Wang, X. C., Liu, R., Luo, J. N., Zhu, P. F., Wang, Y. S. et al. (2022). Effects of water and NPK fertigation on watermelon yield, quality, irrigation-water, and nutrient use efficiency under alternate partial root-zone drip irrigation. Agricultural Water Management, 271(27), 107785. https://doi.org/10.1016/j.agwat.2022.107785 [Google Scholar] [CrossRef]
17. Ju, X. T., Xing, G. X., Chen, X. P., Zhang, S. L., Zhang, L. J. et al. (2009). Reducing environmental risk by improving N management in intensive Chinese agricultural systems. Proceedings of the National Academy of Sciences of the United States of America, 106(19), 8077. https://doi.org/10.1073/pnas.0902655106 [Google Scholar] [CrossRef]
18. Spiertz, J. H. J. (2010). Nitrogen, sustainable agriculture and food security. A review. Agronomy for Sustainable Development, 30(1), 43–55. https://doi.org/10.1051/agro:2008064 [Google Scholar] [CrossRef]
19. Wang, D. P., Zheng, L., Gu, S. D., Shi, Y. F., Liang, L. et al. (2018). Soil nitrate accumulation and leaching in conventional, optimized and organic cropping systems. Plant Soil and Environment, 64(4), 156–163. https://doi.org/10.17221/768/2017-PSE [Google Scholar] [CrossRef]
20. Ye, Y. S., Liang, X. Q., Chen, Y. X., Liu, J., Gu, J. T. et al. (2013). Alternate wetting and drying irrigation and controlled-release nitrogen fertilizer in late-season rice. Effects on dry matter accumulation, yield, water and nitrogen use. Field Crops Research, 144, 212–224. https://doi.org/10.1016/j.fcr.2012.12.003 [Google Scholar] [CrossRef]
21. Ashour, M., Alprol, A. E., Heneash, A. M. M., Saleh, H., Abualnaja, K. M. et al. (2021). Ammonia bioremediation from aquaculture wastewater effluents using arthrospira platensis NIOF17/003: Impact of biodiesel residue and potential of ammonia-loaded biomass as rotifer feed. Materials, 14(18), 5460. https://doi.org/10.3390/ma14185460 [Google Scholar] [PubMed] [CrossRef]
22. Dauda, A. B., Ajadi, A., Tola-Fabunmi, A. S., Akinwole, A. O. (2019). Waste production in aquaculture: Sources, components and managements in different culture systems. Aquaculture and Fisheries, 4(3), 81–88. https://doi.org/10.1016/j.aaf.2018.10.002 [Google Scholar] [CrossRef]
23. Gogoi, M., Mukherjee, I., Chaudhuri, S. R. (2022). Characterization of ammonia remover Bacillus albus (ASSF01) in terms of biofilm-forming ability with application in aquaculture effluent treatment. Environmental Science and Pollution Research, 29(41), 61838–61855. https://doi.org/10.1007/s11356-021-16021-8 [Google Scholar] [PubMed] [CrossRef]
24. Chatvijitkul, S., Boyd, C. E., Davis, D. A., McNevin, A. A. (2017). Pollution potential indicators for feed-based fish and shrimp culture. Aquaculture, 477(1), 43–49. https://doi.org/10.1016/j.aquaculture.2017.04.034 [Google Scholar] [CrossRef]
25. Neori, A., Krom, M. D., van Rijn, J., (2007). Biogeochemical processes in intensive zero-effluent marine fish culture with recirculating aerobic and anaerobic biofilters. Journal of Experimental Marine Biology and Ecology, 349(2), 235–247. https://doi.org/10.1016/j.jembe.2007.05.023 [Google Scholar] [CrossRef]
26. Neethu, K. C., Balasubramanian, C. P., Biju, I. F., Rekha, M. U., Suvana, S. et al. (2020). Recirculating aquaculture system concepts and designs. Aquaculture Spectrum, 3(6), 33–37. [Google Scholar]
27. Wang, H. X., Qi, M., Bo, Y. H., Zhou, C. X., Yan, X. J. et al. (2021). Treatment of fishery wastewater by co-culture of Thalassiosira pseudonana with Isochrysis galbana and evaluation of their active components. Algal Research-Biomass Biofuels and Bioproducts, 60, 102498. https://doi.org/10.1016/j.algal.2021.102498 [Google Scholar] [CrossRef]
28. Dou, X. (2018). China’s inter-basin water management in the context of regional water shortage. Sustainable Water Resources Management, 4(3), 519–526. https://doi.org/10.1007/s40899-017-0134-3 [Google Scholar] [CrossRef]
29. da Silva, A. A., Melo, S. S., Umbelino, B. F., Sa, F. V. D., Dias, N. D. et al. (2021). Cherry tomato production and seed vigor under irrigation with saline effluent from fish farming. Revista Brasileira de Engenharia Agrícola e Ambiental, 25(6), 380–385. https://doi.org/10.1590/1807-1929/agriambi.v25n6p380-385 [Google Scholar] [CrossRef]
30. Khawla, K., Besma, K., Enrique, M., Mohamed, H. (2019). Accumulation of trace elements by corn (Zea mays) under irrigation with treated wastewater using different irrigation methods. Ecotoxicology and Environmental Safety, 170(1–4), 530–537. https://doi.org/10.1016/j.ecoenv.2018.12.025 [Google Scholar] [PubMed] [CrossRef]
31. McIntosh, D., Fitzsimmons, K. (2003). Characterization of effluent from an inland, low-salinity shrimp farm: What contribution could this water make if used for irrigation. Aquacultural Engineering, 27(2), 147–156. https://doi.org/10.1016/s0144-8609(02)00054-7 [Google Scholar] [CrossRef]
32. Xie, Y., Wang, Z. C., Guo, X. P., Lakthan, S., Chen, S. et al. (2019). Effects of different irrigation treatments on aquaculture purification and soil desalination of paddy fields. Water, 11(7), 1424. https://doi.org/10.3390/w11071424 [Google Scholar] [CrossRef]
33. Kolozsvari, I., Kun, A., Jancso, M., Bakti, B., Bozan, C. et al. (2021). Utilization of fish farm effluent for irrigation short rotation willow (Salix alba L.) under lysimeter conditions. Forests, 12(4), 457. https://doi.org/10.3390/f12040457 [Google Scholar] [CrossRef]
34. Qi, D. L., Yan, J., Zhu, J. Q. (2020). Effect of a reduced fertilizer rate on the water quality of paddy fields and rice yields under fishpond effluent irrigation. Agricultural Water Management, 231(1), 105999. https://doi.org/10.1016/j.agwat.2020.105999 [Google Scholar] [CrossRef]
35. Islam, D., Adham, A. K. M., Islam, M. T. (2016). Effects of dairy farms wastewater irrigation on wheat production and soil health. Journal of Environmental Science and Natural Resources, 8(2), 157–162. https://doi.org/10.3329/jesnr.v8i2.26884 [Google Scholar] [CrossRef]
36. Roberts, T. L., Ross, W. J., Norman, R. J., Slaton, N. A., Wilson, C. E. (2011). Predicting nitrogen fertilizer needs for rice in arkansas using alkaline hydrolyzable- nitrogen. Soil Science Society of America Journal, 75(3), 1161–1171. https://doi.org/10.2136/sssaj2010.0145 [Google Scholar] [CrossRef]
37. Jian, Y. X. (2021). Evaluation of economic and environmental benefits of different culture models of Penaeus vannamei in my country (Master Thesis) (In Chinese). Shanghai Ocean University, Shanghai, China. [Google Scholar]
38. Wang, C. S., Sun, Z. L., Wu, G., Yuan, M. M., Wang, J. B. et al. (2023). Effects of optimized fertilization on yield, quality and economic benefit of tomato in greenhouse. Journal of Changjiang Vegetables, (10), 64–66 (In Chinese). https://doi.org/10.3865/j.issn.1001-3547.2023.10.020 [Google Scholar] [CrossRef]
39. Parisi, M., Giordano, L., Pentangelo, A., D’Onofrio, B., Villari, G. (2006). Effects of different levels of nitrogen fertilization on yield and fruit quality in processing tomato. Acta Horticulturae, 700, 129–132. https://doi.org/10.17660/ActaHortic.2006.700.19 [Google Scholar] [CrossRef]
40. Wang, Y. T., Huang, S. W., Liu, R. L., Jin, J. Y. (2007). Effects of nitrogen application on flavor compounds of cherry tomato fruits. Journal of Plant Nutrition and Soil Science, 170(4), 461–468. https://doi.org/10.1002/jpln.200700011 [Google Scholar] [CrossRef]
41. Albornoz, F. (2016). Crop responses to nitrogen overfertilization: A review. Scientia Horticulturae, 205(8), 79–83. https://doi.org/10.1016/j.scienta.2016.04.026 [Google Scholar] [CrossRef]
42. Hernández, V., Hellín, P., Fenoll, J., Flores, P. (2020). Impact of nitrogen supply limitation on tomato fruit composition. Scientia Horticulturae, 264(10), 109173. https://doi.org/10.1016/j.scienta.2020.109173 [Google Scholar] [CrossRef]
43. Ji, S. Y., Wang, Y., Zhang, K., Guo, S. T. (2023). Effect of long-term irrigation with reclaimed water from dairy industry on soil available nitrogen. Water Saving Irrigation, 1, 26–32 (In Chinese). https://doi.org/10.12396/jsgg.2022173 [Google Scholar] [CrossRef]
44. Xiao, Y. X., Deng, L. Y., Pei, H. W., Li, Y. L., Lv, S. W. et al. (2022). Effect of domestic reclaimed water irrigation on main nutrients of soil in turf root zone. Water Saving Irrigation, (5), 60–64 (In Chinese). [Google Scholar]
45. Wu, H. Q., Du, S. Y., Zhang, Y. L., An, J., Zou, H. T. et al. (2019). Effects of irrigation and nitrogen fertilization on greenhouse soil organic nitrogen fractions and soil-soluble nitrogen pools. Agricultural Water Management, 216(5), 415–424. https://doi.org/10.1016/j.agwat.2019.02.020 [Google Scholar] [CrossRef]
46. Hu, Y. X., Zeeshan, M., Wang, G. Y., Pan, Y. Q., Liu, Y. X. et al. (2023). Supplementary irrigation and varying nitrogen fertilizer rate mediate grain yield, soil-maize nitrogen accumulation and metabolism. Agricultural Water Management, 276, 108066. https://doi.org/10.1016/j.agwat.2022.108066 [Google Scholar] [CrossRef]
47. Liu, M., Li, C. C., Xu, X. L., Wanek, W., Jiang, N. et al. (2017). Organic and inorganic nitrogen uptake by 21 dominant tree species in temperate and tropical forests. Tree Physiology, 37(11), 1515–1526. https://doi.org/10.1093/treephys/tpx046 [Google Scholar] [PubMed] [CrossRef]
48. Li, Y. M., Sun, Y. X., Liao, S. Q., Zou, G. Y., Zhao et al. (2017). Effects of two slow-release nitrogen fertilizers and irrigation on yield, quality, and water-fertilizer productivity of greenhouse tomato. Agricultural Water Management, 186(6), 139–146. https://doi.org/10.1016/j.agwat.2017.02.006 [Google Scholar] [CrossRef]
49. Lv, H. F., Zhao, Y. M., Wang, Y. F., Wan, L., Wang, J. G. et al. (2020). Conventional flooding irrigation and over fertilization drives soil pH decrease not only in the top-but also in subsoil layers in solar greenhouse vegetable production systems. Geoderma, 363(1), 114156. https://doi.org/10.1016/j.geoderma.2019.114156 [Google Scholar] [CrossRef]
50. Delaide, B., Teerlinck, S., Decombel, A., Bleyaert, P. (2019). Effect of wastewater from a pikeperch (Sander lucioperca L.) recirculated aquaculture system on hydroponic tomato production and quality. Agricultural Water Management, 226(1–4), 105814. https://doi.org/10.1016/j.agwat.2019.105814 [Google Scholar] [CrossRef]
51. Becerra-Castro, C., Lopes, A. R., Vaz-Moreira, I., Silva, E. F., Manaia, C. M. et al. (2015). Wastewater reuse in irrigation: A microbiological perspective on implications in soil fertility and human and environmental health. Environment International, 75, 117–135. https://doi.org/10.1016/j.envint.2014.11.001 [Google Scholar] [PubMed] [CrossRef]
52. Khalid, S., Shahid, M., Natasha, Bibi, I., Sarwar, T. et al. (2018). A review of environmental contamination and health risk assessment of wastewater use for crop irrigation with a focus on low and high-income countries. International Journal of Environmental Research and Public Health, 15(5), 895. https://doi.org/10.3390/ijerph15050895 [Google Scholar] [PubMed] [CrossRef]
53. Kong, W. D., Zhu, Y. G., Fu, B. J., Marschner, P., He, J. Z. (2006). The veterinary antibiotic oxytetracycline and Cu influence functional diversity of the soil microbial community. Environmental Pollution, 143(1), 129–137. https://doi.org/10.1016/j.envpol.2005.11.003 [Google Scholar] [PubMed] [CrossRef]
54. Peltier, E., Vincent, J., Finn, C., Graham, D. W. (2010). Zinc-induced antibiotic resistance in activated sludge bioreactors. Water Research, 44(13), 3829–3836. https://doi.org/10.1016/j.watres.2010.04.041 [Google Scholar] [PubMed] [CrossRef]
Cite This Article
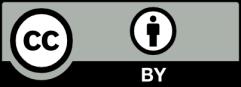
This work is licensed under a Creative Commons Attribution 4.0 International License , which permits unrestricted use, distribution, and reproduction in any medium, provided the original work is properly cited.