Open Access
ARTICLE
Effect of Shoot Control on Flower Bud Differentiation, Flowering, and Fruit Setting in Zanthoxylum armatum DC.
1 Key Laboratory of National Forestry and Grassland Administration on Biodiversity Conservation in Southwest China, Southwest Forestry University, Kunming, 650224, China
2 Key Laboratory of Conservation and Utilization of Forest Resources in the Southwest Mountains, Southwest Forestry University, Kunming, 650224, China
3 College of Forestry, Southwest Forestry University, Kunming, 650224, China
* Corresponding Authors: Zhuogong Shi. Email: ; Jinfang Meng. Email:
Phyton-International Journal of Experimental Botany 2023, 92(12), 3251-3266. https://doi.org/10.32604/phyton.2023.042735
Received 09 June 2023; Accepted 13 September 2023; Issue published 28 December 2023
Abstract
In this study, newly sprouted shoots of Zanthoxylum armatum (Z. armatum), which were collected after the harvesting period, were used as the primary experimental specimens. A randomized block design and paraffin sectioning method were used to investigate the flower bud differentiation process and the quantity and vitality of buds. Furthermore, the study examined the response of flowering and fruiting to cultivation methods for shoot growth, including layering and plant growth regulator application. The results showed that (a) layering and application of plant growth regulators for Z. armatum accelerated the process of flower bud differentiation by approximately 20 days compared to the control group. Additionally, both shoot control methods generated more and larger bud primordia and perianth primordia during the same differentiation phase. (b) The application of plant growth regulators resulted in well-developed buds, exhibiting higher levels of flower bud differentiation than the layering method. The quality of flower bud formation for both shoot control methods was superior to that of the control group. (c) The flowering phenological period was relatively consistent between the two cultivation methods, but the fruit maturity phase for shoot-controlled trees occurred 20 days earlier than the control group. (d) Both layering and the application of plant growth regulators significantly decreased the rates of unfertilized flower shedding and fruit shedding. However, no significant difference was noted in fruit setting per inflorescence and per flower between the two methods and the control. The effect of altitude for both methods on the fruit setting was not significant. Under both shoot control methods, the Z. armatum exhibited earlier morphological differentiation of flower buds, faster differentiation process, improved flower bud quality, and significantly decreased rates of flower and fruit shedding. Thus, these cultivation methods demonstrated the potential to promote flowering, improve fruit setting, and reduce fruit shedding in Z. armatum.Keywords
Zanthoxylum armatum DC (Z. armatum), belonging to the family Rutaceae, is a small tree commonly known as “green pepper” [1,2]. The fruit peel of this plant is a high-quality aromatic spice, and its seeds are used as raw material for premium edible oils in China [3]. With the adjustment of rural industrial structure in mountainous areas in recent years, Z. armatum has been extensively cultivated in Yunnan, Guizhou, Sichuan, and Chongqing of China, and the planting area is expanding. However, the current cultivation and management practices for Z. armatum lack specificity, resulting in excessive shoot elongation, poor flower bud formation quality, and severe flower and fruit shedding. These issues lead to low yield per unit area and low quality, which, to some extent, affect the enthusiasm of Z. armatum cultivators.
Previous studies on Z. armatum have primarily focused on cultivation and management practices [4], control of diseases and insect pests [5–7], genetics and breeding [8–11], medicinal properties [12–14], and processing and utilization [15–18]. With regards to flower bud differentiation of Z. armatum, Chang et al. [19] have made significant contributions by categorizing the process into six different stages for the first time. Additionally, a subsequent study by Hu et al. [20] expanded the classification of Z. armatum flower bud morphology into seven stages, effectively improving the understanding of the biological characteristics underlying the process of flower bud morphological differentiation in Z. armatum. Lv undertook a comparative study on flower bud differentiation between Dahongpao in Fengxian County and Dahongpao in Hancheng [21] further adding to our knowledge in this area. In recent years, multiple studies have focused on exploring the hormonal balance in the context of regulating flower bud differentiation, with a notable emphasis on the interaction between cytokinin (CK), indole-3-acetic acid (IAA), abscisic acid (ABA), and gibberellic acid (GA) [22–26]. The application of growth regulators could inhibit branch elongation, enhance lignification, elevate rates of flower bud differentiation and fruit setting, and increase the yield of “Hanyuan PutaoQingjiao” [27]. Moreover, growth regulators have demonstrated the capability to regulate the flowering period of various plant species [28,29]. Several studies have suggested that hormone treatment could regulate the sex differentiation of flower buds in certain plant species [30,31]. However, the concentration and treatment time of growth regulators confer different effects on different plants, and the underlying regulatory mechanisms are complicated [32]. In terms of flowering and fruit setting, Zeng et al. [33] conducted a study on the topping (removing the unproductive leafy tips) of Zanthoxylum schinifoliumin in dry and hot environmental conditions. Notably, these practices played a role in preventing the shedding of flowers and fruits, thereby promoting flower budding and fruit retention. The impact of varied nutrient ratios and dosages of nitrogen (N), phosphorus (P), and potassium (K) on the flowering and fruit abscission of Z. armatum was explored by Meng et al. [34]. The results unveiled that the spraying of boron fertilizer increased the fruit-setting rate of Z. armatum by 6.73%, with notable significance observed in the effects of different fertilizer treatments on the fruit-setting rate. In addition, the application of a 0.33% micronutrient soluble fertilizer through spraying during the early fruit stage, specifically 20 days after flowering exhibited a remarkable improvement in the fruit set and quality of Z. armatum. The process of fruit shedding is common among various plant species in nature, serving as an adaptive response to the external environment. Extensive research has focused on reducing the plant fruit drop rate to improve agricultural yield and productivity [35]. In numerous plant species, the thinning of flowers and fruits is often practiced, as it has been observed that excessive fruit load tends to compromise overall quality. Of note, Z. armatum is one such plant species frequently affected by fruit shedding [36]. The results showed a noticeable occurrence of fruit drop, accompanied by the withering of most stigmas towards the end of the flowering period. This phenomenon was attributed to the asynchronous fruit setting in the inflorescence, leading to the early shedding of fruits that had been set earlier during the flowering period [37]. There are different periods of fruit-shedding in Z. armatum. In the initial phase, fruits detach from their stalk, which primarily occurs in the early stage of fruit shedding. In the subsequent phase, the fruits detach without stalk, mainly occurring in the later stage of fruit abscission. Some studies have indicated that the shed fruits exhibit significantly lower content of N, P, K, soluble sugars, and other physiological indexes than the retained fruits.
Presently, a common practice in Z. armatum fruit harvesting involves the pruning of all the primary fruit-bearing branches. In the following year, newly-formed branches during the summer season are used as fruit-bearing branches. It is crucial for these new branches to attain a certain length in a short time to ensure sufficient flower bud differentiation and foster buds with robust vigor and vitality. Striking a balance becomes critical as excessively rapid growth of new branches can lead to broader spacing between nodes and poor differentiation of flower buds. On the other hand, if the branch length remains excessively short, the resulting yield will be compromised. Therefore, this issue exhibits dual outcomes, highlighting the need for precision in cultivation management practices to achieve an optimal balance for maximizing fruit production.
There are two main cultivation methods to control the shoot growth of Z. armatum, namely shoot layering and application of plant growth regulators. Shoot layering, also known as branch bending, is a technique of manipulating and adjusting the branches by changing the angles, thereby modifying the growth angle and nutrient balance to promote reproductive growth of Z. armatum [38]. Alternatively, the use of plant growth regulators, for example uniconazole, offers a chemical-controlled method to control branching and facilitate flowering in Z. armatum [39–41]. The growth and flowering of Z. armatum are affected by the altitude and microclimate, leading to different growth and development characteristics in different areas. The effects of the two shoot control methods are also different. In light of the concerns of excessive tree density and the overuse of plant growth regulators in production setting, this study was undertaken to evaluate the aforementioned two methods and investigate the flower bud differentiation process, quantity and vitality of flowering buds, and the changes in flowering and fruit setting. This study also aims to provide a scientific basis for developing effective techniques and practices for shoot regulation to promote the rapid and sustainable development of the Z. armatum industry.
2.1 Experimental Site and Conditions
The experiment was conducted in Daguan County, Zhaotong City, Yunnan Province, China, specifically located at coordinates of 27°36′~28°15′N,103°43′~104°07′E. The altitude ranges between 492 to 2,785 m, with higher altitudes in the north-south direction and lower altitudes in the central region. The site has a subtropical monsoon climate, with relatively consistent temperature variations and notable changes in altitudes. The annual average temperature is 14.5°C, the average annual sunshine duration is 966.3 h, and the average precipitation is 991.3 mm. The soil type in this area is classified as lime soil.
The specimens selected for experimentation were 4–6-year-old trees in the fruiting period and acclimatized to the local environment. The variety used was Z. armatum. Standardized management practices were employed across different altitudes to ensure consistency in the cultivation practices.
2.2.1 Selection of the Experimental Plot and Sampling of the Branches
The experimental plot was set up at altitudes of 600 and 800 m in the Tianxing Town Daguan County. Each experimental plot covered an area of approximately 0.2 hm2. The experiment adopted a random block design with single-plant plots and three replicates. In the two plots, three treatments were carried out including layering, application of plant growth regulators, and a control group (CK; no layering or application of plant growth regulators). At each altitude, three plants were assigned to each treatment, resulting in a total of 18 samples. After the harvesting, the new shoots of Z. armatum were selected as the sampled branches. From each plant and treatment, six shoots were sampled from the central region of the tree canopy, which were distributed in all four cardinal directions.
The cultivation methods for experiments are designated as follows:
Treatment 1 (T1): shoot layering. In mid-September, the sprouting shoots in the central region of the canopy were gently pressed at the base of the branch. After shoot layering, the branches displayed an opening angle of about 80°.
Treatment 2 (T2): plant growth regulator application (uniconazole + brassinosteroids). Following the harvest of Z. armatum, the initial application of plant growth regulators was administered when the new branches reached a length of approximately 30 cm. The whole plant was uniformly sprayed, ensuring complete coverage of the canopy. Subsequently, as the new branches attained a length of about 60 cm, a second round of plant growth regulators was sprayed. A third application occurred when the new branches reached a length of about 90 cm. The concentration of the plant growth regulator during the first application was 1000 mg/L, which was increased to 3000 mg/L during the second application and further escalated to 5000 mg/L during the third application. The concentration of plant growth regulators used at different times was determined based on the growth of the branches. As their growth advanced, the concentration of plant growth regulators was increased accordingly.
Control group (CK): No layering or application of plant growth regulators was conducted.
2.2.2 Bud Collection and Fixation
Buds were collected from the 6th–10th nodes of branches. Bud sampling began in early September, with a frequency of every 30 days. One bud was collected from each sample branch, resulting in a total of five collections. The first bud collection was performed at the 6th node, and the subsequent collection focused on the 7th node. The collected buds were fixed in an FPA70 solution, transported to the laboratory, and stored in a refrigerator at 4°C. The preserved buds were then subjected to microscopic sectioning and morphological observations.
2.2.3 Observation of Flowering and Fruiting Characteristics and the Phenological Stage
From November 2021 to November 2022, the main indicators pertaining to the flowering biological characteristics of Z. armatum were assessed. These indicators included the number of inflorescences on fruiting branches (on all the sampled branches), number of flowers per inflorescence (12 inflorescences per sampled plant), rate of flower shedding (12 inflorescences per sampled plant), rate of fruit shedding (12 inflorescences per sampled plant), fruit setting rate per inflorescence (on all the sampled branches), fruit setting rate of the flowers (12 inflorescences per sampled plant). The calculation formulas for the flower shedding rate, fruit shedding rate, fruit setting rate of inflorescences, and fruit setting rate of flowers are as follows:
Flower shedding rate% = (total number of flowers−number of flowers after flower shedding)/total number of flowers × 100%
Fruit shedding rate% = (total number of fruits after flower shedding−number of fruits before harvest)/total number of fruits after flower shedding × 100%
Fruit setting rate of inflorescences% = number of inflorescences with fruit setting after flower shedding/total number of inflorescences × 100%
Fruit setting rate of flowers% = number of flowers with fruit setting after flower shedding/total number of flowers × 100%
The observation criteria for the phenological stages of Z. armatum are presented in Table 1.
2.2.4 Paraffin Section Preparation
With reference to the protocol detailed by Li et al. [42] and Xiong et al. [43], the experimental methodology involved the utilization of conventional paraffin sections. The stepwise procedure included a series of steps, such as sample, collection, specimen fixation, dissection, dehydration, vacuum impregnation, permeabilization, wax immersion, embedding, block trimming, slicing, slide spreading, fixation, dewaxing, staining, sealing microscopic observation, and image acquisition. The sections of flower buds were observed and photographed under the Axio Vision system.
Statistical analysis and graphical illustrations were performed using Microsoft Excel. Furthermore, the SPSS 26.0 software was used for data processing and analysis. One-way analysis of variance (ANOVA) was used to statistically analyze the data among multiple groups, enabling the comparison of the mean values. What test did you use to compare the mean values? The probability level was set at p < 0.05.
3.1 Effects of Different Shoot Control Methods on Flower Bud Differentiation
Under the treatments of shoot layering or plant growth regulators, Z. armatum, situated at an altitude of 600 m, entered the bud differentiation stage in early November. The bud primordia were visible on the inflorescence axis (Figs. 1A and 1B). Conversely, the control plants were still in the inflorescence axis differentiation stage (Fig. 1C). At an altitude of 800 m, the process of flower bud differentiation in early November was similar to that at 600 m. The protrusions on each node of Z. armatum buds increased gradually in response to the layering and plant growth regulators treatments. The bud primordium and the apical bud primordium were clearly visible, indicating that the flower buds entered the blossom bud differentiation stage (Figs. 1D and 1E). In contrast, the control plants remained at the stage of inflorescence axis differentiation (Fig. 1F).
Figure 1: Structural characteristics of flower buds of Z. armatum at different altitudes in response to different treatments (November 5th)
Note: The left panels (A, D) display the visible bud primordium at the blossom bud differentiation phase under layering treatment, the middle panels (B, E) show the visible bud primordium at the blossom bud differentiation phase under Gr treatment, and the right panels (C, F) demonstrate the inflorescence axis differentiation phase under the CK treatment. FP, flower primordium; IP, inflorescence primordium; Gr, growth regulator.
Following the application of plant growth regulators, the differentiated flower buds at altitudes of 600 and 800 m exhibited a noticeable increase in the bud base size compared to the layering method. These treated buds also displayed increased vitality in comparison to the thin and small buds observed in response to layering treatment (Figs. 1A, 1B, 1D, 1E).
In early December, the flower buds at the base of the Z. armatum flower buds on the inflorescence axis gradually protruded towards the periphery under the layering and plant growth regulators treatments at an altitude of 600 m. The formed apical perianth primordium bent and elongated upward in an arc-like shape, suggesting that the flower buds entered the perianth differentiation stage (Figs. 2A and 2B). In contrast, the control plants remained in the flower bud differentiation stage (Fig. 2C). At an altitude of 800 m, the process of flower bud differentiation in early December reached a similar stage as observed at 600 m. The Z. armatum was also in the perianth differentiation stage in response to layering and plant growth regulator application (Figs. 2D and 2E). The control plants were still in the flower bud differentiation stage (Fig. 2F).
Figure 2: Structural characteristics of flower buds of Z. armatum at different altitudes in response to different treatments (December 7th)
Note: The left panels (A, D) display the visible PP at the flower bud differentiation phase under layering treatment, the middle panels (B, E) show the visible PP at the flower bud differentiation phase under Gr treatment, and the right panels (C, F) demonstrate the inflorescence axis differentiation phase under the CK treatment. FP, flower primordium; PP, perianth primordium; Gr, growth regulator.
During the flower bud differentiation stage under control conditions at an altitude of 800 m, a size increase was observed in the flower primordia when compared to plants cultivated at 600 m. Consistently, both layering and plant growth regulator application at 800 m resulted in larger flower primordia than the corresponding flowers grown at 600 m (Figs. 1 and 2).
In the early stages of March in the subsequent year, the flowering process of Z. armatum gradually sprouted under the cultivation methods of layering and plant growth regulator application. This approach resulted in notable changes within the flower ovary, including its enlargement and the progressive encasement of nucellar cells by integument cells. Moreover, two distinct ovules were observed in the ovary, which commenced division and growth during the female gametophyte developmental stage (Figs. 3A, 3B, 3D, 3E). In contrast, the ovule primordia were differentiated in the ovary of the pistil in the control group (Figs. 3C and 3F). The ovule primordia commenced division and differentiation, thereby resulting in a smaller ovary size in the control group compared to the two treatments at the developmental stage of ovule development.
Figure 3: Structural characteristics of flower buds of Z. armatum at different altitudes in response to different treatments (March 9th)
Note: The left panels (A, D) display the visible OP at the developmental phase of the female gametophytes under layering treatment, the middle panels (B, E) show the visible OP at developmental phase of the female gametophytes under Gr treatment, and the right panels (C, F) demonstrate the ovule development phase under the CK treatment. OP, ovary primordium; Gr, growth regulator.
3.2 Phenological Stages of Flowering and Fruiting in Z. armatum DC.
A comprehensive one-year phenological observation was conducted to analyze the effects of different altitudes and treatments during the phenological period of Z. armatum. The findings regarding the timing of the flowering and fruiting phenological stages at different altitudes and under different treatments are presented in Table 2.
The phenological stages of flowering and fruiting in response to the cultivation methods of layering and plant growth regulator application in Z. armatum were observed to occur earlier and have shorter durations compared to the control group. The initial flowering period for both altitudes of 600 and 800 m under the treatments of layering and plant growth regulator application was in mid-March, about 10 days earlier than the control group. Additionally, the subsequent flowering and fruiting phenological stages under the two treatments occurred 10 days earlier than the control group. In the actual cultivation practices, the application of fertilizers to the Z. armatum trees promotes the preservation of flowers and fruits based on the phenological stages of flowering and fruiting as well as the external climate conditions. An appropriate application of fertilizer for flower and fruit protection effectively promotes the preservation of flowers and fruits in Z. armatum.
There was no significant difference in the phenological stages of Z. armatum between the control treatment and the cultivation methods of layering and plant growth regulator application. No significant differences were noted in the plants cultivated at 600 and 800 m altitudes. The fruit maturity phenological stage of Z. armatum is characterized by specific changes, such as the softening of the fruit peel, translucency in the oil cells, transition into a red color, and the development of good flavor and quality. In Daguan County, at or below an altitude of 800 m, fruit maturity typically occurs in early July. However, mature fruits were observed as early as mid-to-late June in response to the layering method.
3.3 Effects of Shoot Control Methods on Flowering and Fruiting of Z. armatum DC.
3.3.1 Fruit Number and Flowers Number per Inflorescence
The number of fruits set in the inflorescence and the total number of flowers in the inflorescence serve as direct indicators of the quality of flower bud differentiation. The average number of fruits set in the inflorescence and the average number of flowers per inflorescence of sample branches at 600 and 800 m altitudes were statistically analyzed (Table 3).
At an altitude of 600 m, both layering and plant growth regulator application resulted in a larger number of flowers and fruits set than the control group. Specifically, the average number of flowers per inflorescence was 68.61 in the control branches, 78.75 in the layering treatment, and 94.42 in the plant growth regulator application. The number of flowers formed under the layering and plant growth regulator treatments was significantly larger than that in the control group. Moreover, the number of flowers formed after the application of plant growth regulators was significantly larger than that of the layering method. The average number of fruits set per inflorescence was 44.22 in the control branches, 48.64 in the layering treatment, and 58.50 after the application of plant growth regulators. Thus, the number of fruits set after the application of plant growth regulators showed a significant increase compared to the control and the layering treatment. Still, there was no significant difference between layering and control treatments (Table 3).
At an altitude of 800 m, the number of flowers and fruit set under the two treatments was significantly higher than the control group. The average number of flowers per inflorescence was 68.83 in the control branches, 83.56 in the layering treatment, and 90.83 in the plant growth regulator treatment. Likewise, the number of flowers formed after both the layering and plant growth regulator treatments was significantly increased relative to that in the control group, and the application of plant growth regulators led to a significantly larger number of flowers compared to the layering method. The average number of fruits set per inflorescence was 41.67 in the control branches, 52.67 in the layering treatment, and 57.81 in the plant growth regulator treatment. The number of fruits set per flower in the layering and plant growth regulator treatments was significantly larger compared to the control. Nevertheless, no significant difference was witnessed between the layering and plant growth regulator treatments.
Collectively, the flower buds of both treatments exhibited greater vitality in comparison to the control group at both altitudes. This effect resulted in a higher yield of flowers and fruits during the flowering and fruiting stages. During different stages of flower bud differentiation, the number of flower primordia formed in response to the two treatments was also larger compared to that of the control group. From the above analysis, it can be concluded that the flower bud quantity and vitality in Z. armatum followed the order of plant growth regulators > layering > control. Therefore, the two treatments proved to be effective and feasible in promoting flower development and flower preservation in Z. armatum.
3.3.2 Rate of Flower-Shedding and Number of Fruits Set per Inflorescence or Flower
This study proceeded to examine the effects of cultivation methods of layering and plant growth regulator application on the fruit setting rate per inflorescence or flower, as well as the rates of flower-shedding and fruit-shedding of Z. armatum (Figs. 4, 5). At an altitude of 600 m, the flower-shedding rates in the control group, layering method, and plant growth regulator application were 70.10%, 66.07%, and 65.47%, respectively. Similarly, the fruit-shedding rate was 63.21% for the control, 58.08% for layering, and 57.46% for plant growth regulator application. It was observed that both flower shedding and fruit shedding rates were considerably high in Z. armatum. However, the rates of flower shedding and fruit shedding in response to the two treatments were significantly lower compared to the control group (p = 0.01), with no significant differences detected between the two treatments. In addition, the fruit setting rates per inflorescence of the control group, layering method, and plant growth regulator application were 64.55%, 62.271%, and 61.9306%, respectively. There was no significant difference in the fruit setting rates per inflorescence or flower between the control group and the two treatments. Meanwhile, the fruit setting rate per flower of the control was slightly higher compared to the use of plant growth regulators (Fig. 4).
Figure 4: Comparison of the effects of different treatments on the fruit setting rate of inflorescence or flower and the rates of flower-shedding and fruit-shedding of Z. armatum at an altitude of 600 m
Note: Multiple comparisons were performed between the means using the Duncan method at a significance level of p = 0.05. The letters a, b, and c indicate statistically significant differences among the groups.
Figure 5: Comparison of the effects of different treatments on the fruit setting rate of inflorescence or flower and the rates of flower-shedding and fruit-shedding of Z. armatum at an altitude of 800 m
Note: Multiple comparisons were performed between the means using the Duncan method at a significance level of p = 0.05. The letters a, b, and c indicate statistically significant differences among the groups.
At an altitude of 800 m, there was no significant difference between the control group and the two treatments in the fruit setting rate per inflorescence or flower. The rates of flower shedding for the control group, layering method, and plant growth regulator application were 69.57%, 64.78%, and 63.54%, respectively. Although the flower shedding rate was higher than 60%, both treatments led to a significantly lower flower shedding rate than the control group (p = 0.01), with no significant differences between the two treatments. In terms of fruit-shedding, the control group, layering method, and plant growth regulator application demonstrated rates of 65.56%, 58.84%, and 57.74%, respectively. Furthermore, the fruit shedding rate of the control group was significantly higher than that observed in the two treatments (p = 0.01).
The rates of flower and fruit shedding at an altitude of 600 m were found to be comparable to those observed at 800 m. Notably, the different treatments had no significant effect on the fruit setting rate per inflorescence or flower. The physical control approach (layering) and the chemical control approach (plant growth regulator application) conferred protective effects on the preservation and vitality of flowers and fruits.
The quantity and percentage of flower bud differentiation in Z. armatum have been found to correlate with the length, coarseness or fineness, quantity, and maturity time of the branches [44–46]. It is noteworthy that the layering method and plant growth regulator application stimulated earlier flower bud differentiation of Z. armatum than the control group. The flower bud differentiation percentage of the two treatments surpassed that of the control group at the same differentiation stage. In addition, when subjected to the plant growth regulator application, the bud primordium exhibited a higher level of flower bud differentiation, along with larger buds compared to those treated with layering. In Daguan County, Yunnan Province, China, the primary plant growth regulator is uniconazole, which functions as a growth retardant for plants. Its inhibitory effect on gibberellin. synthesis allows for the control of vegetative growth, cell elongation suppression, node spacing reduction, induction of branch wood senescence, enhancement of stress resistance, and stimulation of flower bud differentiation. After the application of plant growth regulators, the branches of Z. armatum quickly transition from the vegetative growth to the reproductive growth phase. The underlying causes of flower and fruit shedding in Z. armatum are complex. Previous studies have suggested that fruit abscission is a normal biological characteristic of Z. armatum and can be affected by inclement weather conditions, such as prolonged precipitation and high humidity, which subsequently contribute to the falling of the flowers. In the dry-hot valley area, the high temperatures in May and June can cause the loss of leaf moisture, resulting in fruit shedding. The flowering and fruit setting stages of Z. armatum need a substantial intake of nutrients. Insufficient nutrient supply, especially during the initial stages, may lead to early leaf senescence and subsequent nutrient depletion, thereby causing extensive flower and fruit shedding. The flowering and fruiting periods of Z. armatum are in spring. Dry weather conditions can impede nutrient transportation in the tree, resulting in the production of abnormal flowers. Both mechanical shedding and natural shedding are primary factors responsible for fruit shedding in Z. armatum. Natural shedding is mainly attributed to genetic regulation of hormones control of cell wall degradation enzymes, such as pectinases and cellulases [47,48]. Multiple factors affect fruit shedding, including external environmental conditions such as temperature, light, and water stress, which contribute to fruit shedding in plants. During the peak fruiting period, the removal of the entire inflorescence is often necessary.
In this experimental study, the flower and fruit shedding rates of Z. armatum were significantly reduced by cultivation methods of layering and plant growth regulator application at two different altitudes. However, there was no notable effect on fruit setting rate per inflorescence and per flower. The plant growth regulators applied in the experiment contain brassinosteroids, which act as natural plant hormones. These hormones can affect the nutrients transport to the fruits, regulate plant growth, and provide protection to flowers and fruits. Interestingly, it was found that an appropriate concentration of plant growth regulators could promote flowering and fruit set, while high concentrations had the potential to hinder flowering or cause fruit shedding [49]. In the present study, the plant growth regulators were applied through foliar spraying, with concentrations of 1000 mg/L in the first application, 3000 mg/L in the second application, and 5000 mg/L in the third application. A previous study by Gui et al. [50] revealed that the application of 400 mg/L eneazole was the most effective approach in stimulating the flowering of Z. armatum “Hanyuan PutaoQingjiao.” Furthermore, the most favorable treatment for increasing the yield of a single plant was 300 mg/L eneazole azole. Therefore, different concentrations of plant growth regulators were applied to different varieties of Z. armatum, resulting in varying effects. In the current investigation, we skillfully increased the concentration of plant growth regulators as the new branches developed. Future research should involve gradient tests using growth regulators application at different concentrations to determine the optimal concentration for flowering promoting and fruit preservation in Z. armatum. Additionally, this study evidenced that the layering method induced earlier differentiation of flower buds in Z. armatum, although its effect on bud differentiation was inferior to that of plant growth regulator application. The key management techniques for Z. armatum at different growth stages were studied by Mu et al. [51]. Their findings proposed that the layering method should apply pressure at the base to prevent the formation of an arch. After the formation of lateral branches, it is advisable to promptly conduct layering in Z. armatum to maintain the apical dominance of the main branch, ultimately promoting its development. This layering technique exhibited similar effects to the application of plant growth regulators. Essentially, it modulates the growth direction of shoots in Z. armatum, thereby reducing the apical dominance, retarding shoot growth, and promoting reproductive growth by altering the angle of the branches. Moreover, the effects of layering endured longer than that of plant growth regulator application. However, its effect on flower bud differentiation, especially in terms of the percentage and vitality of flower bud formation, was not as prominent as that of plant growth regulators. To effectively manage trees or varieties with robust vegetative growth and easily-grown branches, a combination of techniques targeting the apical meristems involving layering method and plant growth regulator application can be employed, as their combined effect surpasses that of individual approaches. Future studies should consider combined techniques of shoot layering and plant growth regulator application for different varieties of Z. armatum. Moreover, a comprehensive evaluation of the effects on flower bud differentiation, flowering, and fruit setting in different branches should be conducted. Such investigations may establish scientific foundations for the appropriate strategies in branch and shoot manipulation to achieve optimal flower and fruit yield.
In this study, certain limitations should be taken into consideration. The evaluation of the two cultivation methods regarding shoot control on the flower bud differentiation was confined to Z. armatum plants at altitudes of 600 and 800 m in a dry, hot valley, characterized by low altitude and high temperatures. This particular setting facilitated easy regrowth of branches after pruning. In contrast, it was observed that the quality of flower bud differentiation was negatively affected by temperature variations at higher altitude areas, resulting in a slower growth rate of branches compared to low-altitude regions. Nonetheless, no definitive conclusions were derived from this study. In the future, it will be necessary to conduct experiments on shoot regulation in areas above 800 m in Daguan County, Yunnan Province, China, to enhance our understanding of the effects of two shoot regulation treatments.
In summary, the utilization of layering and plant growth regulators for shoot control accelerated the process of flower bud differentiation of Z. armatum. Notably, the flower bud differentiation period occurred about 20 days earlier than the control group, while the plant growth regulator application led to a higher percentage and vitality in flower bud formation compared to the layering treatment. The phenological stage of flowering and fruiting in the two treatments in Daguan County, below or at 800 m altitude, were observed to commence about 10 days earlier than the control by promoting flower bud differentiation. Typically, fruit maturity occurs in early July. Whereas, with such flowering control methods, it can occur as early as the mid to late June. In addition, the shoot control method significantly reduced the rates of flower and fruit shedding in Z. armatum. Therefore, the layering and plant growth regulator treatments can effectively promote flower bud differentiation, improve the yield and quality of Z. armatum, and hold promising prospects in cultivation practices.
Acknowledgement: We are very grateful to the editor and reviewers’ comments, which is helpful for improving our manuscript during the manuscript preparation.
Funding Statement: This work was financially supported by the Southwest Forestry University Research Foundation (No. 18210135), the Key Research and Development Program of Yunnan Province, Study and Demonstration on the Key Technology of Improving Quality and Efficiency of Zanthoxylum bungeanum Industry (No. 202102AE090013).
Author Contributions: Xiandang Shen, Zhuogong Shi and Jinfang Meng contributed to the conception design, and drafting of the paper; Runxi He and Xin Li were involved in the material preparation; Xiandang Shen was responsible for the collection and analysis of date. All authors commented on previous versions of the manuscript. All authors agree to be accountable for all aspects of the work. All authors reviewed the results and approved the final version of the manuscript.
Availability of Data and Materials: The data presented in this study are available from the corresponding author upon reasonable request.
Conflicts of Interest: The authors declare that they have no conflicts of interest to report regarding the present study.
References
1. Kashyap, M., Garla, V., Dogra, A. (2021). Zanthoxylum armatum. Himalayan Medicinal Plants, 2021, 327–365. https://doi.org/10.1016/B978-0-12-823151-7.00008-8 [Google Scholar] [CrossRef]
2. Zhang, M., Wang, J., Zhu, L., Li, T., Jiang, W. et al. (2017). Zanthoxylum bungeanum Maxim. (RutaceaeA systematic review of its traditional uses, botany, phytochemistry, pharmacology, pharmacokinetics, and toxicology. International Journal of Molecular Sciences, 18(10), 2172. https://doi.org/10.3390/ijms18102172 [Google Scholar] [PubMed] [CrossRef]
3. Alam, F., Us Saqib, Q. N. (2015). Pharmacognostic study and development of quality control parameters for fruit, bark and leaf of Zanthoxylum armatum (Rutaceae). Ancient Science of Life, 34(3), 147–155. https://doi.org/10.4103/0257-7941.157159 [Google Scholar] [PubMed] [CrossRef]
4. Ullah, B., Ibrar, M., Jelani, G., Ahmad, I. (2014). Leaf, stem bark and fruit anatomy of Zanthoxylum armatum DC. (Rutaceae). Pakistan Journal of Botany, 46(4), 1343–1349. [Google Scholar]
5. Chen, J., Li, S., Zhu, T., Han, S., Qiao, T. et al. (2021). First report of Neofusicoccum parvum causing stem and branch blight disease of Zanthoxylum bungeanum in Sichuan, China. Plant Disease, 105(11), 3742. https://doi.org/10.1094/PDIS-10-20-2131-PDN [Google Scholar] [PubMed] [CrossRef]
6. Cao, M., Zhang, S., Liao, R., Wang, X., Xuan, Z. et al. (2021). Spatial virome analysis of Zanthoxylum armatum trees affected with the flower yellowing disease. Frontiers in Microbiology, 12, 702210. https://doi.org/10.3389/fmicb.2021.702210 [Google Scholar] [PubMed] [CrossRef]
7. Cao, M., Zhang, S., Li, M., Liu, Y., Dong, P. et al. (2019). Discovery of four novel viruses associated with flower yellowing disease of green Sichuan pepper (Zanthoxylum Armatum) by virome analysis. Viruses, 11(8), 696. https://doi.org/10.3390/v11080696 [Google Scholar] [PubMed] [CrossRef]
8. Kim, W. J., Yang, S., Choi, G., Park, I., Noh, P. et al. (2019). Development of conventional PCR and real-time PCR assays to discriminate the origins of Chinese pepper oil and herbal materials from Zanthoxylum. Journal of the Science of Food and Agriculture, 99(4), 2021–2029. https://doi.org/10.1002/jsfa.9458 [Google Scholar] [PubMed] [CrossRef]
9. Liu, C., Guo, H. Y., Liu, H., Yu, J., Li, S. et al. (2022). Differential metabolomics reveals pathogenesis of pestalotiopsis kenyana causing leaf spot disease of Zanthoxylum schinifolium. Journal of Fungi, 8(11), 1208. https://doi.org/10.3390/jof8111208 [Google Scholar] [PubMed] [CrossRef]
10. Alam, F., Saqib, Q. N. U., Ashraf, M. (2018). Zanthoxylum armatum DC extracts from fruit, bark and leaf induce hypolipidemic and hypoglycemic effects in mice- in vivo and in vitro study. BMC Complementary and Alternative Medicine, 18(1), 68. https://doi.org/10.1186/s12906-018-2138-4 [Google Scholar] [PubMed] [CrossRef]
11. Uthamaramasamy, S. (2018). Preventive effect of Zanthoxylum armatum fruit on mitochondrial lipids alteration in isoproterenol-induced myocardial infarcted rats. International Journal of Green Pharmacy, 11(4), S721–S725. https://doi.org/10.22377/ijgp.v11i04.1350 [Google Scholar] [CrossRef]
12. Verma, N., Khosa, R. L. (2009). Pharmacognostical studies on Zanthoxylum armatum leaves. Ancient Science of Life, 29(1), 6–11. [Google Scholar] [PubMed]
13. Kanwal, R., Arshad, M., Bibi, Y., Asif, S., Chaudhari, S. K. (2015). Evaluation of ethnopharmacological and antioxidant potential of Zanthoxylum armatum DC. Journal of Chemistry, 4(5), 1–8. https://doi.org/10.1155/2015/925654 [Google Scholar] [CrossRef]
14. Singh, T. D., Meitei, H. T., Sharma, A. L., Robinson, A., Singh, L. S. et al. (2015). Anticancer properties and enhancement of therapeutic potential of cisplatin by leaf extract of Zanthoxylum armatum DC. Biological Research, 48(1), 46. https://doi.org/10.1186/s40659-015-0037-4 [Google Scholar] [PubMed] [CrossRef]
15. Phuyal, N., Jha, P. K., Raturi, P. P., Rajbhandary, S. (2020). In vitro antibacterial activities of methanolic extracts of fruits, seeds, and bark of Zanthoxylum armatum DC. Journal of Tropical Medicine, 2020, 2803063. https://doi.org/10.1155/2020/2803063 [Google Scholar] [PubMed] [CrossRef]
16. Kim, J. G., Lim, J. J., You, J. S., Kwon, H. J., Lim, H. B. (2021). Comparative study of bioactivity and safety evaluation of ethanolic extracts of Zanthoxylum schinifolium fruit and pericarp. Molecules, 26(19), 5919. https://doi.org/10.3390/molecules26195919 [Google Scholar] [PubMed] [CrossRef]
17. Phuyal, N., Jha, P. K., Raturi, P. P., Rajbhandary, S. (2020). Total phenolic, flavonoid contents, and antioxidant activities of fruit, seed, and bark extracts of Zanthoxylum armatum DC. Scientific World Journal, 2020, 8780704. https://doi.org/10.1155/2020/8780704 [Google Scholar] [PubMed] [CrossRef]
18. Yang, J., Wang, Q., Li, L., Li, P., Yin, M. et al. (2022). Chemical composition and antifungal activity of Zanthoxylum armatum fruit essential oil against Phytophthora capsici. Molecules, 27(23), 8636. https://doi.org/10.3390/molecules27238636 [Google Scholar] [PubMed] [CrossRef]
19. Chang, J. W., Tian, Y. T. (1988). Preliminary observation of flower bud differentiation in Zanthoxylum bungeanum. Forest Science and Technology, 31(4), 24–26. https://doi.org/10.13456/j.cnki.lykt.1988.04.010 [Google Scholar] [CrossRef]
20. Hu, M., Ye, M., Gou, Y. (2019). The flower bud morphological differentiation and bud structure of Zanthoxylum armatum DC. Journal of Nuclear Agricultural Sciences, 33(7), 1415–1422. https://doi.org/10.11869/j.issn.100-8551.2019.07.1423 [Google Scholar] [CrossRef]
21. Lv, X. J. (2013). Studies on the physiological changes during flower bud differentiation processes in Zanthoxylum bungeanum. Northwest A&F University, China. [Google Scholar]
22. Zik, M., Irish, F. V. (2003). Flower development: Initiation, differentiation, and diversification. Annual Review of Cell and Developmental Biology, 19(1), 119–140. https://doi.org/10.1146/annurev.cellbio.19.111301.134635 [Google Scholar] [PubMed] [CrossRef]
23. Tiburcio, A. F., Ravindar, K. S., Galston, A. W. (1988). Polyamine biosynthesis during vegetative and floral bud differentiation in thin layer tobacco tissue cultures. Plant & Cell Physiology, 1988(7), 1241–1249. https://doi.org/10.1016/0303-7207(88)90111-6 [Google Scholar] [CrossRef]
24. Fukuda, N., Yoshida, T., Olsen, J. E., Senaha, C., Jikumaru, Y. et al. (2012). Short main shoot length and inhibition of floral bud development under red light can be recovered by application of gibberellin and cytokinin. Acta Horticulturae, 956, 215–222. https://doi.org/10.17660/ActaHortic.2012.956.23 [Google Scholar] [CrossRef]
25. Hassankhah, A., Rahemi, M., Mozafari, M., Vahdati, K. (2018). Flower development in walnut: Altering the flowering pattern by gibberellic acid application. Notulae Botanicae Horti Agrobotanici Cluj-Napoca, 46(2), 700–706. https://doi.org/10.15835/nbha46211183 [Google Scholar] [CrossRef]
26. Fang, S., Gao, K., Hu, W., Snider, J. L., Wang, S. et al. (2018). Chemical priming of seed alters cotton floral bud differentiation by inducing changes in hormones, metabolites and gene expression. Plant Physiology and Biochemistry, 130, 633–640. https://doi.org/10.1016/j.plaphy.2018.08.010 [Google Scholar] [PubMed] [CrossRef]
27. Zhou, C., Cai, Y., Yang, Z., Wang, H., Deng, F. et al. (2020). Nitrogen, phosphorus and potassium fertilization promotes Zanthoxylum armatum ‘Hanyuan Putao Qingjiao’ flower bud differentiation in Sichuan, China. Horticulture, Environment, and Biotechnology, 61(4), 651–661. https://doi.org/10.1007/s13580-020-00251-9 [Google Scholar] [CrossRef]
28. Okuda, N., Dumrongkittikule, S., Fujime, Y. (2000). Inhibition of flower bud formation in Tsai-Hsin with uniconazole and gibberellin treatments. Acta Horticulturae, (515), 49–58. https://doi.org/10.17660/ActaHortic.2000.515.5 [Google Scholar] [CrossRef]
29. Karki, K. B., Dhakal, D. D. (2003). Effect of paclobutrazol on off-year induction of flowers in mango. Journal of the Institute of Agriculture and Animal Science, 24, 51–57. https://doi.org/10.3126/jiaas.v24i0.373 [Google Scholar] [CrossRef]
30. Samach, A., Smith, H. M. (2013). Constraints to obtaining consistent annual yields in perennials. II: Environment and fruit load affect induction of flowering. Plant Science, 207(1), 168–176. https://doi.org/10.1016/j.plantsci.2013.02.006 [Google Scholar] [PubMed] [CrossRef]
31. Carpenter, D. J., Mathiassen, S. K., Boutin, C., Strandberg, B., Casey, C. S. et al. (2010). Effects of herbicides on flowering. Environmental Toxicology and Chemistry, 39(6), 1244–1256. https://doi.org/10.1002/etc.4712 [Google Scholar] [PubMed] [CrossRef]
32. Gökbayrak, Z., Engin, H., Akçal, A., Gür, E. (2014). Gibberellic acid inhibits floral formation and delays flower differentiation in ‘0900 Ziraat’ sweet cherry cultivar. European Journal of Horticultural Science, 79(5), 260–266. https://doi.org/10.2037/24126876 [Google Scholar] [CrossRef]
33. Zeng, Q. X., Fan, H. G., Li, Z. Y., Ding, Y. P., Jiang, D. H. et al. (2022). Pruning techniques of Z. armatum in dry-hot valley. Forest Science and Technology, 65(3), 88–89. https://doi.org/10.13456/j.cnki.lykt.2021.11.22.0002 [Google Scholar] [CrossRef]
34. Meng, Q. C., Liu, S. M., Sun, B. Y. (2009). The effect of balanced fertilization on yield of Zanthoxylum bungeanum. Journal of Northwest Forestry University, 24(3), 105–108. [Google Scholar]
35. Chung, K. R., Shilts, T., Ertürk, U., Timmer, L. W., Ueng, P. P. (2003). Indole derivatives produced by the fungus Colletotrichum acutatum causing lime anthracnose and postbloom fruit drop of citrus. FEMS Microbiology Letters, 226(1), 23–30. https://doi.org/10.1016/S0378-1097(03)00605-0 [Google Scholar] [PubMed] [CrossRef]
36. Dong, B., Deng, Y., Wang, H., Gao, R., Stephen, G. K. et al. (2017). Gibberellic acid signaling is required to induce flowering of chrysanthemums grown under both short and long days. International Journal of Molecular Sciences, 18(6), 1259. https://doi.org/10.3390/ijms18061259 [Google Scholar] [PubMed] [CrossRef]
37. Ruiz, R., García-Luis, A., Monerri, C., Guardiola, J. L. (2001). Carbohydrate availability in relation to fruitlet abscission in Citrus. Annals of Botany, 87(6), 805–812. https://doi.org/10.1006/anbo.2001.1415 [Google Scholar] [CrossRef]
38. Chutinanthakun, T., Sekozawa, Y., Sugaya, S., Gemma, H. (2015). Effect of bending and the joint tree training system on the expression levels of GA3- and GA2-oxidases during flower bud development in ‘Kiyo’ Japanese plum. Scientia Horticulturae, 193, 308–315. https://doi.org/10.1016/j.scienta.2015.07.023 [Google Scholar] [CrossRef]
39. Han, H., Yang, W., Wenyu, Y. (2009). Influence of uniconazole and plant density on nitrogen content and grain quality in winter wheat in South China. Plant Soil and Environment, 55(4), 159–166. https://doi.org/10.1111/j.1365-3059.2009.02031.x [Google Scholar] [CrossRef]
40. Liu, C., Yu, H., Rao, X., Li, L., Dixon, R. A. (2021). Abscisic acid regulates secondary cell-wall formation and lignin deposition in Arabidopsis thaliana through phosphorylation of NST1. Proceedings of the National Academy of Sciences, 118(5). https://doi.org/10.1073/pnas.2010911118 [Google Scholar] [PubMed] [CrossRef]
41. Baylis, A. D., Hutley-Bull, P. D. (1991). The effects of a paclobutrazol-based growth regulator on the yield, quality and ease of management of oilseed rape. Annals of Applied Biology, 118(2), 445–452. https://doi.org/10.1111/j.1744-7348.1991.tb05645.x [Google Scholar] [CrossRef]
42. Li, H. L., Wan, R. H., Yi, F. (2000). Improvements of the rapid paraffin section method. Fujian Medical Journal, 22(3), 57–57. https://doi.org/10.3969/j.issn.1002-2600.2000.03.052 [Google Scholar] [CrossRef]
43. Xiong, L. Q. (2019). Study on apomictic characteristics of Juglans sigilata dode. Southwest Forestry University, China. https://doi.org/10.27416/d.cnki.gxnlc.2019.000028 [Google Scholar] [CrossRef]
44. Fu, P., Li, W. Y., Liu, M. L. (2020). Improvement of paraffin section preparation method of flower sequence of Vernicia fordii. Journal of Central South University of Forestry and Technology, 40(3), 53–59. https://doi.org/10.14067/j.cnki.1673-923x.2020.03.007 [Google Scholar] [CrossRef]
45. Kosugi, K., Sumitomo, A. (1955). Studies on the flower bud differntiation and development in freesia III. Engei Gakkai Zasshi, 24(3), 204–206. https://doi.org/10.2503/jjshs.24.204 [Google Scholar] [CrossRef]
46. Chen, C., Yu, F. Y. (2020). Advances in flower bud differentiation of forest trees. Forest Science, 56(9), 119–129. https://doi.org/10.11707/j.1001-7488.20200914 [Google Scholar] [CrossRef]
47. Goldschmidt, E. E. (1999). Carbohydrate supply as a critical factor for Citrus fruit development and productivity. Hortscience, 34(6), 1020–1024. https://doi.org/10.1016/S0304-4238(99)00028-X [Google Scholar] [CrossRef]
48. Khalid, A. A. (2006). Effect of acetylene and ethylene gases released from encapsulated calcium carbide on growth and yield of wheat and cotton. Pedobiologia, 50(5), 405–411. https://doi.org/10.1016/j.pedobi.2006.08.002 [Google Scholar] [CrossRef]
49. Yang, J., Zhang, J., Wang, Z., Liu, K., Wang, P. (2006). Post-anthesis development of inferior and superior spikelets in rice in relation to abscisic acid and ethylene. Journal of Experimental Botany, 57(1), 149–160. https://doi.org/10.1093/jxb/erj018 [Google Scholar] [PubMed] [CrossRef]
50. Gui, H. Y. (2019). Effects of plant growth regulators on flower bud differentiation and yield of Z. armatum ‘HanyuanPutaoQingjiao’. Sichuan Agricultural University, China. https://doi.org/10.27345/d.cnki.gsnyu.2019.000941 [Google Scholar] [CrossRef]
51. Mu, Z. L., Zhai, X. Q., Hou, Z. H., Wang, W. J., Zhang, L. et al. (2021). Key management techniques of Zanthoxylum bungeanum in different periods. Journal of Henan Forestry Science and Technology, 41(4), 49–52. https://doi.org/10.3969/j.issn.1003-2630.2021.04.016 [Google Scholar] [CrossRef]
Cite This Article
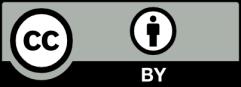
This work is licensed under a Creative Commons Attribution 4.0 International License , which permits unrestricted use, distribution, and reproduction in any medium, provided the original work is properly cited.