Open Access
ARTICLE
Osmotic Regulation, Antioxidant Enzyme Activities and Photosynthetic Characteristics of Tree Peony ( Andr.) in Response to High-Temperature Stress
College of Horticulture and Landscape Architecture, Yangzhou University, Yangzhou, 225009, China
* Corresponding Authors: Jun Tao. Email: ; Daqiu Zhao. Email:
(This article belongs to the Special Issue: Abiotic and Biotic Stress Tolerance in Crop)
Phyton-International Journal of Experimental Botany 2023, 92(11), 3133-3147. https://doi.org/10.32604/phyton.2023.028818
Received 09 January 2023; Accepted 10 March 2023; Issue published 24 October 2023
Abstract
Tree peony (Paeonia suffruticosa Andr.) is a traditional Chinese flower, which prefers cool weather. However, high temperature in summer in the middle and lower reaches of the Yangtze River restricts its growth and development. In this study, osmotic regulation, antioxidant enzyme activities, and photosynthetic characteristics of tree peony in response to high-temperature stress were investigated. The results showed that high-temperature stress had destroyed the cell membrane, manifested as the increased relative electrical conductivity and malondialdehyde content. Moreover, high-temperature stress led to excessive accumulation of reactive oxygen species, thereby, activating antioxidant enzyme activities. Also, photosynthetic parameters and chlorophyll fluorescence parameters directly reflected the damage to the photosystem II reflection center under high-temperature stress. In addition, high-temperature stress led to stomatal closure and chloroplast damage. This study revealed the physiological responses of tree peony to high-temperature stress, laying a foundation for the promotion of tree peony in high-temperature areas and the improvement of high-temperature resistance.
Keywords
Plants are affected by various environmental factors during growth and development, such as temperature, water, and light [1]. In recent years, with the global warming caused by greenhouse gas emissions, high-temperature stress has become an important factor limiting plant growth and productivity [2,3]. Studies have shown that plants can perceive high-temperature stress when 5°C higher than the optimum growth temperature, obvious symptoms of high-temperature stress can destroy of cell homeostasis [4] and thylakoid photochemical reactions in the soma sheet [5]. When the temperature increases by 10°C, carbon metabolism in the chloroplast stroma is impaired [6]. Plants are directly or indirectly damaged by high temperature. The former directly affects the composition of the cytoplasm and reacts in a short time, mainly including the increase in reactive oxygen species (ROS) [7], and damage to the cell membrane structure. The latter mainly refers to the abnormal metabolism of plants under high-temperature stress, including photosynthesis inhibition, enhanced respiration, and accumulation of toxic substances [8]. Plants first mobilize their regulatory mechanisms to adapt to high temperature when it occurs [9]. When the temperature exceeds the maximum the plant can tolerate, it may cause irreversible damage to the plant, and this damage continues throughout the life cycle.
Tree peony (Paeonia suffruticosa Andr.) is a deciduous subshrub of the Paeonia family, which is cultivated and originated in China as a traditional famous flower [10]. Tree peony is a kind of flower with highly ornamental value for varied colors and graceful appearance. In addition, it also has medicinal properties, which can be used as medicine [11]. Tree peony like cool and moderately shaded environments in summer, and the suitable temperature for growth is 17°C–20°C [12], excessive temperature will hinder its growth and development [13]. However, high temperature summer in most of the middle and lower reaches of the Yangtze River in China, which is extremely unfavorable for tree peony to grow and develop, because it can cause burns to the stems and leaves, yellow leaves, scorched edges, and even lead to early leaf wilt, affecting the next year’s flowering. Therefore, it is particularly important to study the physiological response of tree peony to high-temperature stress to improve high-temperature resistance.
Up to now, the studies on tree peony mainly have focused on the flower color [14–16], tissue culture [17], and cultivation [18]. As to the high-temperature stress, only the photosynthetic characteristic response of tree peony was reported [19]. In this study, 3-year-old potted tree peony ‘Huawang’ were used as the materials to investigate the physiological response to high-temperature stress. Firstly, we observed the changes in relative electrical conductivity (REC) and malondialdehyde (MDA) content to judge the damage to the osmotic regulation system. Secondly, ROS accumulation and protective enzyme activity were determined to measure the damage to the redox system. Photosynthetic characteristics and chlorophyll fluorescence parameters were used to evaluate the photosynthetic capacity. Finally, scanning electron microscope (SEM) and transmission electron microscope (TEM) was used to observe the opening and closing state of stomata and the degree of chloroplast damage. These results revealed the physiological responses of tree peony to high-temperature stress, which is of great significance to further popularize the planting of tree peony in the middle and lower reaches of the Yangtze River and southern regions.
2.1 Plant Materials and Treatment
3-year-old potted tree peony ‘Huawang’ were used as plant materials and planted in a 1:1:1 mixture of loam, peat, and perlite. Plants were divided into 3 groups with 3 pots in each group, and 3 treatments were set. One treatment was set as a control (CK-treated) that placed with a daytime temperature of 20°C and nocturnal temperature of 15°C. The second treatment was set as medium temperature treatment (MT-treated) placed with a daytime temperature of 30°C and nocturnal temperature of 25°C. The third treatment was set as high-temperature treatment (HT-treated) placed with a daytime temperature of 40°C and nocturnal temperature of 35°C. Each group was given 60% humidity, 14 h (5:00–19:00) in the daytime, and 10 h (19:00–5:00) at night. Samples were collected at the time heat injury symptoms occurred. The photosynthetic characteristics that were measured from 8 a.m. to 9 a.m., chlorophyll fluorescence parameters, REC, and other physiological indicators of the 3 groups were measured first. 2.5% glutaraldehyde solution was used to fix a small number of samples, and the remaining samples were stored at −80°C.
2.2 Determination of SPAD Value and Chlorophyll Content
Avoided the main leaf veins, the leaves at the third node from top to bottom were selected. The determination of Soil Plant Analysis Development (SPAD) value was recorded by SPAD-502 portable chlorophyll sensing instrument (Konica Minolta Sensing, Japan). Each plant in each group was measured 3–6 times.
We weighed 0.1 g tree peony tissue sample into a mortar, added a small amount of quartz sand, calcium carbonate powder, and 2–3 mL 95% ethanol (or 80% acetone), and then ground it thoroughly. 10 mL ethanol was added and ground until the tissue turns white, then let it stand for 5 min. Placing the filter paper in the funnel, we poured the extract along the glass rod into the funnel and filtered it into the brown volumetric flask. Using ethanol to absorb all the chlorophyll on the filter paper into the volumetric flask until there is no green residue. Finally, we used ethanol to a constant volume of 25 mL and shook it well. The absorbance of chlorophyll extract was measured at 665, 649, and 470 nm.
2.3 Determination of REC and MDA Content
REC was determined according to the method reported by Xu et al. [20]. After rinsing the leaves with distilled water, a 1 cm hole punch was used to punch holes in the leaves, then a 0.1 g sample will be weighed into a syringe containing a small amount of deionized water. The sample was transferred to a glass tube filled with 20 mL of deionized water for 4 h after vacuuming. The initial solution conductivity (C1) and deionized water conductivity (C0) were measured with a conductivity meter (DDS-307, China Lei Magnetic Instrument Co., Ltd., China). The glass tube was heated in boiling water for 30 min, cooled to room temperature, and the conductivity (C2) was measured. REC is calculated according to the following formula: REC (%) = (C1–C0)/(C2–C0) × 100%.
Reference Kit for MDA content determination (Beijing Solarbio Biotechnology Co., Ltd., China).
The accumulation of H2O2 was observed by diamino aniline (DAB) staining. DAB was prepared with 50 mM Tris-acetate buffer at pH 5.0 and 0.1 mg/mL. The fresh leaves were soaked in DAB dye and treated with darkness for 24 h. The leaves were then bathed in boiling water with 95% ethyl alcohol for 15 min before being removed and photographed by fluorescence microscope (Axio Imager D2, ZEISS, Germany). Reference Kit for determination of H2O2 content (Beijing Solarbio Biotechnology Co., Ltd., China).
The accumulation of O2·− was determined using a kit (Shanghai Haling Biotechnology Co., Ltd., China). Avoiding the main veins, fresh leaves were quickly cut with a double-sided blade and placed cut samples were on glass slides and rinsed with cleaning solution, then blotted dry with absorbent paper. 10 μL of fluorescent dye ethidium bromide (DHE) was added dropwise to the surface of the sample and incubated in the dark at 37°C for 20 min. Reference Kit for determination of O2·− content (Beijing Solarbio Biotechnology Co., Ltd., China).
2.5 Determination of Antioxidant Enzyme Activities
Antioxidant enzymes, including superoxide dismutase (SOD), peroxidase (POD), catalase (CAT), and ascorbate peroxidase (APX), were determined by spectrophotometry (BioPhotometer, Eppendorf, Germany) according to the instructions of the corresponding kits (Suzhou Corning Biotechnology Co., Ltd., China).
2.6 Photosynthetic Characteristics
Photosynthetic characteristics were determined with a portable photosynthesis system (LI-6400, Li-Cor, Lincoln, USA). The net photosynthetic rate (Pn), stomatal conductance (Gs), intercellular CO2 concentration (Ci), and transpiration rate (Tr) were recorded separately by measurements taken from 8 a.m. to 9 a.m.
2.7 Chlorophyll Fluorescence Parameters
Plants should be allowed to stand in a dark environment for not less than 4 h before assaying. A chlorophyll fluorescence spectrometer (Heinz Walz GmbH, Germany) was used to measure chlorophyll fluorescence parameters. Using the built-in data processing software PAM Win, we measured and calculated the minimal fluorescence yield of the dark-adapted state (Fo), the maximal quantum yield of PSII (Fv/Fm), the effective quantum yield of PSII photochemistry (Y(II)) and the nonphotochemical quenching coefficient (qN) of chl fluorescence parameters.
The surface of the leaves was observed by SEM. To avoiding leaf veins, we cut fresh leaf samples into small pieces with a razor blade according to the standard of 3 mm × 3 mm and placed them in 2.5% glutaraldehyde solution for more than 2 h. The samples were washed three times with 0.1 mol/L PBS buffer for 15 min each time. The gradients of 30%, 50%, 70%, 80%, 90%, 95%, and 100% ethyl alcohol were used to dehydrate samples for 15 min. The dehydrated samples were then transferred to ethanol containing anhydrous sodium sulfate. After being dried and sprayed with gold (EIKO IB-3, Hitachi, Japan), samples were observed by SEM (Philips XL-30, ESEM, Holland).
Moreover, the anatomical details of leaves were observed by TEM. Similarly, the samples were cut into small pieces of 1 mm × 1 mm with a blade avoiding the veins and fixed in 2.5% glutaraldehyde solution for more than 2 h. Samples were processed before observation. First, the samples were washed 3 times with 0.1 mol/L PBS buffer for 15 min each time. 1% osmic acid was used to fix the samples for 4 h and then washed with 0.1 mol/L PBS buffer three times for 15 min each time. The samples were dehydrated with the above-graded alcohol for 15 min each. The dehydrated samples were treated with anhydrous sodium sulfate in acetone for 15 min and then embedded in resin. Finally, the samples were cut into slices around 70 nm using Leica EM UC6 ultramicrotome (EM UC6, Leica, Germany). Finally, the mesophyll cells and chloroplasts were observed and imaged via TEM (Tecnai 12, Philips, Holland).
All data were repeated 3 times and expressed as mean ± standard deviation. Statistical analysis software package SAS/STAT (version 6.12, SAS Institute, Cary, NC, USA) was used for variance analysis, and GraphPad Prism7 software was used to draw figures.
3.1 Effect of High-Temperature Stress on Plant Phenotype
The phenotypes of tree peony varied under different growth temperatures (Fig. 1). On the 12th day of high-temperature stress, we found that the leaves of HT-treated tree peony turned yellow, and scorched spots appeared on the leaf edge. While the morphological difference between CK and MT is not obvious, the color of MT-treated leaves fades from the edges and the CK-treated still maintain healthy growth, and the leaves remain green.
Figure 1: Effect of high-temperature stress on tree peony phenotype. CK: control, 20/15°C; MT: medium-temperature treatment, 30/25°C; HT: high-temperature treatment, 40/35°C
3.2 Effect of High-Temperature Stress on SPAD Value and Chlorophyll Contents
With the increase in temperature, the SPAD value also decreased significantly (Fig. 2A). Compared with the CK-treated tree peony, the SPAD value of the MT-treated tree peony decreased by about 10%, while the SPAD value of the HT-treated tree peony changed the most obviously, with a reduction of about 50%.
Figure 2: Effect of high-temperature stress on SPAD value and chlorophyll contents of tree peony. (A): SPAD value. (B): Chlorophyll a content. (C): Chlorophyll b content. (D): Chlorophyll (a + b) content. Values represent the mean ± standard deviation (SD) and different letters indicate significant differences according to Duncan’s multiple range test (p < 0.05)
Similarly, chlorophyll contents of the tree peony were significantly reduced under high-temperature treatment, and chlorophyll a, chlorophyll b, and chlorophyll (a + b) respectively decreased by 87%, 73%, and 27% compared with the tree peony under CK treatment (Figs. 2B–2D).
3.3 Effect of High-Temperature Stress on REC and MDA Content
Membrane lipid peroxidation often occurs when plants were damaged, which eventually led to MDA production [21]. Compared with the CK-treated tree peony, MDA content in the MT-treated tree peony was significantly up-regulated. In addition, MDA content in HT-treated tree peony was also significantly up-regulated that about 5 times that of the CK-treated tree peony (Fig. 3A).
Figure 3: Effect of high-temperature stress on osmotic regulation system of tree peony. (A): MDA content. (B): REC. Values represent the mean ± standard deviation (SD) and different letters indicate significant differences according to Duncan’s multiple range test (p < 0.05)
The REC can characterize the degree of damage to plant cell membranes. There was no significant difference between CK-treated and MT-treated tree peony in REC, while high-temperature treatment led to the greatest degree of damage to the plant cell membrane of tree peony, and the corresponding REC was also the largest, which reached 32.7% (Fig. 3B).
3.4 Effect of High-Temperature Stress on ROS Accumulation
The fluorescent probe and DAB staining were used to observe the accumulation of O2·− and H2O2 content in leaves. In the leaves of the CK-treated tree peony, we did not observe the appearance of red fluorescence, and the staining of the leaves was light yellow. However, In MT-treated and HT-treated leaves, the red fluorescence signal was enhanced, especially in HT-treated leaves, the fluorescence signal was the strongest and the staining showed dark brown (Figs. 4A, 4B). In particular, the staining of the upper part of the leaves was significantly deepened, which might be related to the scorch of the edges, indicating that HT-treated leaves were the most seriously damaged.
Figure 4: Effect of high-temperature stress on ROS accumulation of tree peony. (A): Observation of O2·− accumulation by DHE fluorescent probe. (B): Observation of H2O2 accumulation by DAB staining. (C): O2·− content and production rate. (D): H2O2 content. Values represent the mean ± standard deviation (SD) and different letters indicate significant differences according to Duncan’s multiple range test (p < 0.05)
The contents of O2·− and H2O2 in leaves also reflected the above results. The O2·− content and production rate of HT-treated tree peony both increased 10 times that of CK-treated tree peony (Fig. 4C). The H2O2 content of HT-treated tree peony also increased 2 times that of CK-treated tree peony (Fig. 4D).
3.5 Effects of High-Temperature Stress on Antioxidant Enzyme Activity
The activities of antioxidant enzymes of tree peony were positively correlated with increased temperature (Fig. 5). Compared with CK-treated tree peony, MT-treated tree peony had higher activity of SOD, POD, and CAT, and the activity of APX also increased to a certain extent, while the degree of it is not significant as that of CK-treated. By comparison, the antioxidant enzyme activity changed more significantly under high-temperature treatment, and SOD, POD, CAT, and APX activities increased by 53.6%, 71%, 58.0%, and 73.5%, respectively.
Figure 5: Effect of high-temperature stress on antioxidant enzyme activity changes of tree peony. Values represent the mean ± standard deviation (SD) and different letters indicate significant differences according to Duncan’s multiple range test (p < 0.05)
3.6 Effects of High-Temperature Stress on Photosynthetic Parameters
The photosynthetic parameters of tree peony changed significantly with the increase in temperature (Fig. 6). Compared with CK, the Gs, Tr, Ci, and Pn all decreased significantly under HT-treated tree peony. The Gs value was only 51.9% of CK-treated tree peony under high-temperature stress, Tr was only 40.9% of CK, its Ci value decreased by 38.6% compared with that of CK, and Pn was only half of CK. The photosynthetic parameters of leaves under MT also showed a downward trend, but not significantly. The decrease in photosynthetic parameters indicated that high-temperature stress inhibited the photosynthesis of tree peony.
Figure 6: Effects of high-temperature stress on photosynthetic characteristics of tree peony. Values represent the mean ± standard deviation (SD) and different letters indicate significant differences according to Duncan’s multiple range test (p < 0.05)
3.7 Effects of High-Temperature Stress on Chlorophyll Fluorescence Parameters
The chlorophyll fluorescence parameters of tree peony also changed with the increase in temperature (Fig. 7). Compared with CK-treated tree peony, the values of Fv/Fm and Y(II) in tree peony under medium-temperature treatment showed a decreasing trend, while the values of qN and Y(NO) increased. When high temperature occurred, the values of Fv/Fm and Y(II) were only 58.4% and 14.5% of CK, while the values of qN and Y(NO) were 80.3% and 68.4% higher than those of CK. Significant changes in chlorophyll fluorescence parameters indicated that high-temperature stress damages the PSII reflection center.
Figure 7: Effects of high-temperature stress on chlorophyll fluorescence parameters. Values represent the mean ± standard deviation (SD) and different letters indicate significant differences according to Duncan’s multiple range test (p < 0.05)
3.8 Effect of High-Temperature Stress on Leaf Ultrastructure
It was observed by SEM that the stomata of tree peony leaf gradually contracted, and the number gradually decreased with the increase in temperature (Fig. 8). A total of about 26 stomata were observed in the CK-treated tree peony, and about 77% were open. With the increase in the temperature, under MT-treated tree peony, the stomata gradually shrunk and closed in a small amount, the open stomata accounted for only 42 of the total. However, under HT-treated tree peony, nearly half of the stomata were closed, and the remaining stomata shrunk to varying degrees. It can be observed that the stomata gradually open, shrink, and finally close with the increase in temperature through the enlarged image.
Figure 8: Effects of high-temperature stress on epidermis and stomata of tree peony. (A): SEM of CK-treated tree peony leaf. (B): SEM of MT-treated tree peony leaf. (C): SEM of HT-treated tree peony leaf. (D): SEM of partial enlargement of (A). (E): SEM of partial enlargement of (B). (F): SEM of partial enlargement of (C)
Then, TEM was used to observe the state of mesophyll cells and chloroplasts in tree peony leaves (Fig. 9). Compared with CK-treated tree peony, the mesophyll cells of MT-treated tree peony were gradually compressed and deformed, and the chloroplasts tended to disintegrate. The observation results of HT-treated showed that under high-temperature stress, the mesophyll cells of tree peony leaves were completely deformed, and the chloroplasts were also completely disintegrated.
Figure 9: Effects of high-temperature stress on mesophyll cells and chloroplasts of tree peony. (A): TEM of CK-treated tree peony leaf. (B): TEM of MT-treated tree peony leaf. (C): TEM of HT-treated tree peony leaf. (D): Chloroplasts of CK-treated tree peony leaf. (E): Chloroplasts of MT-treated tree peony leaf. (F): Chloroplasts of HT-treated tree peony leaf. CH-chloroplast
High-temperature stress is one of the most common abiotic stresses, which results in a large amount of water loss and enhances transpiration in plants. The leaf is the main vegetative organ for the photosynthesis and transpiration of plants. In this study, CK, MT, and HT-treated tree peony were set, and tree peony leaves under high-temperature stress showed a large area of yellowing, accompanied symptoms of high-temperature stress, such as scorch spots. This phenotype was also observed in peonies used in the same family of Paeonia ostii [22], under high-temperature stress. In addition to the two treatments included in Wang’s study [22], we added a medium-temperature treatment to our study to gain a more comprehensive understanding of the effects of temperature rise on the physiological characteristics of the materials. This allowed us to better identify which indicators are sensitive to temperature rise and which show significant differences only under high-temperature treatment, thus enabling us to take the necessary measures to optimize and improve the phenotype of materials in different environments and ensure their commercial value. Moreover, her object is P. ostii, focusing on the response of plant growth and development under high temperature to the yield and quality of seed oil after flowering and fruiting. In our study, tree peony was used as plant material, which paid more attention to ornamental value.
Cell membrane is the most sensitive part of the leaf to respond to high temperature. High-temperature stress will lead to the accumulation of a large number of O2·− and H2O2 in the plant [23], causing protein and nucleic acid denaturation, further leading to cell membrane damage and lipid peroxidation, expressing by excessive accumulation of ROS [24]. DAB staining was used to observe the color of the stained leaves, and the red fluorescence signal was detected by O2·−. Zhang et al. [21] reported that DAB staining of herbaceous peony leaves was significantly deepened and browned under high-temperature stress, and the observation of O2·− showed that the red fluorescence signal was significantly stronger than CK. The results were consistent with those in our study, and it could be seen that the accumulation of ROS in tree peony showed a progressive trend with the increase in temperature. High-temperature stress resulted in ROS accumulation, which may be an important reason for the damage to chloroplast membrane structure and grana lamella. While high-temperature stress destroys the lipid structure of the cell membrane, the membrane-dependent signal transmission system and metabolic system are disturbed, which eventually leads to the loss of the ability of selective absorption of the inner membrane system, the extravasation of cell fluid, and the increase in electrolyte permeability [25]. REC is an index to measure the stability of the cell membrane, and the higher the REC value, the worse the cell membrane homeostasis [26,27]. The values of REC of HT-treated tree peony increased, indicating that high-temperature stress could lead to plasma membrane damage and decreased osmotic regulation ability, and our results were consistent with those of Wang et al. [22] and Zhang et al. [28]. MDA is the final product of membrane lipid peroxidation and can cause severe damage to biofilms. MDA can undergo a chain polymerization reaction with the enzyme protein, and the enzyme protein is therefore inactivated that causing plant damage. Similarly, the MDA content of tree peony leaves also increased significantly under HT-treated. MDA content was promoted by high-temperature stress. This was consistent with the report of P. ostii [29] under high-temperature stress, and other abiotic stresses, such as drought stress [30,31] and chilling stress [32].
Plants can spontaneously induce osmotic regulation and catalyze a series of antioxidant enzymes under high-temperature stress, thus removing excess ROS and alleviating the damage of high-temperature stress [33]. Antioxidative enzymes mainly include SOD, POD, CAT, and APX, which act synergistically against oxidative damage induced by stress [34]. The activity of antioxidant enzymes will increase when plants are under stress. In this study, the activities of SOD, POD, CAT, and APX all increased with the increase in treated temperature, and the activities of the 4 enzymes were the highest at 40°C, indicating that tree peony could reduce the damage caused by high temperature by enhancing the activity of antioxidant enzymes, and improve the ability to scavenge ROS, and this result was consistent with that of Amaranthus tricolor [35], lily [36] and cucumber [37]. Although the APX enzyme activity under MT was higher than that of CK, it was not statistically significant, which might be caused by the differences between samples. When the temperature rose to 40°C, the antioxidant enzyme activities of the 6 chrysanthemum varieties all dropped significantly to varying degrees, indicating that the high temperature at this time had exceeded the maximum that the practical chrysanthemum could withstand [38]. When the degree of stress reaches a certain level, the activity of antioxidant enzymes in plants will reach saturation, and continued stress will lead to a decrease in their activity. Similar conclusions have also been reported in honeysuckle [39] and marigold [40].
Photosynthesis is the basis of plant growth and development, and temperature changes often lead to changes in the ability of photosynthesis [41], and plant photosynthesis is inhibited by high-temperature stress [42]. Photosynthesis can be measured by the level of chlorophyll content. Under high-temperature stress, the chlorophyll content in the HT-treated leaf was significantly lower than that of CK and MT-treated. The chlorophyll content is not the only indicator for measuring photosynthesis. Photosynthetic parameters and chlorophyll fluorescence parameters also can be used to measure plants’ photosynthesis ability more intuitively. The physiological indices of the 3 groups of tree peony were significantly different on the 12th day of treatment. The photosynthesis of plants is mainly measured by the values of Pn, Ci, Gs, and Tr. Studies show that the photosynthesis of plants will change with the degree and time of stress [21,29,30]. The Pn, Ci, Gs, and Tr of tree peony were significantly decreased under HT-treated. This indicated that plants activated their defense mechanism by closing stomata under high-temperature stress, resulting in a downward trend in Pn and other indices. Chlorophyll fluorescence parameters are a set of variables or constant values used to describe the photosynthesis mechanism and photosynthetic physiological status of plants, which reflect the characteristics of plant ‘intrinsic’ and are regarded as intrinsic probes for studying the relationship between plant photosynthesis and the environment [43]. Fv/Fm is a parameter to measure the maximum light and efficiency of photosystem II, and Y(II) represents the actual light and efficiency of photosystem II. When plants are stressed, Fv/Fm decreases, and the decrease in Fv/Fm is an important indicator of photoinhibition [44]. qN and Y(NO) can reflect the strength of plant photoprotection to a certain extent. The result showed that Fv/Fm and Y(II) of tree peony decreased significantly when high-temperature stress occurred, then the light energy conversion efficiency of PSII of tree peony decreased significantly, which induced the imbalance between electron transport and photochemical activity, and finally seriously damaged chloroplasts, so the light energy conversion efficiency of peony decreased. The increase in Y (NO) and qN indicates the damage caused by excessive or strong light [45], which may be caused by the intense light accompanying the process of high-temperature stress on tree peony. Our study revealed the effect of high-temperature stress on photosynthesis in tree peony, which was consistent with previous studies [46–48].
In conclusion, this study revealed the response of tree peony to high-temperature stress through morphological observation, physiological index measurement, and ultrastructure observation. The changes in plant surface shape under high temperature directly reflect the damage of high-temperature stress on tree peony. The measurement of physiological indices and observation of ultrastructure also proved that high-temperature stress harms the osmotic regulation, ROS accumulation, and photosynthesis of tree peony. However, the increased activity of antioxidant enzymes also reflected the spontaneous protective effect of plants. In the middle and lower reaches of the Yangtze River and the southern region, protective measures should be taken as soon as possible, such as spraying exogenous substances and application of facilities, to reduce the damage caused by high temperature to tree peony to improve the yield and quality, even accelerating the promotion in the southern region, and laying a foundation for the improvement of high-temperature tolerance of tree peony.
Acknowledgement: None.
Funding Statement: This work was supported by Jiangsu Modern Agricultural Industrial Technology System (JATS [2022]489), Agricultural Science and Technology Independent Innovation Fund of Jiangsu Province (CX(22)3186), Policy Guidance Program of Jiangsu Province-Science and Technology Special Project of Northern Jiangsu Province (SZ-SQ2021041), the Qing Lan Project of Jiangsu Province and High-Level Talent Support Program of Yangzhou University.
Author Contributions: Conceptualization, Z.S. and D.Z.; methodology, D.Z.; software, Z.S.; validation, J.T. and D.Z.; formal analysis, Z.S.; investigation, Z.S.; resources, D.Z.; data curation, Z.S.; writing—original draft preparation, S.Z.; writing—review and editing, D.Z. and J.T.; visualization, Z.S. and D.Z.; supervision, D.Z. and J.T.; project administration, D.Z.; funding acquisition, D.Z. All authors have read and agreed to the published version of the manuscript.
Availability of Data and Materials: The data that support the findings of this study are available in the supplementary material of this article. For additional information, contact the corresponding author.
Ethics Approval: None.
Conflicts of Interest: The authors declare that they have no conflicts of interest to report regarding the present study.
References
1. Okunlola, G. O., Olatunji, O. A., Akinwale, R. O., Tariq, A. (2017). Physiological response of the three most cultivated pepper species (Capsicum spp.) in Africa to drought stress imposed at three stages of growth and development. Scientia Horticulturae, 224, 198–205. https://doi.org/10.1016/j.scienta.2017.06.020 [Google Scholar] [CrossRef]
2. Xalxo, R., Yadu, B., Chandra, J., Chandrakar, V., Keshavkant, S. (2020). Alteration in carbohydrate metabolism modulates thermotolerance of plant under heat stress. In: Heat stress tolerance in plants: Physiological, molecular and genetic perspectives. USA: John Wiley & Sons, Ltd. https://doi.org/10.1002/9781119432401.ch5 [Google Scholar] [CrossRef]
3. Hussain, H. A., Men, S. N., Hussain, S., Chen, Y. L., Ali, S. et al. (2019). Interactive effects of drought and heat stresses on morpho-physiological attributes, yield, nutrient uptake and oxidative status in maize hybrids. Scientific Reports, 9(1), 3890. https://doi.org/10.1038/s41598-019-40362-7 [Google Scholar] [PubMed] [CrossRef]
4. Mathur, S., Agrawal, D., Jajoo, A. (2014). Photosynthesis: Response to high-temperature stress. Journal of Photochemistry & Photobiology B: Biology, 137, 116–126. https://doi.org/10.1016/j.jphotobiol.2014.01.010 [Google Scholar] [PubMed] [CrossRef]
5. Hasanuzzaman, M., Nahar, K., Alam, M. M., Roychowdhury, R., Fujita, M. (2013). Physiological, biochemical, and molecular mechanisms of heat stress tolerance in plants. International Journal of Molecular Sciences, 14(5), 9643–9684. https://doi.org/10.3390/ijms14059643 [Google Scholar] [PubMed] [CrossRef]
6. Hu, S., Ding, Y., Zhu, C. (2020). Sensitivity and responses of chloroplasts to heat stress in plants. Frontiers in Plant Science, 11, 375. https://doi.org/10.3389/fpls.2020.00375 [Google Scholar] [PubMed] [CrossRef]
7. Ahmed, I. M., Cao, F. B., Zhang, M., Chen, X. H., Zhang, G. P. et al. (2013). Difference in yield and physiological features in response to drought and salinity combined stress during anthesis in Tibetan wild and cultivated barleys. PLoS One, 8(10). https://doi.org/10.1371/journal.pone.0077869 [Google Scholar] [PubMed] [CrossRef]
8. Farooq, M., Wahid, A., Kobayashi, N., Fujita, D., Basra, S. M. A. (2009). Plant drought stress: Effects, mechanisms and management. EDP Sciences, 29(1), 185–212. https://doi.org/10.1007/978-90-481-2666-8 [Google Scholar] [CrossRef]
9. Anjum, S. A., Farooq, M., Xie, X. Y., Liu, X. J., Ijaz, M. F. (2012). Antioxidant defense system and proline accumulation enables hot pepper to perform better under drought. Scientia Horticulturae, 140, 66–73. https://doi.org/10.1016/j.scienta.2012.03.028 [Google Scholar] [CrossRef]
10. Zhao, D. Q., Tang, Y. H., Hao, Z. J., Tao, J. (2015). Identification of flavonoids and expression of flavonoid biosynthetic genes in two coloured tree peony flowers. Biochemical & Biophysical Research Communications, 459(3), 450–456. https://doi.org/10.1016/j.bbrc.2015.02.126 [Google Scholar] [PubMed] [CrossRef]
11. Kim, Y. I., Lee, S. J., Huh, J., Lee, T. H. (2010). Effect of water extract of Paeonia Suffruticosa and Prunus Percica on anti-inflammation. The Journal of Herbal Formula Science, 18(1), 105–120. [Google Scholar]
12. Hong, D. Y., Pan, K. Y. (2008). A revision of the Paeonia suffruticosa complex (Paeoniaceae). Nordic Journal of Botany, 19(3), 289–300. https://doi.org/10.1111/j.1756-1051.1999.tb01115.x [Google Scholar] [CrossRef]
13. Ohama, N., Sato, H., Shinozaki, K., Yamaguchi Shinozaki, K. (2017). Transcriptional regulatory network of plant heat stress response. Trends in Plant Science, 22, 53–65. https://doi.org/10.1016/j.tplants.2016.08.015 [Google Scholar] [PubMed] [CrossRef]
14. Luan, Y. T., Tang, Y. H., Wang, X., Xu, C., Tao, J. et al. (2022). Tree peony R2R3-MYB transcription factor PsMYB30 promotes petal blotch formation by activating the transcription of the anthocyanin synthase gene. Plant and Cell Physiology, 63(8), 1101–1116. https://doi.org/10.1093/pcp/pcac085 [Google Scholar] [PubMed] [CrossRef]
15. Wang, X. W., Fan, H. M., Li, Y. Y., Sun, X., Sun, X. Z. et al. (2014). Analysis of genetic relationships in tree peony of different colors using conserved DNA-derived polymorphism markers. Scientia Horticulturae, 175, 68–73. https://doi.org/10.1016/j.scienta.2014.05.026 [Google Scholar] [CrossRef]
16. Wang, Q. Y., Zhu, J., Li, B., Li, S. S., Yang, Y. et al. (2023). Functional identification of anthocyanin glucosyltransferase genes: A Ps3GT catalyzes pelargonidin to pelargonidin 3-O-glucoside painting the vivid red flower color of Paeonia. Planta, 257(4). https://doi.org/10.1007/s00425-023-04095-2 [Google Scholar] [PubMed] [CrossRef]
17. Chen, X., Ye, C. Y., Yang, H. M., Ji, W., Xu, Z. et al. (2022). Callogenesis and plant regeneration in peony (Paeonia suffruticosa) using flower petal explants. Horticulturae, 8(5). https://doi.org/10.3390/horticulturae8050357 [Google Scholar] [CrossRef]
18. Tian, Y. F., Chang, K. K., Yang, F. F., Liu, J., Sun, C. et al. (2022). Effects of different cultivation modes on the quality and yield of cortex moutan of Paeonia ostii ‘Fengdan’. Molecular Plant Breeding. [Google Scholar]
19. Ji, W., Luo, H. Y., Song, Y. Q., Hong, E., Li, Z. J. et al. (2022). Changes in photosynthetic characteristics of Paeonia suffruticosa under high-temperature stress. Agronomy, 12(5). https://doi.org/10.3390/agronomy12051203 [Google Scholar] [CrossRef]
20. Xu, J. Q., Jin, J. J., Zhao, H., Li, K. L. (2019). Drought stress tolerance analysis of Populus ussuriensis clones with different ploidies. Journal of Forestry Research, 30(4), 1267–1275. https://doi.org/10.1007/s11676-018-0729-z [Google Scholar] [CrossRef]
21. Zhang, T. T., Tang, Y. H., Luan, Y. T., Cheng, Z. Y., Wang, X. X. et al. (2022). Herbaceous peony AP2/ERF transcription factor binds the promoter of the tryptophan decarboxylase gene to enhance high-temperature stress tolerance. Plant Cell and Environment, 45(9), 2729–2743. https://doi.org/10.1111/pce.14357 [Google Scholar] [PubMed] [CrossRef]
22. Wang, X. X., Fang, Z. W., Zhao, D. Q., Tao, J. (2021). Effects of high-temperature stress on photosynthetic characteristics and antioxidant enzyme system of Paeonia ostii. Phyton-International Journal of Experimental Botany, 91(3), 599–615. https://doi.org/10.32604/phyton.2022.017881 [Google Scholar] [CrossRef]
23. Bhattacharjee, S. (2014). Membrane lipid peroxidation and its conflict of interest: The two faces of oxidative stress. Current Science, 107(11), 1811–1823. [Google Scholar]
24. Zandalinas, S. I., Mittler, R., Balfagón, D., Arbona, V., Gómez-Cadenas, A. (2018). Plant adaptations to the combination of drought and high temperatures. Physiologia Plantarum, 162, 2–12. https://doi.org/10.1111/ppl.12540 [Google Scholar] [PubMed] [CrossRef]
25. He, Y., Liu, Y., Cao, W., Huai, M. F., Xu, B. G. et al. (2005). Effects of salicylic acid on heat tolerance associated with antioxidant metabolism in Kentucky bluegrass. Crop Science, 45(3), 988–995. https://doi.org/10.2135/cropsci2003.0678 [Google Scholar] [CrossRef]
26. Chen, X. M., Qiu, L. L., Guo, H. P., Wang, Y., Yuan, H. et al. (2017). Spermidine induces physiological and biochemical changes in southern highbush blueberry under drought stress. Brazilian Journal of Botany, 40, 841–851. [Google Scholar]
27. Liang, G. T., Bu, J. W., Zhang, S. Y., Jing, G., Zhang, G. et al. (2019). Effects of drought stress on the photosynthetic physiological parameters of Populus × euramericana ‘Neva’. Journal of Forestry Research, 30(2), 409–416. https://doi.org/10.1007/s11676-018-0667-9 [Google Scholar] [CrossRef]
28. Wang, X. B., Shi, X. H., Zhang, R. L., Zhang, K. J., Shao, L. M. et al. (2022). Impact of summer heat stress inducing physiological and biochemical responses in herbaceous peony cultivars (Paeonia lactiflora Pall.) from different latitudes. Industrial Crops and Products, 184. https://doi.org/10.1016/j.indcrop.2022.115000 [Google Scholar] [CrossRef]
29. Zhang, Q., Li, D. M., Wang, Q., Song, X. Y., Wang, Y. B. et al. (2021). Exogenous salicylic acid improves chilling tolerance in maize seedlings by improving plant growth and physiological characteristics. Agronomy, 11(7). https://doi.org/10.3390/agronomy11071341 [Google Scholar] [CrossRef]
30. Zhang, X., Li, Y., Wang, X. M., Peng, L. P., Liu, Z. A. et al. (2023). Overexpression of a novel F-box protein PsFFL1 from tree peony (Paeonia suffruticosa) confers drought tolerance in tobacco. Plant Growth Regulation, 101(1), 131–143. https://doi.org/10.1007/s10725-023-01007-y [Google Scholar] [CrossRef]
31. Wu, Y. Q., Li, T. T., Cheng, Z. Y., Zhao, D. Q., Tao, J. (2021). R2R3-MYB transcription factor PlMYB108 confers drought tolerance in herbaceous peony (Paeonia lactiflora Pall.). International Journal of Molecular Science, 22(21), 11884. https://doi.org/10.3390/ijms222111884 [Google Scholar] [PubMed] [CrossRef]
32. Shu, P., Li, Y. J., Xiang, L. T., Sheng, J. P., Shen, L. (2023). SlNPR1 modulates chilling stress resistance in tomato plant by alleviating oxidative damage and affecting the synthesis of ferulic acid. Scientia Horticulturae, 307. https://doi.org/10.1016/j.scienta.2022.111486 [Google Scholar] [CrossRef]
33. Suleman, P., Redha, A., Afzal, M., Al-Hasan, R. (2012). Temperature-induced changes of malondialdehyde, heatshock proteins in relation to chlorophyll fluorescence and photosynthesis in Conocarpus lancifolius (Engl.). Acta Physiologiae Plantarum, 35(4), 1223–1231. https://doi.org/10.1007/s11738-012-1161-1 [Google Scholar] [CrossRef]
34. Gadelha, C. G., Miranda, R., Alencar, N., Costa, J. H., Prisco, J. T. (2017). Exogenous nitric oxide improves salt tolerance during establishment of Jatropha curcas seedlings by ameliorating oxidative damage and toxic ion accumulation. Journal of Plant Physiology, 212, 69–79. https://doi.org/10.1016/j.jplph.2017.02.005 [Google Scholar] [PubMed] [CrossRef]
35. Sarker, U., Oba, S. (2018). Drought stress effects on growth, ROS markers, compatible colutes, phenolics, flavonoids, and antioxidant activity in Amaranthus triclolr. Applied Biochemistry and Biotechnology, 186(4), 999–1016. https://doi.org/10.1007/s12010-018-2784-5 [Google Scholar] [PubMed] [CrossRef]
36. Yin, H., Yi, C. M. (2008). Effects of short-term heat stress on oxidative damage and responses of antioxidant system in Lilium longiflorum. Plant Growth Regulation, 54(1), 45–54. https://doi.org/10.1007/s10725-007-9227-6 [Google Scholar] [CrossRef]
37. Amin, B., Atif, M. J., Wang, X., Meng, H., Ghani, M. I. et al. (2020). Effect of low temperature and high humidity stress on physiology of cucumber at different leaf stages. Plant Biology, 23(5), 785–796. https://doi.org/10.1111/plb.13276 [Google Scholar] [PubMed] [CrossRef]
38. Jia, Q., Xiang, Y., Luo, J. H. (2022). Physiological response and comprehensive evaluation of heat tolerance of practical chrysanthemum under high-temperature stress. Molecular Plant Breeding, 17. [Google Scholar]
39. Zhou, B. Q., Lu, H., Liu, F., Wang, X., Geng, Y. L. et al. (2019). Effects of different temperature stress on cell membrane permeability, active oxygen metabolism and accumulation of effective substances in Lonicera japonicea. Chinese Journal of Traditional Chinese Medicine, 44(18), 3935–3941. https://doi.org/10.19540/j.cnki.cjcmm.20190701.110 [Google Scholar] [PubMed] [CrossRef]
40. Tian, Z. G., Wang, F., Zhang, W. E., Zhao, X. M. (2011). Effects of heat stress on growth and physiology of marigold cultivars. Acta Horticulturae Sinica, 38(10), 1947–1954. https://doi.org/10.16420/j.issn.0513-353x.2011.10.015 [Google Scholar] [CrossRef]
41. Zhang, J., Li, T. L. (2005). Effects of sub-high temperature on photosynthesis and chloroplast ultrastructure of tomato in solar greenhouse. Acta Horticulturae Sinica, 32(4), 614–619. https://doi.org/10.16420/j.issn.0513-353x.2005.04.010 [Google Scholar] [CrossRef]
42. Sun, X. Y., Du, Z. M., Ren, J., Amombo, E., Hu, T. et al. (2015). Association of SSR markers with functional traits from heat stress in diverse tall fescue accessions. BMC Plant Biology, 15(1), 116. https://doi.org/10.1186/s12870-015-0494-5 [Google Scholar] [PubMed] [CrossRef]
43. Bolhar-Nordenkampf, H. R., Long, S. P., Baker, N. R., Oquist, G., Schreiber, U. et al. (1989). Chlorophyll fluorescence as a probe of the photosynthetic competence of leaves in the field: A review of current instrumentation. Functional Ecology, 3, 497–514. https://doi.org/10.2307/2389624 [Google Scholar] [CrossRef]
44. Wang, Y. Q. (2019). Response mechanism of different wheat cultivars to high temperature, drought and their combined stress (Master Thesis). Shandong Agricultural University, China [Google Scholar]
45. Kramer, D. M., Avenson, T. J., Edwards, G. E. (2004). Dynamic flexibility in the light reactions of photosynthesis governed by both electron and proton reactions. Trends in Plant Science, 9(7), 349–357. https://doi.org/10.1016/j.tplants.2004.05.001 [Google Scholar] [PubMed] [CrossRef]
46. Wu, Y. Q., Li, T. T., Cheng, Z. Y., Zhao, D. Q., Tao, J. (2021). R2R3-MYB transcription factor PlMYB108 confers drought tolerance in herbaceous peony (Paeonia lactiflora Pall.). International Journal of Molecular Science, 22(21), 11884. https://doi.org/10.3390/ijms222111884 [Google Scholar] [PubMed] [CrossRef]
47. Zhao, D. Q., Xia, X., Su, J. H., Wei, M. R., Wu, Y. Q. et al. (2019). Overexpression of herbaceous peony HSP70 confers high temperature tolerance. BMC Genomics, 20(1). https://doi.org/10.1186/s12864-019-5448-0 [Google Scholar] [PubMed] [CrossRef]
48. Zhao, D. Q., Luan, Y. T., Shi, W. B., Zhang, X. Y., Meng, J. S. et al. (2021). A paeonia ostii caffeoyl-CoA Omethyltransferase confers drought stress tolerance by promoting lignin synthesis and ROS scavenging. Plant Science, 303. https://doi.org/10.1016/j.plantsci.2020.110765 [Google Scholar] [PubMed] [CrossRef]
Cite This Article
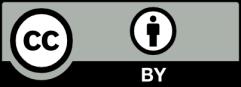