Open Access
ARTICLE
Effects of Root Cutting on Morphological Characteristics and Endogenous Hormone Levels of Quercus variabilis Seedlings
College of Forest, Henan Agricultural University, Zhengzhou, 450002, China
* Corresponding Authors: Jin’e Quan. Email: ; Xitian Yang. Email:
Phyton-International Journal of Experimental Botany 2023, 92(10), 2907-2920. https://doi.org/10.32604/phyton.2023.030689
Received 18 April 2023; Accepted 24 July 2023; Issue published 15 September 2023
Abstract
The objective of this study was to investigate the effects of root cutting stress on the dynamic changes of endogenous hormone content and growth characteristics of Quercus variabilis roots, and to explore the physiological role of endogenous hormones in regulating root-crown interactions in the short term. The morphological characteristics and endogenous hormone contents of normal roots (no root cutting, CK) and cut roots (cut by 1/3 of the length of the main root, RP) were determined by liquid chromatography, which was combined with mass spectrometry at different levels of different developmental stages. The results showed that the root growth indexes and root endogenous hormones in the RP group were superior to those in the CK group. Through comprehensive analysis of endogenous hormones, it was found that the crosstalk of IAA, JA, ABA and SA could activate the root growth defense. After the root cutting treatment, the root growth of Quercus variabilis seedlings could compensate for the inhibition of taproot growth by promoting lateral root growth. The growth and development of compensatory lateral roots contribute to the increase the total root length of plants, thus promoting the absorption of water and nutrients. It is speculated that plant hormones may be the key factors affecting their development, but this is not only related to the content of a single hormone, but more importantly, it is the interaction between various hormones.Keywords
Roots are the principal organ for water and nutrient absorption and the location for synthesizing different hormones, organic acids, and amino acids. They are vital in establishing rhizosphere effects as the interface between plants and soil. The effective ecological functions of ecosystems depend on their roots. Well-developed roots are crucial for cultivating high-quality vegetation restoration seedlings. Afforestation is an efficient method of forest restoration worldwide, particularly in degraded and damaged ecosystems [1]. Root pruning is carried out to facilitate the transplanting and transportation of seedlings. However, root pruning can cause significant damage to the young root tissues, adversely affecting nutrient absorption, seedling growth, and survival. The main reason for the low survival rate of afforestation is often poor root development [2]. Therefore, cultivating high-quality seedlings with well-developed roots is the top priority for nursery workers.
Studies on the relationship between transplanting or root cutting and plant root growth and development have gradually attracted the attention of researchers [3,4]. Root cutting inhibits seedling taproots growth and promotes lateral root development, improving seedling quality. The ideal roots are believed not to require excessively long taproots but relatively well-developed fibrous roots [5]. After root-cutting treatment, the taproot development is suppressed, and the number of lateral roots significantly increases, effectively improving water and nutrient absorption capacity [6]. Zhang et al. [7] conducted topping and root-cutting experiments on Chinese fir. They found that the survival rate of afforestation was related to the seedling diameter at the planting site. Yang et al. [8] found that after lateral root cutting, the main root of young Chinese juniper seedlings was lost. Also, the number and total length of primary lateral roots in the root-cutting-treated seedlings were greater than those of the entire root seedlings, and the position was closer to the root base. Therefore, root cutting effectively increases fruit tree yield [9,10] and improves the afforestation survival rate [11,12].
Quercus variabilis (Q. variabilis), also known as Chinese cork oak, is an essential regional vegetation in North, Northwest, South, and Southwest China. It is crucial for China’s timber, economy, fire prevention, and fuelwood forests. Also, it is essential for ecological security, water and soil conservation, conservation of water sources, and economic growth in the distribution regions [13]. Unfortunately, human activity has negatively influenced Q. variabilis resources in China, and many of the original forests of this species have turned into secondary forests [14]. As a result, forest productivity and ecological and economic advantages have decreased. Therefore, improving the quality of Q. variabilis seedlings, accelerating seedling cultivation, and afforestation are of great economic and environmental significance for sustainable development and the restoration of secondary forests.
There are two significant approaches to breeding Q. variabilis. The first is conventional afforestation via direct seeding, which needs ideal site conditions and is labor-intensive due to the need for inter-planting and replacement because the seeds are prone to insect and rodent damage. The second is seedling cultivation and transplantation. During the Q. variabilis seedling stage, the aboveground growth is slow. The underground portion of the plant has deep roots, with fewer lateral and fibrous roots and primary roots that develop quickly, which frequently leads to a low survival rate for afforestation and transplantation [15].
Transplanted seedlings usually have less established roots than planted seedlings, significantly contributing to secondary disasters like slope collapse in artificial afforestation. Prior studies compared seedling’s physiological indicators and chlorophyll fluorescence characteristics under different levels of root-cutting and water deficit to evaluate the response of Q. variabilis seedlings. Results indicated that proper root cutting was significant for coordinating the growth of various organs and optimizing the efficiency of roots. In particular, cutting off 1/3 of the main root length is the most favorable for the growth and development of Q. variabilis [16].
This study examined the effects of root cutting on the root structure and endogenous hormone content of 1-year-old Q. variabilis seedlings to determine the link between changes in root morphology and changes in endogenous hormone content during lateral root growth. A 1/3 root-cutting treatment (RP) and a no root-cutting control (CK) were used to compare the morphological changes of new lateral roots and the levels of eight endogenous hormones. These hormones were ABA, IAA, ZR, GA3, ACC, SA, JA and SL during the lateral root development. Also, we provided theoretical and practical relevance for creating a scientific vegetation restoration technology system and investigating the development and control mechanism of strong roots of afforestation tree species in arid areas.
The experimental site for cultivating 1-year-old Q. variabilis seedlings was located in Zhengzhou City, Henan Province, China (34°43'N, 113°42'E), in the third living area of the Science and Education Park of Henan Agricultural University. The site has a warm temperate continental monsoon climate with distinct seasons. The annual average temperature is approximately 14.2°C, with the lowest monthly average in January at around −0.2°C and the highest monthly average in July at around 27.3°C. The average annual precipitation, sunshine hours, and frost-free period are 640.90 mm, 2400 h, and 220 days, respectively.
2.2 Plant Materials and Experimental Design
The Q. variabilis seeds were collected from Huixian Forest Farm in Xinxiang City, with a germination rate of about 92%. The seeds were stored in a refrigerator at 4°C at the laboratory. Before sowing, the impurities on the surface of the seeds were removed by rinsing with water, and uniform and high-quality seeds were selected. The seeds were placed in a cool and ventilated place with a suitable temperature (20°C) for sand stratification. After sprouting, the uniform white seeds were sown in nutrient bowls (30 cm(H) × 15 cm(L) × 12 cm(W)) filled with sand, vermiculite, and perlite mixed in a volume ratio of 3:1:1. One seed was planted in each pot and thoroughly watered. Routine management was done in the later stage, and the growth of the seedlings was recorded.
During the experiment, pesticides and fertilizers were not used to avoid the effects of other uncertain factors on the experimental results. After cultivation, uniform and well-developed Q. variabilis seedlings of similar size were selected. The 150 seedlings that were chosen had an average main root length of 22.46 cm, a ground diameter of 2.95 mm, and a height of about 3.56 cm. The entire root system of the Q. variabilis seedlings was used for transplantation, and the main root was cut at a ratio of 1/3 of the length (RP), or no root cutting (CK) was performed as the control. Each treatment group had 20 seedlings in each of the three replicates. The scissors used for root cutting were disinfected with 75% ethanol, and the Q. variabilis seedlings were quickly transplanted back into plastic pots after root cutting.
On the 2nd day (new lateral root induction period), 12th day (new lateral root initiation and production period), and 25th day (new lateral root elongation period), 18 seedlings were collected from the root-cutting group and the whole root control group, respectively (3 seedlings were selected from each replicate in the treatment and control groups). The root samples of nine seedlings selected from each of the two treatment groups were collected immediately, wrapped with tin foil after collection and quickly put into the liquid nitrogen tank, and finally taken back to the laboratory to be stored in the ultra-low temperature refrigerator at −80°C, in preparation for hormone content determination. The remaining seedlings were stored in ice boxes ready for root morphology and biomass measurement. The root cutting group and the whole root control group were selected 3 roots for separate measurement. Therefore, three biological replicates were set for root morphological index determination, biomass determination, and hormone content determination.
2.3 Determination of Endogenous Hormone Content and Growth Indices
The root system of Q. variabilis seedlings was scanned using Epson Performance V 700 photo (Seiko Epson Corp, Suwa, Nagano, Japan) with a resolution of 300 dpi. The scanned images were analyzed by Winrshizo (Version Pro 2007D, Régent Instruments Inc., Qué bec, Canada) and the total root length, root surface area, root volume and primary lateral roots were obtained for each period.
After the impurities on the surface of Q. variabilis seedlings were washed away, they were wiped dry. The seedlings were divided into root, stem and leaf with scissors, and the fresh weights of the root, stem and leaf were weighed with a one-thousandth balance (Sartorius Scientific Instruments, Beijing, China) to obtain the aboveground biomass (the sum of the fresh weights of the stem and leaf) and total biomass (the sum of the fresh weights of the root, stem and leaf) of Q. variabilis seedlings, respectively. The root biomass ratio is the ratio of root biomass to total biomass.
Referring to Li et al. [17], a high performance liquid chromatography-tandem mass spectrometry (HPLC-MS/MS) method was used to determine the content of endogenous hormones (ABA, IAA, ZR, GA3, ACC, SA, JA, and SL) in the root system, with the unit ng/g fw (fresh weight). Each sample was repeated 3 times.
One-way analysis of variance (ANOVA) was performed using SPSS 25.0 software (IBM Corp., Armonk, NY, USA) for multiple comparisons, and significant differences were tested using the LSD test. The partial correlation analysis was used to evaluate the relationship between root indices and hormone content in RP group and CK. Origin 2021 (Originlab, Northampton, USA) was used for plotting graphs.
3.1 Effects of Root-Cutting Treatment on the Growth Characteristics of Q. variabilis Seedling Roots
3.1.1 Dynamic Changes in Lateral Root Morphology of Q. variabilis Seedlings after Root Cutting Treatment
Q. variabilis seedling’s main roots were cut at a ratio of 1/3 of their length (Fig. 1A), with the non-cutting treatment acting as the control (Fig. 1B). According to the morphological observation of new lateral roots, during the initiation and production period, the main root length of CK and RP Q. variabilis seedlings increased in the early stage compared to that of the control (Figs. 1C, 1D). In the root-cutting group, milky white new lateral roots were observed at the root-cutting site (Fig. 1D). During the elongation period of new lateral roots, the length, volume, and surface area of CK and RP increased significantly compared with the early stage (Figs. 1E, 1F). RP showed a larger root length, volume, and surface area than the control (Fig. 1F).
Figure 1: Dynamic changes of root morphology of Q. variabilis seedlings after root pruning treatment. I: Figs. A, C, and E were not root cut, Figs. B, D and F were treated with root cutting; II: A: Induction period of new lateral roots without root cutting; B: Pruning during the induction period of new lateral roots, 1/3 root cutting; C: No root cutting group, starting and producing new lateral roots; D: 1/3 root cutting group, producing more new lateral roots; E: Elongation period of new lateral roots without root cutting; F: 1/3 root cutting group has more and longer lateral roots during the elongation period of new lateral roots
3.1.2 Effects of Root Cutting Treatment on Lateral Root Morphology-Related Indices of Q. variabilis Seedlings
Root morphology, which focuses primarily on indices such as lateral root number, total root length, and root volume, can indicate the growing state of roots (Fig. 2). Roots are a crucial component that sustains the growth and development of seedlings. As shown in the first two figures, during the induction period of new lateral roots, the total length, the number of primary lateral roots of CK were slightly higher than those of RP. Still, there was no significant difference (p > 0.05). The initiation and production period of new lateral roots, the total root length and lateral root number of RP were significantly higher than those of CK (p < 0.05). When the new lateral root elongation period arrived, the total root length and lateral root number of the two groups increased significantly compared with the previous period, and the total root length and lateral root number of RP were 1.14 times and 1.32 times higher than those of CK, respectively. As shown in the last two figures, During the induction period of new lateral roots and the initiation and production period of new lateral roots, the root surface area and root volume of CK were similar to those of RP. In the new lateral root elongation stage, the root surface area and root volume of RP were 1.39 times and 2.04 times that of CK, respectively. Therefore, Q. variabilis seedlings regulated root morphology by increasing total root length, surface area, volume, and the number of primary lateral roots after root-cutting, promoting the formation of a solid root structure.
Figure 2: Changes of root morphological indexes of Q. variabilis seedlings after root pruning treatment Please include the note as part of the figure legend. I: Induction period of new lateral roots; II: Initiation and production period of new lateral roots; III: Elongation period of new lateral roots. Different capital letters above the bars indicate significant differences (p < 0.05) among different treatments in the same period, while the same capital letters indicate no significant difference (p > 0.05); Different lowercase letters indicate significant differences (p < 0.05) among the same treatments in different periods, while the same lowercase letters indicate no significant difference (p > 0.05). The bars represent standard errors (n = 3)
3.1.3 Effects of Root Cutting Treatment on Biomass Allocation of Q. variabilis Seedling Roots
Root biomass is a significant indicator of the quality of seedling roots as it can indicate the potential of seedling underground growth and development (Fig. 3). It can be seen from first three figures that there is no significant difference in root biomass, total biomass and aboveground biomass between CK and RP during the new lateral root induction period and the new lateral root initiation and production period (p > 0.05), but during the new lateral root elongation period, the root biomass, total biomass and aboveground biomass of RP are 1.31 times, 1.23 times and 1.07 times higher than CK, respectively. The last figure demonstrates that the root biomass of RP increased significantly (p < 0.05), and that of CK increased significantly at first (p < 0.05), and then did not change significantly (p > 0.05). There was no significant difference in the root biomass of RP compared with CK in the induction period of new lateral roots and the initiation and generation period of new lateral roots (p > 0.05), but it was significantly higher than CK after reaching the extension period of new lateral roots (p < 0.05). These results indicate that Q. variabilis seedlings can adjust the root-to-shoot ratio by increasing root biomass and biomass ratio after root-cutting damage, promoting the development of a compact root structure.
Figure 3: Changes in biomass allocation of Q. variabilis seedling roots after root pruning treatment
3.2 Effects of Root Cutting Treatment on Endogenous Hormone Levels in Q. variabilis Seedling Roots
As shown in Fig. 4A, the ABA content of CK remained constant (p > 0.05) during the whole process of new lateral root development, but the ABA content of RP decreased significantly (p < 0.05). The ABA content of RP reached a peak value of 29.61 ng·g−1 during the induction period of new lateral roots, which was 29.31 times that of CK in the same period. The ABA content of RP decreased rapidly during the initiation and production phase of new lateral roots, with a decrease of 29.33% compared to the previous period. However, it was still significantly higher than CK during the same period (p < 0.05). After reaching the elongation period of new lateral roots, the ABA content of RP continued to decrease and was similar to the level of ABA content in CK. Our findings suggest that ABA can inhibit the formation of new lateral roots in Q. variabilis seedlings. The increase in ABA content during the induction stage of new lateral roots might improve the stress tolerance of Q. variabilis seedlings in response to root-cutting damage.
Figure 4: Changes in endogenous hormone content in roots of Q. variabilis seedlings after treatment with root cutting
IAA concentration in CK demonstrated a slight upward trend throughout the development of new lateral roots. Still, IAA content in RP showed a significant upward trend, as shown in Fig. 4B (p < 0.05). The IAA content in RP was significantly higher than that in CK throughout the formation of new lateral roots, except for the induction phase (p < 0.05). During the induction period, root-cutting treatment significantly decreased the IAA content in Q. variabilis seedling roots (p < 0.05). However, in lateral root initiation and formation, the IAA content in RP significantly increased (p < 0.05), from 29.54 to 42.79 ng·g−1, with an increase of 44.9%. In the phase of lateral root elongation, the IAA content in RP reached a peak value of 52.64 ng·g−1, which was 27.2% higher than in CK. The findings suggest that appropriate root-cutting treatment can increase the IAA content during lateral root initiation, formation, and elongation, thereby promoting root development when combined with the changes in root morphology.
The ZR content in CK and RP showed an overall increasing and decreasing trend (Fig. 4C). Compared to CK, the root-cutting treatment decreased the roots’ ZR content during the lateral root induction phase (p > 0.05). The ZR content in RP decreased to a trough (0.51 ng·g−1) during the lateral root initiation and formation phase and was significantly lower than that in CK (p < 0.05). ZR content increased in both groups during the phase of lateral root elongation compared to the previous phase, with RP exhibiting a significantly higher ZR content than CK (p < 0.05). The ZR content was low during lateral root initiation and formation but high during elongation. According to the observations, lateral root formation requires a low level of ZR content to produce root primordia, whereas lateral root elongation requires an elevated level of ZR.
Fig. 4D shows an overall increasing trend followed by a downtrend for the content of GA3 in both RP and CK, but RP content was greater than CK. Root-cutting treatment significantly increased the GA3 content in the roots during the induction stage of new lateral roots compared to the control (p < 0.05). Q. variabilis seedlings benefited from the increased GA3 content in RP, which peaked at 1.12 ng. g−1 during the initiation and development stage of new lateral roots. The increase led to improved resistance, growth, and development of new lateral roots. During the elongation stage of new lateral roots, the GA3 content in both groups decreased with no significant differences (p > 0.05). GA3 can promote the synthesis of hydrolytic enzymes, which is conducive to the supply of nutrients, and prepares materials for the initiation and expression of lateral roots. The GA3 content in RP was higher throughout the new lateral root production, which promoted the initiation of new lateral roots.
As shown in Fig. 4E, the ACC content in RP remained stable during the lateral root production, while the ACC content in CK increased and then decreased. During the induction phase of new lateral roots, root-cutting treatment significantly increased the ACC content in the roots compared to CK (p < 0.05). The ACC content in RP slightly decreased compared to the prior period but was significantly higher than CK at the same period during the initiation and development stage of new lateral roots (p < 0.05). Also, the ACC content in RP and CK was not significantly different until the elongation stage of new lateral roots (p > 0.05). In the first two periods, the ACC content in RP was considerably higher than that in CK, which promoted the initiation of lateral roots.
The overall SA content in CK remained stable without significant changes (p > 0.05), while the SA content in RP showed a significant decrease (p < 0.05). The SA content in RP peaked at 29.61 ng·g−1 during the induction period of new lateral roots, which was 51.4% higher than in CK at the same period (p < 0.05). The SA concentration in RP decreased without significant differences after the initiation, development, and elongation phases of new lateral roots compared to CK (p > 0.05). Salicylic acid has various regulatory effects on plant growth and development and participates in plant stress responses. During the induction of new lateral roots, increased SA content in RP improved the stress resistance of Q. variabilis seedlings.
According to Fig. 4G, the JA content in CK did not change significantly (p > 0.05); however, the JA content in RP showed a significant declining trend (p < 0.05). The JA content in RP peaked at 187.54 ng·g−1 during the induction of new lateral roots, significantly higher than in CK during the same period. The JA content in RP decreased without significantly differing from CK during the initiation and development stage of new lateral roots (p > 0.05). During the elongation stage of new lateral roots, the JA content in RP decreased and was lower than CK at the same period, with no significant difference between the two groups (p > 0.05). The results indicated that Q. variabilis seedlings synthesized a large amount of JA in response to mechanical root damage, inhibiting root growth and maintaining the physiological activity of plants. The amount of JA in roots was reduced as a result of self-healing.
While the SL content in CK showed a slight upward trend, the SL content in RP demonstrated an increasing and decreasing pattern (Fig. 4H). During the induction period of new lateral roots, the SL content in RP was higher than that in CK, with no significant difference (p > 0.05). The SL content in RP peaked at 53.18 ng·g−1 during the initiation and development stage of new lateral roots, which was 2.49 times that of CK in the same period. The SL content in both groups did not differ significantly (p > 0.05) after new lateral roots had started to elongate; their respective content ranges were 18.05 and 20.86 ng.g−1. The increase in SL content in the first two developmental periods induced lateral root formation.
3.3 Effects of Root Cutting on the IAA/ABA, IAA/ZR Ratio in Q. variabilis Seedling Roots
As shown in Fig. 5A, the IAA/ABA values in the roots of Q. variabilis seedlings treated with root cutting increased over time. Those of the CK group initially decreased before increasing and reaching a minimum value during the initiation and formation of new lateral roots. The ABA content in the RP group increased significantly throughout the induction phase of new lateral roots, leading to a lower IAA/ABA ratio. The IAA/ABA ratio increased rapidly while the roots experienced self-repair and growth. Still, it remained lower than the CK group even though the IAA content increased and the ABA content decreased. The IAA and ABA content changes jointly regulated the growth and development of Q. variabilis seedling roots.
Figure 5: Alterations in IAA/ABA and IAA/ZR values in the roots of Q. variabilis seedlings following treatment with root cutting
The IAA/ZR values in both the RP and CK groups steadily rose over time, peaking during the initiation and development of new lateral roots and reaching a minimum during the induction phase of new lateral roots, as shown in Fig. 5B. A higher IAA/ZR ratio was beneficial for the production of lateral roots in Q. variabilis seedlings, as evidenced by the IAA/ZR value in the RP group being significantly higher than that in the CK group (p < 0.01) and the number of primary lateral roots in the RP group being significantly higher than that in the CK group (p < 0.05).
4.1 Q. variabilis Root Cutting Induced Compensatory Growth of Q. variabilis Seedlings
Roots play a key role in absorbing water and nutrients from the soil. By promoting the growth of lateral roots, the inhibition of taproot growth is compensated. The growth and development of compensatory lateral roots help to increase the total root length of the plant, thus promoting the absorption of water and nutrients. Many studies have shown that compensatory lateral root growth can be induced by root cutting. Root cutting is a suitable method to analyze the ability of compensatory root growth itself [18]. Kawai et al. [19] found that root cutting induced thicker lateral roots of rice, which compensated for root growth by generating higher-order branches. Appropriate root cutting treatment has a significant positive impact on root morphology construction, primarily by suppressing primary root growth, encouraging the formation of lateral roots, developing compact and well-formed fibrous roots, and ultimately achieving an optimal root state for robust seedling growth [20]. In the present study, we found that root cutting treatment accelerates the formation of new lateral roots and significantly increases the number of primary lateral roots, total root length, root surface area, and root volume of elongating Q. variabilis seedlings, which is beneficial for the formation of a well-developed and compact root structure.
Assessing seedling quality is no longer restricted to aboveground indicators, and there is a growing emphasis on evaluating the balance between aboveground and belowground components [21,22]. Seedlings adapt to environmental changes by changing the allocation of biomass to various tissues, which in turn affects seedling growth and transplant survival rate. Ma et al. [23] found that root cutting treatment can effectively improve the biomass and coordination of root-to-shoot ratio of Dalbergia odorifera container seedlings. The result of compensatory growth of plants under stress depends on the difference between the biomass accumulated by plants under stress and the amount of matter accumulated by normal growing plants. The experimental results revealed that root cutting treatment significantly enhanced both the root biomass and root biomass ratio of Q. variabilis seedlings compared to the control group. These findings are consistent with Liu et al. [24]. These results indicated that moderate root cutting promoted the compensatory growth mechanism of quercus seedlings and accelerated the accumulation of biomass in roots. The response of lateral roots to root cutting injury can adjust their root morphology structure, promote lateral root growth, increase the contact area between roots and the growing medium, and sufficiently enhance seedling root growth. By promoting the growth of Q. variabilis seedling roots, root cutting treatment also caused differences in the aboveground and belowground biomass, towing to the damage to the dominant apex of the seedling’s primary root, Stimulate the compensatory growth of quercus seedlings, which changes the consumption of internal nutrients of the seedling, thus altering biomass allocation to ensure the continuous accumulation of total biomass to adapt to environmental changes.
4.2 Effects of Root Cutting on Physiological and Biochemical Aspects of Q. variabilis Seedling Roots
4.2.1 Effects of Root Cutting on the Production of Endogenous Hormones Related to Lateral Root Formation
Plants usually face a balance between growth and development and defense functions. Under disturbance or stress conditions, this balance may be even more pronounced because plants need to devote more resources to defense, which reduces the investment in growth and development [25]. Plants respond to stress by regulating their own hormone levels [26–28]. The results of this experiment showed that the total root length, root surface area and root volume of quercus seedlings were significantly increased only in the late stage (new lateral root extension stage). Different from the time change of root growth, the hormone level of quercus sinensis roots changed significantly in the early stage of root cutting treatment (new lateral root induction stage). Therefore, root growth and development lag behind the physiological response of root to root cutting. Plant hormones such as abscisic acid, jasmonic acid and salicylic acid can respond to the stress response that plants participate in, and form a fine regulatory network with other plant hormones to improve plant stress resistance [29–32]. In this study, compared with the control group, the contents of ABA, JA, SA and ACC in the root cutting group were significantly increased during the induction period of new lateral roots, indicating that the root system of Quercus sinensis seedlings rapidly responds to the stress of root cutting by adjusting the contents of these hormones.
After root cutting, the formation of lateral roots is a complex process, which is jointly participated and regulated by a variety of endogenous hormones. Auxin is a key hormone in the formation of lateral roots [33]. Liu et al. [34] believed that the application of exogenous auxin could promote the formation of lateral root in rice to some extent, but excessive auxin could inhibit the formation of lateral root primordium. In this study, the content of IAA in the roots of Quercus quercus seedlings increased significantly during the initiation and generation of new lateral roots and the elongation stage, which may be attributed to the fact that root cutting treatment promoted IAA synthesis in the latter two stages, thus promoting the generation and development of new lateral roots, which can be demonstrated by the increase in the number of first-order lateral roots, total root length, root surface area and root volume after root pruning. Gibberellin can promote cell elongation and enlargement and inhibit senescence [35]. Zhao et al. [36] suggested that the increase of GA3 content could promote callus induction and adventitia root formation of Rhododendron strigilis. In this experiment, the GA3 content in the treatment group was always higher than that in the control group during the generation of new lateral roots, which may be attributed to the fact that the root cutting treatment increased the GA3 content in the root system of cork oak seedlings to a certain extent, promoted the hydrolysis of biological macromolecules such as starch, and further promoted the growth and development of lateral roots. Werner et al. [37] found that cytokinin had a negative regulatory effect on the occurrence of Arabidopsis lateral roots, and the number of Arabidopsis lateral roots increased significantly when CTK content decreased. In addition, application of exogenous auxin can counteract the inhibition of cytokinin on lateral root initiation and elongation in rice [38,39]. This study showed that the ZR content in the treatment group was low during the initiation and generation of new lateral roots, and a large number of new lateral roots occurred during this period, indicating that the lower ZR content was conducive to the initiation and development of new lateral roots.
4.2.2 Interactions of Endogenous Hormones on Lateral Root Formation
Different hormones do not act in isolation to regulate lateral root development; rather, they can jointly regulate certain signal transduction activities [40]. Yan [41] suggested that a high IAA/ZR and IAA/ABA ratio can promote lateral root formation. Guo et al. [42] reported a positive correlation between the IAA/ABA ratio and white pine rooting rate. In the experiment, the steady increase in IAA/ABA values was enhanced the induction and development of root primordia. A higher IAA and lower ZR level also induced the formation of lateral root primordia [43]. During the process of new lateral root formation in Q. variabilis seedlings, the IAA/ZR values of both groups increased during the initiation and production period of new lateral roots, which matched with the increase in IAA content and decrease in ZR content, both of which favored the formation of new lateral roots, similar to the conclusions drawn by Wang et al. [44]. The peak of IAA/ZR occurred during the critical period of new lateral root formation, and the peak of IAA/ZR in the root cutting group was higher, suggesting that a higher IAA/ZR ratio promoted the initiation of new lateral roots.
After root cutting treatment, Q. variabilis seedlings showed changes in root morphology, and this was likely influenced by plant hormones. However, this is not only related to the content of a single hormone but also the interaction between various hormones. The focus of this study was to explore the dynamic changes in endogenous hormone content in lateral roots of Q. variabilis seedlings at different developmental stages after root cutting treatment, which suggests that lateral root growth and development were regulated by endogenous hormones in the short term after root cutting stress. The crosstalk between IAA and JA, ABA, SA, and other defense hormones can activate root growth defense. Proper root pruning is an effective approach for promoting root growth and improve seedling survival rate. In addition, the regulatory mechanism of endogenous hormones on root growth and development needs to be further investigated.
Acknowledgement: Not applicable.
Funding Statement: This research was funded by National Natural Science Foundation of China, Grant Number 31700549 and China Postdoctoral Science Foundation Project, Grant Number 2017M612401.
Author Contributions: conceptualization, J.Q. and X.Y.; methodology, J.Q. and H.D.; software, R.N.; validation, R.N. and H.D.; formal analysis, S.B.; investigation, R.N. and H.D.; data curation, R.N.; writing—original draft preparation, R.N. and H.D.; writing—review and editing, J.Q.; supervision, T.W.; project administration, J.Q.; funding acquisition, J.Q. All authors have read and agreed to the published version of the manuscript.
Availability of Data and Materials: All data generated or analysed during this study are included in this published article and its supplementary information files.
Ethics Approval: None.
Conflicts of Interest: The authors declare that they have no conflicts of interest to report regarding the present study.
References
1. Yue, X., Zhang, T., Shao, C. (2021). Afforestation increases ecosystem productivity and carbon storage in China during the 2000s. Agricultural and Forest Meteorology, 296, 108227. https://doi.org/10.1016/j.agrformet.2020.108227 [Google Scholar] [CrossRef]
2. Fanello, D. D., Kelly, S. J., Bartoli, C. G., Cano, M. G., Martínez Alonso, S. et al. (2020). Plasticity of root growth and respiratory activity: Root responses to above-ground senescence, fruit removal or partial root pruning in soybean. Plant Science, 290, 110296. https://doi.org/10.1016/j.plantsci.2019.110296 [Google Scholar] [PubMed] [CrossRef]
3. Yamashita, H., Nishina, Y., Komori, N., Kamoshita, M., Oya, Y. et al. (2021). Cesium uptake and translocation from tea cutting roots (Camellia sinensis L.). Journal of Environmental Radioactivity, 235–236, 106655. https://doi.org/10.1016/j.jenvrad.2021.106655 [Google Scholar] [PubMed] [CrossRef]
4. Ortega, U., Majada, J., Mena-Petite, A., Sanchez-Zabala, J., Rodriguez-Iturrizar, N. et al. (2006). Field performance of Pinus radiata D. Don produced in nursery with different types of containers. New Forests, 31, 97–112. https://doi.org/10.1007/s11056-004-7364-6 [Google Scholar] [CrossRef]
5. Wu, W. (2022). Effect of root pruning on quality of Sapium sebiferum (L.) Roxb. container seedlings. Chinese Agricultural Science Bulletin, 38(3), 25–29. [Google Scholar]
6. Yan, M., Zhang, C., Li, H., Zhang, L., Ren, Y. et al. (2023). Root pruning improves maize water-use efficiency by root water absorption. Frontiers in Plant Science, 13, 1023088. https://doi.org/10.3389/fpls.2022.1023088 [Google Scholar] [PubMed] [CrossRef]
7. Zhang, K. L., Xia, Y. F., Yang, Y. Z. (2013). Effects of cutting root and truncating on root growth of Broussonetia papyrifera nursery-grown seedlings. Journal of Central South University, 33(6), 84–87. [Google Scholar]
8. Yang, X. T., Wang, G. L., Zhao, N., Fan, Z. W. (2010). Effects of different root-cutting treatments on seedling lateral root development. Journal of Henan Agricultural University, 44(2), 155–159. https://doi.org/10.16445/j.cnki.1000-2340.2010.02.018 [Google Scholar] [CrossRef]
9. Thomas, P., Ravindra, M. B. (1997). Effect of pruning or removal of in vitro formed roots on ex vitro root regeneration and growth in micropropagated grapes. Plant Cell Tissue & Organ Culture, 51(3), 177–180. https://doi.org/10.1023/A:1005928615179 [Google Scholar] [CrossRef]
10. Ma, S. C., Xu, B. C., Li, F. M., Huang, Z. B. (2009). Effect of root pruning on root efficiency, water use and yield of winter wheat. Chinese Journal of Applied and Environmental Biology, 15(5), 606–609. https://doi.org/10.3724/SP.J.1145.2009.00606 [Google Scholar] [CrossRef]
11. Lin, Z. P. (2004). Effects on growth of nursery stock and young crop of pinus massoniana by cutting roots when growing seedlings. Journal of Southwest Forestry University, 4, 196–199. [Google Scholar]
12. Deng, J. Y., Liu, Y. F., Pu, T. H., Liu, X. L. (2004). Experiment on root-cutting cultivation of annual seedling of Pinus thunbergii. Journal of Jiangsu Forestry Science & Technology, 4, 196–199. [Google Scholar]
13. Wu, M. Z. (1998). Research progress of Quercus variabilis. Shanxi Forestry Science and Technology, 4, 65–69. [Google Scholar]
14. Zhou, J. Y., Lin, J., He, J. F., Zhang, W. H. (2010). Research progress and future prospect of Quercus variabilis. Journal of Northwest Forestry University, 25(3), 43–49. [Google Scholar]
15. Liu, Y. J., Wang, Y. L., Yang, H. X. (2010). Study on root control seedling raising technology of Quercus variabilis. Forest Inventory and Planning, 35(3), 25–26. [Google Scholar]
16. Zhao, J. B. (2016). Response of Quercus variabilis seedlings to root-cutting and water deficit. China: Henan Agricultural University. [Google Scholar]
17. Li, Y., Zhou, C. X., Yan, X. J., Zhang, J. R. (2016). Simultaneous analysis of ten phytohormones in Sargassum horneri by high-performance liquid chromatography with electrospray ionization tandem mass spectrometry. Journal of Separation Science, 39(10), 1804–1813. https://doi.org/10.1002/jssc.201501239 [Google Scholar] [PubMed] [CrossRef]
18. Correa, J., Postma, J. A., Watt, M., Wojciechowski, T. (2019). Soil compaction and the architectural plasticity of root systems. Journal of Experimental Botany, 70(21), 6019–6034. https://doi.org/10.1093/jxb/erz383 [Google Scholar] [PubMed] [CrossRef]
19. Kawai, T., Chen, Y., Takahashi, H., Inukai, Y., Siddique, K. H. M. (2022). Rice genotypes express compensatory root growth with altered root distributions in response to root cutting. Frontiers in Plant Science, 13, 830577. https://doi.org/10.3389/fpls.2022.830577 [Google Scholar] [PubMed] [CrossRef]
20. Liu, F., Huang, R. L., Jiang, S. H., Jiang, Y., Jiang, Y. (2020). Study on growth law of Keeleria jiangnan plantation. Journal of Central South University of Forestry & Technology, 40(3), 39–52. https://doi.org/10.14067/j.cnki.1673-923x.2020.03.006 [Google Scholar] [CrossRef]
21. Li, X., Shen, Y. B., Zhu, W. J., Li, H. F., Shi, Z. H. (2019). Effects of different bud cutting intensity on the growth of container seedlings of Phoebe sheareri. Journal of Northwest Forestry University, 47(11), 17–22. https://doi.org/10.13759/j.cnki.dlxb.2019.11.004 [Google Scholar] [CrossRef]
22. Bin, Y. M., Su, X., Li, L., Zhao, Y. Q., Ma, J. Y. et al. (2015). Effect of different seedling cutting and raising treatments on growth of Cercis chinensis. Guangxi Forestry Science, 44(1), 63–66. https://doi.org/10.19692/j.cnki.gfs.2015.01.012 [Google Scholar] [CrossRef]
23. Ma, Y., Liu, Z. L., Jia, H. Y., Meng, C. L., Li, L. L. (2016). Effect of seedling cutting root on the growth of container seedling of Dalbergia odorifera. Forest Science and Technology, 3, 35–37. https://doi.org/10.13456/j.cnki.lykt.2016.03.011 [Google Scholar] [CrossRef]
24. Liu, W. J., Liu, S. H., Xin, F. M., Zhao, K. T. (2021). Response of growth and physiological changes of Sophora moorcroftiana seedlings to root cutting intensity. Journal of Zhejiang University (Agriculture and Life Sciences), 47(2), 243–250. https://doi.org/10.3785/j.issn.1008-9209.2020.06.051 [Google Scholar] [CrossRef]
25. Jiao, L., Cai, X. M., Bian, L., Luo, Z. X., Li, Z. Q. et al. (2018). Jasmonates: From induced anti-insect defense responses in plants to growth-defense tradeoffs. Journal of Applied Ecology, 29(11), 3876–3890. https://doi.org/10.13287/j.1001-9332.201811.030 [Google Scholar] [CrossRef]
26. Han, Y. C. (2022). Comparative transcriptomic analysis of root development of Platyclus orientalis seedlings induced by root cutting. China: Henan Agricultural University, https://doi.org/10.27117/d.cnki.ghenu.2022.000622 [Google Scholar] [CrossRef]
27. Yang, R. R., Li, X. S., Liang, Y. Q., Zhang, D. Y. (2022). Research progress on the mechanism of plant hormones in moss growth and development and stress response. Acta Botanica Sinica, 42(3), 527–540. https://doi.org/10.7606/j.issn.1000-4025.2002.03.0527 [Google Scholar] [CrossRef]
28. Feng, Z. P. (2020). Physiological and ecological response of Platyclus orientalis seedlings to root cutting and proteomic analysis. China: Henan Agricultural University. https://doi.org/10.27117/d.cnki.ghenu.2020.000121 [Google Scholar] [CrossRef]
29. Men, X. Y. (2021). Study on the developmental mechanism of Ginkgo biloba basal vertical milk based on hormones and multiple omics. China: Shandong Agricultural University. https://doi.org/10.27277/d.cnki.gsdnu.2021.000003 [Google Scholar] [CrossRef]
30. Suyala, Q. Q. G., Fan, M. S., Jia, L. G., Chen, Y. Z., Zhao, M. L. et al. (2020). Effects of soil PH on tuber development and endogenous hormone content of potato. Soil Bulletin, 5, 6. https://doi.org/10.19336/j.cnki.trtb.2020.05.20 [Google Scholar] [CrossRef]
31. Wu, J. G., Wang, P., Xie, L. Y., Lin, Q. Y., Wu, Z. J. et al. (2010). Effects of rice dwarf virus on the contents of three endogenous hormones and the transcription levels of metabolism-related genes. Journal of Plant Pathology, 2, 8. https://doi.org/10.13926/j.cnki.apps.2010.02.004 [Google Scholar] [CrossRef]
32. Cui, X. X. (2012). The role of lectin receptor protein kinase gene OsLecRK1 in stress resistance in rice. China: Xiamen University. [Google Scholar]
33. Fukaki, H., Tasaka, M. (2009). Hormone interactions during lateral root formation. Plant Molecular Biology, 69(4), 437–449. https://doi.org/10.1007/s11103-008-9417-2 [Google Scholar] [PubMed] [CrossRef]
34. Liu, D. T., Jing, Y. P., Li, D. L., Yu, X. R., Wang, Z. (2013). Research advances in plant lateral root development. Journal of Plant Physiology, 49(11), 1127–1137. [Google Scholar]
35. Li, S. Q. (2020). Effects of nitrogen and gibberellin on growth and leaf nutrient status of Dalbergia japonica. China: Chinese Academy of Forestry. https://doi.org/10.27625/d.cnki.gzlky.2020.000164 [Google Scholar] [CrossRef]
36. Zhao, Y. L., Chen, X., Li, C. C. (2013). Physiological and biochemical analysis of Rhododendron strigata during rooting from cuttings. Journal of Forestry science, 49(6), 45–51. https://doi.org/10.11707/j.1001-7488.20130607 [Google Scholar] [CrossRef]
37. Werner, T., Motyka, V., Laucou, V., Smets, R., Van Onckelen, H. et al. (2003). Cytokinin-deficient transgenic Arabidopsis plants show multiple developmental alterations indicating opposite functions of cytokinins in the regulation of shoot and root meristem activity. The Plant Cell, 15(11), 2532–2550. https://doi.org/10.1105/tpc.014928 [Google Scholar] [PubMed] [CrossRef]
38. Li, X., Mo, X., Shou, H., Wu, P. (2006). Cytokinin-mediated cell cycling arrest of pericycle founder cells in lateral root initiation of Arabidopsis. Plant & Cell Physiology, 47(8), 1112–1123. https://doi.org/10.1093/pcp/pcj082 [Google Scholar] [PubMed] [CrossRef]
39. Márquez, G., Alarcón, M. V., Salguero, J. (2019). Cytokinin inhibits lateral root development at the earliest stages of lateral root primordium initiation in maize primary root. Journal of Plant Growth Regulation, 38, 83–92. https://doi.org/10.1007/s00344-018-9811-1 [Google Scholar] [CrossRef]
40. Benková, E., Hejátko, J. (2009). Hormone interactions at the root apical meristem. Plant Molecular Biology, 69, 383–396. https://doi.org/10.1007/s11103-008-9393-6 [Google Scholar] [PubMed] [CrossRef]
41. Yan, X. L. (2007). Principles and applications of root biology. China: Science Press. 73–115. [Google Scholar]
42. Guo, S. J., Ling, H. Q., Pan, W. C., Li, F. L. (2004). Relationship between rooting characteristics and anatomical structure of cuttings of Pinus bungeana. Journal of Beijing Forestry University, 26(5), 43–46. https://doi.org/10.13332/j.1000-1522.2004.05.010 [Google Scholar] [CrossRef]
43. Duan, L. S., Zhang, Y., He, Z. P., Zhai, Z. X. (2001). Regulation of auxin and cytokinin on root apical dominance in cotton seedlings. Journal of Cotton Science, 3(6), 330–333. [Google Scholar]
44. Wang, Q., Zhang, J., Zhong, C. L., Zhang, Y., Wei, Y. C. et al. (2020). Changes of endogenous hormones and nutrients in the rooting process of Chukrasia tabularis cutting. Journal of Central South University, 40(4), 111–119. https://doi.org/10.14067/j.cnki.1673-923x.2020.04.015 [Google Scholar] [CrossRef]
Cite This Article
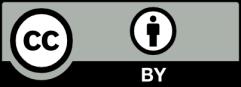