Open Access
ARTICLE
Effects of Ultrasonic Seed Treatment on Rice Performances under the Seawater Irrigation
1 State Key Laboratory for Conservation and Utilization of Subtropical Agricultural Bioresources, South China Agricultural University, Guangzhou, 510642, China
2 Scientific Observing and Experimental Station of Crop Cultivation in South China, Ministry of Agriculture, Guangzhou, 510642, China
3 Guangzhou Key Laboratory for Science and Technology of Aromatic Rice, Guangzhou, 510642, China
4 Golden Rice Agricultural Science & Technology Co., Ltd., Guangzhou, 510642, China
5 College of Coastal Agricultural Sciences, Guangdong Ocean University, Zhanjiang, 524088, China
* Corresponding Author: Xiangru Tang. Email:
Phyton-International Journal of Experimental Botany 2023, 92(1), 121-130. https://doi.org/10.32604/phyton.2022.022697
Received 22 March 2022; Accepted 10 May 2022; Issue published 06 September 2022
Abstract
Irrigation with desalinated seawater is an effective way to use ocean resources and save freshwater resources. However, seawater irrigation would cause yield loss of rice. In order to explore the effects of ultrasonic seed treatment on rice performances under seawater irrigation, the present study was conducted with three irrigation treatments (fresh water (SW0), ten times diluted seawater (SW1%, 0.34% salinity), and five times diluted seawater (SW2%, 0.68% salinity)) and two seed treatments (ultrasonic treated seeds (UT) and untreated seeds (CK)). Compared with SW0 + CK treatment, SW1 + CK and SW2 + CK treatments significantly decreased grain yield by 56.19% and 66.69%, spikelets per panicle by 30.11% and 55.80%, seed-setting rate by 23.05% and 18.87%, and 1000-grain weight by 4.55% and 14.50%, respectively. Seawater irrigation also significantly increased malonaldehyde (MDA) and proline contents and the activities of superoxide dismutase (SOD) and peroxidase (POD). Ultrasonic seed treatment significantly increased the grain number per panicle, seed-setting rate, and grain yield of rice under seawater irrigation. Compared with CK, UT treatment substantially reduced MDA content, SOD activity, and POD activity in SW1 and SW2 conditions. Furthermore, UT treatment significantly increased proline content and down-regulated proline dehydrogenase activity under seawater irrigation. We deduced that ultrasonic seed treatment enhanced the salinity tolerance of rice by inducing the proline accmulation. Our findings indicated that ultrasonic seed treatment could an effective strategy to promote rice productivity under seawater irrigation.Keywords
Rice (Oryza sativa L.) is the world’s most important staple food, providing over 3.5 billion people with 20% of their daily calories, 13% of their protein, and 19% of their energy needs worldwide [1,2]. As a water-intensive crop, rice requires a large amount of water during growing [3]. The study by Tolomio et al. [4] showed that rice production consumes about 80% of the total irrigated water resources in Asia. However, water scarcity is a major issue in rice production, and growing water demands from cities and businesses have exacerbated this problem [5]. Hence, water-saving irrigation methods with the capacity to reduce water use while maintaining or increasing yields to sustain a growing population are critical to lessen the possibility of a rice water shortage.
Seawater irrigation agriculture is an irrigation method that uses seawater and seawater mixed freshwater or underground saltwater for crops with resistance to saline. In 2008, Zhao et al. [6] demonstrated that irrigation with various dilutions of seawater could serve as an alternative water source, helping to save freshwater resources while also increasing agriculture in coastal semi-arid areas. The study by Shaffer et al. [7] also showed that irrigation with desalinated seawater for crop production would play a significant role in meeting rising water demands in water-scarce areas. Developing seawater irrigation agriculture is an effective way to use ocean resources and save freshwater resources. However, it is difficult to apply seawater irrigation in rice production because rice is not a crop with high tolerance to salinity [8]. Seawater irrigation also would cause rice yield loss even with desalination [9]. Therefore, improving rice productivity under seawater irrigation through agronomic techniques is necessary.
Ultrasound is defined as sound wave energy used in various fields due to its high efficiency, repeatability, and versatility [10]. The ultrasonic technique was used to accelerate seed germination and improve plant growth. The previous study of our team has proved that ultrasonic seed treatment can promote the yield formation and physiological properties of rice plants under cadmium stress [11]. Peroxidase (POD), catalase (CAT), and superoxide (SOD) are important antioxidative enzymes in quenching reactive oxygen, and proline helps in maintaining cellular structures and functions when plants face abiotic stress [12]. Earlier studies have shown that ultrasonic seed treatment could enhance antioxidative enzymatic activities in terms of SOD, POD, and CAT. For example, the study by Rao et al. [10] revealed that ultrasonic seed treatment substantially enhanced the activities of antioxidant enzymes such as superoxide dismutase (SOD) and catalase (CAT) in rice under lead stress. The study by Wang et al. [13] also showed that ultrasonic waves could stimulate seed germination and promote plant growth. Considering the enhancement of ultrasonic seed treatment in the growth and antioxidant response of rice plants, we deduced that ultrasonic seed treatment might improve rice performance under seawater irrigation. Therefore, in the present study, we conducted a pot experiment to explore the effects of ultrasonic seed treatment on rice performances under seawater irrigation. Our findings would provide new information for seawater irrigation agriculture and the ultrasonic application in rice production.
2.1 Plant Materials, Growth Conditions, and Experiment Design
The pot experiment was carried out in the greenhouse of the Experimental Research Farm, College of Agriculture, South China Agricultural University, Guangzhou, China (23°09 N, 113°22 E) during March–July, 2021. The seeds of the rice cultivar, RS1, bred and provided by Guangdong Ocean University, were used as plant material. Two seed treatments and three irrigation treatments were adopted in the experiment. For ultrasonic seed treatment (UT), the seeds were put into a tunnel ultrasonic processor (5ZCG-T6, Golden Rice Agricultural Science & Technology Co., Ltd., Guangzhou, China, as shown in Fig. 1) in a stainless-steel plate, treated at 20–40 kHz frequency for 2.0 min at room temperature. The seeds without ultrasonic treatment were taken as control (CK). After germination, both seeds were sown into a plastic tray containing paddy soil for nursery raising, and 20-days-old seedlings were transplanted to pots filled with paddy soil (six seedlings per hill and five hills per pot). Ten pots were for each treatment. For irrigation treatments, three water, i.e., freshwater (SW0), ten times diluted seawater (SW1), and five times diluted seawater (SW2) were used for irrigation during the whole growth period after the transplanting, respectively. The seawater was collected at the seaside, Nansha District, Guangzhou, Guangdong, China (22°80 N, 113°53 E) with 3.4% salinity.
Figure 1: Tunnel ultrasonic processor (5ZCG-T6, Golden Rice Agricultural Science & Technology Co., Ltd., Guangzhou, China)
2.2 Determination of Physio-Biochemical Traits
At the heading stage, thirty random flag leaves were separated from the main plants from each treatment, washed with double distilled water, and stored at −80°C till physio-biochemical analysis. The POD activity was determined according to the methods of Basit et al. [14]. The enzyme extract After reacting with 0.3% hydrogen peroxide and 0.2% guaiacol, the absorbance was read at 470 nm. The POD activity was expressed as U g−1 min−1. The estimation of SOD and CAT activities was carried out according to the methods of Dwivedi et al. [15] and expressed as U g−1 min−1. The determination of MDA content was carried out according to the methods of Mostofa et al. [16]. After reacting with thiobarbituric acid at a boiling water bath for 20 min, the absorbance of the supernatant was read at 532 nm, 600 nm, and 450 nm. The final result was expressed as μmol g−1. The determination of proline was carried out according to the methods of Li et al. [17], using sulfosalicylic for extraction and acidic-ninhydrin for chromogenic reaction. The absorbance was read at 520 nm, and proline content was expressed as μg g−1. The activity of PDH was determined according to the methods of Ncube et al. [18]. The reaction mixture contained 15 mM L-proline, 0.01 mM cytochrome c, 0.1 M phosphate buffer, 0.5% (v/v) Triton X-100, and the enzyme extract. The absorbance was read at 440 nm, and the final result was expressed as U g−1 h−1. The determination of P5CS was carried out according to the methods of Sanchez et al. [19]. The reaction mixture contained 50 mM L-glutamate, 20 mM magnesium chloride, 10 mM adenosine triphosphate, and 100 mM hydroxamate-HCl. The absorbance was read at 535 nm, and the final result was expressed as U g−1 h−1. The determination of OAT was carried out according to the methods of Luo et al. [20]. The absorbance was read at 440 m, and the final result was expressed as U g−1 h−1.
2.3 Determination of Yield and Yield-Related Traits
At maturity, three random pots in each treatment were harvested and threshed manually. After sun drying to adjust the grain moisture to 14%, the grain yield was weight and measured. At maturity, the plants from the other three random pots in each treatment were collected to count and measure the effective panicle number per pot, spikelets per panicle, seed-setting rate, and 1000-grain weight.
Analysis of variance was performed with Statistix 8.1 (Analytical Software, Tallahassee, FL, USA), and the means of treatments were compared based on the least significant difference (LSD) test at the 0.05 probability level. The figures were made using SigmaPlot 12.5 (Systat Software Inc., California, USA).
3.1 Grain Yield and Yield-Related Traits
The analysis of variance showed that ultrasonic seed treatment had significant effects on spikelets per panicle, seed-setting rate, and grain yield, and seawater irrigation significantly affected effective panicle number, spikelets per panicle, seed-setting rate, 1000-grain weight, and grain yield (Table 1). Compared with SW0 + CK treatment, SW1 + CK and SW2 + CK treatments significantly decreased grain yield by 56.19% and 66.69%, respectively. Lower spikelets per panicle, seed-setting rate, and 1000-grain weight were recorded in SW1 + CK and SW2 + CK treatments than SW0 + CK treatment. The negative effects due to seawater irrigation on the yield formation of rice were alleviated by ultrasonic seed treatment. In comparison with SW1 + CK treatment, SW1 + UT treatment substantially increased spikelets per panicle, seed-setting rate, and grain yield by 20.44%, 30.22%, and 44.58%, respectively. Similarly, in comparison with SW2 + CK treatment, SW2 + UT treatment substantially increased spikelets per panicle and grain yield by 86.25% and 35.79%, respectively. There was no significant difference between SW1 + CK and SW1 + UT treatments in 1000-grain weight, and a similar trend was observed between SW2 + CK and SW2 + UT treatments. The analysis of variance also showed that the interaction between ultrasonic seed treatment and seawater irrigation had significant effects on spikelets per panicle, seed-setting rate, and grain yield.
SW0: Irrigation with freshwater (SW0); SW1: irrigation with ten times diluted seawater; SW2: irrigation with five times diluted seawater; UT: ultrasonic seed treatment; CK: without ultrasonic treatment. Data represent the mean of data ± standard error. The different letter above the table indicates difference at P ≤ 0.05 by LSD tests. U: ultrasonic seed treatment; SW: seawater treatment; ns represents not significant at the 0.05 probability level; * and ** represent significance at the 0.05 and 0.01 probability level, respectively.
3.2 Antioxidant Responses and MDA Content
Ultrasonic seed treatment and water irrigation substantially affected the activities of SOD, CAT, POD, and MDA content (Fig. 2). Compared with SW0 + CK treatment, SW1 + CK and SW2 + CK treatments induced the up-regulation of SOD and POD activities. SW1 + CK and SW2 + CK treatments significantly increased MDA content by 38.74% and 75.83%, respectively. However, there was no significant difference among SW0 + CK, SW1 + CK, and SW2 + CK treatments. Compared with SW1 + CK and SW2 + CK treatments, SW1 + UT and SW2 + UT treatments significantly reduced the MDA content. Lower SOD, CAT, and POD activities were recorded in SW1 + UT and SW2 + UT treatments than SW1 + CK, and SW2 + CK treatments.
Figure 2: Effects of ultrasonic seed treatment and seawater irrigation on SOD, CAT, POD activities, and MDA contents. SW0: irrigation with freshwater (SW0); SW1: irrigation with ten times diluted seawater; SW2: irrigation with five times diluted seawater; UT: ultrasonic seed treatment; CK: without ultrasonic treatment. Means sharing a common letter do not differ significantly at P ≤ 0.05 according to the least significant difference (LSD) test
As shown in Fig. 3, ultrasonic seed treatment and water irrigation significantly affected the proline content. Compared with SW0 + CK treatment, SW1 + CK and SW2 + CK treatments significantly increased proline content by 86.12% and 140.23%, respectively. Compared with SW1 + CK treatment, SW1 + UT treatment significantly increased proline content by 15.92%. Compared with SW2 + CK treatment, SW2 + UT treatment significantly increased proline content by 45.03%.
Figure 3: Effects of ultrasonic seed treatment and seawater irrigation on proline content. SW0: irrigation with freshwater (SW0); SW1: irrigation with ten times diluted seawater; SW2: irrigation with five times diluted seawater; UT: ultrasonic seed treatment; CK: without ultrasonic treatment. Means sharing a common letter do not differ significantly at P ≤ 0.05 according to the least significant difference (LSD) test
3.4 OAT, PDH, and P5CS Activities
The activities of enzymes, i.e., OAT, PDH, and P5CS, which are related to proline metabolism, were shown in Fig. 4. For OAT activity, compared with SW0 + CK treatment, SW1 + CK and SW2 + CK treatments significantly enhanced OAT activities by 24.75% and 27.70%, respectively, while lower activities were recorded in SW1 + UT and SW2 + UT treatments than SW1 + CK and SW2 + CK treatments. For PDH activity, compared with SW0 + CK treatment, SW1 + CK and SW2 + CK treatments significantly enhanced PDH activities by 24.69% and 76.40%, respectively. Lower PDH activity was recorded in SW1 + UT treatment compared with SW1 + CK treatment, and a similar trend was observed between SW2 + UT and SW2 + CK treatments. For P5CS activity, the activities in SW1 + CK and SW2 + CK treatments were higher than SW0 + CK treatment. 2.54% higher P5CS activity was recorded in SW1 + UT treatment than SW1 + CK treatment. There was no significant difference between SW2 + UT and SW2 + CK treatments.
Figure 4: Effects of ultrasonic seed treatment and seawater irrigation on OAT, PDH, and P5CS activities. SW0: Irrigation with freshwater (SW0); SW1: irrigation with ten times diluted seawater; SW2: irrigation with five times diluted seawater; UT: ultrasonic seed treatment; CK: without ultrasonic treatment. Means sharing a common letter do not differ significantly at P ≤ 0.05 according to the least significant difference (LSD) test
The present study revealed the effects of ultrasonic seed treatment on yield formation, antioxidant responses, and proline metabolism of rice under seawater irrigation. First of all, our results showed that irrigation with desalinated seawater caused severe yield loss, and the loss was aggravated with the increase of seawater concentration. The results were consistent with the study by Marcos et al. [21]. Our data indicated that seawater irrigation disturbed the panicle differentiation and grain-filling process to induce yield loss. Compared with control, seawater irrigation substantially decreased spikelets per panicle, seed-setting rate, and 1000-grain weight, while the effective panicle had not been significantly affected. However, ultrasonic seed treatment alleviated those negative effects. Under the SW1 treatment, ultrasonic seed treatment reduced yield loss by reducing the decline of spikelets per panicle and seed-setting rate. Under SW2 treatment, ultrasonic seed treatment reduced the decline of spikelets per panicle to alleviate the yield loss. In addition, the response of grain yield to ultrasonic seed treatment in SW2 treatment decreased compared with SW1 treatment. Considering the significant effect of the interaction between seawater and ultrasonic on grain yield, we deduced that the effects of ultrasonic seed treatment on reducing yield loss would decline with the increase of the seawater concentration. Our deduction was supported by the studies by Abdelgadir et al. [22], which showed that high salinity stress to rice was difficult to alleviate by cultivation measures.
MDA production is a key indicator of oxidative stress in plants, and the over-production of MDA could be attributed to the oxidation of polyunsaturated fatty acids [12]. In the present study, the MDA content remarkably increased under the irrigation of seawater and also increased with the increases of seawater concentration, which indicated that seawater irrigation caused oxidative stress to rice, and the stress intensity enhanced with the increase of seawater concentration. Higher activities of SOD and POD were observed under seawater irrigation. SOD, CAT, and POD are the important enzymes to dismutase superoxide radicals and scavenge hydrogen peroxide in plant tissue. The oxidative stress would induce the up-regulation of their activities when the stress has not destroyed the structure and function [23]. The study by Saha et al. [24] showed that drought stress increased CAT and SOD activities in rice. In our study, seawater irrigation induced oxidative stress in rice plants and led to the enhancement of SOD and POD activities. On the other hand, we observed that ultrasonic seed treatment substantially reduced MDA content and lowered the activity under seawater irrigation. The reduced MDA content indicated that ultrasonic seed treatment improved the salinity tolerance of rice plants so that the oxidative stress caused by seawater was alleviated. However, we observed ultrasonic seed treatment did not enhance the activities of antioxidant enzymes, including POD, SOD, and CAT, under seawater irrigation, which indicated that ultrasonic seed treatment did not improve the salinity tolerance of rice plants through the enhancement of antioxidant enzymes but other pathways. The decline of antioxidant enzymatic activities manifested the improvement of the oxidation environment in plant tissue under seawater irrigation.
The negative effects of seawater irrigation on rice were mainly attributed to the high salinity. The response of rice to salt stress is multifaceted and complex, which depends on the duration of salt stress, rice development stage, sunshine duration, and other factors [25,26]. Osmotic stress is the main stress that plants face in salt stress, and under salt stress, plants synthesize and accumulate osmotic protectants such as proline to increase the electrolyte extravasation rate of plant tissues and promote the osmotic balance at the cell level and deal with salt stress [27]. In the present study, seawater irrigation significantly increased proline content, and the content increased with the increment of seawater irrigation. Moreover, higher proline content was recorded in ultrasonic seed treatment than control under seawater irrigation. The proline accumulation due to seawater irrigation might be attributed to the enhanced OAT activity. OAT, PDH, and P5CS are the important enzymes involved in proline metabolism in plant tissue, while PDH is responsible for proline degradation [28]. In our study, over-production of proline induced the up-regulation of PDH activity under seawater irrigation when the seeds were without ultrasonic treatment. Moreover, ultrasonic seed treatment significantly down-regulated the PDH activity under seawater irrigation. Proline plays an important role in osmoregulation and helps maintain cellular structures and functions [12]. The study by Nguyen et al. [29] showed that proline accumulation is a strategy to cope with salinity stress in rice plants. Abdelaziz et al. [30] also demonstrated that the salinity-tolerant varieties had higher proline accumulation than the salinity-susceptible cultivars. In our study, the rice plants after the ultrasonic seed treatment exhibited higher proline content under seawater irrigation. Thus, we deduced that ultrasonic seed treatment enhanced salinity tolerance of rice by reducing the proline degradation to lead the proline accumulation so that the cellular osmotic pressure, structures, and functions, could be better maintained or/and improved under seawater irrigation. Furthermore, because of the improvement in the osmotic environment, the oxidative stress caused by seawater irrigation was alleviated, and the yield formation was promoted.
Seawater irrigation significantly decreased the spikelets per panicle, seed-setting rate, 1000-grain weight, and caused yield loss of rice, while yield loss aggravated with the increase of seawater concentration. Seawater irrigation increased MDA and proline contents. Higher activities of SOD and POD were observed under seawater irrigation. The yield loss and oxidative damage caused by seawater were alleviated by ultrasonic seed treatment. Compared with control, ultrasonic seed treatment significantly increased proline content and reduced the rise of SOD and POD activities in rice under seawater irrigation. Our study indicated that ultrasonic seed treatment could improve rice performances under seawater irrigation.
Authorship: study conception and design: X.T, Z.Y; data collection: Y.Z, J.L, G.R; analysis and interpretation of results: Y.Z; draft manuscript preparation: Y.Z, J.L. All authors reviewed the results and approved the final version of the manuscript.
Funding Statement: This study was supported by National Natural Science Foundation of China (31971843), The Technology System of Modern Agricultural Industry in Guangdong (2020KJ105) and Guangzhou Science and Technology Project (202103000075).
Conflicts of Interest: The authors declare that they have no conflicts of interest to report regarding the present study.
References
1. Pantoja-Benavides, A. D., Garces-Varon, G., Restrepo-Díaz, H. (2021). Foliar growth regulator sprays induced tolerance to combined heat stress by enhancing physiological and biochemical responses in rice. Frontiers in Plant Science, 12, 702892. DOI 10.3389/fpls.2021.702892. [Google Scholar] [CrossRef]
2. Huang, M., Tao, Z., Lei, T., Cao, F., Chen, J. et al. (2021). Improving lodging resistance while maintaining high grain yield by promoting pre-heading growth in rice. Field Crops Research, 270, 108212. DOI 10.1016/j.fcr.2021.108212. [Google Scholar] [CrossRef]
3. Ishfaq, M., Akbar, N., Zulfiqar, U., Ali, N., Shah, F. et al. (2022). Economic assessment of water-saving irrigation management techniques and continuous flooded irrigation in different rice production systems. Paddy and Water Environment, 20(1), 37–50. DOI 10.1007/s10333-021-00871-6. [Google Scholar] [CrossRef]
4. Tolomio, M., Borin, M. (2018). Water table management to save water and reduce nutrient losses from agricultural fields: 6 years of experience in North-Eastern Italy. Agricultural Water Management, 201, 1–10. DOI 10.1016/j.agwat.2018.01.009. [Google Scholar] [CrossRef]
5. Mekonnen, M. M., Hoekstra, A. Y. (2016). Four billion people facing severe water scarcity. Science Advances, 2(2), e1500323. DOI 10.1126/sciadv.1500323. [Google Scholar] [CrossRef]
6. Zhao, G., Liu, Z., Chen, M., Guo, S. (2008). Soil properties and yield of Jerusalem artichoke (Helianthus tuberosusL.) with seawater irrigation in North China plain. Pedosphere, 18(2), 195–202. DOI 10.1016/S1002-0160(08)60007-7. [Google Scholar] [CrossRef]
7. Shaffer, D. L., Yip, N. Y., Gilron, J., Elimelech, M. (2012). Seawater desalination for agriculture by integrated forward and reverse osmosis: Improved product water quality for potentially less energy. Journal of Membrane Science, 415, 1–8. DOI 10.1016/j.memsci.2012.05.016. [Google Scholar] [CrossRef]
8. Mohammadi-Nejad, G., Singh, R. K., Arzani, A., Rezaie, A. M., Sabouri, H. et al. (2010). Evaluation of salinity tolerance in rice genotypes. International Journal of Plant Production, 4(3), 199–207. [Google Scholar]
9. Sultana, N., Ikeda, T., Kashem, M. A. (2002). Effect of seawater on photosynthesis and Dry matter accumulation in developing rice grains. Photosynthetica, 40(1), 115–119. DOI 10.1023/A:1020175130290. [Google Scholar] [CrossRef]
10. Rao, G., Ashraf, U., Huang, S., Cheng, S., Abrar, M. et al. (2018). Ultrasonic seed treatment improved physiological and yield traits of rice under lead toxicity. Environmental Science and Pollution Research, 25(33), 33637–33644. DOI 10.1007/s11356-018-3303-5. [Google Scholar] [CrossRef]
11. Huang, S., Rao, G., Ashraf, U., Deng, Q., Dong, H. et al. (2021). Ultrasonic seed treatment improved morpho-physiological and yield traits and reduced grain Cd concentrations in rice. Ecotoxicology and Environmental Safety, 214, 112119. DOI 10.1016/j.ecoenv.2021.112119. [Google Scholar] [CrossRef]
12. Kong, L., Ashraf, U., Cheng, S., Rao, G., Mo, Z. et al. (2017). Short-term water management at early filling stage improves early-season rice performance under high temperature stress in South China. European Journal of Agronomy, 90, 117–126. DOI 10.1016/j.eja.2017.07.006. [Google Scholar] [CrossRef]
13. Wang, Q., Chen, G., Yersaiyiti, H., Liu, Y., Cui, J. et al. (2012). Modeling analysis on germination and seedling growth using ultrasound seed pretreatment in switchgrass. PLoS One, 7(10), e47204. DOI 10.1371/journal.pone.0047204. [Google Scholar] [CrossRef]
14. Basit, F., Chen, M., Ahmed, T., Shahid, M., Noman, M. et al. (2021). Seed priming with brassinosteroids alleviates chromium stress in rice cultivars via improving ROS metabolism and antioxidant defense response at biochemical and molecular levels. Antioxidants, 10(7), 1089. DOI 10.3390/antiox10071089. [Google Scholar] [CrossRef]
15. Dwivedi, S. K., Kumar, S., Mishra, J. S., Prakash, V., Rao, K. K. et al. (2022). Interactive effect of elevated [CO2] and temperature on the photosynthetic process, anti-oxidative properties, and grain yield of rice. Journal of Agronomy and Crop Science, 208(3), 384–393. DOI 10.1111/jac.12579. [Google Scholar] [CrossRef]
16. Mostofa, M. G., Rahman, M. M., Siddiqui, M. N., Fujita, M., Tran, L. P. (2020). Salicylic acid antagonizes selenium phytotoxicity in rice: Selenium homeostasis, oxidative stress metabolism and methylglyoxal detoxification. Journal of Hazardous Materials, 394, 122572. DOI 10.1016/j.jhazmat.2020.122572. [Google Scholar] [CrossRef]
17. Li, M., Li, R., Liu, S., Zhang, J., Luo, H. et al. (2019). Rice-duck co-culture benefits grain 2-acetyl-1-pyrroline accumulation and quality and yield enhancement of fragrant rice. The Crop Journal, 7(4), 419–430. DOI 10.1016/j.cj.2019.02.002. [Google Scholar] [CrossRef]
18. Ncube, B., Finnie, J. F., Van Staden, J. (2013). Dissecting the stress metabolic alterations in vitro Cyrtanthus regenerants. Plant Physiology and Biochemistry, 65, 102–110. DOI 10.1016/j.plaphy.2013.01.001. [Google Scholar] [CrossRef]
19. Sánchez, E., Ruiz, J. M., Romero, L. (2002). Proline metabolism in response to nitrogen toxicity in fruit of French bean plants (Phaseolus vulgaris L. cv Strike). Scientia Horticulturae, 93(3–4), 225–233. DOI 10.1016/S0304-4238(01)00342-9. [Google Scholar] [CrossRef]
20. Luo, H., Duan, M., Kong, L., He, L., Chen, Y. et al. (2021). The regulatory mechanism of 2-acetyl-1-pyrroline biosynthesis in fragrant rice (Oryza sativa L.) under different soil moisture contents. Frontiers in Plant Science, 12. 772728. DOI 10.3389/fpls.2021.772728. [Google Scholar] [CrossRef]
21. Marcos, M., Sharifi, H., Grattan, S. R., Linquist, B. A. (2018). Spatio-temporal salinity dynamics and yield response of rice in water-seeded rice fields. Agricultural Water Management, 195, 19537–19546. DOI 10.1016/j.agwat.2017.09.016. [Google Scholar] [CrossRef]
22. Abdelgadir, E. M., Oka, M., Fujiyama, H. (2005). Nitrogen nutrition of rice plants under salinity. Biologia Plantarum, 49(1), 99–104. DOI 10.1007/s10535-005-0104-8. [Google Scholar] [CrossRef]
23. Lei, S., Rossi, S., Huang, B. (2022). Metabolic and physiological regulation of aspartic acid-mediated enhancement of heat stress tolerance in perennial ryegrass. Plants, 11(2), 199. DOI 10.3390/plants11020199. [Google Scholar] [CrossRef]
24. Saha, S., Begum, H. H., Nasrin, S., Samad, R. (2020). Effects of drought stress on pigment and protein contents and antioxidant enzyme activities in five varieties of rice. Bangladesh Journal of Botany, 49(4), 997–1002. DOI 10.3329/bjb.v49i4.52516. [Google Scholar] [CrossRef]
25. Thorne, S. J., Stirnberg, P. M., Hartley, S. E., Maathuis, F. (2022). The ability of silicon fertilisation to alleviate SalinityStress in rice is critically dependent on cultivar. Rice, 15, 8. [Google Scholar]
26. Chen, T., Shabala, S., Niu, Y., Chen, Z., Shabala, L. et al. (2021). Molecular mechanisms of salinity tolerance in rice. The Crop Journal, 9(3), 506–520. DOI 10.1016/j.cj.2021.03.005. [Google Scholar] [CrossRef]
27. Hmida-Sayari, A., Gargouri-Bouzid, R., Bidani, A., Jaoua, L., Savouré, A. et al. (2005). Overexpression of Δ1-pyrroline-5-carboxylate synthetase increases proline production and confers salt tolerance in transgenic potato plants. Plant Science, 169(4), 746–752. DOI 10.1016/j.plantsci.2005.05.025. [Google Scholar] [CrossRef]
28. Szabados, L., Savouré, A. (2010). Proline: A multifunctional amino acid. Trends in Plant Science, 15(2), 89–97. DOI 10.1016/j.tplants.2009.11.009. [Google Scholar] [CrossRef]
29. Nguyen, H., Das, B. S., Long, H., Cheng, Y., Mundree, S. et al. (2021). Rapid accumulation of proline enhances salinity tolerance in Australian wild rice Oryza australiensis domin. Plants, 10(10), 2044. DOI 10.3390/plants10102044. [Google Scholar] [CrossRef]
30. Abdelaziz, M., Xuan, T., Mekawy, A., Wang, H., Khanh, T. (2018). Relationship of salinity tolerance to Na + exclusion, proline accumulation, and antioxidant enzyme activity in rice seedlings. Agriculture, 8(11), 166. DOI 10.3390/agriculture8110166. [Google Scholar] [CrossRef]
Cite This Article
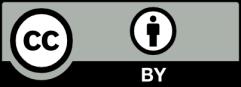
This work is licensed under a Creative Commons Attribution 4.0 International License , which permits unrestricted use, distribution, and reproduction in any medium, provided the original work is properly cited.