Open Access
ARTICLE
Effects of Different Arbuscular Mycorrhizal Fungi on Physiology of Viola prionantha under Salt Stress
College of Landscape Architecture, Northeast Forestry University, Harbin, 150040, China
* Corresponding Author: Chunxue Yang. Email:
(This article belongs to the Special Issue: Mycorrhizal Fungi and Sustainable Development of Agriculture)
Phyton-International Journal of Experimental Botany 2023, 92(1), 55-69. https://doi.org/10.32604/phyton.2022.022159
Received 23 February 2022; Accepted 20 April 2022; Issue published 06 September 2022
Abstract
Arbuscular mycorrhizal (AM) fungi distribute widely in natural habits and play a variety of ecological functions. In order to test the physiological response to salt stress mediated by different AM fungi, Viola prionantha was selected as the host, the dominant AM fungus in the rhizosphere of V. philippica growing in Songnen saline-alkali grassland, Rhizophagus irregularis, and their mixtures were used as inoculants, and NaCl stress was applied after the roots were colonized. The results showed that V. philippica could be colonized by AM fungi in the field and the colonization rate ranged from 73.33% to 96.67%, and Claroideoglomus etunicatum was identified as the dominant AM fungi species in the rhizosphere of V. philippica by morphology combined with sequencing for AM fungal AML1/AML2 target. Inoculation with both the species resulted in the formation of mycorrhizal symbiosis (the colonization rate was more than 70%) and AM fungi significantly enhanced plants’ tolerance to salt stress of varying magnitude. Higher activity of antioxidant enzymes and augmented levels of proline and other osmoregulators were observed in AM plants. The content of MDA in CK was higher than that in the inoculations with the stress of 100, 200, and 250 mM. All indices except soluble protein content and MDA content were significantly correlated with AM fungal colonization indices. The analysis for different AM fungal effects showed that the mixtures and R. irregularis worked even better than C. etunicatum. These results will provide theoretical support for the exploration and screening of salt-tolerant AM fungi species and also for the application of AM-ornamental plants in saline-alkali urban greening.Keywords
Soils around the world were severely salinized and the area of agricultural land decreased sharply in recent years [1]. The global saline-alkali area is approximately 956 million hectares at present [2]. China’s salinized land covers an area of 520 million acres [3], and the salinized region is classified into five areas according to the differences in bio-climate and other environmental factors. Among which, Songnen saline-alkali grassland is situated in the northeast region [4], which is one of the three soda salinized soil distribution areas in the world and usually occurs salinization simultaneously with sodication [5]. Several measures have been taken to alleviate the pressure on ecological and economic development brought by the increasingly serious soil salinization in this area. The method of growing plants can greatly reduce the cost and improve the soil in a depth [4]. Therefore, it is necessary to promote the plants’ development growing in saline-alkali land.
As an excellent ground cover, V. philippica is important for afforestation and enjoying in early spring in Northern China. Relevant studies have proved that V. philippica had strong saline-alkali tolerance [6,7] and naturally distributed in some areas of Songnen saline-alkali grassland. Arbuscular mycorrhizal (AM) fungi can form a symbiosis with 90% of vascular plants and play important roles in the ecosystem [8], such as uptaking and transporting nutrients, regulating soil properties, and improving the relationships between plants and other biogroup [9]. Nowadays, many scholars have tried to investigate the effects of AM fungi on plant salt tolerance [10,11], which to the benefit of exploring more effective measures to restore salty land. When the hosts are salt-stressed, AM fungi can improve root viability and reduce leaf water loss [12], effectively maintaining the ionic balance by promoting the accumulation of K+ and Ca2+ and reducing the Na+ content [13], in addition, it can also regulate the salt tolerance of plants in molecular level [14,15]. Previous investigations proved that Songnen saline-alkali grassland was rich in AM fungi resources [16]. However, no paper reported the AM fungal species in the rhizosphere of V. philippica and it was not clear whether AM fungi were involved in the growth regulation. It is worth noting that pH directly affected the AM fungal diversity and the distribution of some species [17,18]. Therefore, the exploration of V. philippica-AM in different pH conditions would provide a basis for realizing the relationship between AM fungi and the salt tolerance of V. philippica growing in saline-alkali grassland and also for the application of V. philippica-AM symbiosis in salty cities. Molecular biological analysis and morphological identification were the major methods to identify AM fungi. The species obtained by morphology possess more comprehensive information, but the researchers should equip with an overall understanding for the morphological characteristics of the discovered and newly recorded species [19]. AM fungal AML1/AML2 target showed interspecific differences and the alignment result could accurately annotate the species [20]. The combination of the above-mentioned methods contributes to the correct analysis of AM fungal species.
V. prionantha, which is the related species of V. philippica, has an earlier flowering period and different morphology characteristics, although they belong to the same genus and both of them possess salt tolerance [21]. It has been verified that Rhizophagus irregularis performed well in improving the physiology and secondary metabolites of Viola tricolor in the Viola genus [22]. To widen the extent of AM fungal application and compare the effects among different AM fungi species, V. prionantha was selected as the host and after which inoculate R. irregularis and the dominant species isolated in the experiment. After the salt stress for a while, enzyme activities, membrane lipid peroxidation, and the content of osmoregulators in hosts were measured, so that the physiological response to salt stress mediated by different AM fungi was clarified. The results will provide theoretical support for the application of plant-AM symbionts in salty habitats.
The view that Glomus was a broad-spectrum symbiotic system has been proved in previous research, and Glomus was the dominant AM fungal genera in various environments [23,24]. Many studies also pointed out that the colonization was better than single inoculation when two species were inoculated simultaneously [25,26]. Besides, mixtures have worked best in several studies [25,27], and local fungus might be more effective than commercial fungi due to the local adaptation [28]. Therefore, the following hypotheses will be tested in this experiment: (a) The dominant species in the rhizosphere of V. philippica belongs to Glomus. (b) The colonization of mixtures is better than that of single inoculation. (c) All the inoculations significantly enhance plants’ tolerance to salt stress of varying magnitude and the mixtures work the best, followed by the dominant species.
2.1 Samples Collection and Determination of Soil pH
The sampling sites were located in the saline-alkali land around Zhaodong, Heilongjiang Province in the middle of Songnen Plain (125°54’2”–125°54’15.13” E, 46°2’52”–46°2’58.16” N). The climate of this region was characterized by semi-humid-semi-arid and the soil was soda salinized. On April 27, 2019, a total of nine sites of V. philippica growth were selected randomly within 90 hectares. According to the “multipoint parallel sampling method” and the “five sampling method” [29], plants in each plot were randomly harvested from four directions to ensure that the samples collected in this study were widely representative. After being dug up, the plants were vigorously shaken to remove the excess soil, and those still stuck to the roots were considered the rhizosphere soil [30]. Subsequently, the materials including roots and rhizosphere soils (approximately 0.5 Kg) from the depth of 10–20 cm were collected at each site. The roots were immersed in FAA fixing solution (5 ml formalin, 5 ml glacial acetic acid, and 90 ml of 70% ethyl alcohol) after they were cut into the 1 cm-long segments. 300 g of rhizosphere soil was reserved, numbered, and marked, then stored at 4°C.
Soil pH was measured by a pH meter (METTLER TOLEDO FE20, China). 10 g rhizosphere soil was weighed from each sample and it was mixed into suspension (water:soil = 2.5:1). After the solution precipitated for 0.5 h, its pH value was measured using the pH meter. The average of three measurements, determined after the pH meter reading stabilized, was taken as the pH of each sampling site.
2.2 AM Fungal Colonization in the Roots of V. philippica
The root segments soaked in FAA solution were taken out, and they were incubated in 10% KOH to soften and transparentize after being washed with distilled water. Subsequently, the roots were bleached with 10% hydrogen peroxide. After that, the root segments were neutralized with 2% hydrochloric acid for 30 min, and then they were stained with the 0.05% trypan-blue reagent in accordance with Philips et al. [31]. The decolorization was performed with glycerin lactate solution. Finally, the morphology of arbuscular, vesicles, and hyphae were observed under the microscope (OLYMPUS-DSX500, Japan). The AM fungal colonization in V. philippica was evaluated as the description in Trouvelot et al. [32].
2.3 Isolation and Morphological Identification of AM Fungal Spores
AM fungal spores in rhizosphere soil (50 g) were separated using wet screening and sucrose density-gradient centrifugation method [33]. The spores were enumerated under a dissecting microscope (Leica MDG33, Germany) after they were cleaned in an ultrasonic washer (AS, Tianjin Autoscience Instrument Co., Ltd., China). Subsequently, a single spore was sucked onto a glass slide and so that the sporal characteristics which involved walls, content, color, size, hyphae were observed under a digital optical microscope (OLYMPUS-DSX500, Japan). Then the spores were stained using Melzer’s reagent and the resulting changes were recorded. Finally, the species identification was performed based on the characteristics obtained in the above-mentioned operation and was also referred to as “Chinese AM fungal resources and germplasm resources” [34], INVAM International website (http://invam.wvu.edu/the-fungi/species-descriptions), Janusz Blaszkowski of Poland agricultural university (http://www.zor.zut.edu.pl/Glomeromycota) and morphological descriptions, pictures, and literature newly published.
2.4 Determination of Dominant AM Fungal Species by Morphology
The separation frequency, relative abundance, and importance value of the spores identified by morphology were calculated referring to the method proposed by Yang et al. [35]. The dominant species was determined according to the importance value.
Separation frequency (F) = occurrence frequency of individual species/total sample number × 100%
Relative abundance (RA) = spore number of individual species/total quantity of AM fungal spores × 100%
Importance value (IV) = (F + RA)/2 × 100%.
2.5 Molecular Identification of Dominant AM Fungal Spores
The dominant AM fungal spores cleaned by an ultrasonic washer were transferred into a sterilized petri dish filled with ddH2O. A single spore (1 μl) that was used as the amplification template was aspirated repeatedly until it was clean and then it was placed in sterilized PCR tube. After which the spore was thoroughly crushed with a sterile pipette tip under a stereoscope. Subsequently, a two-step nested PCR was performed to amplify the AML1/AML2 target of AM fungal 18 s rDNA and the amplified fragment was about 800 bp in length. The AM fungal universal primers GeoA2 (5´-CCAGTAGTCATATGCTTGTCTC-3´) and Geo11 (5´-ACCTTGTTACGACTTTTACTTCC-3´) were used for the first PCR. And the second PCR employed specific primers AML1 (5´-ATCAACTTTCGATGGTAGGATAGA-3´) and AML2 (5´-GAACCCAAACACTTTGGTTTCC-3´) [36]. The reaction system and process were shown in Appendix A.
The OMEGA Gel Extraction Kit (D2500-01) was used to purify the amplified products that the length had been examined by 1.0% agarose gel electrophoresis. Sequencing was completed by Qingke Biotechnology Co., Ltd. and the GeneBank accession number was MW349795. A phylogenetic tree (Maximum-likelihood) was constructed using MEGA 7.0.
The seeds of V. prionantha were purchased from Small Ant Shop in Ningxia, China. The dominant species identified in this experiment and the fungi which performed well in Viola (R. irregularis, RI) were selected as the experimental inoculants and they were purchased from China AMF Germplasm Bank (BGC). The spore density was about 18/g.
The V. prionantha was seeded into the sterilized substrate (peat soil: vermiculite = 3:1) after the seeds were disinfected with 0.3% KMnO4. The seedlings were transplanted when two euphylla appeared and four inoculations were added at the same time: 12 g CE, RI, and CE + RI were placed around the roots of V. prionantha in three inoculation groups, respectively, and the control group which without inoculation (CK) was applied equal amount of sterilized substrate. Maintaining light 14 h, light intensity 5000lx, the temperature at 20°C–25°C, in addition, irrigating 100 ml distilled water every two days. 80 days after the inoculation, NaCl stress (0, 50, 100, 200 and 250 mM) was applied. The plants were irrigated with 50 ml NaCl solution every day and the experiment lasted for 10 days. All the groups were replicated three times. At the end of the 10-day salt tolerance test, the roots were harvested and immersed in FAA fixing solution at 4°C, the leaves were sub-packaged with foil and then they were frozen in liquid nitrogen and preserved at −80°C till further use.
2.7 Physiological Response of V. prionantha to Salt Stress Mediated by AM Fungi
The AM fungal colonization in the roots was observed referring to the staining method of Philips et al. [31]. The osmoregulators concentration, enzyme activities, and membrane lipid peroxidation were measured by referring to “Principles and Techniques of Plant Physiological Biochemical Experiment (Version 2)” [37]. The specific test methods were as follows: the content of malondialdehyde (MDA) was determined by thiobarbituric acid method, proline by acid ninhydrin, soluble sugar by anthrone colorimetry, and soluble protein by Coomassie Brilliant Blue G-250, the activities of peroxidase (POD), superoxide dismutase (SOD), catalase (CAT) were determined using guaiacol, NBT and ammonium molybdate, respectively.
MYCOCALC was used for the analysis of AM fungal colonization, SPSS 25.0 for the one-way ANOVA test, and also for the Pearson correlation analysis, the post hoc applied LSD Duncan. And chart drawing was completed with Sigmaplot12.5 and Photoshop 2019.
3.1 The Soil pH and AM Fungal Colonization in V. philippica
The pH of sampling sites ranged from 6.74 to 7.95. V. philippica growing in all the sites were colonized with the colonization rate of 73.33%–96.67%. The differences analysis of pH and various AM fungal colonization indices were performed at the 95% confidence interval (Table 1). The results showed that there were significant differences in soil pH, colonization intensity, arbuscular abundance, and vesicle abundance among different plots, while colonization rate showed insignificant difference in 9 plots. The analysis proved that the soil pH varied among sampling sites, and it also indicated that the investigation results of AM fungal species in 9 sampling sites were convincing for understanding the resources in the rhizosphere of V. philippica growing in Songnen saline-alkali grassland.
3.2 Dominant Species in the Rhizosphere of V. philippica Identified by Morphology
Thirty species of 11 AM fungal genera were identified from the rhizosphere soil of V. philippica by morphology. Among these, 9 species of Glomus, 7 species of Acaulospora, 2 species each of Ambispora, Rhizophgus, Septoglomus, Scutellospora, and Claroideoglomus, and 1 species each of Sclerocystis, Funneliformis, Entrophospora, and Gigaspora. The No. 119 distributed in 7 sampling sites and it was identified as the dominant species with the isolation frequency, relative abundance, and importance values of 88.89%, 25.5%, and 57.2%, respectively, while the relative abundance and importance values of other species only were 0.5%–12.5% and 5.81%–52.5%, respectively. Images of the thirty species could be found in the previous investigation of our team [38].
The morphological characteristics of No. 119 were shown in Fig. 1: The sporal color was orange to red-brown, the shape was globose or subglobose and the diameter was 60–160 μm with an average of 129 μm. The spore wall consisted of two layers (L1 and L2) that differentiated consecutively as spores develop. As an outer layer, L1 had some plasticity with an uneven outer surface and it became pink to reddish-purple after staining in Melzer’s reagent. L1 degraded and sloughed as spores age so that it might be present in patches or appeared as a granular layer. In addition, the hypha connected to the spore was shaped as cylindrical to slightly flared. The characteristics of No. 119 were highly similar to the Claroideoglomus etunicatum.
Figure 1: Dominant AM fungi (No. 119) in the rhizosphere of V. philippica
3.3 Results of Molecular Identification
The length of the AML1/AML2 target of No. 119 was 764 bp (Fig. 2a). The maximum-likelihood phylogenetic tree (Fig. 2b) was constructed using MEGA7.0 according to the results of sequence alignment conducted with the NCBI database. The 12 sequences with high homology of more than 98% to No. 119 were selected. The results showed that No. 119 clustered with the MN726592.1 C. etunicatum. Therefore, No. 119 was identified as C. etunicatum by molecular sequencing combined with morphological identification.
Figure 2: (a) Electrophoresis map of nested-PCR amplified products; (b) Phylogenetic tree of No. 119
3.4 AM Fungal Colonization in the Roots of V. prionantha under Salt Stress
Inoculation with both species resulted in the formation of mycorrhizal symbiosis (Fig. 3). AM fungi formed hyphae outside the roots of V. prionantha (Fig. 3a), besides, large numbers of hyphae would be present in the cortical cells after colonizing the roots and some of them expanded to be vesicles (Figs. 3b, 3c). In addition, some hyphae branched densely to form arbuscules (Figs. 3d–3h). The roots inoculated with CE formed Paris-type (P) mycorrhizal structure in which hyphae circles could be observed (Figs. 3c, 3g, 3i). A typical Arum-type (A) mycorrhizal structure was observed in the roots of inoculating RI. And both the two types were found in the segments colonized with CE + RI and the mycorrhizal structure was determined as Intermediate-type (I).
Figure 3: Symbiont of AM-V. prionantha. h-hypha, v-vesicule, a-arbuscule, hc-hyphal coil
All the root segments inoculated RI were colonized. The colonization rate, colonization intensity, and vesicle abundance were by the tendency of RI > CE + RI > CE. The results of the one-way ANOVA test for different inoculations were as follows (Table 2). There was an inconspicuous difference in colonization indices of CE while arbuscular abundance in RI decreased distinctly with the increasing NaCl concentrations (P < 0.05). Arbuscular abundance and colonization intensity in CE + RI were significantly different (P < 0.05) and all of them decreased with the continuously aggravated NaCl stress. It was noteworthy that the colonization of mixtures was not better than that of single inoculation.
3.5 Physiological Response of V. prionantha to Salt Stress Mediated by AM Fungi
3.5.1 The Response of Membrane System to Salt Stress Mediated by AM Fungi
In keeping with common sense, MDA showed an upward trend in CK with the NaCl stress aggravated (Fig. 4). The content of MDA in CK was higher than that in the inoculations with the stress of 100, 200, and 250 mM. Comparing with CK, MDA concentrations in CE, RI and CE + RI decreased by 41.15%, 53.86% and 3.35% at 100 mM NaCl stress, respectively, 65.52%, 55.58% and 29% at 200 mM, 54.73%, 31.69% and 53.04% at 250 mM, respectively. The CE reduced the content significantly compared with other inoculations at concentrations of 0, 50, and 200 mM (P < 0.05). The effect of CE + RI on alleviating membrane lipid peroxidation was relatively poor and that of RI was the worst under extremely heavy salt stress (250 mM). In conclusion, CE worked the best in reducing membrane damage of V. prionantha under salt stress.
Figure 4: MDA content of V. prionantha under salt stress mediated by AM fungi
Note: Different letters in a certain stress indicated significant differences among inoculations, and we marked the largest value with a, followed by b, c, d.
3.5.2 The Response of Antioxidant Enzymes to Salt Stress Mediated by AM Fungi
SOD activity (Fig. 5a) in CK decreased with the augment of NaCl stress. The activity in CE only increased (175.31%) at 250 mM compared with CK. The performance of CE + RI was the similarity to that of CE, and the activity of CE + RI was 33.12% higher than CK at a NaCl concentration of 250 mM. The results also showed that the SOD activity of V. prionantha increased significantly in three inoculations at the extreme stress of 250 mM (P < 0.05). RI positively regulated the SOD when the NaCl concentration reached 100 mM. In conclusion, RI worked perfectly in SOD regulation.
Figure 5: The activities of antioxidant enzymes under salt stress mediated by AM fungi (a) SOD activity; (b) POD activity; (c) CAT activity. Different letters in a certain stress indicated significant differences among inoculations, and we marked the largest value with a, followed by b, c, d
POD activity increased first and then decreased with the stress augmented (Fig. 5b). The value of CE, RI, and CE + RI reached the maximum at 200 mM, which were increased by 117.83%, 319.29%, and 294.58%, respectively. RI and CE + RI increased the activity significantly at every NaCl concentration (P < 0.05) while CE inhibited the enzyme activity at a lower concentration. In brief, RI and the mixtures (CE + RI) worked even better.
All inoculations improved the CAT activity of V. prionantha under salt stress of varying magnitude (Fig. 5c). A remarkable effect was presented in CE + RI under the serious stress of 100, 200, and 250 mM compared with others (P < 0.05). CE, RI, and CE + RI increased the activity by 98.6%, 230.1%, and 370.0% contrasted with CK at 250 mM, respectively. The results indicated that CE + RI improved CAT activity the best at NaCl ≥ 100 mM while the RI performed well at slight stress.
3.5.3 The Response of Osmoregulators to Salt Stress Mediated by AM Fungi
The response of soluble sugar content (Fig. 6a) to various NaCl concentrations mediated by AM fungi was different. The content in the CE-plants outnumbered the CK at stress concentration ≤ 100 mM and it increased by 47.19% as the NaCl at 100 mM. However, the contents in RI and CE + RI were higher than that of CK at every NaCl concentration almost, and the effects were significant at multiple concentrations (P < 0.05). The highest increment in RI was 148.27% as the concentration at 50 mM and the maximum (43.67%) in CE + RI presented at the stress of 250 mM.
Figure 6: The osmoregulators under salt stress mediated by AM fungi (a) Soluble sugar content; (b) Soluble protein content; (c) Proline content. Different letters in a certain stress indicated significant differences among inoculations, and we marked the largest value with a, followed by b, c, d
The inoculations of CE, RI, and CE + RI increased the content of soluble protein under adversity (Fig. 6b), and the content in each inoculation was significantly augmented at 250 mM (P < 0.05), which increased by 52.08%, 54.11%, and 185.44%, respectively. In CE plants, the content first increased and then decreased with the strengthening of NaCl stress, the result indicated that CE performed well at all the concentrations (P < 0.05). The optimum effect of RI presented at 200 mM (increased by 152.28%) and CE + RI only performed well at 250 mM (increased by 185.44%). In conclusion, the effect of CE was the most stable, followed by RI.
Proline content (Fig. 6c) in V. prionantha under NaCl stress was improved to varying degrees in inoculations so that the osmoregulators in AM-plants were balanced. The content in CE only increased when the NaCl reached 250 mM (increased by 8.13%). The inoculation effects of RI and CE + RI were consistent and their values were higher than that of CK at salinity ≥ 100 mM. Furthermore, the preponderance of RI expressed significantly at 200 and 250 mM (P < 0.01) with the increment of 184.32% and 93.83%, respectively. Therefore, the effects of the three inoculations were as follows: RI > CE + RI > CE.
3.6 Correlation between AM Fungal Colonization and Physiology of Host under NaCl Stress
The correlation between AM fungal colonization and the physiology of host was analyzed to understand the relationship between them (Table 3). There was a significant negative correlation between soluble sugar content, CAT activity, and colonization intensity, and a negative association also existed between arbuscular abundance and the proline, soluble sugar, and POD. It was worth noting that a significant positive correlation existed between the vesicle abundance and some physiological indices (proline content, POD, and SOD). In addition, the colonization rate was only related to POD activity.
C. etunicatum has been reported in previous investigations operated in Songnen saline-alkali grassland [16,33,39–41]. However, C. etunicatum was identified as the dominant species for the first time and it only occurred as a common species in the rhizosphere of other plants. These might be related to the characteristics and genotype of hosts, which could play an impact on AM fungal community composition [42]. In addition, the AM fungal composition affected the reestablishment of degraded grassland [28], C. etunicatum, which appeared in songnen saline grassland frequently, might accelerate the vegetation restoration in saline-alkali land. The dominant species obtained in this research deserved further study.
Environmental conditions exerted an influence on AM fungal colonization [43] and that was proved in this experiment. The average colonization rate of CE and CE + RI in V. prionantha gradually declined with the aggravating NaCl stress, which was consistent with the relevant studies of AM fungi under salt stress [44]. A similar result as previous studies was obtained, in which the colonization of mixtures was not better than that of single AM fungi [45]. It could be speculated that the spores of multiple AM fungal species were inoculated and germinated simultaneously in the laboratory and the drastic competition for plant nutrients occurred in high-concentration NaCl stress, resulting in the decrease in colonization rate.
Inoculations could improve the physio-biochemical characteristics of hosts under stress and AM fungi played a significant role in improving the salt tolerance of V. prionantha in this experiment. AM fungal effects in multiple indices similar to the colonization, in which the mixtures was not better than single inoculation. The reason was likely that the malignant competition for living space and nutrients after colonizing simultaneously weakened the effects [46]. All the physiological indices of Trifolium repens inoculated with C. etunicatum were positively improved under salt stress of each concentration [45]. However, inoculations sometimes exerted the negative effects of inhibiting enzyme activities, increasing MDA content, and reducing osmolytes content in this study. These controversial indices differed among studies [47,48]. These might be related to the mechanism of salt tolerance mediated by AM fungi. Little damage will be brought to V. prionantha with salt tolerance by slight NaCl stress and the AM-plant symbiosis increased the nutrients output so that the negative effects appeared. Nevertheless, AM fungi transferred water and nutrients from the soil to hosts and regulated the physiology of plants to ensure the stability of symbiosis under high-concentration stress [49]. AM fungal regulation effects on various substances were different, improvement in certain indices was often accompanied by the interference to other indices [50], which was consistent with the experiment. The results showed that CE only worked well in the regulation of MDA and soluble protein, while R. irregularis and mixtures played good effects on four indicators. Studies have shown that some species in Rhizophagus could induce the specific expression of transporters and improve plant growth under adversity [51], besides, AM fungi played roles by community in the field, some species would appear together to stabilize the community composition but performed minor biological functions [52], moreover, there was almost no vesicle in the roots colonized with CE, suggesting that CE was underdeveloped in V. prionantha [53], which would affect the functions. These were likely the reasons for the better effects of RI and CE + RI. Though R. irregularis was not isolated from the rhizosphere of V. philippica, the role of R. irregularis in a community should be further verified to provide theoretical support for its application in saline-alkali land.
The colonization intensity represented the amounts of symbionts in roots and the arbuscular provided sites for exchanging nutrients and transferring carbohydrates, the augment of them would accelerate the nutrient consumption of hosts [54]. These were the reasons that colonization intensity and arbuscular abundance were negatively associated with multiple indices. Besides, the results showed that multiple indicators positively correlated to vesicle abundance. It was might relate to the function of the vesicle that reserved nutrients [8]. A large vesicle abundance indicated that sufficient nutrient was supplied for fungi growth and host development, and it ensured that AM fungi could play a stable ecological role and reduce the nutrient uptake from hosts. But that’s just a guess based on the existing information. The relationship between AM fungal colonization and physiological responses of hosts under stress was necessary to further explore.
The results showed that our hypothesis was incorrect. The dominant species (C. etunicatum) in the rhizosphere of V. philippica didn’t belong to Glomus. The colonization of mixtures was not better than that of single inoculation. Inoculations improved the physio-biochemical characteristics of V. prionantha under salt stress, a higher activity of antioxidant enzymes and augmented levels of osmoregulators were observed in AM plants. RI and the mixtures (CE + RI) worked even better because they exerted a good effect on multiple indices. Besides, all indices except soluble protein and MDA were significantly correlated with AM fungal colonization indices.
Acknowledgement: We thank the Biotechnology Company (Qingke Biotechnology Co., Ltd., Beijing, China) for the technical support, and we are sincerely thankful to the help from Yunhui Zhou and Jiamei Xu.
Funding Statement: Research was funded by National Natural Science Foundation of China with the Grant No. 31601986 and Heilongjiang Postdoctoral Scientific Research Developmental Fund (LBH-Q16005).
Conflicts of Interest: The authors declare that they have no conflicts of interest to report regarding the present study.
Reference
1. Garcia-Franco, N., Wiesmeier, M., Hurtarte, L. C. C., Fella, F., Martinez-Mena, M. et al. (2021). Pruning residues incorporation and reduced tillage improve soil organic matter stabilization and structure of salt-affected soils in a semi-arid Citrus tree orchard. Soil and Tillage Research, 213, 105–129. DOI 10.1016/j.still.2021.105129. [Google Scholar] [CrossRef]
2. Sa, W. D. (2021). Study on the characteristics of nutritional quality changes and fungal community structure of alfalfa after cutting in saline-alkali soil (Ph.D. Thesis). Inner Mongolia Agricultural University, China. [Google Scholar]
3. Yu, R. P., Chen, D. M. (1999). Exploitation and utilization of salted soil resources in China. Journal Soil Science, 30(4), 158–159. [Google Scholar]
4. Chi, C. M. (2016). Study on adversity stress of soda-saline soil in Songnen Plain. Chengdu, China: Southwest Jiaotong University Press. [Google Scholar]
5. Wang, Z. Q., Zhu, S. Q., Yu, R. P. (1993). Chinese saline soil. Beijing, China: Science press. [Google Scholar]
6. Guo, S. Y., Ma, J. G., Ma, Y. L. (2011). Study of growth dynamics of Viola philippica in different saline alkaline soils. Journal of Anhui Agricultural Science, 39(14), 8420–8421+8428. [Google Scholar]
7. Garcia-Caparros, P., Lao, M. T. (2018). The effects of salt stress on ornamental plants and integrative cultivation practices. Scientia Horticulturae, 240, 430–439. DOI 10.1016/j.scienta.2018.06.022. [Google Scholar] [CrossRef]
8. Yang, F., Cao, H. M., Chen, Y. Y., Wang, J. L. (2019). Research progress on structure and identification method of arbusular mycorrhizal fungi. Modern Agricultural Science and Technology, 16, 152–154+157. [Google Scholar]
9. Powell, J. R., Rilling, M. C. (2018). Biodiversity of arbuscular mycorrhizal fungi and ecosystem function. New Phytologist, 220, 1059–1075. DOI 10.1111/nph.15119. [Google Scholar] [CrossRef]
10. Wang, H., Liang, L. Y., Liu, B. X., Huang, D., Liu, S. et al. (2020). Arbuscular mycorrhizas regulate photosynthetic capacity and antioxidant defense systems to mediate salt tolerance in maize. Plants, 9(11), 1430. DOI 10.3390/plants9111430. [Google Scholar] [CrossRef]
11. Duc, N. H., Vo, A. T., Haddidi, I., Daood, H., Posta, K. (2020). Arbuscular mycorrhizal fungi improve tolerance of the medicinal plant Eclipta prostrata (L.) and induce major changes in polyphenol profiles under salt stresses. Frontiers in Plant Science, 11, 612299. DOI 10.3389/fpls.2020.612299. [Google Scholar] [CrossRef]
12. Davis, F. T., Potter, J. R., Linuerman, R. G. (1993). Drought resistance of mycorrhizal pepper plants independent of leaf P concentration-response in gas exchange and water relations. Physiologia Plantarum, 87(1), 45–53. DOI 10.1111/j.1399-3054.1993.tb08789.x. [Google Scholar] [CrossRef]
13. Zhang, L., Yang, C. X. (2018). Enzyme activity and free amino acids of Puccinellia tenuiflora-arbuscular mycorrhizal symbiont under saline-alkali stress. Journal of Northeast Forestry University, 46(11), 91–96. [Google Scholar]
14. Yang, C. X., Zhao, W. N., Wang, Y. N., Zhang, L., Huang, S. C. et al. (2020). Metabolomics analysis reveals the alkali tolerance mechanism in Puccinellia tenuiflora plants inoculated with arbuscular mycorrhizal fungi. Microorganisms, 8(3), 327. DOI 10.3390/microorganisms8030327. [Google Scholar] [CrossRef]
15. Wang, Y. N., Lin, J. X., Huang, S. C., Zhang, L., Zhao, W. N. et al. (2019). Isobaric tags for relative and absolute quantification-based proteomic analysis of Puccinellia tenuiflora inoculated with arbuscular mycorrhizal fungi reveal stress response mechanisms in alkali-degraded soil. Land Degradation & Development, 30(13), 1584–1598. DOI 10.1002/ldr.3346. [Google Scholar] [CrossRef]
16. Yang, C. X., Chen, F., Yue, Y. N., Yan, X. F. (2015). Diversity characteristics of arbuscular mycorrhizal fungi in the rhizosphere of twenty six species of plants in Songnen saline-alkaline grasslan. Pratacultural Science, 32(12), 2008–2020. [Google Scholar]
17. Adenan, S., Oja, J., Alatalo, J. M., Shraim, A. M., Alsafran, M. et al. (2020). Diversity of arbuscular mycorrhizal fungi and its chemical drivers across dryland habitats. Mycorrhiza, 31(6), 685–697. DOI 10.1007/s00572-021-01052-3. [Google Scholar] [CrossRef]
18. Carvalho, L. M., Correia, P. M., Cacador, I., Martins-Loucao, M. A. (2003). Effects of salinity and flooding on the infectivity of salt marsh arbuscular mycorrhizal fungi in Aster tripolium L. Biology and Fertility Soils, 38(3), 137–143. DOI 10.1007/s00374-003-0621-6. [Google Scholar] [CrossRef]
19. Yang, C. X., Li, L. L. (2014). Research progress in arbuscular mycorrhizal fungi identification method application. Guizhou Agricultural Sciences, 42(7), 93–94. [Google Scholar]
20. van Tuinen, D., Jacquot, E., Zhao, B., Gollotte, A., Gianinazzi-Pearson, V. (1998). Characterization of root colonization profiles by a microcosm community of arbuscular mycorrhizal fungi using 25S rDNA-targeted nested PCR. Molecular Ecology, 7(7), 879–887. DOI 10.1046/j.1365-294x.1998.00410.x. [Google Scholar] [CrossRef]
21. Zhang, Z. R., Zhang, X. W., Jiang, W., Wang, B. B., Sun, H. D. et al. (2017). Responses of growth and physiological characteristics of three ground cover plants to salinity stress. Tianjin Agricultural Sciences, 23(8), 20–24. [Google Scholar]
22. Zubek, S., Rola, K., Szewczyk, A., Majewska, M. L., Turnau, K. (2015). Enhanced concentrations of elements and secondary metabolites in Viola tricolor L. induced by arbuscular mycorrhizal fungi. Plant and Soil, 390(1–2), 129–142. DOI 10.1007/s11104-015-2388-6. [Google Scholar] [CrossRef]
23. Araujo, A. S. F., Melo, V. M. M., Pereira, A. P. D., Lopes, A. C. D., Rocha, S. M. B. et al. (2021). Arbuscular mycorrhizal community in soil from different Brazilian cerrado physiognomies. Rhizosphere, 19, 100375. DOI 10.1016/j.rhisph.2021.100375. [Google Scholar] [CrossRef]
24. Haug, I., Setaro, S., Suarez, J. P. (2021). Global AM fungi are dominating mycorrhizal communities in a tropical premontane dry forest in Laipuna, South Ecuador. Mycological Progress, 20(6), 837–845. DOI 10.1007/s11557-021-01699-4. [Google Scholar] [CrossRef]
25. Wang, N., Chen, F., Yue, Y. N., Yang, C. X. (2017). Effects of two dominant AM fungi on salt tolerance of Medicago sativa in Songnen saline-alkali grassland. Jiangsu Agricultural Sciences, 45(24), 146–148. [Google Scholar]
26. Yang, H. X., Li, S. M., Li, M., Guo, S. X. (2014). Effects of arbuscular mycorrhizal fungi on salinity tolerance of Trifolium repens L. Journal of Qingdao Agricultural University (Natural Science), 31(2), 85–90. [Google Scholar]
27. Liu, Z. N., Guo, S. X., Li, W. (2017). Effect of arbuscular mycorrhizal fungi on growth and physiological characteristics of Lilium brownii. Acta Prataculturae Sinica, 26(11), 85–93. [Google Scholar]
28. Koziol, L., Bever, J. D. (2017). The missing link in grassland restoration: Arbuscular mycorrhizal fungi inoculation increases plant diversity and accelerates succession. Journal of Applied Ecology, 54(5), 1301–1309. DOI 10.1111/1365-2664.12843. [Google Scholar] [CrossRef]
29. Meng, F. R. (1996). Mycorrhizology of forest trees. Harbin: Northeast Forestry University Press. [Google Scholar]
30. Jin, H., Yang, X. Y., Liu, H. Y., Zhao, Y. H., Zheng, W. L. et al. (2021). The structure of rhizosphere soil and endophytic fungal communities associated with Stipa purpurea and their correlation with soil environmental factors. Acta Microbiologica Sinica, 61(11), 3520–3541. [Google Scholar]
31. Philips, J. M., Hayman, D. S. (1970). Improved procedures for clearing and staining parasitic and vesicular-arbuscular mycorrhizal fungi for rapid assessment of infection. Transactions British Mycological Society, 55, 158–161. DOI 10.1016/S0007-1536(70)80110-3. [Google Scholar] [CrossRef]
32. Trouvelot, A., Kough, J. L., Gianinazzi-Pearson, V. (1986). Mesure du taux de mycorhization VA dun systeme radiculaire. Recherche de methodes destimation ayant une signification fonctionnelle. In: Gianinazzi-Pearson, V., Gianinazzi, S. (Eds.Physiological and genetical aspects of mycorrhizae, pp. 217–221. Paris: Institut Nationale de la Recherche Agronomigue Press. [Google Scholar]
33. Chen, F. (2017). Research of diversity and function of AM fungi from puccinellia tenuiflora rhizosphere in Songnen alkaline grassland, China (Master Thesis). Northeast Forestry University, China. [Google Scholar]
34. Wang, Y. S., Zhang, S. B., Zhang, M. Q. (2012). Chinese AM Fungal Resources and Germplasm Resources, Beijing: China Agriculture Science Press. [Google Scholar]
35. Yang, J., He, X. L., Zhao, L. L. (2011). Species diversity of arbuscular mycorrhizal fungi in the rhizosphere of Salix psammophila in Inner Mongolia desert. Biodiversity Science, 19(3), 377–385. DOI 10.3724/SP.J.1003.2011.09221. [Google Scholar] [CrossRef]
36. Yuan, T. (2019). Diversity analysis of arbuscular mycorrhizal fungi in the rhizospheric soil of five forest types in fanjingshan national nature reserve, China (Master Thesis). Guizhou University, China. [Google Scholar]
37. Wang, X. K. (2015). Princilpes and techniques of plant physiological biochemical experiment, 3rd ed. Beijing, China: Higher Education Press. [Google Scholar]
38. Zhao, W. N., Yang, C. X. (2021). Investigation of AM fungal diversity in the rhizosphere of Viola philippica in songnen saline-alkali grassland. Journal of Northeast Forestry University, 49(9), 83–89. [Google Scholar]
39. Yang, C. X., Huang, S. C., Chen, F., Li, L. L. (2017). Infection characteristics and diversity of arbuscular mycorrhizal fungi in the rhizosphere of Inula japonica in Songnen saline-alkaline grassland. Pratacultural Science, 34(2), 231–239. [Google Scholar]
40. Gao, X. M., Wang, Y. D., Yang, C. X. (2019). Mycorrhizal fungal infection characteristics and the diversity of Taraxacum mongolicum rhizosphere in Songnen saline-alkaline grassland. Journal of China Agricultural University, 24(5), 90–97. [Google Scholar]
41. Wang, Y. D., Yang, C. X. (2020). Infection characteristics and diversity of AM fungi in rhizosphere of Potentilla anserina. Acta Agriculturae Boreali-Occidentalis Sinica, 29(2), 254–265. [Google Scholar]
42. Wang, H., Wu, A. J., Liu, B. X., Liu, R. J., Chen, Y. L. (2020). Interactions between mycorrhizal fungal diversity and plant diversity: A review. Microbiology China, 47(11), 3918–3932. [Google Scholar]
43. Zhang, Y. F., Bi, Q., Yang, Y. F., Zhang, Z. H., Hu, C. Q. et al. (2015). Arbuscular mycorrhizal fungi diversity in saline-alkaline Leymus chinensis grasslands on the songnen plain. Acta Prataculturae Sinica, 24(9), 80–88. [Google Scholar]
44. Ben Laouane, R., Meddich, A., Bechtaoui, N., Oufdou, K., Wahbi, S. (2019). Effects of arbuscular mycorrhizal fungi and rhizobia symbiosis on the tolerance of Medicago sativa to salt stress. Gesunde Pflanzen, 71(2), 135–146. DOI 10.1007/s10343-019-00461-x. [Google Scholar] [CrossRef]
45. Huang, S. C., Chen, F., Li, L. L., Yang, C. X. (2017). Effect of AMF on growth and physio-biochemistry of Trifolium repens under stress of salt alkaline soil in songnen plain. Guizhou Agricultural Sciences, 45(7), 61–67. [Google Scholar]
46. Shi, N. (2016). Mechanism of inter-specific interactions among arbuscular mycorrhizal fungi and phosphata solubilizing bacteria to improve P uptake of maize (Ph.D. Thesis). China Agricultural University, China. [Google Scholar]
47. Parihar, M., Rakshit, A., Rana, K., Tiwari, G., Jatav, S. S. (2020). Arbuscular mycorrhizal fungi mediated salt tolerance by regulating antioxidant enzyme system, photosynthetic pathways and ionic equilibrium in pea (Pisum sativum L.). Biologia Futura, 71(3), 289–300. DOI 10.1007/s42977-020-00037-1. [Google Scholar] [CrossRef]
48. Santander, C., Ruiz, A., Garcia, S., Aroca, R., Cumming, J. et al. (2019). Efficiency of two arbuscular mycorrhizal fungal inocula to improve saline stress tolerance in lettuce plants by changes of antioxidant defense mechanisms. Journal of the Science of Food and Agriculture, 100(4), 1577–1587. DOI 10.1002/jsfa.10166. [Google Scholar] [CrossRef]
49. Dastogeer, K. M. G., Zahan, M. I., Tahjib, U. A. M., Akter, M. A., Okazaki, S. (2020). Plant salinity tolerance conferred by arbuscular mycorrhizal fungi and associated mechanisms: A meta-analysis. Frontiers in Plant Science, 10, 550–558. DOI 10.3389/fpls.2020.588550. [Google Scholar] [CrossRef]
50. Pereira, L. S., de Araujo, R. P., de Oliveira, P. S., da Silva, L. D., Alves, P. A. C. et al. (2017). Cadmium induced changes in Solidago chilensis Meyen (Asteraceae) grown on organically fertilized soil with reference to mycorrhizae, metabolism, anatomy and ultrastructure. Ecotoxicology and Environmental Safety, 150, 76–85. DOI 10.1016/j.ecoenv.2017.12.017. [Google Scholar] [CrossRef]
51. Cheng, K., Wei, M., Jin, X. X., Tang, M., Zhang, H. Q. (2022). LbAMT3-1, an ammonium transporter induced by arbuscular mycorrhizal in Lycium barbarum, confers tobacco with higher mycorrhizal levels and nutrient uptake. Plant Cell Reports. DOI 10.1007/s00299-022-02847-0. [Google Scholar] [CrossRef]
52. Li, Y., Jiao, H., Xu, L. J., Zhao, H. H., Liu, R. J. (2010). Advances in the study of community structure and function of arbuscular mycorrhizl fungi. Acta Ecologica Sinica, 30(4), 1089–1096. [Google Scholar]
53. Ruotsalainen, A. L., Aikio, S. (2004). Mycorrhizal inoculum and performance of nonmycorrhizal Carex bigelowii and mycorrhizal Trientalis europaea. Canadian Journal of Botany, 82(4), 443–449. DOI 10.1139/b04-011. [Google Scholar] [CrossRef]
54. Harrison, M. J. (2012). Cellular programs for arbuscular mycorrhizal symbiosis. Current Opinion in Plant Biology, 15(6), 691–698. DOI 10.1016/j.pbi.2012.08.010. [Google Scholar] [CrossRef]
Cite This Article
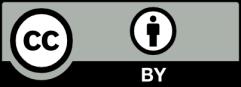
This work is licensed under a Creative Commons Attribution 4.0 International License , which permits unrestricted use, distribution, and reproduction in any medium, provided the original work is properly cited.