Open Access
ARTICLE
In vitro Evaluation of Seed Germination in Twelve Alfalfa Cultivars under Salt Stress
Plant Production Department, College of Food and Agriculture Sciences, King Saud University, Riyadh, 11451, Saudi Arabia
* Corresponding Author: Walid Soufan. Email:
(This article belongs to the Special Issue: Symbiotic Associations for Nutrients Management and Complexes Formation for Better Agricultural Crops Productivity under Biotic and Abiotic Stresses)
Phyton-International Journal of Experimental Botany 2023, 92(1), 111-120. https://doi.org/10.32604/phyton.2022.023115
Received 10 April 2022; Accepted 03 May 2022; Issue published 06 September 2022
Abstract
Alfalfa (Medicago sativa L.), when exposed to abiotic stress such as salinity, suffers significant losses in yield and productivity. The present study evaluated the salinity tolerance of 12 alfalfa cultivars in vitro using five concentrations of sodium chloride (NaCl), ranging from 0 to 250 mmol L−1 . The results obtained in the current study revealed that the Saudi cultivars, Kasimi and Hassawi, and the German cultivar (Berlin) had the highest salinity tolerance in terms of germination percentage (GP), corrected germination rate index (CGRI), days to reach 50% germination (GT50), and ability to form cotyledonary and true leaves. Under mmol L−1 NaCl, the Saudi cultivar Kasimi cultivar showed GP, CGRI, and GT50 of 55.20%, 123.15, and 3.77 days, respectively. Similarly, the German cultivar (Berlin) showed GP, CGRI, and GT50 of 50.06%, 86.61, and 5.17 days, respectively. These findings might reveal a pivotal aspect in salt tolerance in alfalfa. Our results will help to select salt-tolerant alfalfa cultivars that could thrive in arid and semi-arid areas with salinity problems.Keywords
Nomenclature
CGRI | Corrected germination rate index |
GP | Germination percentage |
GT50 | Days required to reach 50% germination |
MS medium | Murashige and Skoog medium |
w/v | Weight/volume |
Abiotic stresses such as salinity cause significant losses in alfalfa yield and greatly reduce productivity. Salinity affects over 1.0 billion hectares around the world, including natural and secondary salinity, which accounts for about 50% of the global land surface, and salinity resulting from irrigation affects around 1,400 million hectares, which accounts for 20% of all irrigated agricultural areas [1]. In general, salinity can result from natural resources and/or human impact [2]. Arid and semi-arid areas such as Saudi Arabia are among the regions most affected by salinity problems because of natural climatic characteristics including high temperatures and inadequate rainfall that led to more salt accumulation in agricultural soil [3,4]. Salt stress is mainly a result of plant exposure to high soil concentrations of sodium (Na+) and chlorine (Cl−) ions [5]. Salt stress mainly comprises secondary hyperosmotic stress resulting from reduced water potential, and ion toxicity stress resulting from high Na+ accumulation [6]. However, initial osmotic stress is followed by ionic stress. Moreover, salinity is likely to increase as a result of climate change that could result in higher temperatures and less precipitation. Therefore, the selection of crops for cultivation that have higher salinity tolerance is a highly successful means of coping with salinity problems.
Alfalfa (Medicago sativa L. Fabaceae) is considered as the most prevalent and important forage legume crop worldwide due to its high quantity and quality of yield, longevity, and ability to improve soils [7]. Alfalfa is moderately salt-sensitive with less salt sensitivity than other legume crops [8]. However, soil salinity in the range of 50–200 mM sodium chloride (NaCl) might significantly reduce the growth and productivity of alfalfa [9,10]. Nevertheless, the nutritive value of alfalfa as a consequence of exposure to salinity is increased [11]. Legume crops are the second in terms of agricultural importance as they provide more than 30% of human nitrogen needs [12]. Therefore, the need to adapt legume crops with high salinity tolerance while maintaining productivity has been extensively emphasized [11]. The high genetic variability of alfalfa crops might help with selecting the most tolerant cultivars and/or genotypes. However, selecting cultivars in field trials is laborious as the entire production cycle must be completed to measure changes in biomass and final yield. Evaluating alfalfa cultivars to assess morphological and physiological traits under various controlled stress conditions in vitro could be a suitable alternative to field evaluation [13]. Tissue culture is considered a rapid, convenient, and valid tool that that enables evaluating a single stress factor under controlled conditions. Alfalfa cultivar variations and salinity levels affect germination rates [14], and the ability to germinate under salt stress also significantly varies. For example, germination percentage of the Ladak 65 cultivar increased by about 3.75-fold after cultivation on agar medium containing 1.75% NaCl [15]. The W75RS salt-tolerant cultivar was identified using tissue culture techniques [16–18]. Such studies showed that salinity problems might be remedied by selecting cultivars that are more salt-tolerant.
Furthermore, previous studies examined the salinity tolerance in alfalfa plants in vitro [17,19,20]. However, the selection of salt tolerant cultivars requires consideration of all local genetic variations represented by cultivars that have adapted to the climatic conditions of the country where they grow. Here, we evaluated the salinity tolerance, in vitro, of 12 Medicago sativa L. cultivars from Saudi Arabia, Germany, USA, Australia, and Egypt. The evaluation process depended mainly on the following germination indicators: germination percentage, corrected germination rate, the number of days required to reach 50% germination, and the ability of each plant to form cotyledonary and true leaves under varied NaCl concentrations. We also compared salinity tolerance among alfalfa cultivars from Saudi Arabia and from other countries to optimize the selection of cultivars that could thrive under high-salt environments.
2.1 Seed Material and Surface Sterilization
Twelve alfalfa cultivars of M. sativa were obtained from Australia, Egypt, Germany, Saudi Arabia, and USA during April 2015. Table 1 shows the names and origins of the cultivars, as well as the fresh weight and moisture content of 1 × 103 seeds from each. Moisture content was estimated after drying the seeds at 70°C for 24 h. All seeds were surface-sterilized in 2% sodium hypochlorite (NaOCl) for 10 min, then washed three times with sterile distilled water to prevent contamination before starting experiments.
2.2 Screening Alfalfa for Salt Tolerance, and Culture Conditions
Salt tolerance was screened by culturing the seeds in sterile plastic Petri dishes (9 cm), each containing 30 mL half-strength MS medium [21] with 3% (w/v) sucrose and 0.8% (w/v) agar-agar and supplemented with 0, 100, 150, 200 and 250 mmol L−1 of NaCl. The pH of the medium was adjusted to 5.8 before autoclaving at 121°C and 1.2 kg cm–2 for 15 min. All dishes were incubated for 10 days at 25°C under a 16 h photoperiod with a 60 μmol m−2 s−1 photosynthetic photon flux density (PPF) provided by cool white fluorescent tubes.
2.3 Measurements of Seed Germination Variables
Germinated seeds were counted daily for 10 days. Seeds were considered as germinated when the tip of the radicle had emerged free of the seed coat [22]. Parameters including the final germination percentage (GP), and corrected germination rate index (CGRI) were recorded according to Esechie [23] and reported after 10 days of cultivation. The amount of time (days) required to reach 50% germination (GT50) was recorded according to Hsu et al. [24].
2.4 Experimental Design and Statistical Analysis
All experiments proceeded in a completely randomized design. Five conditions were replicated three times, and each replicate comprised a Petri dish containing 50 seeds. Ratios (%) were arcsine transformed before analyses. Data were shown and compared as means of 3 biological replicates ± standard error of means. The collected data were analyzed using two-way analysis of variance (ANOVA) via SPSS 9.2 software. Means were compared using least significant difference (LSD) method (P ≤ 0.05) for each factor (cultivar and salt concentration) and their interaction (cultivar × salt concentration).
3.1 Final Germination Percentage
The two-way ANOVA revealed that both single factors and their interaction significantly affect the GP of the studied alfalfa cultivars. Regardless of NaCl concentration, Hassawi, Kasimi, Berlin, Oscarde Star, and Saltine cultivars showed the highest GP among the 12 cultivars (P < 0.001). Server cultivars showed the lowest germination percentage (28.82 ± 18.41); however, the high standard deviation value indicates the high variability of GP observed for these cultivars under control or salt-stressed conditions (Table 2). These results are clearly supported by the significant reduction observed in the average GP of all cultivars under different NaCl concentration. Approximately 62.7% reduction in GP was observed under 250 mmol L−1 NaCl as compared to control. Indeed, a gradual decrease of GP was observed with increasing NaCl concentration indicating the expected adverse effects of salinity on germination percentages in all cultivars.
The interaction effect of cultivars and salinity concentrations on GP are presented in Table 3. Increasing salinity concentrations resulted in a gradual decrease in the ratios of seeds germinated by all cultivars (Table 2). However, the amounts of reductions varied among the cultivars. The most affected cultivars were Server, Magic, WG CAL 10 and Cuf 101 with GP reductions of 88.70%, 79.89%, 79.17% and 79.10%, respectively, at 250 mmol L−1 compared with the control (0 mmol L−1). In contrast, the Berlin and Kasimi cultivars were the most tolerant to salinity, with GP reductions of 27.43% and 35.92% at 250 mmol L−1 NaCl compared with the control. In most cultivars, concentrations < 150 mmol L−1 NaCl did not produce significant changes in the GP as compared to control (0 mmol L−1 NaCl). Nevertheless, 200 mmol L−1 NaCl caused significant differences in some cultivars, e.g., WG CAL 10 and Server (77.08% and 67.19% GP reduction, respectively). At 250 mmol L−1 NaCl, the GP significantly differed among the cultivars, with the GP being the highest and lowest in Kasimi and Server cultivars, respectively (55.20% vs. 5.74%; Table 3).
3.2 Corrected Germination Rate Index
We calculated the CGRI for the studied alfalfa cultivars under various salinity levels as an indicator of germination speed. The Kasimi cultivar showed the highest CGRI (193.93 ± 42.70) among all studied cultivars with about 15% increase comparing to the following Hassawi cultivar which showed the second highest CGRI (Table 2). Similar to GP, a significant gradual reduction in average CGRI of all cultivars was observed with increased salinity levels. CGRI was reduced by roughly 75% under 250 mmol L−1 NaCl as compared to control conditions.
Variations among the cultivars at salinity levels < 200 mmol L−1 NaCl were observed. However, the average CGRI of all cultivars were significantly reduced when the seeds were incubated with 200 and 250 mmol L−1 NaCl. The average CGRI of control plants was 225.07, whereas the average CGRI of plants incubated with 200 and 250 mmol L−1 NaCl were 96.70 and 66.41, respectively. The least affected cultivar in terms of CGRI was the Kasimi cultivar, with 48.30% reduction when seeds were incubated with 250 mmol L−1 NaCl compared with the control. In contrast, the Cuf 101 cultivar was the most affected (84.73% reduction in CGRI when seeds were incubated with 250 mmol L−1 NaCl compared with the control). The CGRI at 250 mmol L−1 NaCl was the highest and lowest in the Berlin and Server cultivars (123.10 vs. 28.80; Table 4).
3.3 Number of Days Required to Reach 50% Germination
The number of days required to reach 50% germination (GT50), which also reflected germination speed, was calculated. Kasimi cultivar showed the lowest GT50 among all the studied cultivars (Table 2). On the other hand, Server cultivar had the longest GT50. Regardless of cultivar, increasing NaCl concentration from 0 to 250 mmol L−1 prolonged the GT50 values by 4.5 days. However, higher standard deviation values were observed at 250 mmol L−1 concentration indicating the variation in cultivars response to salinity stress.
The GT50 varied considerably among cultivars, especially between 200 and 250 mmol L−1 NaCl (Table 5). The average GT50 values of all cultivars incubated without NaCl (0 mmol L−1) and with 250 mmol L−1 NaCl were 1.75 and 6.29 days, respectively. The GT50 was the longest in the Server, Cuf 101, and Magic cultivars incubated with 250 mmol L−1 NaCl (8.21, 7.49 and 7.11 days, respectively). The changes in GT50 between control and 250 mmol L−1 NaCl was the lowest and highest in the Kasimi and Server cultivars with respective differences of 2.18 and 6.02 days.
3.4 Ability of Alfalfa Cultivars to Produce Cotyledonary and True Leaves under Varied NaCl Concentrations
The GP, CGRI and GT50 findings showed that all cultivars were mostly affected by 200 and 250 mmol L−1 NaCl. Therefore, we assessed the ratios (%) of cotyledonary (CL) and true (TL) leaf formations under both conditions (Fig. 1). At 200 mmol L−1 NaCl, 30% to 90% of plants in each cultivar created CL. The ratios of CL were the lowest in the WG CAL 10 and Server cultivars, which also did not produce TL at 200 mmol L−1 NaCl (Fig. 1A). Kasimi and Hassawi were the most effective among the cultivars in terms of forming CL and TL at 200 mmol L−1 NaCl. More than 60% of Saltine, Super 10, Oscarde Star and Vulcan individuals formed CL, but significantly less TL either at 200 or 250 mmol L−1 NaCl (Fig. 1B). Moreover, the Super 10 cultivar did not form TL under 250 mmol L−1 (about 55% of the seeds formed CL). Overall, incubation with 250 mmol L−1 NaCl decreased the ability of all tested cultivars to form TL. Nevertheless, the Kasimi and Hassawi cultivars both formed TL with similarly high efficiency. The Berlin cultivar formed more CL than Hassawi cultivars but could not form as many TL as the Hassawi and Kasimi cultivars.
Figure 1: Ratios (%) of cotyledonary and true leaves of 12 alfalfa cultivars after 10 days of seed cultivation with (A) 200 and (B) 250 mmol L−1 of NaCl (Bars are means ± standard error)
Salinity is one of the most important environmental stresses that adversely affect global plant growth and productivity. Response of plants to salinity stress varied based on the development stage; however, it is well established in the majority of crop plants that the most salt-sensitive stages are seed germination and seedling establishment [25]. In vitro germination analysis has been applied previously to assess the salinity tolerance of alfalfa [17,19,20,25]. These reports indicated high variation in germination of alfalfa seeds under salinity stress. In general, different alfalfa cultivars germinate effectively when suitable temperatures and adequate moisture are available [14]. Nevertheless, increasing salinity levels may adversely affect alfalfa seeds’ germination percentage [26,27]. The present findings revealed considerable variations in GP among 12 alfalfa cultivars. Alfalfa has moderate salinity tolerance. Increasing salinity concentrations up to 200 and 250 mmol L−1 NaCl led to reductions in the average GP of all cultivars. These results confirm previous findings of salinity effects on GP among alfalfa cultivars [14,19,28,29] as well as rice [30] and the halophyte quinoa [31]. Our results indicated that the local cultivars (Kasimi and Hassawi) showed salinity tolerance comparable to the tolerant German cultivar (Berlin). The inhibitive effects of salinity on germination could be attributed to several factors including limited water availability, ionic toxicity of Na+ and Cl− ions on seed embryos, protein denaturation, alteration of carbohydrate metabolism, and reduction of absorbed water amounts [29,32].
In terms of CGRI, our results showed that the Kasimi cultivar was the most salt tolerant, whereas Saltine and Server cultivars were very sensitive to increasing salinity concentrations. Kasimi and Hassawi are Saudi cultivars with relatively high tolerance to abiotic stresses such as salinity and drought. This fact was supported by the present findings because these cultivars had the shortest GT50 regardless of salinity, indicating rapid germination rates even at 250 mmol L−1 NaCl. In a recent study, Kankarla et al. [25] found that germination index of alfalfa seeds was adversely affected by soil salinity. Previous studies indicated the positive relation between crop’s ability to germinate under severe salinity conditions and the potential good performance under salinity stress in greenhouse and/or open field conditions [14].
The Kasimi and Hassawi cultivars formed the most TL, indicating good survival under conditions of high salinity. The Kasimi, Berlin and Hassawi cultivars were the least affected by salinity stress and thus are more tolerant to salinity than the other cultivars studied. Previous studies confirmed high salinity tolerance in alfalfa cultivars [23,33,34]. However, deeper analysis of crop productivity may confirm the salinity tolerance levels of those cultivars. It was shown salinity levels observed at the initial growth stages under laboratory conditions differ from those found under field conditions in other crops, e.g., Sorghum bicolor [35]. The identification of salinity tolerance in vitro is widely accepted and has been applied to several plants. The current study confirmed the high salinity tolerance of the Saudi alfalfa cultivars Kasimi and Hassawi based on their germination and survival rates under various NaCl concentrations.
In conclusion, we assessed salinity tolerance in alfalfa in vitro to accurately identify sensitive and tolerant cultivars. The Saudi alfalfa cultivars, Kasimi and Hassawi were highly tolerant of NaCl stress with only a slight decrease in germination indicators and high survival rates at high NaCl concentrations. Adapting the tolerant cultivars would enhance the production of forage alfalfa and save fresh water by providing potential for irrigation with relatively low salinity level. Further studies are needed to determine the effects of irrigation with saline water on the later growth stages of these cultivars, as well as biomass accumulation and yield.
Acknowledgement: The authors extend their appreciation to Researchers Supporting Project No. (RSP-2021/390), King Saud University, Riyadh, Saudi Arabia.
Authorship: All authors contributed equally for the conception and writing of the manuscript. All authors critically revised the manuscript and approved the final version.
Funding Statement: This research was funded by the Researchers Supporting Project No. (RSP-2021/390), King Saud University, Riyadh, Saudi Arabia.
Conflicts of Interest: The authors declare that they have no conflicts of interest to report regarding the present study.
References
1. Chinnusamy, V., Jagendorf, A., Zhu, J. K. (2005). Understanding and improving salt tolerance in plants. Crop Science, 45(2), 437–448. DOI 10.2135/cropsci2005.0437. [Google Scholar] [CrossRef]
2. Rengasamy, P. (2016). Soil chemistry factors confounding crop salinity tolerance—A review. Agronomy, 6(4), 53. DOI 10.3390/agronomy6040053. [Google Scholar] [CrossRef]
3. Pariente, S. (2001). Soluble salts dynamics in the soil under different climatic conditions. Catena, 43(4), 307–321. DOI 10.1016/S0341-8162(00)00130-2. [Google Scholar] [CrossRef]
4. Tani, E., Sarri, E., Goufa, M., Asimakopoulou, G., Psychogiou, M. et al. (2018). Seedling growth and transcriptional responses to salt shock and stress in Medicago sativa L., Medicago arborea L., and their hybrid (Alborea). Agronomy, 8(10), 231. DOI 10.3390/agronomy8100231. [Google Scholar] [CrossRef]
5. Ismail, A., Takeda, S., Nick, P. (2014). Life and death under salt stress: Same players, different timing? Journal of Experimental Botany, 65(12), 2963–2979. DOI 10.1093/jxb/eru159. [Google Scholar] [CrossRef]
6. Apse, M. P., Aharon, G. S., Snedden, W. A., Blumwald, E. (1999). Salt tolerance conferred by overexpression of a vacuolar Na+/H+ antiport in Arabidopsis. Science, 285(5431), 1256–1258. DOI 10.1126/science.285.5431.1256. [Google Scholar] [CrossRef]
7. Soufan, W., Okla, M. K., Salamatullah, A., Hayat, K., Abdel-Maksoud, M. A. et al. (2021). Seasonal variation in yield, nutritive value, and antioxidant capacity of leaves of alfalfa plants grown in arid climate of Saudi Arabia. Chilean Journal of Agricultural Research, 81(2), 182–190. DOI 10.4067/S0718-58392021000200182. [Google Scholar] [CrossRef]
8. Munns, R., Tester, M. (2008). Mechanisms of salinity tolerance. Annual Review of Plant Biology, 59(1), 651–681. DOI 10.1146/annurev.arplant.59.032607.092911. [Google Scholar] [CrossRef]
9. Li, R., Shi, F., Fukuda, K., Yang, Y. (2010). Effects of salt and alkali stresses on germination, growth, photosynthesis and ion accumulation in alfalfa (Medicago sativa L.). Soil Science & Plant Nutrition, 56(5), 725–733. DOI 10.1111/j.1747-0765.2010.00506.x. [Google Scholar] [CrossRef]
10. Yang, Q., Wu, M., Wang, P., Kang, J., Zhou, X. (2005). Cloning and expression analysis of a vacuolar Na+/H+ antiporter gene from Alfalfa. DNA Sequence, 16(5), 352–357. DOI 10.1080/10425170500272742. [Google Scholar] [CrossRef]
11. Ferreira, J. F. S., Cornacchione, M. V., Liu, X., Suarez, D. L. (2015). Nutrient composition, forage parameters, and antioxidant capacity of alfalfa (Medicago sativa L.) in response to saline irrigation water. Agriculture, 5(3), 577–597. DOI 10.3390/agriculture5030577. [Google Scholar] [CrossRef]
12. Graham, P. H., Vance, C. P. (2003). Legumes: Importance and constraints to greater use. Plant Physiology, 131(3), 872–877. DOI 10.1104/pp.017004. [Google Scholar] [CrossRef]
13. Mills, D., Benzioni, A. (1992). Effect of NaCl salinity on growth and development of Jojoba clones: 11. nodal segments grown in vitro. Journal of Plant Physiology, 139(6), 737–741. DOI 10.1016/S0176-1617(11)81720-7. [Google Scholar] [CrossRef]
14. Scasta, J. D., Trostle, C. L., Foster, M. A. (2012). Evaluating alfalfa (Medicago sativa L.) cultivars for salt tolerance using laboratory, greenhouse and field methods. Journal of Agricultural Science, 4(6), 90–103. DOI 10.5539/jas.v4n6p90. [Google Scholar] [CrossRef]
15. Carlson Jr., J. R., Ditterline, R. L., Martin, J. M., Sands, D. C., Lund, R. E. (1983). Alfalfa seed germination in antiobiotic agar containing NaCl. Crop Science, 23(5), 882–885. DOI 10.2135/cropsci1983.0011183X002300050016x. [Google Scholar] [CrossRef]
16. Croughan, T. P., Stavarek, S. J., Rains, D. W. (1978). Selection of a NaCl tolerant line of cultured alfalfa cells. Crop Science, 18(6), 959–963. DOI 10.2135/cropsci1978.0011183X001800060012x. [Google Scholar] [CrossRef]
17. Smith, M., McComb, J. (1983). Selection for NaCl tolerance in cell cultures of Medicago sativa and recovery of plants from a NaCl-tolerant cell line. Plant Cell Reports, 2(3), 126–128. DOI 10.1007/BF00269335. [Google Scholar] [CrossRef]
18. Smith, M. K., McComb, J. (1981). Use of callus cultures to detect NaCl tolerance in cultivars of three species of pasture legumes. Functional Plant Biology, 8(5), 437–442. DOI 10.1071/PP9810437. [Google Scholar] [CrossRef]
19. Dragiiska, R., Djilianov, D., Denchev, P., Atanassov, A. (1996). In vitro selection for osmotic tolerance in alfalfa (Medicago sativa L.). Bulgarian Journal of Plant Physiology, 22(3–4), 30–39. [Google Scholar]
20. Campanelli, A., Ruta, C., Morone-Fortunato, I., Mastro, G. (2013). Alfalfa (Medicago sativa L.) clones tolerant to salt stress: In vitro selection. Open Life Sciences, 8(8), 765–776. DOI 10.2478/s11535-013-0194-1. [Google Scholar] [CrossRef]
21. Murashige, T., Skoog, F. (1962). A revised medium for rapid growth and bio assays with tobacco tissue cultures. Physiologia Plantarum, 15(3), 473–497. DOI 10.1111/j.1399-3054.1962.tb08052.x. [Google Scholar] [CrossRef]
22. Dewir, Y. H., El-Mahrouk, M. E. S., Naidoo, Y. (2011). Effects of some mechanical and chemical treatments on seed germination of Sabal palmetto and Thrinax morrisii palms. Australian Journal of Crop Science, 5(3), 248–253. [Google Scholar]
23. Esechie, H. A. (1994). Interaction of salinity and temperature on the germination of sorghum. Journal of Agronomy and Crop Science, 172(3), 194–199. DOI 10.1111/j.1439-037X.1994.tb00166.x. [Google Scholar] [CrossRef]
24. Hsu, F., Nelson, C., Matches, A. (1985). Temperature effects on germination of perennial warm-season forage grasses. Crop Science, 25(2), 215–220. DOI 10.2135/cropsci1985.0011183X002500020005x. [Google Scholar] [CrossRef]
25. Kankarla, V., Shukla, M. K., Picchioni, G. A., VanLeeuwen, D., Schutte, B. J. (2020). Germination and emergence responses of alfalfa, triticale and quinoa irrigated with brackish groundwater and desalination concentrate. Agronomy, 10(4), 549. DOI 10.3390/agronomy10040549. [Google Scholar] [CrossRef]
26. Soufan, W., Al-Suhaibani, N., Tillmann, P., Okla, M. K., Abdel-Salam, E. M. (2020). Changes in yield and forage quality of aix alfalfa cultivars in response to sustainable irrigation with treated wastewater. International Journal of Agriculture and Biology, 24(5), 1286–1292. [Google Scholar]
27. Soufan, W., Okla, M. K., Al-Ghamdi, A. A. (2019). Effects of irrigation with treated wastewater or well water on the nutrient contents of two alfalfa (Medicago sativa L.) cultivars in Riyadh, Saudi Arabia. Agronomy, 9(11), 729. DOI 10.3390/agronomy9110729. [Google Scholar] [CrossRef]
28. Dezfuli, A. A. S., Dehcheshmeh, S. M., Boroujeni, F. R., Shiran, B. (2016). Evaluation of salinity tolerance of alfalfa genotypes during germination stage using multivariate analysis. Journal of Applied Environmental and Biological Sciences, 6(3), 51–56. [Google Scholar]
29. Ansari, M., Shekari, F., Mohammadi, M. H., Biró, B., Végvári, G. (2017). Improving germination indices of alfalfa cultivars under saline stress by inoculation with beneficial bacteria. Seed Science and Technology, 45(2), 475–484. DOI 10.15258/sst.2017.45.2.02. [Google Scholar] [CrossRef]
30. Hosseini, S. J., Sarvestani, Z. T., Pirdashti, H. (2012). Analysis of tolerance indices in some rice (Oryza sativa L.) genotypes at salt stress condition. International Research Journal of Applied and Basic Sciences, 3(1), 1–10. [Google Scholar]
31. Panuccio, M. R., Jacobsen, S. E., Akhtar, S. S., Muscolo, A. (2014). Effect of saline water on seed germination and early seedling growth of the halophyte quinoa. AoB PLANTS, 6, plu047. DOI: 10.1093/aobpla/plu047. [Google Scholar] [CrossRef]
32. Jahromi, F., Aroca, R., Porcel, R., Ruiz-Lozano, J. M. (2007). Influence of salinity on the in vitro development of Glomus intraradices and on the in vivo physiological and molecular responses of mycorrhizal lettuce plants. Microbial Ecology, 55(1), 45. DOI 10.1007/s00248-007-9249-7. [Google Scholar] [CrossRef]
33. Hussain, G., Al-Jaloud, A. A., Ai-Shammary, S. F., Karimulla, S. (1995). Effect of saline irrigation on the biomass yield, and the protein, nitrogen, phosphorus, and potassium composition of alfalfa in a pot experiment. Journal of Plant Nutrition, 18(11), 2389–2408. DOI 10.1080/01904169509365073. [Google Scholar] [CrossRef]
34. Badran, A., ElSherebeny, E. A., Salama, Y. (2015). Performance of some alfalfa cultivars under salinity stress conditions. Journal of Agricultural Science, 7(10), 281–290. DOI 10.5539/jas.v7n10p281. [Google Scholar] [CrossRef]
35. Rajabi Dehnavi, A., Zahedi, M., Ludwiczak, A., Cardenas Perez, S., Piernik, A. (2020). Effect of salinity on seed germination and seedling development of sorghum (Sorghum bicolor (L.) Moench) genotypes. Agronomy, 10(6), 859. DOI 10.3390/agronomy10060859. [Google Scholar] [CrossRef]
Cite This Article
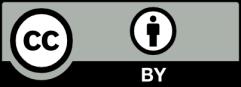