Open Access
ARTICLE
Transcriptome Analysis and Physiological Responses of Economic Macroalga Gracilariopsis lemaneiformis under Sulfate Deficiency
1
Southern Marine Science and Engineering Guangdong Laboratory (Guangzhou), Guangzhou, 511458, China
2
Institute of Marine Sciences, Guangdong Provincial Key Laboratory of Marine Biotechnology and STU-UNIVPM Joint Algal
Research Center, College of Sciences, Shantou University, Shantou, 515063, China
* Corresponding Author: Hong Du. Email:
(This article belongs to the Special Issue: Plant Omics in Challenging Environment)
Phyton-International Journal of Experimental Botany 2023, 92(1), 91-110. https://doi.org/10.32604/phyton.2022.022663
Received 20 March 2022; Accepted 25 April 2022; Issue published 06 September 2022
Abstract
Sulfur is an essential macronutrient for the growth of all photosynthetic organisms and plays important roles in different metabolic pathways. However, sulfur metabolism and its related research on macroalgae with important ecological value is rather limited. In this study, marine ecological valued macroalga Gracilariopsis lemaneiformis was used to study the general physiological responses and transcriptome profiling under the sulfate deficiency. The relative growth rate of algae under sulfate deficiency was statistically significantly lower than that of control after 6 days. However, no significant differences were observed in the pigments content and Fv/Fm value, indicating that the photosynthesis was not obviously affected under the sulfate deficiency. Furthermore, the significantly increased soluble protein and carbohydrate contents, and indistinctively differentially expressed sulfate transporter/permease (ST) and ATP sulfurylase (ATPS) genes suggesting that G. lemaneiformis cells did not use sulfur from internal protein and carbohydrate pools but utilize stored sulfur from vacuole to satisfy their nutritional requirements during the sulfate deficiency. Transcriptome data showed that most annotated differentially expressed genes (DEGs) were down-regulated under the sulfate deficiency, including a large proportion of genes involved in the pathway of sulfur metabolism. Therefore, it was concluded that the pathway of sulfur metabolism was suppressed under the sulfate deficiency in G. lemaneiformis. The results and analysis in this study provide a comprehensive understanding for the physiological and molecular response of G. lemaneiformis to sulfate deficiency.Keywords
Sulfur is an essential macronutrient for all photosynthetic organisms [1]. Simultaneously, sulfur is one of the most abundant elements in the cells of photosynthetic organisms [2]. The major form of sulfur acquired by photosynthetic organisms is inorganic sulfate. Inorganic sulfate is firstly transported into the cells via sulfate transporter/permease. Intracellular sulfate will be activated by ATP sulfurylase (ATPS) and to form adenosine 5’-phosphosulfate (APS). Afterwards, APS is used for the synthesis of sulfur-containing metabolites through the reductive or phosphorylated pathways, such as amino acids (e.g., cysteine and methionine) and its derivatives (e.g., methionine and glutathione), vitamins (e.g., biotin and thiamine), and sulfated polysaccharides (e.g., agar and carrageenan) [3–5]. In contrast to the low sulfate concentration in freshwater and terrestrial ecosystems (10-50 μM), the sulfate concentration in the marine ecosystem remains stable and can reach 25–28 mM [1]. It was proposed that high sulfate concentration is an evolutionary driver in the expansion of modern marine phytoplankton [6]. Therefore, what are the effects of sulfate deficiency on the marine algae?
Sulfur metabolism and its related research had been extensively studied in different algae [5,7–11]. Among these studies, transcriptome responses of algae under sulfate deficiency mainly focus on some model algae, such as green alga Chlamydomonas reinhardtii, cyanobacteria Synechocystis sp. and diatom Emiliania huxleyi [1,12–14]. Previous transcriptome data showed that genes encoding sulfate transporters and extracellular arylsulfatase were up-regulated, the reductive capacity of sulfate was enhanced, the metabolism of amino acids/metabolites containing sulfur was reinforced during sulfate deficiency [15]. Additionally, a recent study indicated that sulfur deprivation can significantly influence microalgal physiological characterizations, including the content of metabolites (e.g., starch, protein and alcohol), photosynthetic rate, the maximum effective quantum yield of photosystem II, the activities of antioxidant enzymes and metabolic pathways [16–18]. However, the study on physiological and transcriptome responses of macroalgae with important ecological value is rather limited [5]. Consequently, it is very curious to study if different algae, especially between microalgae and economical macroalgae, have diverse responses to sulfate deficiency?
Gracilariopsis lemaneiformis is widely cultured along the coasts of China, and it is a red macroalga of great economic value for the biotechnology industry because it produces raw materials, such as agar [19,20]. It was reported that Gracilariopsis genus account for 60–80% of the total agar production [21]. Besides, G. lemaneiformis can also be used as food for human and fish, and plays an important role in bioremediation [22,23]. In this study, biochemical methods were used to analyze the general physiological responses (growth rate, the contents of pigments, soluble protein and soluble carbohydrate) of G. lemaneiformis. The growth rate was significantly reduced, the contents of pigments were not obviously changed, while the contents of soluble protein and carbohydrate were significantly increased under the sulfate deficiency of G. lemaneiformis. Furthermore, next-generation sequencing was used to obtain a transcriptome profiling of G. lemaneiformis responses to sulfate deficiency. The transcriptome data presented that the sulfur metabolism pathway was suppressed under the sulfate deficiency. The results and analysis in this study provide a comprehensive understanding for the physiological and molecular responses of G. lemaneiformis to sulfate deficiency.
2.1 Algal Growth and Treatments
Healthy G. lemaneiformis with dark red and dense branches were pre-cultured for two weeks under the following conditions: temperature 20°C, photoperiod Light:Dark = 14 h:10 h, light intensity 50–60 μmol photons m−2 s−1. After preculture, 2 g of healthy G. lemaneiformis were cultured in 1 L of sterile artificial seawater using 3 L conical flask. In control group (sulfate sufficiency) 25 mmol L−1 sulfate was used, while sulfate was not added in the sulfate deficiency group. Samples and control groups were cultured in triplet. The seawater was changed in each two days with freshly filtered sterile seawater during the period of culture.
2.2 Physiological Measurements
Algae were collected, dried, and weighed after the culturing of 0, 2, 4, 8, 10, 12, 14 and 16 days. The relative growth rate was calculated by the formula: [(Wt/W0)1/t−1] × 100%, Wt is the fresh weight of algae at time t (g), while W0 is the fresh weight of algae at the beginning (g), t is the time between the two-detection time (day) [20,24].
After the culture of 0, 8 and 16 days, 100 mg dried algae were collected and finely powdered in liquid nitrogen using a mortar. Powdered samples were used to analyze the physiological data. Phycoerythrin (PE), chlorophyll a, soluble carbohydrate and soluble protein were measured via UV-VIS spectrophotometry at different wavelengths [23,25]. The maximum effective quantum yield of photosystem II (PSII) was determined by Fv/Fm value. The Fv/Fm ratio was measured with a pulse amplitude modulated (PAM) fluorometer (Imaging PAM, Walz, Effelrich, Germany) as introduced in our previous papers [23,24,26]. After the culture of 0, 8 and 16 days, 2 mg algae were collected and dried to constant weight at 60°C. Dried samples were well powdered in liquid nitrogen using a mortar. Finally, the samples were used to analyze the amounts of C, N and S elements by elemental analyzer (Elementar Analysensysteme GmbH, Hanau, Germany).
2.3 Data Statistics and Analysis
All the original data were calculated and statistical analyzed with SPSS 20.0 software for windows. Significant differences among different groups were analyzed by student’s t test (α = 0.05). All data were calculated with three biological replicates and reported as the means ± SD.
2.4.1 RNA Isolation, Library Preparation and Sequencing
The total RNA from each sample (16th day culture) was extracted using the DP432 kit (Tiangen Biochemical Technology Co., Ltd., Beijing, China) following manufacturer’s recommendation. RNA quality and concentration were measured by a 2100 Bioanalyzer at 260 nm and 280 nm. RNA integrity number of all samples was above 6.0. Isolated RNA was used for sequencing and qRT-PCR (Quantitative Reverse Transcriptase Polymerase Chain Reaction) analysis.
A total of 1 µg RNA per sample was used to generate paired-end RNA-seq library using NEBNext®Ultra™ RNA Library Prep Kit for Illumina® (NEB, USA). The library fragments were purified with AMPure XP system (Beckman Coulter, Beverly, USA). The library quantity was assessed using the Agilent Bioanalyzer 2100 system.
2.4.2 Quality Controls, De Novo Assembly and Clustering
The clustering of the index-coded samples was performed on a cBot Cluster Generation System using TruSeq PE Cluster Kit v3-cBot-HS (Illumia) according to the manufacturer’s instructions. After cluster generation, the library was sequenced on an Illumina Hiseq 2000 platform.
Based on the technology of edge synthesis and edge sequencing (Sequencing By Synthesis, SBS), the cDNA library was sequenced using Illumina Hiseq high throughput sequencing platform. The raw data was filtered to remove the connector sequence and low-quality reads to obtain clean data with high quality. After obtaining high quality sequencing data, trinity platform was used for transcriptome assembly.
2.4.3 Gene Function Annotation and Classification
The Unigene sequences were compared with NR, Swiss-Prot, GO, COG, KOG, eggNOG, KEGG by using BLAST software. KEGG Orthology results of Unigene in KEGG were obtained by using KOBAS2.0. After predicting the amino acid sequences of Unigene, the comment information of Unigene was obtained by comparing HMMER software with Pfam database. The E-value for BLAST parameter was smaller than 1e–5, for HMMER parameter was smaller than 1e–10. Finally, Unigenes with annotated information were obtained.
2.4.4 Identification of Differentially Expressed Genes (DEGs)
DESeq2 was used to analyze the differentially expressed genes among the samples. During the process of differential expression analysis, the recognized effective Benjamini-Hochberg method was used to correct the significant P value obtained from the original hypothesis test. The key index of gene screening was to reduce the false positive caused by independent statistical hypothesis test of many gene expression values. The screening criterion was as following: the false discovery rate (FDR) was less than 0.001, the difference multiple Fold Change (FC) was greater than or equal to 2. FC represents the ratio of expression levels between the two groups.
Verification of DEGs was carried out on the same RNA samples for RNA-Seq experiment. Totally, the expression levels of nine unigenes were verified by qRT-PCR. The primers were shown in Table S2. 1 mL RNA with high quality was used to synthesize cDNA via KP118-02 kit (Tiangen Biochemical Technology Co., Ltd., Beijing, China). The expression levels of genes were measured with a BIO-RAD-T100 using Talent qPCR PreMix (SYBE Green) (Tiangen Biochemical Technology Co., Ltd., Beijing, China) according to the manufacturer’s instructions. The experiments were done as following: 95°C for 3 min, followed by 40 cycles of amplification (95°C for 5 s and 60°C for 10 s, 72°C for 15 s). 2−ΔΔCt method was used to obtain the raw Ct values [5,27]. Finally, the expression levels of these selected genes from qRT-PCR were compared to that of differentially expressed genes (DEGs) from RNA-Seq.
The authors confirmed that the ethical policies of the journal, as noted on the journal’s author guidelines page, have been adhered to. No ethical approval was required as macroalga was used as material in this study.
3.1 Physiological Responses of G. lemaneiformis under Sulfate Deficiency
The relative growth rate of G. lemaneiformis under sulfate sufficiency and deficiency was shown in Fig. 1. During the first 6 days, the relative growth rate did not show significant difference between sulfate sufficiency and deficiency (P > 0.05). However, the relative growth rate of algae under sulfate deficiency was significantly lower than that of sulfate sufficiency after 6 days (P < 0.05). Consistent phenomenon was observed in other algae. For example, the growth of cells were severely diminished during sulfur limitation in the green microalga Chlamydomonas reinhardtii [28]. Compared to sulfur sufficiency, sulfur limitation/deprivation reduced the growth rate of the halotolerant chlorophyte Dunaliella salina [17,29]. These results indicated that sulfur is an essential macronutrient for the growth of algae.
Figure 1: Relative growth rate of G. lemaneiformis under sulfate deficiency. *represents significant difference between sulfate sufficiency and deficiency, **represents extremely significantly difference between sulfate sufficiency and deficiency
The changes of chlorophyll a, phycoerythrin content, Fv/Fm value, photosynthetic oxygen release capacity, soluble carbohydrate content and soluble protein of G. lemaneiformis were shown in Fig. 2 under sulfate sufficiency and deficiency conditions. Considering the invariable color of algae during sulfate deficiency, the contents of the photosynthetic pigment chlorophyll a and phycoerythrin were analyzed. Although the content of chlorophyll a was gradually increased during the culture, compared to that of sulfate sufficiency the accumulation of chlorophyll a was not significantly difference on the 8th and 16th days of culture in sulfate deficiency (P > 0.05) (Fig. 2A). Chlorophyll a content was also increased under the sulfur deprivation in D. salina and higher than that on the 8th day of culture in sulfur sufficiency [17]. These results implicated that there was a similar defense mechanism between D. salina and G. lemaneiformis during sulfate deficiency. However, total chlorophyll content was found to be unaffected by the sulfur limitation in D. salina [29]. The PE content showed no significant difference between sulfate sufficiency and deficiency on days 0, 8 and 16 (Fig. 2B). However, compared to the beginning of culture chlorophyll and PE contents were increased on the 8th and 16th days. PE is a major phycobiliprotein in red macroalgae, which works on the capture of light and transfer the light to photosystem II. Owing to its antioxidant property, anti-tumor activity and solubility in water, PE was used in different industries, including cosmetic, clinical diagnostics and food industries and so on [30]. It is well known that phycoerythrin plays a decisive role in the red color of normal G. lemaneiformis. Therefore, the content of PE without significant difference explained the invariable color of algae under the sulfate deficiency.
Figure 2: Physiological data of G. lemaneiformis under sulfate deficiency (A) is chlorophyll a content, (B) is phycoerythrin (PE) content, (C) is Fv/Fm, (D) is photosynthetic oxygen release capacity, (E) is the content of soluble protein, (F) is the content of soluble carbohydrate. *represents significant difference between sulfate sufficiency and deficiency, **represents extremely significantly difference between sulfate sufficiency and deficiency
The value of Fv/Fm under the sulfate deficiency was reduced during the culture, however, it was not significantly different to that on the 8th and 16th days of the sulfate sufficiency (P > 0.05) (Fig. 2C). It was reported that sulfur limitation or deprivation affected the photosynthetic efficiency and structures of the photosynthetic apparatus, and resulted in the reduction of photosynthetic capacity and the decrease of proteins related with photosynthesis (e.g., rubisco and chlorophyll a/b binding proteins) in algae [29,31,32]. Light-saturated photosynthesis rate was declined in D. salina in S-deprived medium [18]. These reports explained the reduced Fv/Fm value under the sulfate deficiency in G. lemaneiformis. The data of photosynthetic oxygen release showed that the photosynthetic oxygen release in the sulfate deficiency was significantly higher than that in the sulfate sufficiency group on the 8th day (P < 0.05). However, the photosynthetic oxygen release in the sulfate deficiency was observably lower than that in the sulfate sufficiency group on the 16th day (P < 0.05) (Fig. 2D). These results were thought to be a specific response necessary to deal with the S limitation. However, there are still no references to explain why the results of Fv/Fm and photosynthetic oxygen release are so different.
The soluble protein data showed that the content was increased, especially, the content of soluble protein under the sulfate deficiency was prominently higher than that of sulfate sufficiency on the day 16 (P < 0.05) (Fig. 2E). Sulfate deficiency resulted in the increase of soluble protein content in G. lemaneiformis, similarly to the case in autotrophic Galdieria phlegrea, but not in heterotrophic G. phlegrea [9], Chlorella sorokiniana [8] and Chlamydomonas reinhardtii [33]. The content of soluble carbohydrate was gradually decreased during sulfate sufficiency and sulfate deficiency (Fig. 2F). It was shown that the content of soluble carbohydrate during sulfate deficiency was significantly higher than that in sulfate sufficiency on the day 16th (P < 0.05). Soluble protein and carbohydrate were significantly increased, it could be proposed that the sulfate deficiency cells of G. lemaneiformis did not utilize S from the internal protein and carbohydrate pools to redistribute the resource in order to satisfy their nutritional requirements [34]. It was reported that excess sulfate will be stored in the vacuole [31]. Therefore, our results indicated that G. lemaneiformis cells used the stored sulfate from algal vacuoles during sulfate deficiency.
As shown in Fig. 3, the percentage of sulfur under sulfate deficiency was significantly lower than that under the condition of sulfate sufficiency on the day 8th and 16th (P < 0.05) (Fig. 3A). However, no significant difference of total C percentage, total N percentage and C/N ratio were observed between the two groups (Figs. 3B and 3C). Similar results were also observed in a previous paper where showed that total C and N were not strongly affected, while total S was decreased under S starvation in autotrophic microalga Galdieria phlegrea [9]. Our results suggested that sulfur, but not carbon and nitrogen was significantly affected by sulfate deficiency in G. lemaneiformis.
Figure 3: Elements of G. lemaneiformis under sulfate deficiency (A) is total sulfur percent, (B) is total nitrogen percent, (C) is total carbon percent, (D) is the ratio of total carbon/ nitrogen. *represents significant difference
3.2 General Transcriptome Analysis of G. lemaneiformis Response to Sulfate Deficiency
Because no-reference genome can be used in this transcriptome analysis, clean reads obtained from this project were assembled into different length contigs using overlap information until the contigs could not be extended anymore [35]. After the assembling, 55,443 transcripts and 39,440 unigenes (>300 bp) were generated, with an N50 of 4,484 and 2,513 bp, respectively. Besides, it was shown that the length of transcripts and unigenes was mainly focused on the range of 300–500 bp. The total lengths of transcripts and unigenes were respective 107,954,061 and 48,908,123 bp, with a mean length of 1,947 and 1,240 bp.
3.2.1 Functional Categories of Genes Affected by Sulfate Deficiency in G. lemaneiformis
Comparing unigene sequences with major databases, a total of 25,023 unigenes were commented on GO, COG, KEGG, KOG, Pfam, Swissprot, eggNOG and NR database to 13,367 (53.42%), 11,759 (46.99%), 12,938 (51.70%), 16,725 (66.84%), 24,389 (97.47%), 14,216 (56.81%), 21,202 (84.73%) and 22,905 (91.54%), respectively.
The distribution of GO, KOG and KEGG annotation was shown in Fig. 4. 13,667 unigenes were annotated in the GO database. In the cellular component, most of the unigenes were involved in the cell, cell part and organelle. In the molecular function, unigenes were mostly related to catalytic activity, binding, and structural molecular activity. In the biological process, most of the unigenes were annotated to the cellular process, metabolic process, and the single-organism process (Fig. 4A).
Figure 4: Functional annotation of all unigenes from G. lemaneiformis (A): Gene Ontology (GO) annotation based on the three major categories: cellular component, molecular function, and biological process. (B): The eukaryotic Orthologous Groups database (KOG) analysis of the annotated unigenes. (C): Kyoto Encyclopedia of Genes and Genomes (KEGG) pathways classification of the annotated unigenes
KOG analysis showed that a total of 11,759 unigenes were annotated (Fig. 4B). Among them most of the annotated genes (2,436, 19.46%) were involved in translation, ribosomal structure, and biogenesis. Afterwards, 1,452 (11.6%) genes were related to post-translational modification, protein turnover and chaperones.
Unigenes marked with the KEGG database were divided into 5 pathways (Fig. 4C). These were cellular processes, environmental information processing, genetic information processing and biological systems, metabolism, and organic systems. The number of abundant unigenes of each pathway was different. The most abundant unigenes were endocytosis-related genes (367) in the cellular process. The most abundant unigenes were phosphatidylinositol signaling-related genes (118) in environmental information processing. The largest number of unigenes was ribosome-related genes (2,107) in genetic information processing and biological systems. The most abundant unigenes were related to carbon metabolism (590), followed by genes related to biosynthesis of amino acids (466) in the metabolic process. The largest number of unigenes were associated with plant-pathogen interactions (94) in organic synthesis.
3.2.2 Differentially Expressed Genes (DEGs) Affected by Sulfate Deficiency in G. lemaneiformis
The great majority of unigenes in this study did not have high expression fold-change in the samples of sulfate sufficiency and deficiency being compared, therefore, two expression fold-change as a threshold value in defining DEGs was carried out. Totally, 2,238 differentially expressed unigenes were obtained, 139 up-regulated genes (red dots) and 2,099 down-regulated genes (green dots) were found in sulfate deficiency samples compared to that in sulfate sufficiency samples. Most of genes (33,853) did not have significant difference in expression (black dots) (Fig. 5A). Subsequently, all DEGs were subjected to GO term and KEGG pathway enrichment analysis. Totally, 875 DEGs were annotated in Go database. In the biological process, most of the DEGs were annotated to the cellular process, metabolic process, and the single-organism process. In the cellular component, most of the DEGs were involved in the cell, cell part and organelle. In the molecular function, DEGs were mostly related to catalytic activity, binding and structural molecular activity (Fig. 5B). Overall, 2,238 DEGs were classified into 20 KEGG pathways. Among the 20 KEGG pathways, the pathway related with ribosome (200, 8.9%) represented the largest group, with the high reliable in the enrichment significance of DEGs in the ribosome pathway (Fig. 5C).
Figure 5: Differentially expressed genes (DEGs) in G. lemaneiformis (A): Volcano plot of gene expression difference between control and sulfate deficiency samples. Each dot represents a gene. Log2 (FDR) is the logarithmic value of the mean expression in the two samples, which is used to show the difference in expression. (B): GO analysis of the DEGs. The abscissa is GO classification. The left side of the ordinate is the percentage of the number of genes, and the right side is the number of genes. (C): KEGG pathway functional enrichment analysis of the DEGs. Each circle in the graph represents a KEGG pathway, the longitudinal coordinate represents the name of the pathway, and the abscissa is the enrichment factor
3.3 Transcriptional Regulation of Sulfur Metabolism in G. lemaneiformis under the Sulfate Deficiency
3.3.1 Sulfur Acquisition and Assimilation
The genes encoding sulfate transporter/permease (ST) and ATP sulfurylase (ATPS) were identified in the transcriptome data of G. lemaneiformis, but they were not differentially expressed under the sulfate deficiency compared to that in sulfate sufficiency (Fig. 6). The expression of ST and ATPS genes was not affected, indicating that G. lemaneiformis used some other sulfate transporter/permease and/or ATP sulfurylase to transport or assimilate sulfate from surrounding environment under the condition of sulfate deficiency. The sulfur storage of most photosynthetic organisms in cells was very limited, a main response of photosynthetic organisms to sulfate deficiency is to increase the capacity of the cell for transporting and/or assimilating sulfate from surrounding environment [31,36]. Consistent with the results from physiology research, it was speculated that unlike other photosynthetic organisms G. lemaneiformis could use vacuolar sulfur to satisfy their nutritional requirements. Subsequently, adenosine 5’-phosphosulfate (APS) will be assimilated for the synthesis of sulfur-containing metabolites through the reductive or phosphorylated pathways. Genes encoding APS reductase (APR) and APS kinase (APK) are the key node in the reductive and phosphorylated pathways. Nevertheless, the two genes were not found in the transcriptome data of G. lemaneiformis under sulfate deficiency. Similar with G. lemaneiformis, APR gene was also not found in Gracilaria changii and Gracilaria salicornia under sulfate deprivation [5]. Therefore, it was speculated that some alternative reductase/phosphorylase or pathways exist to meet the demands of the reduction and phosphorylation of APS. In this study, a gene encoding sulfohydrolase/ sulfurylase (SH) was down regulated in sulfate deficiency G. lemaneiformis.
Figure 6: The pathway of sulfur metabolism in G. lemaneiformis
The pathway of sulfur metabolism was modified from previous papers [3,5]. Unigenes in red or green rectangles indicate that the expression of the unigenes in G. lemaneiformis under sulfate deficiency was up-regulated or down-regulated compared to those in sulfate sufficiency, respectively. Unigenes in blue rectangles indicate that they are only present in G. lemaneiformis under sulfate deficiency and sufficiency, but do not have up-and down-regulation. Whereas grey rectangles indicate these unigenes were not found in G. lemaneiformis under sulfate deficiency and sufficiency. APS, adenosine 5’-phosphosulfate; APK, APS kinase; APR, APS reductase; ATPS, ATP sulfurylase; BPNT, 3’(2’), 5’-bisphosphate nucleotidase; CBL, cystathionine beta-lyase; CGS, cystathionine gamma-lyase; GGCS, γ-glutamylcysteine synthetase; GSHS, glutathione synthetase; GST, glutathione-S-transferase; MS, methionine synthase; OAST-TL, O-acetylserine (thiol)-lyase; PAPS, 3’-phosphoadenosine 5’-phosphosulfate; SAH, S-adenosylhomocysteine; SAHH, SAH hydrolase; SAM, S-adenosylmethionine; SAMS, SAM synthetase; SAT, serine acetyltransferase; SH, sulfohydrolase/ sulfurylase; SiR, sulfite reductase; SO, sulfite oxidase; SOT, sulfotransferase; ST, sulfate transporter/permease.
Additionally, one gene encoding sulfite oxidase (SO) was down regulated in this experiment. Consistency result was also observed in sulfate-deficiency G. salicornia, but not in sulfate deficiency C. reinhardtii [5,12]. The diverse results might be due to different algal species. SO plays a role in the recycling of sulfite to form intracellular sulfate. This means that the regeneration of free sulfate was decreased, excess sulfite was used to synthesize cysteine in G. lemaneiformis. Together with SO gene, a gene encoding sulfite reductase (SiR) was also down regulated. Since most genes were down regulated during the pathway of sulfate reduction, therefore, it was suggested that the sulfate reduction pathway was inhibited in sulfate deficiency G. lemaneiformis.
3.3.2 Metabolism of Glutathione and Methionine
The metabolisms of glutathione and methionine are the extend of sulfate reductive pathways. Serine acetyltransferase (SAT), the only up-regulated gene in the pathway of sulfur metabolism in sulfate deficiency G. lemaneiformis, is responsible for the biosynthesis of O-acetylserine from serine (Fig. 6). The up regulation of SAT gene might be explained by that serine was continually synthesized from nitrogen metabolism. Hydrogen sulfide derived from the reduction of sulfate is inserted into the construction of O-acetylserine to form cysteine under the action of O-acetylserine(thiol)lyase (OAS-TL). Cysteine is the first stable organic sulfur compound during the pathway of sulfur metabolism. It was reported that the synthesis of cysteine is regulated by the assemblage and dis-assemblage of SAT and OAS-TL complex [37]. SAT is only active when it is associated in the complex, while OAS-TL is active when it is free [38]. However, the gene encoding OAS-TL was not found from the transcriptome data in this study. The content of cysteine showed that it was significantly increased under the sulfate deficiency compared to that in sulfate sufficiency on the 16th day (Fig. S1A). Consequently, it was speculated that an alternative gene exists in G. lemaneiformis function as OAS-TL, however, the expression of alternative gene was restrained.
It was known that genes encoding cystathionine gamma-lyase (CGS), cystathionine beta-lyase (CBL), methionine synthase (MS) and SAM synthetase (SAMS) are in-charge of the methionine synthesis. Additionally, genes encoding γ-glutamylcysteine synthetase (GGCS) and glutathione synthetase (GSHS) are responsible for the synthesis of glutathione [3,5]. Nevertheless, all these genes were down-regulated to different extents in G. lemaneiformis during sulfate deficiency. Our results indicated that the biosynthesis of methionine and glutathione was suppressed by sulfate deficiency in G. lemaneiformis. However, it was observed that the content of methionine during sulfate deficiency was not significantly reduced from that of sulfate sufficiency (Fig. S1B). Therefore, it was proposed that methionine biosynthesis in alga G. lemaneiformis was regulated by the combination of transcriptional and post-transcriptional mechanisms.
3.3.3 Other Identified Differentially Expressed Genes Involved in Sulfur Metabolism
Some unigenes involved in sulfur metabolism were also analyzed in G. lemaneiformis, the data of selected differentially expressed unigenes were shown in Table S2. It was found that genes encoding heparan-sulfate-6-O-sulfotransferase (HSS) and glycosyltransferase (GT) were down-regulated in sulfate-deficient G. lemaneiformis. Previous study showed that the down-regulation of HSS gene was consistent with the significant decrease of sulfate content in agar, and proposed that the different expression of GT gene might increase the gel strength of gel [5]. Therefore, the down regulated HSS and GT genes might change the quality of agar in G. lemaneiformis. Furthermore, some unigenes related with sulfate transport were also analyzed from the transcriptome of G. lemaneiformis during sulfate deficiency. The results showed that genes encoding S-type anion channel, ABC transporter related protein, ABC transporter permease and mitochondrial carrier protein/S adenosylmethionine carrier were all down-regulated in their sulfate deficient algal samples (Table S2). Therefore, it was concluded that the whole sulfur metabolism was suppressed in G. lemaneiformis under the sulfate deficiency.
3.4 Verification of DEGs with qRT-PCR
Nine genes were selected from G. lemaneiformis and were verified by qRT-PCR (Fig. 7). Three genes are related with sulfur metabolism, including C87474, C137071 and C133127. Three genes are involved in nitrogen metabolism, including C116395, C116845 and C129388. While the other three genes (C135653, C135893 and C137592) participate the process of photosynthesis. As a result, the expression profiles of nine unigenes in G. lemaneiformis, generated by RNA-Seq, corroborated with those obtained from qRT-PCR (Fig. 7). The highly consistency between the results obtained from RNA-Seq and qRT-PCR indicated that transcriptome data of G. lemaneiformis under the sulfate sufficiency and deficiency were credible.
Figure 7: Validation of DEGs via qRT-PCR
In this study, the relative growth rate of algae was significantly inhibited after 6 days under sulfate deficiency. However, pigments content and Fv/Fm value were not significantly decreased, indicating that the photosynthesis was not obviously affected under the sulfate deficiency. The soluble protein and carbohydrate contents were increased, suggesting that G. lemaneiformis cells did not use sulfur from internal protein and carbohydrate pools but utilize stored sulfur from vacuole to satisfy their nutritional requirements during the sulfate deficiency. Furthermore, it was concluded that most annotated differentially expressed genes (DEGs) were down regulated under the sulfate deficiency via transcriptome analysis, including large number of genes involved in the sulfur metabolism pathway. Therefore, it was speculated that the pathway of sulfur metabolism was inhibited in G. lemaneiformis under the sulfate deficiency.
Data Availability Statement: The data used to support the findings of this study are available from the corresponding author upon request. Sequence reads are available by the NCBI sequence read archives sulfate sufficiency (SRR12206572; SRR12213124; SRR12215316) and sulfate deficiency (SRR12223594; SRR12226568; SRR12235007).
Authors Contribution: HD and XL designed the experiments and supervised the project. HJ, MA, TL, CZ, XX, NH, HL, PP, PL and WC performed the experiments. XL analyzed the data and wrote the draft manuscript. All authors discussed the results and implications and commented on the manuscript at all stages.
Funding Statement: This work was supported by Key Special Project for Introduced Talents Team of Southern Marine Science and Engineering Guangdong Laboratory (Guangzhou) (Grant No. GML2019ZD0606), National Natural Science Foundation of China (Grant No. 41976125), Special Fund for Science and Technology of Guangdong Province (Grant No. STKJ2021193), Natural Science Foundation of Guangdong Province (Grant No. 2022A1515012141), Guangdong Basic and Applied Basic Research Foundation (Grant No. 2021A1515110001) and Innovation Project of Guangdong University (Grant No. 2018KCXTD012).
Conflicts of Interest: The authors declare that they have no conflicts of interest to report regarding the present study.
References
1. Bochenek, M., Koprivova, A., Mugford, S. T., Bell, T. G., Malin, G. et al. (2013). Transcriptome analysis of sulfate deficiency response in marine microalga Emiliania huxleyi. New Phytologist, 199, 650–662. [Google Scholar]
2. Giordano, M. (2013). Homeostasis: An underestimated focal point of ecology and evolution. Plant Science, 211, 92–101. [Google Scholar]
3. Takahashi, H., Kopriva, S., Giordano, M., Saito, K., Hell, R. (2011). Sulfur assimilation in photosynthetic organisms: Molecular functions and regulations of transporters and assimilatory enzymes. Annual Review of Plant Biology, 62, 157–184. [Google Scholar]
4. Garcia-Jimenez, P., Llorens, C., Roig, F. J., Robaina, R. R. (2018). Analysis of the transcriptome of the red seaweed Grateloupia imbricata with emphasis on reproductive potential. Marine Drugs, 16, 490. [Google Scholar]
5. Lee, W. K., Namasivayam, P., Abdullah, J. O., Ho, C. L. (2017). Transcriptome profiling of sulfate deprivation responses in two agarophytes Gracilaria changii and Gracilaria salicornia (Rhodophyta). Scientific Reports, 7, 1–14. [Google Scholar]
6. Ratti, S., Knoll, A. H., Giordano, M. (2011). Did sulfate availability facilitate the evolutionary expansion of chlorophyll a+c phytoplankton in the oceans? Geobiology, 9, 301–312. [Google Scholar]
7. Giordano, M. (2005). Sulfur and primary production in aquatic environments: An ecophysiological. Photosynthesis Research, 86(3), 86409–86417. [Google Scholar]
8. Carfagna, S., Salbitani, G., Vona, V., Esposito, S. (2011). Changes in cysteine and O-acetyl-l-serine levels in the microalga Chlorella sorokiniana in response to the S-nutritional status. Journal of Plant Physiology, 168, 2188–2195. [Google Scholar]
9. Carfagna, S., Bottone, C., Cataletto, P. R., Petriccione, M., Pinto, G. et al. (2018). Impact of sulfur starvation in autotrophic and heterotrophic cultures of the extremophilic microalga Galdieria phlegrea (Cyanidiophyceae). Plant & Cell Physiology, 57, 1890–1898. [Google Scholar]
10. Zalutskaya, Z., Filina, V., Ostroukhova, M., Ermilova, E. (2018). Regulation of alternative oxidase 1 in Chlamydomonas reinhardtii during sulfur starvation. European Journal of Protistology, 63, 26–33. [Google Scholar]
11. de Mia, M., Lemaire, S. D., Choquet, Y., Wollman, F. A. (2019). Nitric oxide remodels the photosynthetic apparatus upon S-starvation in Chlamydomonas reinhardtii. Plant Physiology, 179, 718–731. [Google Scholar]
12. González-Ballester, D., Casero, D., Cokus, S., Pellegrini, M., Merchant, S. S. et al. (2010). RNA-seq analysis of sulfur-deprived Chlamydomonas cells reveals aspects of acclimation critical for cell survival. Plant Cell, 22, 2058–2084. [Google Scholar]
13. Nguyen, A. V., Thomas-Hall, S. R., Malnoë, A., Timmins, M., Mussgnug, J. H. et al. (2008). Transcriptome for photobiological hydrogen production induced by sulfur deprivation in the green alga Chlamydomonas reinhardtii. Eukaryot Cell, 7, 1965–1979. [Google Scholar]
14. Zhang, Z., Pendse, N. D., Phillips, K. N., Cotner, J. B., Khodursky, A. (2008). Gene expression patterns of sulfur starvation in Synechocystis sp. PCC 6803. BMC Genomics, 344, 1–14. [Google Scholar]
15. Maruyama-Nakashita, A., Inoue, E., Watanabe-Takahashi, A., Yamaya, T., Takahashi, H. (2003). Transcriptome profiling of sulfur-responsive genes in Arabidopsis reveals global effects of sulfur nutrition on multiple metabolic pathways. Plant Physiology, 132, 597–605. [Google Scholar]
16. Lv, H., Qiao, C., Zhong, C., Jia, S. (2018). Metabolic fingerprinting of Dunaliella salina cultured under sulfur deprivation conditions. Journal of Applied Phycology, 30, 355–365. [Google Scholar]
17. Lv, H., Cui, X., Wahid, F., Xia, F., Zhong, C. et al. (2016). Analysis of the physiological and molecular responses of Dunaliella salina to macronutrient deprivation. PLoS One, 11, e0152226. [Google Scholar]
18. Cao, H., Zhang, L., Melis, A. (2001). Bioenergetic and metabolic processes for the survival of sulfur-deprived Dunaliella salina (Chlorophyta). Journal of Applied Phycology, 13, 25–34. [Google Scholar]
19. Liu, X., Zhang, Q., Huan, Z., Zhong, M., Chen, W. et al. (2018). Identification and characterization of glutamine synthetase isozymes in Gracilaria lemaneiformis. Aquatic Botony, 146, 23–30. [Google Scholar]
20. Du, H., Liang, H., Jiang, Y., Qu, X., Yan, H. et al. (2018). Proteome responses of Gracilaria lemaneiformis exposed to lead stress. Marine Pollution Bulletin, 135, 311–317. [Google Scholar]
21. Bixler, H. J., Porse, H. (2011). A decade of change in the seaweed hydrocolloids industry. Journal of Applied Phycology, 23, 321–335. [Google Scholar]
22. Xu, S. Y., Huang, X., Cheong, K. L. (2017). Recent advances in marine algae polysaccharides: Isolation, structure, and activities. Marine Drugs, 15, 388. [Google Scholar]
23. Wang, Y., Feng, Y., Liu, X., Zhong, M., Chen, W. et al. (2018). Response of Gracilaria lemaneiformis to nitrogen deprivation. Algal Reserch, 34, 82–96. [Google Scholar]
24. Liu, X., Wen, J., Zheng, C., Jia, H., Chen, W. et al. (2019). The impact of nitrogen deficiency and subsequent recovery on the photosynthetic performance of the red macroalga Gracilariopsis lemaneiformis. Journal of Applied Phycology, 31, 2699–2707. [Google Scholar]
25. Liu, X., Wen, J., Chen, W., Du, H. (2019). Physiological effects of nitrogen deficiency and recovery on the macroalga Gracilaria lemaneiformis (Rhodophyta). Journal of Phycology, 55, 830–839. [Google Scholar]
26. Wang, Y., Feng, Y., Wang, H., Zhong, M., Chen, W. et al. (2016). Physiological and proteomic analyses of two Gracilaria lemaneiformis strains in response to high-temperature stress. Journal of Applied Phycology, 28, 1847–1858. [Google Scholar]
27. Pfaffl, M. W. (2001). A new mathematical model for relative quantification in real-time RT-PCR. Nucleic Acids Reserch, 29, 202–207. [Google Scholar]
28. Saroussi, S., Sanz-Luque, E., Kim, R. G., Grossman, A. R. (2017). Nutrient scavenging and energy management: Acclimation responses in nitrogen and sulfur deprived Chlamydomonas. Current Opinion in Plant Biologu, 39, 114–122. [Google Scholar]
29. Giordano, M., Pezzoni, V., Hell, R. (2000). Strategies for the allocation of resources under sulfur limitation in the green alga Dunaliella salina. Plant Physiol, 124, 857–864. [Google Scholar]
30. Huang, X., Zang, X., Wu, F. Y., Jin, H., Wang, C. et al. (2017). Transcriptome sequencing of Gracilariopsis lemaneiformis to analyze the genes related to optically active phycoerythrin synthesis. PLoS One, 12, 1–17. [Google Scholar]
31. Giordano, M., Norici, A., Hell, R. (2005). Sulfur and phytoplankton: Acquisition, metabolism and impact on the environment. New Phytologist, 166, 371–382. [Google Scholar]
32. Wykoff, D. D., Davies, J. P., Melis, A., Grossman, A. R. (1998). The regulation of photosynthetic electron transport during nutrient deprivation in Chlamydomonas reinhardtii. Plant Physiology, 117, 129–139. [Google Scholar]
33. Ravina, C. G., Barroso, C., Vega, J. M., Gotor, C. (1999). Cysteine biosynthesis in Chlamydomonas reinhardtii. Molecular cloning and regulation of O-acetylserine(thiol)lyase. European Journal of Biochemistry, 264, 848–853. [Google Scholar]
34. Carfagna, S., Vona, V., di Martino, V., Esposito, S., Rigano, C. (2011). Nitrogen assimilation and cysteine biosynthesis in barley: Evidence for root sulphur assimilation upon recovery from N deprivation. Environmental and Experimental Botany, 71, 18–24. [Google Scholar]
35. Sciences, M. (2018). Transcriptome analysis in Haematococcus pluvialis: Astaxanthin induction by high light with acetate and Fe2+. International Journal of Molecular Sciences, 19, 1–18. [Google Scholar]
36. Zhang, Z., Shrager, J., Jain, M., Chang, C. W., Vallon, O. et al. (2004). Insights into the survival of Chlamydomonas reinhardtii during sulfur starvation based on microarray analysis of gene expression. Eukaryotic Cell, 3, 1331–1348. [Google Scholar]
37. Giordano, M., Norici, A., Ratti, S., Raven, J. A. (2008). Role of sulfur for algae: Acquisition, metabolism, ecology and evolution. In: Sulfur metabolism in phototrophic organisms, pp. 397–415. [Google Scholar]
38. Hell, R., Wirtz, M. (2011). Molecular biology, biochemistry and cellular physiology of cysteine metabolism in Arabidopsis thaliana. Arabidopsis Book, 9, e0154. [Google Scholar]
Appendix
Figure S1: Contents of cysteine and methionine amino acids. (A) The content of methionine. (B) The content of cysteine
Cite This Article
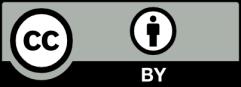
This work is licensed under a Creative Commons Attribution 4.0 International License , which permits unrestricted use, distribution, and reproduction in any medium, provided the original work is properly cited.