Open Access
ARTICLE
Extraction, Antioxidant Activity and Identification of Flavonoids from Root Tubers of Kosteletzkya virginica
School of Life Sciences and Chemical Engineering, Jiangsu Second Normal University, Nanjing, 210013, China
* Corresponding Author: Yu Zai. Email:
(This article belongs to the Special Issue: Plant Bioactive Compounds-Chemotaxonomic Significance, Antioxidant Properties, Potential Application as Food Ingredients as well as Therapeutics, and the Analytical Technology in Their Efficient Discovery)
Phyton-International Journal of Experimental Botany 2023, 92(1), 225-236. https://doi.org/10.32604/phyton.2022.022576
Received 16 March 2022; Accepted 17 May 2022; Issue published 06 September 2022
Abstract
Kosteletzkya virginica (K. virginica) is used for revegetation of salt-affected coastal tidal flats and as a raw material of biodiesel. K. virginica root tuber, a biowaste with low economic value, is rich in bioactive compounds. This study aimed to extract and identify flavonoids from K. virginica root tubers. The optimal extraction conditions were 1/25 (w/v) solid/liquid ratio, 40% ethanol concentration at 40°C for 60 min. Under these conditions, 65.2 ± 3.7 mg/g total flavonoid content was extracted from the roots, which were collected from salinized soil in late autumn of the third year. Antioxidant activity was evaluated through 1,1-diphenyl-2-picrylhydrazyl, hydroxyl radical, and superoxide anion scavenging assays. The extracted flavonoids exhibited antioxidant activity in a dose-dependent manner. Five flavonoids, glucoliquiritin apioside, licoisoflavone B, 5-methoxy-7,8-diprenyl- flavone, 7,2′-dihydroxy-6,8-dimethyl-4′,5′-methylenedioxyflavan, and 5,7,4′-trihydroxy-3′-methoxy-6,8-di-Cmethylflavanone, were identified by ultra-performance liquid chromatography–tandem mass spectrometry. Our results suggest that the flavonoids of K. virginica root tubers might be potent antioxidants and can be effectively applied as an ingredient in food and natural medicine.Keywords
Kosteletzkya virginica is a perennial halophytic species of the Malvaceae family native to the mid-Atlantic coasts and southeastern parts of the United States, and has higher adaptability in terms of oxidative status than glycophytes [1,2]. Seaside mallow is introduced to coastal regions of China as a potential species to improve saline soils and develop saline agriculture, because of its high tolerance to harsh conditions, such as salinity and waterlogging [3,4]. Seashore mallow has been utilized because it has high contents of fatty acid and bioactive compounds such as polysaccharides, saponins and flavonoids [5].
Flavonoids have attracted great attention due to the antioxidant, anticancer, antibiosis and anti-inflammatory properties [6–8]. Therefore, a simple, fast, and efficient method should be developed for the extraction of flavonoids from K. virginica. Traditional refluxing is time consuming and need a large amount of solvent, which can cause the loss of flavonoids due to ionization, hydrolysis, and oxidation. Methods, such as conventional Soxhlet extraction, ultrasonic-assisted extraction (UAE), microwave-assisted extraction, and supercritical fluid extraction, have been developed [9,10]. Acoustic cavitation can disrupt the cell wall, reduce particle size, and accelerate the solvent to enter the cell membrane [11]. The yield of flavonoid extract from Folium eucommiae reached 17.2% through UAE under 1:60 solid-to-liquid ratio (40% ethanol) for 70 min. Increasing the temperature could reduce the time for the extraction of Prunella vulgaris L. [12]. In this study, UAE was conducted to extract flavonoids because of high efficiency, short extraction time, fine extraction homogeneity, and simple operation. The extraction parameters should be evaluated to better utilized root tuber extracts and minimize cost.
Flavonoids are a class of secondary metabolism associated with plant protection. Environmental factors, such as temperature and soil, could induce plants to produce considerable flavonoids [13]. In this study, we optimized the extraction of flavonoids from K. virginica root tuber, investigated the possible correlation between the flavonoids and free radical scavenging capacity, and evaluated K. virginica root tuber as a cheap source of bioactive flavonoids by determining their constituents.
K. virginica root tubers were collected from the planting base of Jinhai Agricultural Experimental Farm in Jiangsu Province, China (33°26′44.8″N, 118°10′06.5″E). The dried roots were milled into powder using a pulverizer (Weibochuang Machinery Equipment Co., Ltd., Beijing, China) and passed through a 100-mesh sieve. Analytic grade rutin standards, aluminum nitrate, ferric trichloride, potassium ferricyanide, pyrogallol, ascorbic acid, trichloroacetic acid, salicylic acid, and ethanol were purchased from Jiuyi Chemical Reagent Co., Ltd. (Shanghai, China). 1,1-diphenyl-2-picrylhydrazyl (DPPH) was purchased from Aladdin Reagent Co., Ltd. (Shanghai, China). HPLC grade methanol and formic acid were obtained from J&K Scientific Ltd. (Shanghai, China).
A BILON-1000D ultrasonic (Woxin Instrument Co., Ltd., Wuxi, China) was used to extract the flavonoids. A TU-1800 spectrophotometer (Shimadzu Corporation, Japan) was used for the analysis of total flavonoid content, and an LTJ-12 labconco (Beijing Songyuan Huaxing Technology Co., Beijing, China) was used for the concentration of samples. Ultra-performance liquid chromatography–tandem mass spectrometry (UPLC-MS/MS) (Thermo, Ultimate 3000LC, Orbitrap Elite) was used for the identification of total flavonoids.
2.2 Ultrasound-Assisted Extraction of Total Flavonoids
Each extraction variable was determined through one-factor-at-a-time method. K. virginica root powder (5 g) was soaked with ethanol (varying ethanol concentration from 30% to 80%, v/v; varying solid/liquid ratio from 1/10 to 1/35, w/v), placed in ultrasonic bath, and sonicated at 28 kHz and 200 W for a certain time (varying extraction time from 20 to 80 min) at 30°C to 80°C. The solid was extracted with fresh solvent and the number of extraction was optimized (one to four times). The extract was filtered through a 0.45 μm organic microporous membrane. Then, the filtrate was combined and dried to determine the total flavonoid content. Extractions were conducted in triplicate. A four-variable, three-level design L9 (34) orthogonal experiment based on the result of single-factor test was conducted (Table 1) to determine the maximum extraction rate of total flavonoid content.
2.3 Determination of Total Flavonoid Content
Total flavonoid content was determined using a colorimetric method. Briefly, different volumes of rutin standard solution (0.11 mg/mL) were transferred to 10 mL volumetric flasks. Then, 0.3 mL of 5% sodium nitrite solution was added to each volumetric flask. After mixing for 5 min, 0.3 mL of 10% aluminum nitrate solution was added, and 4.0 mL of 4% NaOH solution was added after 5 min. Each flask was diluted to the mark with 50% methanol and mixed well. A working calibration plot was obtained based on the absorbance data at 510 nm.
Total flavonoid content was determined using rutin as calibration standard at the range of 0.11–0.55 mg/mL. The calibration curve was y = 2.5455x + 0.038 (R2 = 0.9992), where y = absorbance, and x = sample concentration.
The concentration of total flavonoids was calculated based on the standard curve, and extraction efficiency was calculated by using the following equation:
Extraction rate of total flavonoid content (%) = C × Solution volume/Sample mass × 100, where C is the concentration of the solution.
2.4 Effects of Growth Age and Soil Property on Total Flavonoid Content
K. virginica seeds were sowed at Jinhai Agricultural Experimental Farm (32°59′–33°03′ N, 120°46′–120°52′ E) in Jiangsu Province, China, on 16 April, 2012. Samples were collected from two different sampling sites (site 1 and site 2) on October 2012, April 2013, and October 2014. Prior to the planting stage of the experiment, the soil from site 1 had the following properties: pH, 8.22; electrical conductivity (EC), 1.32 mS/cm; hydrolysable N, 41.34 mg/kg; total K, 28.9 g/kg; available P, 15.77 mg/kg; organic matter, 13.42 g/kg. The soil from site 2 was an artificially improved non-salinized soil in which its properties were the same as site 1 except with lower EC (0.2 mS/cm). At each sampling site, three sampling points were collected the root, and 10 tubers were randomly picked at each sampling point. The dynamic change of total flavonoid content of fresh plant material was analyzed using the above method.
2.5 Determination of Antioxidant Activity of Flavonoids DPPH Free Radical
DPPH free radical scavenging activities were evaluated as described previously [14]. Briefly, DPPH solution (2.5 mL, 2 × 10−4 mol/L) was added to 1.0 mL of K. virginica root extract (0.0252, 0.0504, 0.0756, 0.1008, and 0.1260 mg/mL), and completely mixed for 30 min, and absorbance Ai was recorded at 517 nm. Anhydrous ethanol was used to measure Ab at 517 nm. The absorbance of the reaction of 2.5 mL DPPH solution with 2 × 10−4 mol/L concentration and 1.0 mL anhydrous ethanol as reference was marked as Ac. The clearance rate was calculated as:
Ai is the absorbance value of the DPPH mixture and sample;
Ab is the absorbance value of the mixture of anhydrous ethanol and sample;
Ac is the absorbance value of the DPPH mixture and anhydrous ethanol.
A model reaction system was determined using Fenton reaction with some modifications. K. virginica root extract (0.0252, 0.0504, 0.0756, 0.1008, and 0.1260 mg/mL concentrations, 1 mL) was added to five sample tubes, which included 2.0 mL FeSO4 (9 mmol/L) and 2.0 mL salicylic acid ethanol (9 mmol/L). H2O2 (2.0 mL) (8.8 mmol/L) was added. Each test was in three replicates. The distilled water was the blank solution. All tubes were incubated on a water bath at 37°C for 30 min, and absorbance was measured at 510 nm. The clearance rate was calculated as:
where A0 is the absorbance of the blank reference solution, Ax is the absorbance of the extract of K. virginica root, and Ax0 is the absorbance of the sample without H2O2.
O2−⋅ scavenging activity was evaluated as described [15]. Tris-HCl solution (4.5 mL, 50 mmol/L, pH 8.2) and 4.2 mL ultrapure water were added to a 10 mL colorimetric tube. The tube was shaken and allowed to equilibrate at 25°C for 20 min. Pyrogallol (0.3 mL, 3 mmol/L) was added (10 mmol/L HCl was used rather than pyrogallol as the blank solution), the tube was rapidly shaken, and absorbance was measured at 325 nm every 30 s up to 5 min. The rate of change (F0) of absorbance with time was calculated. Tris-HCl solution (4.5 mL) (50 mmol/L, pH 8.2) and 3.2 mL ultrapure water were mixed with 1 mL K. virginica root extracts with different concentrations (0.0252, 0.0504, 0.0756, 0.1008, and 0.1260 mg/mL). The tubes were placed in a water bath at 25°C for 20 min. Pyrogallol (0.3 mL, 3 mmol/L) was added to each tube, the mixture was vigorously shaken, and absorbance at 325 nm was measured every 30 s up to 5 min. The rate of absorbance change (Fx) with time of extract sample was determined. The change rate (F0) of pyrogallol autoxidation was analyzed using ultrapure water substitution on the sample in the mixture. All measurements were performed in triplicate. Pyrogallol clearance rate was calculated as:
Flavonoid extracts were dissolved in methanol, and fenclonine was added as internal standard substance. Chromatographic separations were accomplished on a Waters ACQUITY UPLC HSS T3 column (2.1 mm × 100 mm, 1.8 μm). Eluent A was water + 0.1% formic acid, and eluent B was acetonitrile + 0.1% formic acid. The column temperature was maintained at 40°C, the flow rate was 0.3 mL/min, the volume of injected sample was 4 μL, and automatic injector temperature was 4°C. Mobile phase was used with a gradient elution condition following Table 2. The mass spectra were recorded in positive and negative modes in a mass range at m/z 50–1000. The desolvation temperature was set to 350°C, and capillary voltage was 3.2 kV.
All statistical analyses were performed using SPSS 18.0 software. Measurement data were expressed as mean ± standard deviation of three replicates and analyzed by using the Student’s T-test.
3.1 Range Determination for Total Flavonoid Extraction Variables
The range of each extraction variable was evaluated through single-factor tests. The preliminary ranges of extraction variables during optimization were determined (Fig. 1).
Figure 1: The effects of different parameters on the extraction efficiency of total flavonoids in the root tuber of K. virginica. a. solid/solvent ratio; b. ethanol concentration; c. time; d. temperature; e. times of extraction
The extraction rate of total flavonoid content in K. virginica root tubers gradually increased with the decrease of solid/liquid ratio in the extraction medium. The extraction efficiency of flavonoids was 53.7% (Fig. 1a) when the solid/liquid ratio was 1/20. Therebefore, 1/20–1/30 (w/v) was selected for optimization.
The extraction rate of total flavonoid content gradually increased with the increase of ethanol and reached the peak at 50% (Fig. 1b). Approximately 50% ethanol is suitable for the extraction of flavonoid glycosides. The dissolvability of flavonoids decreased with the increase of ethanol concentration. Therebefore, ethanol content range of 40%–60% was selected for the optimization in the orthogonal test.
The extraction rate of flavonoids markedly increased with the increase of ultrasonic extraction time from 20 to 50 min. The result suggested that the changes of extraction time had a significant effect on the extraction rate of flavonoids. The highest extraction rate of total flavonoid content was 53.2% when the extraction time was 50 min (Fig. 1c). The extraction rate showed a limited rising trend, which only increased 0.75% within 30 min when the extraction time was more than 50 min. Extraction time between 40–60 min was selected for subsequent optimization.
The extraction rate was achieved at an optimum point (50°C) and gradually decreased when the temperature exceeded the point. Therefore, the temperature range between 30°C–50°C was used in the orthogonal test.
Under the conditions of the above mentioned best single factors, the raw material was repeatedly leached to compare the flavonoid yields. As shown in Fig. 1e, any increase in the extraction rate for leaching of more than two times was marginal, and subsequent extractions only wasted the extraction solvent. Therefore, this factor was not considered in the orthogonal experiment.
3.2 Analysis of the Orthogonal Test
The extraction parameters of flavonoids from K. virginica root tubers were optimized, and the order of parameters was ascertained. The results showed that these independent variables significantly affected the extraction yield of total flavonoids. As shown in Table 3, four factors based on orthogonal experiments were sorted in terms of their effects in descending order: extraction temperature > extraction time > ethanol concentration > solid/liquid ratio. The extraction temperature was proved as a distinct parameter. Few interactions between the extraction parameters contributed to the high recovery of total flavonoids. The optimal combination of the range analysis was A2B3C1D2, namely, optimal UAE conditions for K. virginica root tuber extracts were 40% ethanol concentration at 40°C with 60 min and solid/liquid ratio of 1/25 (w/v).
3.3 The Effects of Growth Age, Season, and Soil Property on Total Flavonoid Contents
K. virginica seeds were sowed at Jinhai Agricultural Experimental Farm, where the annual mean temperature is 14.7°C, and the monthly mean temperature in January and July is 0.8 and 26.8°C, respectively. The annual mean rainfall ranges are from 900 to 1060 mm. The area has a non-frost period of 230 days with a total sunshine radiation of 2241–2496 h annually. The K. virginica fruit ripens during early August and early mid-September, and the aboveground parts slowly die, indicating that the growth period is approximately 160 days.
The total flavonoid content under different sampling time for three years is shown in Table 4. The flavonoid content increases during the wintering period, which protects plant organisms from low-temperature stresses. The hydroxyl of flavonoids can remove free radicals accumulated under low-temperature stress through single electron transfer because it possesses strong electron supply capacity.
The total flavonoid content in the triennial root tuber of K. virginica was significantly higher than that in the annual (P < 0.05), revealing an increase of 24% in the flavonoid contents at site 1 and an increase of 30.8% at site 2, respectively. The total flavonoid contents may be related with growth ages.
The total flavonoid contents in K. virginica root tubers harvested from salinized soil (site 1) were significantly higher than that from nonsalinized soil (site 2) (P < 0.05), whether the tubers were collected annually or triennially or whether in Spring or Autumn (Table 4). The total flavonoid content was higher than under nonsalinized stress. Considering that no significant difference was observed between autumn and next spring, the largest flavonoid production in the tubers was obtained in late autumn of the third year under salinized stress, which were collected as the materials of antioxidant experiments.
3.4 Scavenging Effects of Total Flavonoid Extracts on DPPH, ⋅OH, and O2−⋅
To understand the antioxidant mechanism of flavonoids, K. virginica root tuber extracts were evaluated for radical scavenging activities against ⋅OH, O2−⋅, and DPPH. The extract quenched DPPH free radical in positive correlation and dose-dependent manner, as shown in Table 5. ⋅OH clearance rate gradually increased with the increase of extract concentration. ⋅OH clearance rate was 48.35 ± 0.97% compared to 45.64 ± 1.23% of ascorbic acid at 126 g/mL. Significant scavenging capacity of flavonoids of K. virginica demonstrated that they might reduce the concentration of catalyzing transition metal in lipid peroxidation. K. virginica root tuber extract scavenged O2−⋅ in a concentration-dependent manner. The scavenging (23.11 ± 0.58%–76.20 ± 3.01%) of superoxide radicals was evident at the tested concentrations (0.0252–0.126 μg/mL). Based on a series of concentrations shown in Table 5, we calculated EC50 value of K. virginica root tuber extract as 78 μg/mL, which was 1.25 times lower than that of ascorbic acid (97.5 μg/mL).
3.5 Identification of Flavonoids by UPLC-MS/MS
Five flavonoids were identified in terms of their retention times, mass spectral data, molecular formulas, and MS fragmentation patterns (Fig. 2). The 7,2′-dihydroxy-6,8-dimethyl-4′,5′-methylenedioxyflavan had the highest content among the extracts, and the remaining four, namely, glucoliquiritin apioside, licoisoflavone B, 5-methoxy-7,8-diprenylflavone, and 5,7,4′-trihydroxy-3′-methoxy-6,8-di-C-methylflavanone exhibited relatively smaller contents.
Figure 2: The total ion chromatograms and the mass spectrum of the flavonoids in the root tuber of K. virginica. a. total ion chromatogram of sample (ESI+); b. total ion chromatogram of sample (ESI–); c. mass spectrum of Licoisoflavone B; d. mass spectrum of glucoliquiritin apioside; e. mass spectrum of 5-methoxy-7,8-diprenylflavone; f. mass spectrum of 7,2′-dihydroxy-6,8-dimethyl-4′,5′-methylenedioxyflavan; g. mass spectrum of 5,7,4′-trihydroxy-3′-methoxy-6,8-di-C-methylflavanone
In the present study, extraction of flavonoids from K. virginica root tuber through UAE was optimized as follows: Solid/liquid ratio, 1/25; ethanol concentration, 40%; extraction temperature, 40°C; extraction time, 60 min. Under these conditions, we verified that K. virginica root tuber planted in saline soil for three years contained considerable flavonoids.
Previous experiments showed that the decomposition of flavonoids may occur with prolonged extraction time [16]. Long leaching time consume considerable energy and incur high costs. Therefore, we selected extraction time between 40–60 min for optimization.
Temperature is an important parameter of total flavonoid extraction [9]. The temperature accelerated molecular movement between 30°C–50°C, which was beneficial for flavonoids to release from plant cells. Meanwhile, high temperature caused denaturation of thermo-sensitive flavonoids in the sample and accelerated solution evaporation. Therefore, the temperature range between 30°C–50°C was used in the orthogonal test.
Sheng et al. reported that the yield of total flavonoids from Flos Populi was 53.08 ± 0.43 mg/g, which lasted for 2.27 h under 94.66°C [9]. In comparison, in this study UAE shortened the extraction time, lowered the temperature, and improved the extraction rate.
Variation in environmental resources (e.g., location and season) could represent a significant factor on the secondary metabolism in plant materials [17]. In this study, we found that flavonoid content increases during the wintering period, which protects plant organisms from low-temperature stress. The hydroxyl of flavonoids can remove or control free radicals generated and accumulated under low-temperature stress through single electron transfer.
In addition, we found that total flavonoid content was higher than under nonsalinized stress when we compared site 1 and site 2 with salinized soil or not. Concentration of flavonoids in the roots reduces oxidative damage caused by salt stress. This condition may be due to the reduction of malondialdehyde content and H2O2 level and the improvement of salt tolerance of the plants [18].
Flavonoids in plants are recognized as powerful antioxidants due to their ability to donate hydrogen or electrons and form stable radical intermediates [19]. In this study, K. virginica root tuber extracts showed excellent antioxidant activity in the DPPH assay, and EC50 occurred at 71.5 μg/ml extract, lower than sarang semut extract [20]. Other studies have shown that flavonoids could form slightly reactive flavonoid phenoxyl radicals and chelate transition metal ions, such as Fe2+ ion, to retain free radical scavenging capacity [21]. Significant scavenging capacity of flavonoids of K. virginica demonstrated that they might reduce the concentration of catalyzing transition metal in lipid peroxidation. Meanwhile, as good electron donors, flavonoid extracts may suppress the superoxide-driven Fenton reaction and accelerate the conversion of H2O2 into H2O3 [22]. Superoxide radical is normally formed in cellular oxidation reactions and could produce other types of cell-damaging free radicals and oxidizing agents. However, some flavonoids scavenge O2−· radicals to achieve effective antioxidant properties [23].
Previous studies showed that the flavonoids of K. virginica root tuber had potential immune and antitumor activities [4,8]. The results suggest that K. virginica is an invaluable natural source of bioactive components with high antioxidant capacities that can be used in food and pharmaceutical industries. By UPLC-MS/MS analysis we identified flavonoids in K. virginica root tuber. Further studies are needed for quantitative analysis of flavonoids that contribute to antioxidant activity of K. virginica root tuber.
In conclusion, we optimized the extraction of flavonoids from K. virginica root tuber and investigated the correlation between the flavonoids and free radical scavenging capacity. The flavonoids of K. virginica root tubers might be potent antioxidants and can be effectively applied as an ingredient in food and natural medicine.
Authorship: Study conception and design: Yu Zai; data collection and analysis: Yu Zai and Shaohua Liu; draft manuscript preparation: Yu Zai. All authors reviewed the results and approved the final version of the manuscript.
Funding Statement: This study was supported by the General Project of Natural Science Research in Jiangsu Province (22KJB180011), National Natural Science Foundation of China (82101587).
Conflicts of Interest: The authors declared that they have no conflicts of interest to report regarding the present study.
References
1. Gallagher, J. L. (1985). Halophytic crops for cultivation at seawater salinity. Plant and Soil, 89, 323–336. DOI 10.1007/BF02182251. [Google Scholar] [CrossRef]
2. Ellouzi, H., Hamed, K. B., Cela, J., Munné-Bosch, S., Abdelly, C. (2011). Early effects of salt stress on the physiological and oxidative status of Cakile maritima (halophyte) and Arabidopsis thaliana (glycophyte). Physiologia Plantarum, 142(2), 128–143. DOI 10.1111/j.1399-3054.2011.01450.x. [Google Scholar] [CrossRef]
3. Ruan, C. J., Li, H., Guo, Y. Q., Qin, P., Gallagher, J. L. et al. (2008). Kosteletzkya virginica, an agroecoengineering halophytic species for alternative agricultural production in China’s east coast: Ecological adaptation and benefits, seed yield, oil content, fatty acid and biodiesel properties. Ecological Engineering, 32, 320–328. DOI 10.1016/j.ecoleng.2014.10.021. [Google Scholar] [CrossRef]
4. Qin, P., Han, R. M., Zhou, M. X., Zhang, H. S., Fan, L. S. et al. (2015). Ecological engineering through the biosecure introduction of Kosteletzkya virginica (seashore mallow) to saline lands in China: A review of 20 years of activity. Ecological Engineering, 74, 174–186. [Google Scholar]
5. Moser, B. R., Seliskar, D. M., Gallagher, J. L. (2016). Fatty acid composition of fourteen seashore mallow (Kosteletzkya pentacarpos) seed oil accessions collected from the atlantic and gulf coasts of the United States. Industrial Crops and Products, 87, 20–26. DOI 10.1016/j.indcrop.2016.04.018. [Google Scholar] [CrossRef]
6. Huang, H., Miao, H., Wang, J., Wu, D., Lei, Q. et al. (2021). Advances on anticancer new drugs in China and the USA in 2020: From ongoing trial to drug approval. Journal of the National Cancer Center, 1(4), 147–152. DOI 10.1016/j.jncc.2021.08.002. [Google Scholar] [CrossRef]
7. Liu, Y., Wang, J., Xiao, Y., Shi, X., Zeng, Y. (2021). Diversity analysis of chlorophyll, flavonoid, anthocyanin, and nitrogen balance index of tea based on dualex. Phyton-International Journal of Experimental Botany, 90(5), 1549–1558. DOI 10.32604/phyton.2021.015557. [Google Scholar] [CrossRef]
8. Perez-Vizcaino, F., Fraga, C. G. (2018). Research trends in flavonoids and health. Archives of Biochemistry and Biophysics, 646, 107–112. DOI 10.1016/j.abb.2018.03.022. [Google Scholar] [CrossRef]
9. Sheng, Z. L., Wan, P. F., Dong, C. L., Li, Y. H. (2013). Optimization of total flavonoids content extracted from Flos Populi using response surface methodology. Industrial Crops and Products, 43(1), 778–786. DOI 10.1016/j.indcrop.2012.08.020. [Google Scholar] [CrossRef]
10. Liza, M. S., Rahman, R. A., Mandana, B., Jinap, S., Rahmat, A. et al. (2010). Supercritical carbon dioxide extraction of bioactive flavonoid from Strobilanthes crispus (Pecah Kaca). Food and Bioproducts Processing, 88(2–3), 319–326. DOI 10.1016/j.fbp.2009.02.001. [Google Scholar] [CrossRef]
11. Oniszczuk, A., Podgórski, R. (2015). Influence of different extraction methods on the quantification of selected flavonoids and phenolic acids from Tilia cordata inflorescence. Industrial Crops and Products, 76, 509–514. DOI 10.1016/j.indcrop.2015.07.003. [Google Scholar] [CrossRef]
12. Zhang, G., He, L., Hu, M. (2011). Optimized ultrasonic-assisted extraction of flavonoids from Prunella vulgaris L. and evaluation of antioxidant activities in vitro. Innovative Food Science & Emerging Technologies, 12(1), 18–25. DOI 10.1016/j.ifset.2010.12.003. [Google Scholar] [CrossRef]
13. Zidorn, C. (2016). Secondary metabolites of seagrasses (Alismatales and Potamogetonales; AlismatidaeChemical diversity, bioactivity, and ecological function. Phytochemistry, 124, 5–28. DOI 10.1016/j.phytochem.2016.02.004. [Google Scholar] [CrossRef]
14. Zhu, Y. J., Tan, J. (2008). Antioxidant effect of corn peptide. Journal of the Chinese Cereals and Oils Association, 23(1), 36–43. [Google Scholar]
15. Mykhailenko, O., Bezruk, I., Volochai, V., Mishchenko, V., Ivanauskas, L. et al. (2022). Phytochemical analysis and antioxidant activity of Crocus speciosus leaves. Phyton-International Journal of Experimental Botany, 91(1), 207–221. DOI 10.32604/phyton.2022.016458. [Google Scholar] [CrossRef]
16. Wen, H., An, X., Hai, N., Jia, Z., Wang, J. (2009). Optimised ultrasonic-assisted extraction of flavonoids from Folium eucommiae and evaluation of antioxidant activity in multi-test systems in vitro. Food Chemistry, 114(3), 1147–1154. DOI 10.1016/j.foodchem.2008.10.079. [Google Scholar] [CrossRef]
17. Bouslimi, H., Ferreira, R., Dridi, N., Brito, P., Martins-Dias, S. et al. (2021). Effects of barium stress in Brassica juncea and Cakile maritima: The indicator role of some antioxidant enzymes and secondary metabolites. Phyton-International Journal of Experimental Botany, 90(1), 145–158. DOI 10.32604/phyton.2020.011752. [Google Scholar] [CrossRef]
18. Li, S., Sun, L., Bai, L., Wang, W., Wan, J. et al. (2017). Flavonoid is associated with salt stress tolerance in Atriplex centralasiatica seedlings. Chinese Journal of Eco-Agriculture, 25(9), 1345–1350. [Google Scholar]
19. Scalbert, A., Manach, C., Morand, C., Rémésy, C., Jiménez, L. (2005). Dietary polyphenols and the prevention of diseases. Critical Reviews in Food Science and Nutrition, 45(4), 287–306. DOI 10.1080/1040869059096. [Google Scholar] [CrossRef]
20. Engida, A. M., Kasim, N. S., Tsigie, Y. A., Ismadjib, S., Huynh, L. H. et al. (2013). Extraction, identification and quantitative HPLC analysis of flavonoids from sarang semut (Myrmecodia pendan). Industrial Crops and Products, 41(1), 392–396. DOI 10.1016/j.indcrop.2012.04.043. [Google Scholar] [CrossRef]
21. Rice-Evans, C. A., Miller, N. J., Bolwell, G. P., Bramley, P. M., Pridham, J. B. (1995). The relative antioxidants activities of plant-derived polyphenolic flavonoids. Free Radical Research, 22(4), 375–383. DOI 10.3109/10715769509145649. [Google Scholar] [CrossRef]
22. Wang, J., Yuan, X., Jin, Z., Tian, Y., Song, H. (2007). Free radical and reactive oxygen species scavenging activities of peanut skins extract. Food Chemistry, 104(1), 242–250. DOI 10.1016/j.foodchem.2006.11.035. [Google Scholar] [CrossRef]
23. Robak, J., Gryglewski, R. J. (1988). Flavonoids are scavengers of superoxide anions. Biochemical Pharmacology, 37(5), 837–841. DOI 10.1016/0006-2952(88)90169-4. [Google Scholar] [CrossRef]
Cite This Article
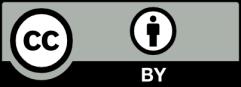
This work is licensed under a Creative Commons Attribution 4.0 International License , which permits unrestricted use, distribution, and reproduction in any medium, provided the original work is properly cited.