Open Access
ARTICLE
Effects of Fertilization on Soil CO2 Efflux in Chinese Hickory (Carya cathayensis) Stand
1
Zhejiang Provincial Key Laboratory of Carbon Cycling in Forest Ecosystems and Carbon Sequestration, Zhejiang A&F University,
Hangzhou, 311300, China
2
State Key Laboratory of Soil and Sustainable Agriculture, Institute of Soil Science, Chinese Academy of Sciences, Nanjing, 210008,
China
* Corresponding Author: Juan Liu. Email:
(This article belongs to the Special Issue: The Effect of Soil Quality Degradation on the Plant Growth, Quality and Food Safety in Subtropical Agroforestry Ecosystems)
Phyton-International Journal of Experimental Botany 2023, 92(1), 271-283. https://doi.org/10.32604/phyton.2022.023397
Received 24 April 2022; Accepted 20 May 2022; Issue published 06 September 2022
Abstract
Chinese hickory (Carya cathayensis Sarg.) is a popular nut tree in China, but there is little information about the influences of fertilization on soil CO2 efflux and soil microbial biomass. This study evaluated the short-term effects of different fertilizer applications on soil CO2 efflux and soil microbial biomass in Chinese hickory stands. Four fertilizer treatments were established: control (CK, no fertilizer), inorganic fertilizer (IF), organic fertilizer (OF), and equal parts organic and inorganic N fertilizers (OIF). A field experiment was conducted to measure soil CO2 effluxes using closed chamber and gas chromatography techniques. Regardless of the fertilization practices, soil CO2 effluxes of all the treatments showed a similar temporal pattern, with the highest value in summer and the lowest in winter. The mean annual soil CO2 efflux in the IF treatment was significantly higher than that in the CK, OIF, and OF treatments. There was no significant difference in soil CO2 efflux between the OIF, OF, and CK treatments. Soil CO2 effluxes were significantly affected by soil temperature. Soil dissolved organic carbon (DOC) was positively correlated with soil CO2 efflux only in the CK treatment. Regression analysis, including soil temperature, moisture, and DOC, showed that soil temperature was the primary factor influencing soil CO2 effluxes. Both OF and OIF treatments increased concentrations of soil microbial biomass carbon (MBC) and microbial biomass nitrogen (MBN), but decreased the ratio of MBC:MBN. These results reveal that applying organic fertilizer, either alone or combined with inorganic fertilizer, may be the optimal strategy for mitigating soil CO2 emission and improving soil quality in Chinese hickory stands.Keywords
As the largest component of carbon storage in the terrestrial ecosystem, the carbon concentration stored in forests is more than twice that of the atmosphere, and most of them are stored in soil [1]. Therefore, forest ecosystems play a key role in adjusting the global carbon cycle [2–4].
Application of organic and mineral fertilizers can influence soil CO2 efflux by altering soil properties [5–8]. In the majority of studies, forest soil respiration reduces with the addition of inorganic fertilizer, especially in the case of N addition [9]. Organic fertilizer is considered to have advantages over inorganic fertilizer because it is beneficial for soil physical properties such as structure and water retention and the accumulation of soil organic matter [10,11]. Increases in soil CO2 efflux are frequently observed in agricultural soils amended with organic fertilizer [12,13]. However, very few studies have investigated the impacts of organic fertilizer on soil CO2 efflux in subtropical forests [7,14].
As the living component of soil organic matter, soil microorganism biomass plays a significant role in the biogeochemical cycle and responds more quickly to management measures such as fertilization regime and land use change than soil organic matter as a whole [15,16]. Fertilization can have positive, negative, or no effects on soil microorganisms. A study conducted in Indian Head, Saskatchewan, Canada, showed that N applied at the rates of 50–80 kg ha−1 did not affect soil MBC in barley and corn [17]. As described in a review by Geisseler et al. [18], soil MBC was raised by the application of inorganic fertilizer in intensively managed ecosystems.
Chinese hickory (Carya cathayens Sarg.), together with American pecan (Carya illinoinensis), are the two most popular tree nut species belonging to the Carya genus [19]. Chinese hickory is an economically and commercially viable tree that is mainly distributed in the Tianmushan areas of subtropical China and is receiving increased attention as a healthy food [20,21]. At present, more than 9.33 × 104 ha of Chinese hickory is in cultivation and the total yield reached 31500 Mg in 2018 [22,23]. To achieve maximum yield and economic return, increasing numbers of growers have introduced intensive management practices on their land such as tillage, inorganic fertilizer, and organic fertilizer applications [22]. However, to our knowledge, no information is available about the effects of fertilization on soil CO2 efflux in Chinese hickory stands. This study evaluates the effects of different fertilization practices on soil CO2 efflux, soil MBC, and MBN in Chinese hickory stands in subtropical China, to explore improved fertilization practices, which could mitigate greenhouse gas emissions and have soil quality benefits.
2.1 Experimental Site Description
The study was carried out in Taihuyuan Town, Hangzhou City, Zhejiang Province, China (30°19′ N, 119°35′ E). It has a subtropical climate. The mean annual temperature is 15.8°C. The rainfall is 1600 mm. The monthly mean temperature and precipitation during the experiment are shown in Fig. 1. The attitude of the experiment site is about 100–150 m.
Figure 1: Monthly mean air temperature and cumulative rainfall during the experimental period
The Chinese hickory stands were established about 35 years before the experiment. At the time of the study, the stocking density was 300 trees ha−1 with 70% coverage. The understorey vegetation was mainly Poa annua, Ceratium glomeratum, Aster ageratoides, Ehmannia chingii, Semiaguilegia adoxoides, and Rumex japonicas, with a mean height of 0.1 m and 30% coverage. The soil type was characterized as Ferralsols [24]. The important properties of the soil (0–0.2 m) are as follows: bulk density, 1.20 g cm−3; pH (H2O), 4.69; organic C, 17.19 g kg−1; and total N, 1.06 g kg−1.
The study was established in a Chinese hickory stand, which had not received any specific previous management except for routine harvest. Sixteen 20 m × 20 m plots were randomly selected and subjected to four treatments with four replications: control (CK, no fertilizer), inorganic fertilizer (IF), organic fertilizer (OF), and equal parts organic and inorganic N fertilizers (OIF). Except for the control plots, all the other treatments received the same N rates (Table 1). The rate of N, P, and K application was 33.8, 10.8, and 22.4 kg ha−1, respectively, according to the locally recommended doses for Chinese hickory (Table 1) [25]. The organic fertilizer was commercially produced in Deqing City, Zhejiang Province, China. The contents of C, N, P, and K in organic fertilizer were 351, 30, 7.9, and 22.0 g kg−1, respectively. All urea, superphosphate, potassium chloride, and organic fertilizers were sprayed onto the soil surface and manually cultivated into 0–0.2 m soil in late May.
2.3 Measurement of Soil CO2 Efflux and Soil Sampling
Soil CO2 efflux was measured using the closed chamber method and gas chromatography techniques between July 2011 to June 2012. The chamber base (0.3 m × 0.3 m × 0.1 m) was installed and the tank was full of distilled water before gas sampling. Four samples were collected with 60 mL plastic syringes first, then stored in the airbags and determined by a chromatograph (Shimadzu, GC-2014, Japan) [26]. The sampling interval is 10 min. The gases were homogenized by pumping the gases inside three times with the sampling syringe before sampling [27]. All gas sampling was conducted at a frequency of approximately once a month, between 9:00 and 11:00 in the morning, as the CO2 efflux rate in this period was approximate to the average value [26].
At the same time of gas sampling, four topsoil (0–0.2 m) was sampled from each plot for the determination of soil moisture content and DOC. At the last collection of gas samples, the composite soil samples were gathered to analyse MBC and MBN.
2.4 Analysis of Soil Chemical and Physical Properties
Soil temperature at a depth of 0.05 m was detected with a thermometer. Soil bulk density was determined by collecting soil samples with a bulk density corer of 200 cm3. Soil moisture content was determined using the drying method in the oven at 105°C for 24 h. Soil organic C was determined with the wet combustion method. Total N was determined by the Kjeldahl method and pH value was analysed with a pH meter at the soil to extractant ratio of 1:5 (w:v).
2.5 Analysis of DOC, MBC, and MBN
Soil DOC was extracted from 10 g moist soil by adding 20 mL of distilled water. After shaking at 200 rpm for 30 min and centrifuging for 10 min at 20,000 rpm, then filtering through 0.45 μm filterable membrane, the organic carbon in the filtrate was determined by an automated TOC-TN analyser [28].
Soil MBC and MBN were analyzed using the fumigation–extraction method [29,30]. Both the fumigated and non-fumigated soil samples were extracted with K2SO4 solutions of 0.5 mol L−1 with a ratio of extractant to the soil of 5:1 (v:w). The resulting extracts were analyzed for organic carbon and nitrogen by an automated TOC-TN analyzer. The concentrations of C and N were calculated using the difference of the extracts of non-fumigated and those of the chloroform-fumigated, as described by Zhang et al. [27]. The extraction efficiency factor applied was 0.45 for C and 0.54 for N, respectively [29,30].
The effects of fertilization on CO2 and environmental factors were examined by using the one-way ANOVA and LSD test. The data were log-transformed in case of heterogeneity of variance before the ANOVA analysis. A linear regression model was adopted to analyze the relationship between soil CO2 and soil DOC, or moisture content. An exponential equation was adopted to build the relationship between soil CO2 and soil temperature. A logarithmically transformed multiple regression was conducted to analyse the relationship between soil CO2 efflux and environmental factors. The soil CO2 efflux and the cumulative CO2 emissions were calculated by the equation stated by Liu et al. [26].
Soil CO2 effluxes showed distinct dynamic changes with seasons (Fig. 2). Regardless of fertilization treatment, the effluxes were the highest in summer and the lowest in winter. Soil CO2 effluxes ranged from 51.3 to 306.2, 96.5 to 576.0, 74.3 to 284.6, and 39.1 to 360.4 mg m−2 h−1 for CK, IF, OIF, and OF treatments, respectively. The mean annual soil CO2 efflux in the IF treatment was 266.9 ± 8.4 mg m−2 h−1 (mean ± s.e.), significantly higher than that in the CK, OIF, and OF treatments (P < 0.05). The cumulative emission in the IF treatment was 18.6 ± 1.4 Mg ha−1 year−1, which was significantly higher than that in the CK, OIF, and OF treatments (P < 0.05). No significant difference was detected between CK, OIF, and OF treatments.
Figure 2: Dynamic changes in soil CO2 efflux, soil moisture content, soil temperature, and DOC concentration in the control (CK, no fertilizer), inorganic fertilizer (IF), equal parts organic and inorganic N fertilizers (OIF), and organic fertilizer (OF) treatments in Chinese hickory stands. Error bars are standard errors of means (n = 4)
3.2 Soil Temperature, Moisture, and DOC
Soil temperature at 0.05 m depth followed a seasonal pattern similar to soil CO2 efflux within the range of 0.30°C to 29.31°C (Fig. 2). The average values under CK, IF, OF, and OIF treatments were 16.17°C, 16.15°C, 16.16°C, and 16.19°C, respectively. A significant exponential correlation between soil CO2 effluxes and soil temperature was found under all treatments (Table 2).
Soil moisture content ranged from 218 to 413 g kg−1, with an average of 306 g kg−1 during the experimental period (Fig. 2). No significant difference was detected between treatments. Correlation analysis showed that there was no significant relationship between soil CO2 effluxes and soil moisture in all treatments (Table 2).
Concentrations of DOC under all treatments peaked in summer and were lowest between December to February, increasing after March (Fig. 2). Soil DOC concentrations ranged from 30.4 to 138.5, 24.2 to136.2, 39.0 to 145.4, and 40.6 to 142.0 mg kg−1 in the CK, IF, OIF, and OF treatments, respectively. The DOC concentration was significantly lower under the IF treatment than that under the CK, OF, and OIF treatments (P < 0.05). A significant correlation was determined between DOC and soil CO2 efflux in the CK treatment (P < 0.05), however, no significant correlation was observed in the three fertilization treatments (Table 2). A multiple regression analysis including soil temperature, soil moisture, and soil DOC accounted for 52%–89% of temporal variation in soil CO2 effluxes, which was very close to the results from a regression equation including soil temperature alone (Tables 2 and 3).
3.3 Soil MBC, MBN, and MBC:MBN
MBC and MBN ranged from 296.4 to 319.6 mg kg−1 and 34.6 to 74.2 mg kg−1, respectively (Fig. 3). The OF and OIF treatments showed significant increases in MBC and MBN, compared to the control (Fig. 3). The ratio of MBC:MBN was 8.3 in the control, and decreased to 4.3–6.4 when organic fertilizer was applied. The application of inorganic fertilizer did not affect soil MBC, MBN, or the ratio of MBC:MBN (Fig. 3).
Figure 3: Soil MBC, MBN, and MBC:MBN in the control (CK, no fertilizer), inorganic fertilizer (IF), equal parts organic and inorganic N fertilizers (OIF), and organic fertilizer (OF) treatments in Chinese hickory stands. Different letters represent significant differences at P < 0.05
4.1 Soil CO2 Effluxes in Chinese Hickory Stands
Information on soil respiration from Chinese hickory stands is scarce. Our results revealed that the annual soil CO2 emissions ranged from 11.8 to 18.6 Mg ha−1 year−1 in different fertilizer treatments from Chinese hickory stands in subtropical China. On average, soils in the experimental field released CO2 about 14.7 Mg ha−1 year−1 into the atmosphere, which was similar to that in Chinese fir forest (15.9 Mg ha−1 year−1) in subtropical China [31]. However, this value was much lower than that in other subtropical orchards, such as longan orchards (52.3 Mg ha−1 year−1) [32], citrus orchards (25.6 Mg ha−1 year−1) [33], and peach orchards (26.7 Mg ha−1 year−1) [34]. According to a review conducted by Song et al. [35], soil CO2 emissions range from 15.9 to 68.2 Mg ha−1 year−1 in major forest ecosystems in subtropical China. These results demonstrate that the types of land use and tree species substantially influence soil CO2 emissions in subtropical regions.
4.2 Effects of Soil Temperature, Soil Water Content, and DOC on Soil CO2 Effluxes
Soil temperature has widely been identified as the dominant factor leading to the temporal variation of soil CO2 effluxes [36,37]. The increase of soil temperature will promote microbial growth and enzyme activity and thus soil respiration. There was a significant exponential correlation between soil CO2 effluxes and soil temperature in this study, which was consistent with the results shown from other subtropical forest soils [7,33].
No significant correlation was found between soil CO2 effluxes and soil moisture in all treatments. This was in accordance with the results reported for Chinese chestnut plantations [27] and Chinese fir and Moso bamboo forests in subtropical condition [35]. This is probably because the soils are seldom affected by frequent prolonged drought in the subtropical areas. Thus, soil moisture might not limit the activities of microbes [33].
In this study, DOC showed a similar seasonal pattern to soil CO2 effluxes; however, soil DOC and CO2 efflux was positively correlated only in the CK treatment. There was no significant relationship between DOC and soil CO2 effluxes following fertilizer application. Soil disturbance caused by fertilizer application may weaken the relationship between DOC and soil CO2 efflux, as reported in other studies [26,38].
Due to the simultaneous changes of soil temperature and soil moisture, the impact of soil moisture on soil CO2 effluxes may be masked by soil temperature [39,40]. A multiple regression equation including several environmental factors showed different results when compared with an equation with only one factor included [41,42]. The results in the current study indicated that the multiple regression equation accounted for 52%–89% of the soil CO2 effluxes, which was almost the same as the conclusion of the regression model using only soil temperature (Tables 2 and 3). This suggests that soil temperature was the main factor affecting soil respiration in Chinese hickory stands.
4.3 Effects of Fertilization on MBC, MBN, and MBC:MBN
Organic amendments can promote soil microbial population increase and thereby enhance soil MBC and MBN contents due to the increased organic matter substrates and the increased root biomass [43–46]. Soil MBC and MBN were increased by 3.9%–4.2%, and 37%–112%, respectively, in the OF and OIF treatments compared with the CK treatment. In contrast, inorganic fertilizer tended to decrease soil MBC and MBN, although this was not statistically significant [47]. The ratio of MBC:MBN was between 4.3 and 8.6 in the present study, which is comparable to the result (4.7 to 8.9) of eroded red soil by artificial revegetation in subtropical China reported by Xu et al. [28]. The application of organic fertilizer alone or combined with inorganic fertilizer significantly decreased the ratio of MBC:MBN, compared with the CK treatment [47,48]. In general, fungi have a higher MBC:MBN ratio than bacteria [49,50]. Therefore, the decrease in the MBC:MBN ratio suggests that the dominant soil microbes change from fungi to bacteria groups [51]. This is supported by Joergensen et al. who reported that farmyard fertilizer application decreased the ratio of fungal groups to bacterial groups [52]. The increases in soil FB ratio might promote the conversion of more refractory C to CO2 [53–55]. This may explain the decrease of soil CO2 emissions in OF and OIF treatments of the current study partly.
4.4 Effects of Fertilization on Soil CO2 Effluxes
The nutrients from organic fertilizer are expected to affect soil CO2 efflux in the soil by increasing the availability of C substrates and enhancing microbial activity. At present, few datasets can be used to describe the impact of organic fertilizer application (either alone or in combination with inorganic fertilizer) on soil CO2 efflux from forest soil [14]. In this study, no significant difference in soil CO2 emissions was detected between the OF, OIF, and CK treatments. Microbial activity and the corresponding decomposition rate of organic amendments are usually related to the ratio of C/N [56]. Microbes need to immobilize more inorganic N when they decompose organic matter with a C/N ratio exceeding 25, the threshold for mineralization of organic N [57–60]. Although the C/N ratio of organic fertilizer applied in this experiment was only 12, there was a significant increase of MBN, and reduction of MBC:MBN under OF and OIF treatments compared with those under the CK treatment (Fig. 3). This suggests that N immobilization occurs during the decomposition of organic fertilizer. Thus, the stimulating effects of organic fertilizer application on soil CO2 efflux might be partially masked by microbial N immobilization, but further long-term studies are required to verify this potential suggestion. In addition, the relatively low application rate of organic fertilizer (34 kg ha−1 of N) may contribute to the response of soil CO2 efflux in the present study, because higher rates of organic manure (60 kg ha−1 of N) have been shown to increase soil CO2 emission in tropical forest [61].
The application of inorganic fertilizer increased soil CO2 efflux compared with the CK treatment, which is identical with the study of van Miegroet et al. [62]. With the application of inorganic fertilizer, soil microbial activity and demand for substrate was increased, which could lead to the enhancement of soil CO2 efflux and reduction of soil C content [63]. Dissolved organic C is considered to be easily utilized by microorganisms to promote CO2 emissions [27,34]. The highest cumulative soil CO2 emission and the lowest DOC concentration of IF among all the treatments in this study further confirm that the application of inorganic fertilizer increases the utilization of easily degradable organic carbon for microorganisms. Additionally, the N-limited experimental stand was partially accounting for the increase of soil CO2 efflux [64,65]. Soil CO2 efflux decreased with N additions in N-rich soils and increased in N-limited forests [66,67], which are similar to our experimental stands.
Soil CO2 effluxes over 12 months period in Chinese hickory stands indicated that there is a seasonal pattern in soil CO2 effluxes. Soil CO2 effluxes were significantly affected by soil temperature. A significant positive correlation between DOC and soil CO2 efflux rate was established only in the CK treatment. There was no correlation relationship between DOC and soil CO2 effluxes when fertilizer was applied. The logarithmic transformation multiple regression equation including soil temperature, soil moisture, and DOC does not perform better than the model only including soil temperature. Inorganic fertilizer application enhanced soil CO2 emission. The organic fertilizer, either alone or integrated with inorganic fertilizer, had no significant effect on soil CO2 emission; however, it increased soil MBC and MBN contents. We, therefore, conclude that at least in the short-term, organic fertilizer either alone or integrated with inorganic fertilizer may represent the optimal strategy for mitigating soil CO2 effluxes and maintaining soil quality in Chinese hickory stands.
Authorship: The authors confirm contribution to the paper as follows: study conception and design: Liu J. and Chen X.S.; draft manuscript preparation: Liu J. and Zheng M.Q. All authors reviewed the results and approved the final version of the manuscript.
Funding Statement: The study was funded by the Key Research and Development Plan of Zhejiang Province (No. 2019C02008-03) and the State Key Laboratory of Soil and Sustainable Agriculture, Institute of Soil Science, Chinese Academy of Sciences (No. 0812000056).
Conflicts of Interest: The authors declare that they have no conflicts of interest to report regarding the present study.
References
1. Sabine, C. L., Heimann, M., Artaxo, P., Bakker, D. C. E., Chen, C. T. A. et al. (2004). Current status and past trends of the global carbon cycle. In: Field, C. B., Raupach, M. R. (Eds.Global carbon cycle: Integrating humans, climate, and the natural world, pp. 17–44. Washington DC: Island Press. [Google Scholar]
2. Bond-Lamberty, B., Thomson, A. (2010). A global database of soil respiration data. Biogeosciences, 7(6), 1915–1926. DOI 10.5194/bg-7-1915-2010. [Google Scholar] [CrossRef]
3. Pan, Y., Birdsey, R. A., Fang, J., Houghton, R., Kauppi, P. E. et al. (2011). A large and persistent carbon sink in the world’s forests. Science, 333(6045), 988–993. DOI 10.1126/science.1201609. [Google Scholar] [CrossRef]
4. Boyd, P. W., Claustre, H., Levy, M., Siegel, D. A., Weber, T. (2019). Multi-faceted particle pumps drive carbon sequestration in the ocean. Nature, 568(7752), 327–335. DOI 10.1038/s41586-019-1098-2. [Google Scholar] [CrossRef]
5. Chen, Z. M., Xu, Y. H., He, Y. J., Zhou, X. H., Fan, J. L. et al. (2018). Nitrogen fertilization stimulated soil heterotrophic but not autotrophic respiration in cropland soils: A greater role of organic over inorganic fertilizer. Soil Biology and Biochemistry, 116, 253–264. DOI 10.1016/j.soilbio.2017.10.029. [Google Scholar] [CrossRef]
6. Subedi, P., Jokela, E. J., Vogel, J. G., Bracho, R., Inglett, K. S. (2021). The effects of nutrient limitations on microbial respiration and organic matter decomposition in a Florida Spodosol as influenced by historical forest management practices. Forest Ecology and Management, 479, 118592. DOI 10.1016/j.foreco.2020.118592. [Google Scholar] [CrossRef]
7. Huang, K., Li, Y., Hu, J., Tang, C., Cai, Y. (2021). Rates of soil respiration components in response to inorganic and organic fertilizers in an intensively-managed Moso bamboo forest. Geoderma, 403, 115212. DOI 10.1016/j.geoderma.2021.115212. [Google Scholar] [CrossRef]
8. Zhang, S. B., Li, Y. F., Singh, B. P., Wang, H. L., Cai, X. Q. et al. (2021). Contrasting short-term responses of soil heterotrophic and autotrophic respiration to biochar-based and chemical fertilizers in a subtropical Moso bamboo plantation. Applied Soil Ecology, 157, 103758. DOI 10.1016/j.apsoil.2020.103758. [Google Scholar] [CrossRef]
9. Janssens, I. A., Dieleman, W., Luyssaert, S., Subke, J. A., Reichstein, M. et al. (2010). Reduction of forest soil respiration in response to nitrogen deposition. Nature Geoscience, 3(5), 315–322. DOI 10.1038/ngeo844. [Google Scholar] [CrossRef]
10. Latif, M. A., Mehuys, G. R., Mackenzie, A. F., Alli, I., Faris, M. A. (1992). Effects of legumes on soil physical quality in a maize crop. Plant and Soil, 140(1), 15–23. DOI 10.1007/BF00012802. [Google Scholar] [CrossRef]
11. Fraser, D. G., Doran, J. W., Sahs, W. W., Lesoing, G. W. (1988). Soil microbial populations and activities under conventional and organic management. Journal of Environmental Quality, 17(4), 585–590. DOI 10.2134/jeq1988.00472425001700040011x. [Google Scholar] [CrossRef]
12. Ding, W. X., Meng, L., Yin, Y. F., Cai, Z. C., Zheng, X. H. (2007). CO2 emission in an intensively cultivated loam as affected by long-term application of organic manure and nitrogen fertilizer. Soil Biology and Biochemistry, 39(2), 669–679. DOI 10.1016/j.soilbio.2006.09.024. [Google Scholar] [CrossRef]
13. Zheng, J. F., Zhang, X. H., Li, L. Q., Zhang, P. J., Pan, G. X. (2007). Effect of long–term fertilization on C mineralization and production of CH4 and CO2 under anaerobic incubation from bulk samples and particle size fractions of a typical paddy soil. Agriculture, Ecosystems & Environment, 120(2–4), 129–138. DOI 10.1016/j.agee.2006.07.008. [Google Scholar] [CrossRef]
14. Zhang, J. J., Li, Y. F., Jiang, P. K., Zhou, G. M., Shen, Z. M. et al. (2013). Effects of fertilization on soil CO2 flux in Castanea mollissima stand. Chinese Journal of Applied Ecology, 24(9), 2431–2439 (in Chinese). [Google Scholar]
15. Wardle, D. A. (1992). A comparative assessment of factors which influence microbial biomass carbon and nitrogen levels in soil. Biological Reviews, 67(3), 321–358. DOI 10.1111/j.1469-185X.1992.tb00728.x. [Google Scholar] [CrossRef]
16. Araújo, A. S. F., Melo, W. J. (2010). Soil microbial biomass in organic farming system. Ciência Rural, 40(11), 2419–2426. DOI 10.1590/S0103-84782010005000192. [Google Scholar] [CrossRef]
17. Lupwayi, N. Z., Lafond, G. P., Ziadi, N., Grant, C. A. (2012). Soil microbial response to nitrogen fertilizer and tillage in barley and corn. Soil and Tillage Research, 118, 139–146. DOI 10.1016/j.still.2011.11.006. [Google Scholar] [CrossRef]
18. Geisseler, D., Scow, K. M. (2014). Long-term effects of mineral fertilizers on soil microorganisms–A review. Soil Biology and Biochemistry, 75, 54–63. DOI 10.1016/j.soilbio.2014.03.023. [Google Scholar] [CrossRef]
19. Li, Z. J., Qian, F. L. (1992). Achievements and measures of increase production of Carya cathayensis Sarg. Journal of Zhejiang Forestry Science and Technology, 12(6), 49–53 (in Chinese). [Google Scholar]
20. Zhao, K. L., Zhang, L. Y., Dong, J. Q., Wu, J. S., Ye, Z. Q. et al. (2020). Risk assessment, spatial patterns and source apportionment of soil heavy metals in a typical Chinese hickory plantation region of southeastern China. Geoderma, 360, 114011.DOI 10.1016/j.geoderma.2019.114011. [Google Scholar] [CrossRef]
21. Fu, W. J., Dong, J. Q., Ding, L. Z., Yang, H. S., Ye, Z. Q. et al. (2022). Spatial correlation of nutrients in a typical soil-hickory system of southeastern China and its implication for site-specific fertilizer application. Soil and Tillage Research, 217, 105265. DOI 10.1016/j.still.2021.105265. [Google Scholar] [CrossRef]
22. Wu, J. S., Qian, J. F., Tong, Z. P., Huang, J. Q., Zhao, K. L. (2014). Changes in soil organic carbon and soil microbial functional diversity of Carya cathayensis plantations under intensive managements. Chinese Journal of Applied Ecology, 25(9), 2486–2492 (in Chinese). DOI 10.13287/j.1001-9332.20140627.002. [Google Scholar] [CrossRef]
23. Shen, Y., Qian, J., Zheng, X., Yuan, Z., Wu, J. (2016). Spatial-temporal variation of soil fertility in Chinese walnut (Carya cathayensis) plantation. Scientia Silvae Sinicae, 52(7), 1–12 (in Chinese). DOI 10.11707/j.1001-7488.20160701. [Google Scholar] [CrossRef]
24. World Reference Base for Soil Resources (WRB) (2006). A framework for international classification, correlation and communication. Rome: Food and Agriculture Organization of the United Nations. [Google Scholar]
25. Huang, C. P., Wu, J. S., Xu, Q. F., Jiang, P. K. (2012). Runoff losses of nitrogen and phosphorus under Carya cathayensis Sarg. stand with different fertilization. Journal of Soil and Water Conservation, 26(1), 43–52 (in Chinese). [Google Scholar]
26. Liu, J., Jiang, P. K., Wang, H. L., Zhou, G. M., Wu, J. S. et al. (2011). Seasonal soil CO2 efflux dynamics after land use change from a natural forest to Moso bamboo plantations in subtropical China. Forest Ecology and Management, 262(6), 1131–1137. DOI 10.1016/j.foreco.2011.06.015. [Google Scholar] [CrossRef]
27. Zhang, J. J., Li, Y. F., Chang, S. X., Jiang, P. L., Zhou, G. M. et al. (2014). Understory vegetation management affected greenhouse gas emissions and labile organic carbon pools in an intensively managed Chinese chestnut plantation. Plant and Soil, 376(1–2), 363–375. DOI 10.1007/s11104-013-1996-2. [Google Scholar] [CrossRef]
28. Xu, Q. F., Jiang, P. K., Wang, H. L. (2010). Improvement of biochemical and biological properties of eroded red soil by artificial revegetation. Journal of Soils and Sediments, 10(2), 255–262. DOI 10.1007/s11368-009-0100-2. [Google Scholar] [CrossRef]
29. Brookes, P. C., Landman, A., Pruden, G., Jenkinson, D. S. (1985). Chloroform fumigation and the release of soil nitrogen: A rapid direct extraction method for measuring microbial biomass nitrogen in soil. Soil Biology and Biochemistry, 17(6), 837–842. DOI 10.1016/0038-0717(85)90144-0. [Google Scholar] [CrossRef]
30. Vance, E. D., Brookes, P. C., Jenkinson, D. S. (1987). An extraction method for measuring soil microbial biomass C. Soil Biology and Biochemistry, 19(6), 703–707. DOI 10.1016/0038-0717(87)90052-6. [Google Scholar] [CrossRef]
31. Wang, G. J., Tian, D. L., Yan, W. D., Zhu, F., Xiang, W. H. et al. (2009). Effects of aboveground litter exclusion and addition on soil respiration in a Cunninghamia lanceolata plantation in China. Chinese Journal of Plant Ecology, 33(4), 739–747 (in Chinese). DOI 10.3773/j.issn.1005-264x.2009.04.012. [Google Scholar] [CrossRef]
32. Liu, H., Zhao, P., Lu, P., Wang, Y. S., Lin, Y. B. et al. (2008). Greenhouse gas fluxes from soils of different land-use types in a hilly area of South China. Agriculture, Ecosystems & Environment, 124(1–2), 125–135. DOI 10.1016/j.agee.2007.09.002. [Google Scholar] [CrossRef]
33. Sheng, H., Yang, Y. S., Yang, Z. J., Chen, G. S., Xie, J. S. et al. (2010). The dynamic response of soil respiration to land–use changes in subtropical China. Global Change Biology, 16(3), 1107–1121. DOI 10.1111/j.1365-2486.2009.01988.x. [Google Scholar] [CrossRef]
34. Iqbal, J., Hu, R. G., Du, L. J., Lu, L., Lin, S. et al. (2008). Differences in soil CO2 flux between different land use types in mid-subtropical China. Soil Biology and Biochemistry, 40(9), 2324–2333. DOI 10.1016/j.soilbio.2008.05.010. [Google Scholar] [CrossRef]
35. Song, X. Z., Yuan, H. Y., Kimberley, M. O., Jiang, H., Zhou, G. M. et al. (2013). Soil CO2 flux dynamics in the two main plantation forest types in subtropical China. Science of the Total Environment, 444, 363–368. DOI 10.1016/j.scitotenv.2012.12.006. [Google Scholar] [CrossRef]
36. Paré, D., Boutin, R., Larocque, G. R., Raulier, F. (2006). Effect of temperature on soil organic matter decomposition in three forest biomes of Eastern Canada. Canadian Journal of Soil Science, 86(2), 247–256.DOI 10.4141/S05-084. [Google Scholar] [CrossRef]
37. Ruehr, N. K., Buchmann, N. (2010). Soil respiration fluxes in a temperate mixed forest: Seasonality and temperature sensitivities differ among microbial and root–rhizosphere respiration. Tree Physiology, 30(2), 165–176. DOI 10.1093/treephys/tpp106. [Google Scholar] [CrossRef]
38. Cook, B. D., Allan, D. L. (1992). Dissolved organic carbon in old field soils: Total amounts as a measure of available resources for soil mineralization. Soil Biology and Biochemistry, 24(6), 585–594. DOI 10.1016/0038-0717(92)90084-B. [Google Scholar] [CrossRef]
39. Wagai, R., Brye, K. R., Gower, S. T., Norman, J. M., Bundy, L. G. (1998). Land use and environmental factors influencing soil surface CO2 flux and microbial biomass in natural and managed ecosystems in southern Wisconsin. Soil Biology and Biochemistry, 30(12), 1501–1509. DOI 10.1016/S0038-0717(98)00041-8. [Google Scholar] [CrossRef]
40. Davidson, E. A., Verchot, L. V., Cattânio, J. H., Ackerman, I. L., Carvalho, J. E. M. (2000). Effects of soil water content on soil respiration in forests and cattle pastures of Eastern Amazonia. Biogeochemistry, 48(1), 53–69. DOI 10.1023/A:1006204113917. [Google Scholar] [CrossRef]
41. Ding, W. X., Yu, H. Y., Cai, Z. C., Han, F. X., Xu, Z. H. (2010). Responses of soil respiration to N fertilization in a loamy soil under maize cultivation. Geoderma, 155(3–4), 381–389. DOI 10.1016/j.geoderma.2009.12.023. [Google Scholar] [CrossRef]
42. Ni, K., Ding, W. X., Cai, Z. C., Wang, Y. F., Zhang, X. L. (2012). Soil carbon dioxide emission from intensively cultivated black soil in Northeast China: Nitrogen fertilization effect. Journal of Soils and Sediments, 12(7), 1007–1018. DOI 10.1007/s11368-012-0529-6. [Google Scholar] [CrossRef]
43. Gong, W., Yan, X. Y., Wang, J. Y., Hu, T. X., Gong, Y. B. (2009). Long-term fertilizer and fertilizer effects on soil organic matter fractions and microbes under a wheat–maize cropping system in northern China. Geoderma, 149(3–4), 318–324. DOI 10.1016/j.geoderma.2008.12.010. [Google Scholar] [CrossRef]
44. Gu, Y. F., Zhang, X. P., Tu, S. H., Lindström, K. (2009). Soil microbial biomass, crop yields, and bacterial community structure as affected by long-term fertilizer treatments under wheat-rice cropping. European Journal of Soil Biology, 45(3), 239–246. DOI 10.1016/j.ejsobi.2009.02.005. [Google Scholar] [CrossRef]
45. Manna, M. C., Bhattacharyya, P., Adhya, T. K., Singh, M., Wanjari, R. H. et al. (2013). Carbon fractions and productivity under changed climate scenario in soybean–wheat system. Field Crops Research, 145, 10–20. DOI 10.1016/j.fcr.2013.02.004. [Google Scholar] [CrossRef]
46. Nie, J., Zhou, J. M., Wang, H. Y., Chen, X. Q., Du, C. W. (2007). Effect of long–term rice straw return on soil glomalin carbon and nitrogen. Pedosphere, 17(3), 295–302. [Google Scholar]
47. Liu, E. K., Yan, C. H., Mei, X. R., He, W. Q., Bing, S. H. et al. (2010). Long-term effect of chemical fertilizer, straw, and manure on soil chemical and biological properties in Northwest China. Geoderma, 158(3–4), 173–180. DOI 10.1016/j.geoderma.2010.04.029. [Google Scholar] [CrossRef]
48. Anderson, J. P. E., Domsch, K. H. (1980). Quantities of plant nutrients in the microbial biomass of selected soils. Soil Science, 130(4), 211–215. DOI 10.1097/00010694-198010000-00008. [Google Scholar] [CrossRef]
49. Wang, X., Zhang, W., Shao, Y., Zhao, J., Zhou, L. et al. (2019). Fungi to bacteria ratio: Historical misinterpretations and potential implications. Acta Oecologica, 95, 1–11. DOI 10.1016/j.actao.2018.10.003. [Google Scholar] [CrossRef]
50. Chu, H. Y., Grogan, P. (2010). Soil microbial biomass nutrient availability and potential among vegetation-types in a row Arctic tundra landscape. Plant and Soil, 329(1–2), 411–420. DOI 10.1007/s11104-009-0167-y. [Google Scholar] [CrossRef]
51. Joergensen, R. G., Mäder, P., Fließbach, A. (2010). Long-term effects of organic farming on fungal and bacterial residues in relation to microbial energy metabolism. Biology and Fertility of Soils, 46(3), 303–307. DOI 10.1007/s00374-009-0433-4. [Google Scholar] [CrossRef]
52. Zhou, T., Wang, C., Zhou, Z. (2020). Impacts of forest thinning on soil microbial community structure and extracellular enzyme activities: A global meta-analysis. Soil Biology and Biochemistry, 149, 107915. DOI 10.1016/j.soilbio.2020.107915. [Google Scholar] [CrossRef]
53. Lei, L., Xiao, W., Zeng, L., Zhu, J., Huang, Z. et al. (2018). Thinning but not understory removal increased heterotrophic respiration and total soil respiration in Pinus massoniana stands. Science of the Total Environment, 621, 1360–1369. DOI 10.1016/j.scitotenv.2017.10.092. [Google Scholar] [CrossRef]
54. Lei, L., Wen, F. X., Zeng, L. X., Frey, B., Huang, Z. L. et al. (2021). Effects of thinning intensity and understory removal on soil microbial community in Pinus massoniana plantations of subtropical China. Applied Soil Ecology, 167, 104055. DOI 10.1016/j.apsoil.2021.104055. [Google Scholar] [CrossRef]
55. Muhammad, W., Vaughan, S. M., Dalal, R. C., Menzies, N. W. (2011). Crop residues and fertilizer nitrogen influence residue decomposition and nitrous oxide emission from a Vertisol. Biology and Fertility of Soils, 47(1), 15–23. DOI 10.1007/s00374-010-0497-1. [Google Scholar] [CrossRef]
56. Sollins, P., Spycher, G., Glassman, C. A. (1984). Net nitrogen mineralisation from light and heavy–fraction forest soil organic matter. Soil Biology and Biochemistry, 16(1), 31–37. DOI 10.1016/0038-0717(84)90122-6. [Google Scholar] [CrossRef]
57. Zak, D. R., Tilman, D., Parmenter, R. R. (1994). Plant production and soil microorganisms in late–successional ecosystems: A continental–scale study. Ecology, 75(8), 2333–2347. DOI 10.2307/1940888. [Google Scholar] [CrossRef]
58. Janssen, B. H. (1996). Nitrogen mineralization in relation to C/N ratio and decomposability of organic materials. Plant and Soil, 181(1), 39–45. DOI 10.1007/BF00011290. [Google Scholar] [CrossRef]
59. Powlson, D. S., Hirsch, P. R., Brookes, P. C. (2001). The role of soil microorganisms in soil organic matter conservation in the tropics. Nutrient Cycling in Agroecosystems, 61(1–2), 41–51. DOI 10.1023/A:1013338028454. [Google Scholar] [CrossRef]
60. de Urzedo, D. I., Franco, M. P., Pitombo, L. M., do Carmo, J. B. (2013). Effects of organic and inorganic fertilizers on greenhouse gas (GHG) emissions in tropical forestry. Forest Ecology and Management, 310, 37–44. DOI 10.1016/j.foreco.2013.08.018. [Google Scholar] [CrossRef]
61. Hasselquist, N. J., Metcalfe, D. B., Högberg, P. (2012). Contrasting effects of low and high nitrogen additions on soil CO2 flux components and ectomycorrhizal fungal sporocarp production in a boreal forest. Global Change Biology, 18(12), 3596–3605. DOI 10.1111/gcb.12001. [Google Scholar] [CrossRef]
62. van Miegroet, H., Jandl, R. (2007). Are nitrogen–fertilized forest soils sinks or sources of carbon? Environmental Monitoring and Assessment, 128(1–3), 121–131. DOI 10.1007/s10661-006-9410-7. [Google Scholar] [CrossRef]
63. Hyvönen, R., Ågren, G. I., Linder, S., Persson, T., Cotrufo, M. F. et al. (2007). The likely impact of elevated CO2, nitrogen deposition, increased temperature and management on carbon sequestration in temperate and boreal forest ecosystems: A literature review. New Phytologist, 173(3), 463–480. DOI 10.1111/j.1469-8137.2007.01967.x. [Google Scholar] [CrossRef]
64. Yan, E. R., Wang, X. H., Zhou, W. (2008). N: P stoichiometry in secondary succession in evergreen broad–leaved forest, Tiantong, East China. Chines Journal of Plant Ecology, 32(1), 13–22 (in Chinese). DOI 10.3773/j.issn.1005-264x.2008.01.002. [Google Scholar] [CrossRef]
65. Cusack, D., Silver, W., Torn, M., McDowell, W. (2011). Effects of nitrogen additions on above- and belowground carbon dynamics in two tropical forests. Biogeochemistry, 104(1–3), 203–225. DOI 10.1007/s10533-010-9496-4. [Google Scholar] [CrossRef]
66. Gao, Q., Hasselquist, N. J., Palmroth, S., Zheng, Z., You, W. H. (2014). Short-term response of soil respiration to nitrogen fertilization in a subtropical evergreen forest. Soil Biology and Biochemistry, 76, 297–300. DOI 10.1016/j.soilbio.2014.04.020. [Google Scholar] [CrossRef]
67. Marschner, P., Kandeler, E., Marschner, B., Patra, A. K. (2003). Structure and function of the soil microbial community in a long–term fertilizer experiment. Soil Biology and Biochemistry, 35(3), 453–461. DOI 10.1016/S0038-0717(02)00297-3. [Google Scholar] [CrossRef]
Cite This Article
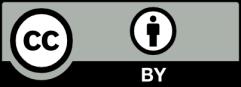