Open Access
ARTICLE
Observation on Flower Bud Differentiation of Crape Myrtle in Red Soil Environment
1
College of Landscape and Architecture, Zhejiang Agriculture & Forestry University, Hangzhou, 311300, China
2
Zhejiang Provincial Key Laboratory of Germplasm Innovation and Utilization for Garden Plants, Zhejiang Agriculture & Forestry
University, Hangzhou, 311300, China
3
Key Laboratory of National Forestry and Grassland Administration on Germplasm Innovation and Utilization for Southern Garden
Plants, Zhejiang Agriculture & Forestry University, Hangzhou, 311300, China
4
Jiyang College of Zhejiang Agriculture & Forestry University, Shaoxing, 311800, China
* Corresponding Authors: Cuihua Gu. Email: ; Qun Wang. Email:
# These authors contributed equally to this work
(This article belongs to the Special Issue: The Effect of Soil Quality Degradation on the Plant Growth, Quality and Food Safety in Subtropical Agroforestry Ecosystems)
Phyton-International Journal of Experimental Botany 2022, 91(12), 2607-2617. https://doi.org/10.32604/phyton.2022.022485
Received 12 March 2022; Accepted 14 April 2022; Issue published 29 August 2022
Abstract
Flower bud differentiation is a key component of plant blooming biology and understanding how it works is vital for flowering regulation and plant genetic breeding, increasing the number and quality of flowering. Red soil is the most widely covered soil type in the world, and it is also the most suitable soil type for crape myrtle planting. The flower buds of crape myrtle (Lagerstroemia indica) planted in red soil were employed as experimental materials in this study, and the distinct periods of differentiation were identified using stereomicroscopy and paraffin sectioning. We optimized the steps of dehydration, transparency, embedding, sectioning and staining when employing paraffin sections. When seen under a microscope, this optimization can make the cell structure of paraffin sections obvious, the tissue structure complete, and the staining clear and natural. The flower bud differentiation process is divided into 7 periods based on anatomical observations of the external morphology and internal structure during flower bud differentiation: undifferentiated period, start of differentiation period, inflorescence differentiation period, calyx differentiation period, petal differentiation period, stamen differentiation period, and pistil differentiation period. The differentiation time is concentrated from the end of May to mid-June. Crape myrtle flower bud differentiation is a complicated process, and the specific regulatory mechanism and affecting elements need to be investigated further.Keywords
Crape myrtle (Lagerstroemia indica) is the most common deciduous shrub or small tree in the genus Lagerstroemia of Lythraceae [1]. With a flowering duration of up to 100 d, it is one of the few woody plants that bloom in the summer and is widely used around the world [2]. The red soil in Hangzhou is rich in iron and aluminum, which can greatly improve the growth and development of plants [3]. Flower bud differentiation is an important stage of plant blooming, which is related to the quality and quantity of plant flowering [4–6]. At present, crape myrtle research is mostly focused on resource evaluation, molecular markers and new variety breeding [7–10]. There is no research on the outward morphological and internal structural changes that occur during crape myrtle flower bud differentiation. Therefore, it is of great significance to study the flower bud differentiation process of crape myrtle to improve and regulate the flowering period.
Red soil is rich in organic matter, mainly distributed in the south of the Yangtze River. From south to north, the red soil series includes laterite, dry red soil, latosolic red soil, red soil, and yellow soil [11]. High temperatures and rain are common in red soil locations, as well as distinct dry and wet seasons [3]. It is the most suitable soil type for crape myrtle. At present, wild crape myrtle is mainly distributed in red soil areas. The study of crape myrtle flower bud differentiation in red soil has some practical implications for cultivating original species.
Paraffin sectioning is a method used to observe the structural morphology of cells as well as tissues. Conventional paraffin sections are cumbersome, with long experimental cycles, poor sectioning results and low success rates [12], and cannot meet the needs of observing the structural and morphological changes in the flower buds of crape myrtle. The internal structural changes during bud differentiation were detected using crape myrtle flower buds as experimental material, and the enhanced paraffin sectioning method was used to capture the outer morphology of flower buds. We want to learn the fundamentals of flower bud differentiation, grasp the differentiation pattern, and establish a reference base for flower bud differentiation research in crape myrtle.
2.1 Study Site and Plant Materials
These resources were obtained from the crape myrtle Germplasm Resource Bank of Zhejiang Agriculture & Forestry University (located at 30°15′2″ N/119°43′37″E). As test materials, perennial crape myrtle plants with steady, vigorous growth and no illnesses or pests were chosen. The flower buds near the same position of the terminal buds were randomly selected from the shoots after the second shooting in different directions on the current year’s branches. The observation started on March 09, 2021, and the sampling period lasted from May 07 to June 30.
The shoots were observed every 5 d after the second shooting. When it was found that the vegetative growth was completed, the flower buds began to differentiate, and the observation time was shortened to once a day, recorded and sampled. Three samples were randomly selected from different branch directions in the middle and upper part of the crown of 6 crape myrtle trees, and at least 20 flower buds were collected for each sample. After each sampling, one sample was selected and placed under a stereomicroscope to observe the external structure and record the external changes of flower buds, The other two samples were fixed in FAA fixation solution (50% anhydrous ethanol, formaldehyde and glacial acetic acid in a volume ratio of 8:1:1) for 24 h and liquid nitrogen at –80°C, respectively. The internal structure of the sections was observed using a modified paraffin sectioning method.
On the basis of routine preparation, the technical process of paraffin preparation was optimized and the preparation cycle was shortened in this study by enhancing the operating procedures of dehydration, transparency, embedding, and staining. The treatment was carried out step by step in a gradient from low to high concentration in the dehydration and transparency phases. Adjusting the period of use of the transparent agent (dimethylbenzene) effectively decreased the risk of harmful chemicals to the operator while also improving the safety of the experiment. The usage of a pathological embedding box for material embedding has increased embedding success rates, outperforming the traditional paper box approach. Sections were generated using a laboratory biological sectioning machine (Leica RM2335, Germany) with five different section thickness settings to identify the best suited sectioning results for comparison. Finally, the final staining of the plant material was carried out using four staining methods: Hematoxylin staining, Fast green fast staining method, Muscovy solid green double staining method and Aniline blue staining method. The samples were sealed with neutral balata and then observed and photographed using an Axio Imager A2 positive fluorescence microscope (Carl Zeiss, Oberkochen, Germany).
All data were counted by Excel (Microsoft Office Home and Student, 2019), and the broken line diagram was drawn by origin 2021 software (OlriginLab Co., Northampton, MA, USA) and labeled with the significance of variations between time periods, and the bud differentiation process was plotted in Adobe Illustrator CC 2019.
From March 28, 2021, the manually pruned crape myrtle began to show newborn nutritional bud on old branches. On April 06, the nutritional buds began to spread their leaves, and new branches continued to develop, with a second shooting at the top of the new branches near the terminal buds, which were still in the nutritional growth period (Fig. 1).
Figure 1: Vegetative growth period of crape myrtle. A: Bud spots appear on old branches; B: Leaf unfolding; C: Vegetative growth period
Records were started at 0 d (March 28, 2021) when crape myrtle grew newborn nutritional buds. Nutritional growth was completed 57 d after the second shooting (May 25, 2021), pistils and stamens developed 82 d later (June 18, 2021), and floral bud differentiation ended 92 d later (June 28, 2021), developing floral organs into the early flowering period (Fig. 2). In the process of flower bud differentiation, the outer shape and size of crape myrtle changed, and the diameter and length of flower bud increased significantly (Fig. 3).
Figure 2: Flower bud differentiation process of crape myrtle. UP, Undifferentiated period; SDP, Start of differentiation period; IDP, Inflorescence differentiation period; CDP, Calyx differentiation period; PDP, Petal differentiation period; PSDP, Pistil and stamen differentiation period
Figure 3: Morphological changes of flower bud differentiation in crape myrtle. UP, Undifferentiated period; SDP, Start of differentiation period; IDP, Inflorescence differentiation period; CDP, Calyx differentiation period; PDP, Petal differentiation period; PSDP, Pistil and stamen differentiation period
To acquire the ideal section thickness for observing V. purpurea buds, five section thickness gradients were set: 7 μm, 9 μm, 10 μm, 12 μm, and 15 μm. The sectioning effects were observed under the microscope after the implanted materials were sectioned, stained, and sealed. Tissue integrity and staining effect were best when the section thickness was 9 μm; less than this thickness, cell crumpling, tissue breakage, and relatively light staining occurred; greater than this thickness, cell accumulation, blurred section tissue, overly deep staining, and unclear arrangement of cell structure occurred; and greater than this thickness, cell accumulation, blurred section tissue, overly deep staining, and unclear arrangement of cell structure occurred (Fig. 4).
Figure 4: Renderings of different section thicknesses (×5, Bar = 200 μm). A: The thickness of flower bud section was 7 μm; B: The thickness of flower bud section was 9 μm; C: The thickness of flower bud section was 12 μm
The staining materials were chosen from the same dehydrated and translucent wax immersion time treatment as before to ensure uniformity of the staining results, and the section thickness was decided to be 9 μm, which yielded the best results. The morphology of the buds could be seen in the hematoxylin stained sections, but the cellular organization and structure were barely visible, and the internal cellular arrangement was blurred; the morphology of the buds could be seen in the solid green fast stained sections, but the staining was too thick, and the cellular structure and other tissues were blurred; the sections stained with muscovy solid green double staining showed a blue-green color, the morphology of flower buds was clear, the morphology and structure were clearly visible, and the arrangement of cell tissues was very intuitive; the sections stained with aniline blue color showed a blue color, the morphology of flower buds was difficult to observe, and the phenomenon of wax strips falling off during the filming process was not suitable for observation (Fig. 5).
Figure 5: Renderings of crape myrtle flower buds dyed by different staining methods (×5, Bar = 200 μm). A: Hematoxylin staining method; B: Fast green fast staining method; C: Muscovy solid green double staining method; D: Aniline blue staining method
3.3 Periods Division of Flower Bud Differentiation
The growth cone of the crape myrtle develops initially from the terminal flower primordium, followed by the continued creation of fresh floret primordium on either side, eventually forming a complete panicle. Based on the results of the improved paraffin sectioning method and stereomicroscope observation records, the flower bud differentiation process is divided into 7 periods: undifferentiated period, start of differentiation period, inflorescence differentiation period, calyx differentiation period, petal differentiation period, stamen differentiation period, and pistil differentiation period (Figs. 6 and 7). Differentiation is rather quick from the start to the finish of bud differentiation, which takes roughly 35 d in total.
Figure 6: Morphological and structural changes of crape myrtle during flower bud. A: Undifferentiated period; B: Start of differentiation period; C: Inflorescence differentiation period; D: Calyx differentiation period; E: Petal differentiation period; F: Pistil and stamen differentiation period
Figure 7: Paraffin section of internal structure of crape myrtle during flower bud process (×5, Bar = 200 μm). A: Undifferentiated period; B: Start of differentiation period; C: Inflorescence differentiation period; D: Calyx differentiation period; E: Petal differentiation period; F: Stamen differentiation period; G: Pistil differentiation period; H: Flower organ formation period. GP: Growing point; FL: Flower primordium; SF: Florets primordium; SE: Sepal primordium; PE: Petal primordium; ST: Stamen primordium; PI: Pistil primordium; Ova: Ovary
The fresh flower bud of crape myrtle is a soft green color. The entire bud is smooth and hairless, with the tip of new bud being sharper and still a leafy bud at this point, and both sides of its growth are covered in immature scales (Fig. 6A). The growth points inside the buds are cuspidate, the growth cones are narrower, and the cells are smaller and more tightly grouped, as shown in this section (Fig. 7A).
3.3.2 Start of Differentiation Period
During this time, the flower bud begins to expand at the base, becoming more pointed at the tip and fuller at the bottom, as opposed to the undifferentiated period (Fig. 6B). The growth cone inside the bud begins to flatten and swell thickly to a hemispheric form, the top of the floral primordium is smooth and darkly coloured, and the cells are tightly organized, as shown in this section (Fig. 7B).
3.3.3 Inflorescence Differentiation Period
The base of the flower bud extends to a semi-circular form, and the bud tips flatten out as it continues to stretch and become coarser (Fig. 6C). The inner protruding growth cone continues to widen and grow from the inside to the outside, with a spherical top, and multiple small protrusions differentiated on both sides of the growth cone, each protrusion being a florets primordium, which forms a panicle on the inflorescence axis after the florets primordium is formed (Fig. 7C).
3.3.4 Calyx Differentiation Period
In comparison to the inflorescence differentiation period, the entire flower bud has a somewhat flattened exterior morphological structure. However, the base of the flower bud becomes broader (Fig. 6D). Section shows that the inner growth cone of the flower bud gradually elongates and depresses until it is flattened and no longer semicircular, and two bumps appear, which are the sepal primordium (Fig. 7D).
3.3.5 Petal Differentiation Period
With two sepals wrapped around the outside of the flower bud, the flower bud gets longer and wider, the overall structure is flatter than during the calyx differentiation period, and the middle region of the flower bud has been clearly divided from the leafy buds (Fig. 6E). The section shows that the inner growth cone of the bud elongates and deforms, with depressions and bumps at the periphery, and then curved into a semi-closed calyx tube from bottom to top, with multiple small protrusions on the inner growth point of the calyx tube, which is the petal primordium (Fig. 7E).
3.3.6 Stamen Differentiation Period
The overall structure of the flower bud expands and fills out, and there are two sepals wrapped around it. The hue is slightly purple red (Fig. 6F). Many little granular protuberances around the inner growth cone of the closed calyx tube, which are stamen primordium, can be seen in sections. Many long and short stamens are introduced at the base of the calyx tube and differentiate into many whorls of stamen primordium that are closely packed from outside to inside in crape myrtle (Fig. 7F).
3.3.7 Pistil Differentiation Period
The floral organ was developed quickly after the flower bud differentiation and entered the early flowering period (Fig. 7H), and there was no notable variation in appearance between the flower bud and stamen periods (Fig. 6F). Multiple whorls of stamen primordium and a pointed protuberance in the middle of the top, which is the pistil primordium, may be seen in section of the inner growth cone of the closed calyx tube (Fig. 7G).
Crape myrtle is a summer and autumn flowering plant, and floral bud differentiation in woody plants is primarily comprised of the differentiation of growth points on their branches into nutritive buds, which are then turned into floral buds [13]. Flower bud differentiation is a physiological, biochemical, and morphogenetic process that is required for plant flowering [14]. Flower bud differentiation in plants is a continuous process [15] with a specific temporal sequence, and the morphology remains largely the same throughout differentiation, which is usually artificially divided into periods based on the appearance of each part of the primordium, and the rate of differentiation reflects the plant’s own characteristics [16]. Flower bud differentiation is a more important stage in the growth and development of flowering plants, so it is crucial to investigate the mechanism of flower bud differentiation in crape myrtle in terms of the entire process of branch changes, flower bud appearance and morphology, and internal structure for flowering regulation and breeding. Flower bud differentiation in Magnolia sinostellata was separated into five periods by Fan et al. [17] each lasting 32 d: undifferentiated, early flower bud differentiation, petal primordium differentiation, stamen primordium differentiation, and pistil primordium differentiation. The flower development of Eriobotrya japonica [18] was separated into five periods based on morphological and tissue alterations: physiological differentiation, morphological establishment, petal primordium differentiation, stamen differentiation, and pistil differentiation. In this experiment, the flower bud differentiation period of crape myrtle was divided into seven periods: undifferentiated, beginning of differentiation, inflorescence differentiation, calyx differentiation, petal differentiation, stamen differentiation, and pistil differentiation, which lasted 35 d from the beginning of differentiation to the end of differentiation, using a modified paraffin section technique.
The flower buds of crape myrtle were small in the undifferentiated stage, but enlarged and expanded when the pistil and stamen formed, according to stereomicroscopy and vernier caliper results. The axillary buds near the top shoot twice after one month of new vegetative bud growth, then enter the vigorous period of vegetative growth, and eventually enter the state of flower bud differentiation. However, it was discovered that during the process of flower bud differentiation during the vegetative growth period, the apex of the branch was prone to ‘black’ fracture, which was most likely caused by pests and diseases [19]. Once the vegetative growth is complete, it will reach the flower bud differentiation stage, which is very susceptible to this. From the start of differentiation until the appearance of pistil and stamen takes only 25 d. Flower bud differentiation is a complicated process that is not only influenced by numerous aspects of its own genetic regulation [20,21], but is also intricately tied to environmental factors [22,23]. In this study, crape myrtle began flower bud differentiation at the end of May. With the progress of flower bud differentiation, it entered the pistil and stamen primordium differentiation period on June 18 and the initial flowering period around July 02. Crape myrtle of Hunan Academy of Forestry Sciences can see the pistil and stamen around 09 June and partially bloom from June 23 to 30 [24]. The L. indica ‘Petite pinkie’ in Wuhan was in the pistil differentiation stage on May 24, flowering last from late May to September [25]. Compared with the red soil in Hangzhou, the soil in Changsha is more fertile, consisting of red soil and fluvial alluvial soil [26]. Wuhan area is light loam and yellow sand soil, which are rich in organic matter and provide more comprehensive plant nutrients. This may be one of the reasons effecting its flower bud differentiation and late flowering in Hangzhou. In woody plants, various branches of the same tree exist, yet flower bud differentiation does not occur at the same time [27]. In crape myrtle, trimming towards the end of the initial anthesis could induce secondary flower bud differentiation and anthesis [28]. Therefore, the factors affecting the flower bud differentiation of crape myrtle need to be further studied.
The traditional paraffin sectioning procedure cannot be used on all tissues due to the unique characteristics of the plant material. If the dehydrating agent is promptly sucked for 5–8 min at the appropriate time for dehydration, the tissue structure will not distort [29]. Because Vernicia fordii has a complex inflorescence structure, Fu et al. [30] were able to increase production by removing extraneous bracts, branches, and other tangled fluff outside the sepals of florets, which can cause the solvent and wax liquid to become inseparable. In this experiment, we looked at the steps of dehydration, transparency, wax dipping, sectioning, and staining with the material of crape myrtle flower buds, and discovered that the best sectioning effect was obtained by combining the dehydration and transparency times of 50 min and 45 min, respectively. crape myrtle flower buds should be completely immersed in wax for 3 d, with the wax being replenished every 12 h. The slice thickness should be 9 μm, and using the Muscovy solid green double staining technique for 20 s improves the result. This approach allows for the observation of clear cell structures, entire tissue structures, and clear and natural dyed slices under the microscope.
In summary, our study revealed morphological changes during floral bud differentiation in crape myrtle through an improved paraffin sectioning technique. Emerging nutritive buds started to differentiate into flower buds after 57 d of leaf expansion. The differentiation process can be divided into seven periods based on morphological changes in bud differentiation: undifferentiated period, beginning of differentiation, inflorescence differentiation, calyx differentiation, petal differentiation, stamen differentiation, and pistil differentiation, with the differentiation time concentrating primarily in late May to mid-late June. The current research provides a theoretical foundation for studying flower bud differentiation and a morphological foundation for studying the molecular process of flower development in crape myrtle, as well as some guidance for flowering management and variety selection in crape myrtle.
Author Contributions: Cuihua Gu and Qun Wang were involved in the conception and design, drafting of the paper; Linxue Shang, Dandan Ma, Sidan Hong, Yu Zhao, Guozhe Zhang, Qingqing Ma were involved in the material preparation, data collection and analysis. The first draft of the manuscript was written by Linxue Shang, Dandan Ma, Sidan Hong, Yu Zhao, Guozhe Zhang, and all authors commented on previous versions of the manuscript. All authors agree to be accountable for all aspects of the work.
Funding Statement: This work was financially supported by Zhejiang Provincial Natural Science Foundation of China (No. LY21C160001); Zhejiang Science and Technology Major Program on Agricultural New Variety Breeding (No. 2021C02071-4); Natural Science Foundation of Zhejiang Province (LQ17C160005).
Conflicts of Interest: The authors declare that they have no conflicts of interest to report regarding the present study.
References
1. Pooler, M. (2006). Crape myrtle. In: Anderson, N. O. (Ed.Flower breeding and genetics: Issues, challenges and opportunities for the 21st century, pp. 439–457. Springer, Netherlands. [Google Scholar]
2. Zhang, Q. X. (1991). Studies on cultivars of crape myrtle (Lagerstroemia indica) and their uses in urban greening. Journal of Beijing Forestry University, 13(4), 57–66. DOI 10.13332/j.1000-1522.1991.04.009. [Google Scholar] [CrossRef]
3. Xiao, D., Huang, Y., Feng, S. Z., Ge, Y. H., Zhang, W. et al. (2018). Soil organic carbon mineralization with fresh organic substrate and inorganic carbon additions in a red soil is controlled by fungal diversity along a pH gradient. Geoderma, 321(3), 79–89. DOI 10.1016/j.geoderma.2018.02.003. [Google Scholar] [CrossRef]
4. Chutinanthakun, T., Sekozawa, Y., Sugaya, S., Gemma, H. (2015). Effect of bending and the joint tree training system on the expression levels of GA3- and GA2-oxidases during flower bud development in ‘Kiyo’ Japanese plum. Scientia Horticulturae, 193, 308–315. DOI 10.1016/j.scienta.2015.07.023. [Google Scholar] [CrossRef]
5. Liang, C. L., Liu, M. J., Zhao, J. (2005). Research progress on plant seeds abortion. Molecular Plant Breed, 3(1), 117–122. [Google Scholar]
6. Shao, F. X., Wang, S., Chen, J., Hong, R. Y. (2019). Megasporogenesis, microsporogenesis, and development of female and male gametophytes of Ziziphus jujuba Mill. ‘Zhongqiusucui’. HortScience, 54(10), 1686–1693. DOI 10.21273/HORTSCI14237-19. [Google Scholar] [CrossRef]
7. Ye, Y. J., Liu, Y., Cai, M., He, D., Shen, J. S. et al. (2015). Screening of molecular markers linked to dwarf trait in crape myrtle by bulked segregant analysis. Genetics and Molecular Research, 14(2), 4369–4380. DOI 10.4238/2015.April.30.10. [Google Scholar] [CrossRef]
8. Zheng, T. C., Qin, B., Li, S. Z., Cai, M., Pan, H. T. et al. (2019). Screening of applicable SSR molecular markers linked to creeping trait in crape myrtle. Forests, 10(5), 429. DOI 10.3390/f10050429. [Google Scholar] [CrossRef]
9. Li, S. Z., Zheng, T. C., Zhuo, X. K., Li, Z. J., Li, L. L. et al. (2020). Transcriptome profiles reveal that gibberellin-related genes regulate weeping traits in crape myrtle. Horticulture Research, 7(1), 54. DOI 10.1038/s41438-020-0279-3. [Google Scholar] [CrossRef]
10. Pounders, C., Scheffler, B. E., Rinehart, T. A. (2013). ‘Ebony Embers’, ‘Ebony Fire’, ‘Ebony Flame’, ‘Ebony Glow’, and ‘Ebony and Ivory’ Dark-leaf crapemyrtles. HortScience, 48(12), 1568–1570. DOI 10.21273/HORTSCI.48.12.1568. [Google Scholar] [CrossRef]
11. Liang, Y., Li, D. C., Lu, X. X., Yang, X., Pan, X. Z. et al. (2010). Soil erosion changes over the past five decades in the red soil region of Southern China. Journal of Mountain Science, 7(1), 92–99. DOI 10.1007/s11629-010-1052-0. [Google Scholar] [CrossRef]
12. Sun, C. Q., Chen, F. D., Teng, N. J., Liu, Z. L., Fang, W. M. et al. (2010). Factors affecting seed set in the crosses between Dendranthema grandiflorum (Ramat.) Kitamura and its wild species. Euphytica, 171(2), 181–192. DOI 10.1007/s10681-009-0005-6. [Google Scholar] [CrossRef]
13. Chen, C., Yu, F. Y. (2020). Advances in flower bud differentiation of forest trees. Forest Science, 56(9), 119–129. DOI 10.11707 /j.1001-7488.20200914. [Google Scholar]
14. Fadón, E., Herrero, M., Rodrigo, J. (2015). Flower development in sweet cherry framed in the BBCH scale. Scientia Horticulturae, 192, 141–147. DOI 10.1016/j.scienta.2015.05.027. [Google Scholar] [CrossRef]
15. Zik, M., Irish, F. V. (2003). Flower development: Initiation, differentiation, and diversification. Annual Review of Cell and Developmental Biology, 19(1), 119–140. DOI 10.1146/annurev.cellbio.19.111301.134635. [Google Scholar] [CrossRef]
16. Brukhin, V., Hernould, M., Gonzalez, N., Chevalier, C., Mouras, A. (2003). Flower development schedule in tomato Lycopersicon esculentum cv. sweet cherry. Sexual Plant Reproduction, 15(6), 311–320. DOI 10.1007/s00497-003-0167-7. [Google Scholar] [CrossRef]
17. Fan, L. J., Chen, M. Q., Dong, B., Wang, N. H., Yu, Q. et al. (2018). Transcriptomic analysis of flower bud differentiation in magnolia sinostellata. Genes, 9(4), 212. DOI 10.3390/genes9040212. [Google Scholar] [CrossRef]
18. Jiang, Y. Y., Zhu, Y. M., Zhang, L., Su, W. B., Peng, J. R. et al. (2020). EjTFL1 genes pomote growth but inhibit flower bud differentiation in loquat. Frontiers in Plant Science, 11, 576. DOI 10.3389/fpls.2020.00576. [Google Scholar] [CrossRef]
19. Lee, S. Y., Lee, Y. H., Park, S. J., Lee, H., Lee, J. J. et al. (2016). Flower bud chloranthy disease caused by caeoma makinoi on Prunus mume. Korean Journal of Mycology, 44(4), 377–381. DOI 10.4489/KJM.2016.44.4.37710.4489. [Google Scholar] [CrossRef]
20. Hassankhah, A., Rahemi, M., Mozafari, M., Vahdati, K. (2018). Flower development in walnut: Altering the flowering pattern by gibberellic acid application. Notulae Botanicae Horti Agrobotanici Cluj-Napoca, 46(2), 700–706. DOI 10.15835/nbha46211183. [Google Scholar] [CrossRef]
21. Fang, S., Gao, K., Hu, W., Snider, J. L., Wang, S. et al. (2018). Chemical priming of seed alters cotton floral bud differentiation by inducing changes in hormones, metabolites and gene expression. Plant Physiology and Biochemistry, 130, 633–640. DOI 10.1016/j.plaphy.2018.08.010. [Google Scholar] [CrossRef]
22. Koutinas, N., Pepelyankov, G., Lichev, V. (2010). Flower induction and flower bud development in apple and sweet cherry. Biotechnology & Biotechnological Equipment, 24(1), 1549–1558. DOI 10.2478/V10133-010-0003-9. [Google Scholar] [CrossRef]
23. Pescie, M., Lovisolo, M., Magistris, A. D., Strik, B., López, C. et al. (2011). Flower bud initiation in southern highbush blueberry cv. O’Neal occurs twice per year in temperate to warm temperate conditions. Journal of Applied Horticulture, 13(1), 8–12. DOI 10.37855/jah.2011.v13i01.02. [Google Scholar] [CrossRef]
24. Guo, Y. M., Ye, Y. M., Huang, Y., Tong, J., Feng, B. et al. (2007). Studies on flower bud morphological differentiation of Lagerstroemia indica ‘Petite pinkie’. Northern Horticulture, 4, 149–151. [Google Scholar]
25. Xu, H., Ma, Y. Z., Zeng, H. J., Qiao, Z. Q., Wang, X. M. et al. (2015). Study on the changes of endogenous hormones during the flower organ differentiation of crape myrtle. Guangdong Agricultural Sciences, 42(2), 37–42. DOI 10.16768/j.issn.1004-874x.2015.02.020. [Google Scholar] [CrossRef]
26. Li, Z. W., Zeng, G. M., Zhang, H., Yang, B., Jiao, S. (2007). The integrated eco-environment assessment of the red soil hilly region based on GIS—A case study in Changsha City, China. Ecological Modelling, 202(3), 540–546. DOI 10.1016/j.ecolmodel.2006.11.014. [Google Scholar] [CrossRef]
27. Wei, H. L., Gao, C., Qiu, J., Guo, Z. Y., Wang, B. et al. (2022). Flower bud differentiation, Mega-micro spore production, and male-female gametophyte development in Camellia weiningensis. Journal of the American Society for Horticultural Science, 147(2), 104–115. DOI 10.21273/jashs05134-21. [Google Scholar] [CrossRef]
28. Patil, H. B., Chaurasia, A. K., Kumar, S., Krishna, B., Subramaniam, V. R. et al. (2022). Synchronized flowering in pomegranate, following pruning, is associated with expression of the flowering locus T homolog, PgFT1. Physiologia Plantarum, 174(1), e13620. DOI 10.1111/ppl.13620. [Google Scholar] [CrossRef]
29. Takahashi, H., Kamakura, H., Sato, Y., Shiono, K., Abiko, T. et al. (2010). A method for obtaining high quality RNA from paraffin sections of plant tissues by laser microdissection. Journal of Plant Research, 123(6), 807–813. DOI 10.1007/s10265-010-0319-4. [Google Scholar] [CrossRef]
30. Fu, P., Li, W. Y., Liu, M. L. (2020). Improvement of paraffin section preparation method of flower sequence of Vernicia fordii. Journal of Central South University of Forestry and Technology, 40(3), 53–59. DOI 10.14067/j.cnki.1673-923x.2020.03.007. [Google Scholar] [CrossRef]
Cite This Article
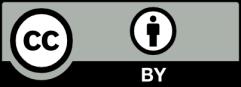
This work is licensed under a Creative Commons Attribution 4.0 International License , which permits unrestricted use, distribution, and reproduction in any medium, provided the original work is properly cited.