Open Access
ARTICLE
Arbuscular Mycorrhizal Fungal Diversity Associated with Olea europaea L. Growing in Yunnan Province, Southwestern China
Yunnan Academy of Forestry and Grassland, Kunming, 650201, China
* Corresponding Author: Yuebo Jing. Email:
(This article belongs to the Special Issue: Mycorrhizal Fungi and Sustainable Development of Agriculture)
Phyton-International Journal of Experimental Botany 2022, 91(12), 2719-2732. https://doi.org/10.32604/phyton.2022.021989
Received 16 February 2022; Accepted 19 April 2022; Issue published 29 August 2022
Abstract
Olive (Olea europaea L.) is one of the most important and widely cultivated fruit trees, with high economic, ecological, cultural and scientific value. China began introducing and cultivating olive in the 1960s, and Yunnan Province is one of the main growing areas. Improving the cultivation and productivity of this tree crop species is an important challenge. Olive is a typical mycotrophic species and the potential of arbuscular mycorrhizal fungi (AMF) for this plant is well recognized; nevertheless, studies of olive AMF in China are still very limited. Roots and rhizosphere soils of olive were sampled from five representative growing sites in the Yunnan Province of China to investigate the AMF colonization status in the root systems, the AMF community in the olive orchards and the edaphic factors influencing the arbuscular mycorrhizal (AM) parameters. Root samples of olive trees from different growing sites generally showed AMF colonization, suggesting that autochthonous AMF manifest a high efficiency in colonizing the roots of olive plants. The spore density on the five sites ranged from 81.6 to 350 spores per 20 g soil. Twenty-three AMF species from 9 genera were identified in total, and Glomeraceae was the dominant family. The findings of our study suggested a high AMF diversity harbored by olive growing in different areas of the Yunnan Province, Southwestern China. Furthermore, the hyphal colonization in roots positively correlated with soil pH and EC. The arbuscule colonization in olive roots negatively correlated with soil pH, EC, OM, TN, TP and AN. The spore density positively correlated with OM, TN, AN, AP and sand content. Finally, the Shannon index of AMF in the rhizosphere soil positively correlated with the clay content, but negatively correlated with soil pH, TN and silt content. The high diversity of autochthonous AMF in Yunnan is promising for screening AMF isolates for utilization in the efficient cultivation of this crop.Keywords
Arbuscular mycorrhizal fungi (AMF) comprise a main component of the soil microbiota, accounting for 5% to 50% of the biomass of soil microbes [1]. AMF can form symbiotic associations with the majority of terrestrial plants, including most crops [2–4], and have the beneficial ability to enhance host-plant uptake of water and soil nutrients and to improve the host plant’s tolerance to various biotic and abiotic stresses [2,5,6]. AMF may also improve soil aggregate stability by building up a macroporous structure of soil that allows penetration of water and air and prevents erosion [7]. Arbuscular mycorrhizal (AM) symbiosis is the most widespread association between plant roots and fungi in natural and agricultural ecosystems [8]; it is important for promoting the productivity of croplands and for the maintenance of biodiversity [9].
Olive (Olea europaea L.) is one of the world’s most important oil-producing tree crops and has been considered the most emblematic tree in the Mediterranean basin [10]; it is now spreading to many other new areas, such as Australia, Chile, Argentina and Peru, with an increasing international demand for olive oil and table olives [11]. China began importing olive seeds and seedlings from Albania in the 1960s and now cultivates olive trees in 14 provinces, mainly Gansu, Sichuan, and Yunnan [12], covering an area of 167,000 ha [13]. The olive plant is a highly mycotrophic species that rely on AM symbiosis [14–18]. Many studies have demonstrated the contribution of AMF to olive growth [19,20], nutrients uptake [21] and resilience to abiotic stress like drought [22,23] and salinity [24], and biotic stress like Verticillium wilt disease [25,26]. Information about AMF diversity in olive has been reported mostly from Mediterranean countries, which are the natural habitats of this species [16,27].
Understanding the diversity and community structure of AMF is an essential prerequisite for effectively managing and utilizing this important soil microorganism to promote the efficient cultivation of this crop. Currently, olive AMF research conducted in China is still very limited. In this context, it is important to study the AM status of olive at the field, explore the AMF community associated with this important tree crop species and know the edaphic parameters driving the AMF diversity and composition. Choosing five representative olive growing sites in the Yunnan Province of China for sampling, the objectives of this study were: (1) to determine the AM status of olive trees in the main growing sites of Yunnan; (2) to identify the AMF communities associated with olive growing at different geographical sites; and (3) to explore the relationships among the root AMF colonization, AMF community, and edaphic factors.
2.1 Location of Olive Orchards and Rhizosphere Sampling
Soil and root samples were collected from five commercial orchards located in representative olive introduction and cultivation areas along the Jinsha River, hot-arid valley in Yunnan Province, China (Fig. 1). Sites XYR (25°58′N 101°40′E, 1700 m asl.) and LYR (26°01′N 101°37′E, 1710 m asl.) were in Yongren County, SQL (27°16′N 100°16′E, 1724 m asl.) and TYL (27°18′N 100°15′E, 1774 m asl.) were in Lijiang County, and BZD (28°14′N 99°17′E, 2022 m asl.) was in Deqin County. All sites are characterized by a semi-arid subtropical monsoon climate with a dry winter, and rainfall occurring mainly in summer. The detailed climatic characteristics of these study sites were described in Ma et al. [28]. The soil type of the two sites in Yongren County was purple soil, and that of two sites in Lijiang County and one site in Deqin County was fluvisols. At sites XYR, LYR, SQL, TYL, and BZD, the sampled olive plantations were 5, 10, 11, 3, and 19 years old, respectively. The cultivars at XYR and LYR were ‘EZhi-8’, and those of orchards in SQL, TYL, and BZD were all ‘Frantoio’. Except for Deqin (rain-fed, fertilization in the first three years after planting), the other orchards were irrigated during the dry season and fertilized annually to obtain a high yield. The BZD orchard was not tilled, and the soil was covered by natural cover crops. The soil management of the other four sites was similar, and the soils received a shallow tillage to a low depth (0.1 m). Sampling was conducted in September 2015; at each of the sampling orchards, 5 individual plants were randomly chosen. For each sample tree, fine roots of olive and rhizosphere soil were collected from four directions (east, west, south, and north) around the tree using a small garden shovel (10–20 cm depth, because most of the olive roots were distributed in this layer and a greater microbial activity was detected), and the four subsamples were then mixed and homogenized to a composite sample (ca. 1000 g). Overall, twenty-five root samples and 25 soil samples were collected. The samples were placed in polyethylene bags and transported to the laboratory. Root samples were freshly processed for AMF colonization analysis. Soil samples were separated into two parts: the first part was for physicochemical analysis, and the second part was air-dried and stored at 4°C for isolation and identification of AM fungal spores.
Figure 1: Map showing the locations of the five sampled olive orchards in the Yunnan Province, Southwestern China. XYR and LYR in Yongren County, SQL and TYL in Lijiang County, BZD in Deqin County
2.2 Analysis of Soil Properties
Soil samples were air-dried at room temperature and homogenized by sieving through a 2-mm mesh to remove plant debris and coarse sand. A pH meter was used for potentiometric pH measurements of a soil-water suspension at a ratio of 1:2.5. The organic matter content (OM) was determined using potassium dichromate as an oxidizing agent in the presence of sulfuric acid. Total soil nitrogen (TN) was determined after digestion with sulfuric acid, and analysis of the amount was performed by an automatic Kjeldahl nitrogen analyzer. Total soil phosphorus (TP) was determined by alkali fusion-antimony molybdenum spectrophotometry. Total soil potassium (TK) was digested in a microwave system and determined by an atomic absorption spectrophotometer. Soil available nitrogen (AN) was determined by the alkali solution diffusion method. Soil available phosphorus (AP) was extracted with sodium bicarbonate and determined by antimony molybdenum ultraviolet spectrophotometry. Soil available potassium (AK) was extracted with ammonium acetate and determined by flame photometry. The soil electrical conductivity (EC) was determined by the electrode method. The detailed methods for soil chemical analysis are fully described by Du et al. [29]. The particle size analysis was conducted by the pipette method using the U.S. Department of Agriculture System [30].
2.3 Determination of AM Fungal Colonization in Olive Roots
Fine roots were rinsed with clean water, cleared with 10% (w/v) KOH at 90°C in a water bath for approximately 60 min. After cooling to room temperature, root samples were thoroughly washed with tap water, stained with blue ink (Hero® 203, Shanghai, China), mounted on microscope slides, and then examined under a compound light microscope (Olympus-BX53, Olympus Corporation, Tokyo, Japan) to determine AM fungal structures. The percentage of root length occupied by hyphae, arbuscules, and vesicles was quantified for at least 200 intersections using the modified line intersection method [31]. The percentages of root length with AMF hyphae, arbuscules, and vesicles were calculated as the number of AMF-positive intersections/number of observed intersections × 100.
2.4 AMF Spore Assessment and Identification
AMF spores were extracted from 20 g of air-dried, rhizosphere soil samples by the wet sieving and decanting method [32], collected on filter paper, and counted, characterized, and identified under a stereomicroscope and compound microscope. Spore density was calculated as the total number of spores in the 20 g soil sample, and a sporecarp was recorded as one unit. Each spore type was mounted in PVLG (polyvinyl-lactoglycerol) and PVLG/Melzer’s reagent.
Identification was based on spore color, size, surface ornamentations, wall structure, and hyphal attachments with reference to the manual of Schenck et al. [33], the originally published species descriptions, and information provided by INVAM (https://invam.ku.edu/species-descriptions). To assess AMF community composition and structure, the relative abundance, Shannon index, and Simpson index were calculated.
In the present study, spore density (SD) was defined as the number of AM fungal spores in 20 g dry soil; relative abundance (RA) = spore number of a certain species/total quantity of AM fungal spores × 100%. Species diversity was assessed using the Shannon index and Simpson index. Variations in soil physicochemical characteristics were analyzed using the Kruskal–Wallis non-parametric test. The AMF parameter data were subjected to an analysis of variance (ANOVA) to compare the mean values, and differences between treatment means were determined using the Duncan’s test (P < 0.05). The relationships between edaphic factors and AMF parameters were examined using Pearson’s correlation coefficient.
3.1 Soil Physicochemical Properties
The physicochemical properties of the soils of different olive growing sites are presented in Table 1. The pH, OM, AN, AP, AK, TN, TP, TK, EC, clay percentage, sand percentage, and silt percentage were significantly different among the five investigated orchards (P < 0.05). OM, AN and AP values were highest in the orchard of BZD and lowest in the orchard of XYR.
3.2 AM Fungal Colonization Characteristics in Roots of Olive
AMF structures were present in all sampled roots, including intra- and intercellular hyphae, hyphal coils, arbuscules, and vesicles (Fig. 2). The intensity of mycorrhizal colonization of the root samples varied with different growing sites. The percentages of root length with hyphae (% RLH), arbuscles (% RLA) or vesicles (% RLV) varied widely between 15.14% and 75.77%, 3.87% and 34.13% or 30.83% and 51.12%, respectively (Fig. 3). The percentages of RLH and RLV of olive trees growing in the two sites of Lijiang County (SQL and TYL) were higher than those of olive trees sampled in Yongren County (XYR and LYR) and Deqin County (BZD). The only exception was the percentage on RLH which was equal at XYR and TYL (Fig. 3). The percentage of RLA of olive trees sampled in Yongren County (XYR and LYR) was higher than those of SQL, TYL, and BZD (Fig. 3).
Figure 2: Light micrographs showing mycorrhizal colonization in roots of olive plants, H: hypha, HC: hyphal coil, A: arbuscule, V: vesicle
Figure 3: AMF attributes on olive orchards from different study sites. Root length colonization percentage of AMF hyphae (a), arbuscules (b), and vesicles (c) in roots of olive trees, AMF spore densities (d) (spore number/20 g soil), Shannon index (e), and Simpson index (f) of AMF communities in rhizosphere soil samples from different sampling sites. Histograms are the mean ± SD. Different letters on the histograms indicate significant differences among sites according to the Duncan’s multiple range test at P < 0.05
3.3 Morphological Identification of AM Fungi in the Olive Rhizosphere Soil
Twenty-three AM fungal species belonging to 9 genera were identified according to the morphological characteristics of spores extracted from the rhizosphere soil samples (Table 2). Seven of the 23 species belonged to Acaulospora; one species belonged to Diversispora; two species belonged to Funneliformis; one species belonged to Gigaspora; seven species belonged to Glomus; one species belonged to Rhizophagus; two species belonged to Sclerocystis; one species belonged to Scutellospora and one species belonged to Septoglomus (Table 2). Micrographs of some AMF spores are shown in Fig. 4.
Figure 4: AMF spores isolated from rhizosphere soils of olive trees growing in different sites in the Yunnan Province. (a) Acaulospora morrowiae, (b) A. paulinae, (c) Diversispora trimurales, (d) Funneliformis geosporum, (e) F. mosseae, (f) Glomus hyderabadensis, (g) G. magnicaule, (h) G. multicaule, (i) Rhizophagus intraradices, (j) Sclerocystis rubiformis, (k) S. sinuosa, (l) Septoglomus constrictum
5, 9, 8, 11, and 7 species of AM fungi were detected in the olive orchards at XYR, LYR, SQL, TYL, and BZD, respectively (Table 2). F. geosporum was the only species that occurred in all olive orchards. The most dominant species in the soil samples of XYR were F. geosporum, G. microcarpum, and S. sinuosa. At site LYR, F. geosporum, Sep. constrictum, and A. sp.3 were the most dominant species. At site SQL, F. geosporum, Sep. constrictum, and G. microcarpum were the most dominant species. At site TYL, the most dominant species were Sep. constrictum, F. geosporum, and G. microcarpum. At site BZD, Sep. constrictum was the most dominant species (Table 2).
As shown in Table 2, most species were detected only in particular orchards. G. magnicaule occurred only at XYR; A. capsicula, A. morrowiae, A. paulinae, A. sp.3 and Gigaspora sp.1 were exclusively found in LYR; G. hyderabadensis, Glomus sp.1 and Glomus sp.2 were detected only in SQL; D. trimurales, G. multicaule, Glomus sp.3, R. intraradices and Scu. sp.1 occurred only in TYL, and A. foveata and S. rubiformis were found only in BZD. All the AMF species showed different abundances of AMF spores among the investigated orchards (P < 0.0003).
The AMF spore densities at sites XYR, LYR, SQL, TYL, and BZD were 81.6, 121.2, 116.0, 198.2, and 350.0 spores per 20 g soil, respectively (Fig. 3). The spore density at BZD was the highest, significantly higher than those at the other four sites, followed by TYL, which was significantly lower than that of BZD and significantly higher than those of XYR, LYR, and SQL. The Shannon index of the AMF composition of site LYR was 2.66, which was the highest, followed by SQL. The Shannon index of BZD was 1.29, which was the lowest among all the examined sites. The Shannon index for each site varied significantly. The Simpson indexes of the AMF composition of sites XYR, LYR, SQL, TYL, and BZD were 0.66, 0.81, 0.78, 0.41, and 0.42, respectively, those of LYR and SQL were significantly higher than other three study sites (Fig. 3).
3.4 Relationship between Soil Parameters and Arbuscular Mycorrhizal Fungal Attributes
Except for total and available potassium, the other examined soil physicochemical properties affected the AMF parameters of olive, and the influences varied. Hyphal colonization in olive roots was positively influenced by soil pH and EC. The arbuscule colonization in olive roots was negatively influenced by most of the properties, including soil pH, EC, OM, TN, TP, and AN. Vesicle colonization was not significantly correlated with any of the soil physicochemical properties. Spore density was positively influenced by OM, TN, AN, AP, and sand content and negatively influenced by clay content. The Shannon index and Simpson index of AMF in the rhizosphere soil were positively influenced by the clay content in the soil. The Shannon index of AMF in the rhizosphere soil was negatively influenced by soil pH, TN, and silt content (Table 3). Finally, the Simpson index of AMF in the rhizosphere soil was negatively influenced by pH, OM, and TN.
This study highlighted the olive root colonization by AMF at different growing sites of the Yunnan Province, Southwestern China. This mycorrhizal colonization in the roots of olive plants is the result of AMF spores that developed in areas with specific conditions, as a result of adaptation to the environment and identity to the host plant. The intensity of AMF hyphal colonization of olive trees ranged from 15.14% to 75.77%. These values are higher than those reported for ten-year-old olive trees in Italy (22.5% to 31.3%) [34] and comparable to a field study carried out in Algeria (35% to 47%) [35]. The intensity of AMF hyphal colonization in our study is lower than values reported for olive rooted cuttings artificially inoculated with various AMF: 75% to 80% [36], 92% to 97% [21] and 97% [18]. The different values in the percentage of colonized root length obtained for our olive trees may be mainly attributed to either different plant ages, geographical sites, or the fungal species that colonized the roots.
Mycorrhizal parameters of the olive tree also revealed the importance of AMF. Notably, our study revealed that arbuscule colonization intensity was significantly higher than that in previous studies. Mekahlia et al. [17] explored root AMF colonization intensity in olive orchards from three different sites in Algeria and discovered that the colonization intensity of arbuscules was extremely low, with the highest intensity of 4% only among all the study root samples. In our study, the arbuscule intensities of XYR, LYR, SQL, TYL, and BZD were 22.14%, 34.13%, 15.47%, 12.69%, and 3.87%, respectively (Fig. 3). Arbuscules represent the sites of functional exchanges between the AMF and the roots of the host plant [37], suggesting the critical role of AMF native to the growing sites of the Yunnan Province.
The spore density in a young plantation (6–7 years) located in Ain Ben Beida of Algeria was 648 spores/10 g of soil, as reported by Meddad-Hamza et al. [35]. In addition, they identified 10 species belonging to 7 genera—Glomus, Septoglomus, Funneliformis, Rhizophagus, Gigaspora, Scutellospora, and Entrophospora—in their survey on different aged orchards located at different geographical sites.
Most of the AMF isolated in this study have been identified from the soil of olive orchards. In particular, A. foveata, A. morrowiae, F. geosporum, F. mosseae, G. hyderabadensis, G. microcarpum, G. multicaule, R. intraradices, and Sep. constrictum were reported by Chliyeh et al. [38] and Msairi et al. [39] in the olive orchards of Morocco. F. mosseae and Sep. constrictum are very common AMF species associated with olive and are generally isolated in different olive growing areas of the world [35,40–42]. Notably, three species were reported for the first time in our study from the rhizospheres of olive trees: A. capsicula, A. paulinae, and G. magnicaule.
Our study results showed that Glomeraceae was the dominant taxon of AMF in the sampled olive orchards from five different growing sites, with relative abundances of 95.54%, 59.03%, 95.7%, 95.54%, and 95.99% at sites XYR, LYR, SQL, TYL, and BZD, respectively. This is consistent with previous studies on the AMF composition of olive. Chliyeh et al. [38] reported that the genus Glomus was dominant in the rhizosphere soil of olive trees (74%) 30 months after inoculation of a composite, native AMF community; Glomeraceae species were prevalent in the rhizosphere of olive trees cultivated along a climatic gradient north–south of Algeria [35]. A study on olive orchards in Spain also revealed that Glomeraceae was the dominant taxa [16]. All these findings support previous data on the prevalence of members of such a family in agricultural soils, because of their ruderal lifestyle and high tolerance to disturbance [43–45].
It is generally accepted that edaphic properties, such as soil pH, P, N, and OM, have a key role in controlling the pool of AMF in soils [35,46–50]. In the present study, we noted that soil pH and EC were positively correlated with AMF hyphal colonization in olive roots, and soil OM, TN, AN, AP, and sand (%) were positively correlated with AMF spore density in olive rhizosphere soil. The clay content was positively correlated with the Shannon index and Simpson index of the AMF composition in the rhizosphere of olive trees. In contrast, clay (%) was determined to be negatively correlated with the AMF spore density in olive rhizosphere soil, and silt (%) was revealed to be negatively correlated with the Shannon index of AMF composition in the olive rhizosphere. In a study conducted on olive orchards in Spain, Montes-Borrego et al. [51] identified that the C/N ratio, soil C, OM, soil pH, clay, sand, N content, and extractable P were the most important edaphic variables showing a significant effect on the AMF community composition. The data obtained in our work reinforce the concept that the general AMF assemblage structure and composition in olive might be influenced by soil properties, as has been shown in other woody crops [52,53].
A high AMF colonization level was revealed in the roots of olive planted in the main introduction and cultivation areas of the Yunnan Province, southwestern China; a high AMF diversity (23 species of 9 genera) was identified with a relatively high spore content. Glomeraceae was the most dominant taxon in the olive rhizosphere of the sampling sites. Soil parameters had significant effects on olive root mycorrhizal colonization, spore density, AMF diversity, and composition. In conclusion, this study emphasized the existence and importance of AM in olive growing out of its traditional cultivation area, and provided some data and information for mycorrhizal technology development. Further research is required to explore more about the AMF diversity of olive, screening out the potential efficient AMF species/isolates, which will in turn facilitate the cultivation and development of this important crop.
Acknowledgement: The authors would like to thank Research Prof. Delu Ning and Associate Research Prof. Ting Ma of Yunnan Academy of Forestry and Grassland, for helping collect the samples. We also thank Ms. Liqun Yang and Ms. Feng’e Zhang of Yunnan Academy of Forestry and Grassland, for their assistance in the laboratory work.
Funding Statement: This work was supported by the National Natural Science Foundation of China (31760222), State Forestry Administration of China 948 Project “Introduction of Olive Water and Fertilizer Precise Control Technology” (2014-4-23) and the “Plant Nutrition and Mycorrhiza Research” Discipline Team Building Project of Yunnan Academy of Forestry and Grassland (LKYTD-2018-6).
Conflicts of Interest: The authors declare that they have no conflicts of interest to report regarding the present study.
References
1. Olsson, P., Thingstrup, I., Jakobsen, I., Bååth, E. (1999). Estimation of the biomass of arbuscular mycorrhizal fungi in a linseed field. Soil Biology and Biochemistry, 31(13), 1879–1887. DOI 10.1016/S0038-0717(99)00119-4. [Google Scholar] [CrossRef]
2. Smith, S., Read, D. (2008). Mycorrhizal symbiosis. Oxford, UK: Academic Press. [Google Scholar]
3. Calaça, F. J. S., Bustamante, M. M. C. (2022). Richness of arbuscular mycorrhizal fungi (Glomeromycota) along a vegetation gradient of Brazilian Cerrado: Responses to seasonality, soil types, and plant communities. Mycological Progress, 21(2), 27. DOI 10.1007/s11557-022-01785-1. [Google Scholar] [CrossRef]
4. Yilmaz, A., Karik, Ü (2022). AMF and PGPR enhance yield and secondary metabolite profile of basil (Ocimum basilicum L.). Industrial Crops and Products, 176(3), 114327. DOI 10.1016/j.indcrop.2021.114327. [Google Scholar] [CrossRef]
5. Pereira, C. M. R., da Silva, D. K. A., de Almeida Ferreira, A. C., Goto, B. T., Maia, L. C. (2014). Diversity of arbuscular mycorrhizal fungi in Atlantic forest areas under different land uses. Agriculture, Ecosystems & Environment, 185, 245–252. DOI 10.1016/j.agee.2014.01.005. [Google Scholar] [CrossRef]
6. Raklami, A., Bechtaoui, N., Tahiri, A. I., Anli, M., Meddich, A. et al. (2019). Use of rhizobacteria and mycorrhizae consortium in the open field as a strategy for improving crop nutrition, productivity and soil fertility. Frontiers in Microbiology, 10, 1106. DOI 10.3389/fmicb.2019.01106. [Google Scholar] [CrossRef]
7. Rajeshkumar, P. P., Thomas, G. V., Gupta, A., Gopal, M. (2015). Diversity, richness and degree of colonization of arbuscular mycorrhizal fungi in coconut cultivated along with intercrops in high productive zone of Kerala, India. Symbiosis, 65(3), 125–141. DOI 10.1007/s13199-015-0326-2. [Google Scholar] [CrossRef]
8. Salvioli, A., Zouari, I., Chalot, M., Bonfante, P. (2012). The arbuscular mycorrhizal status has an impact on the transcriptome profile and amino acid composition of tomato fruit. BMC Plant Biology, 12(1), 44. DOI 10.1186/1471-2229-12-44. [Google Scholar] [CrossRef]
9. Croll, D., Sanders, I. (2009). Recombination in Glomus intraradices, a supposed ancient asexual arbuscular mycorrhizal fungus. BMC Evolutionary Biology, 9(1), 13. DOI 10.1186/1471-2148-9-13. [Google Scholar] [CrossRef]
10. Besnard, G., Terral, J. F., Cornille, A. (2017). On the origins and domestication of the olive: A review and perspectives. Annals of Botany, 121(3), 385–403. DOI 10.1093/aob/mcx145. [Google Scholar] [CrossRef]
11. Torres, M., Pierantozzi, P., Searles, P., Rousseaux, M. C., Garcia-Inza, G. et al. (2017). Olive cultivation in the southern hemisphere: Flowering, water requirements and oil quality responses to new crop environments. Frontiers in Plant Science, 8, 1830. DOI 10.3389/fpls.2017.01830. [Google Scholar] [CrossRef]
12. Rao, G., Zhang, J., Liu, X., Lin, C., Xin, H. et al. (2021). De novo assembly of a new Olea europaea genome accession using nanopore sequencing. Horticulture Research, 8(1), 64. DOI 10.1038/s41438-021-00498-y. [Google Scholar] [CrossRef]
13. Li, J. Z. (2018). Olive introduction and industrial development in China. Beijing: China Forestry Publishing House. [Google Scholar]
14. Hayman, D. S., Barea, J. M., Azcon, R. (1976). Vesicular-arbuscular mycorrhiza in Southern Spain: Its distribution in crops growing in soil of different fertility. Phytopathologia Mediterranea, 15(1), 1–6. [Google Scholar]
15. Roldán-Fajardo, B., Barea, J. (1986). Mycorrhizal dependency in the olive tree (Olea europaea L.). In: Gianninazzi-Pearson, V., Gianninazzi, S. (Eds.Les mycorhizes: Physiologie et gènètique. INRA, Paris. [Google Scholar]
16. Calvente, R., Cano, C., Ferrol, N., Azcón-Aguilar, C., Barea, J. M. (2004). Analysing natural diversity of arbuscular mycorrhizal fungi in olive tree (Olea europaea L.) plantations and assessment of the effectiveness of native fungal isolates as inoculants for commercial cultivars of olive plantlets. Applied Soil Ecology, 26(1), 11–19. DOI 10.1016/j.apsoil.2003.10.009. [Google Scholar] [CrossRef]
17. Mekahlia, M. N., Beddiar, A., Chenchouni, H. (2013). Mycorrhizal dependency in the olive tree (Olea europaea) across a xeric climatic gradient. Advances in Environmental Biology, 7(9), 2166–2174. [Google Scholar]
18. Briccoli Bati, C., Santilli, E., Lombardo, L. (2015). Effect of arbuscular mycorrhizal fungi on growth and on micronutrient and macronutrient uptake and allocation in olive plantlets growing under high total Mn levels. Mycorrhiza, 25(2), 97–108. DOI 10.1007/s00572-014-0589-0. [Google Scholar] [CrossRef]
19. Dag, A., Yermiyahu, U., Ben-Gal, A., Zipori, I., Kapulnik, Y. (2009). Nursery and post-transplant field response of olive trees to arbuscular mycorrhizal fungi in an arid region. Crop and Pasture Science, 60(5), 427–433. DOI 10.1071/CP08143. [Google Scholar] [CrossRef]
20. Bompadre, M. J., Pérgola, M., Fernández Bidondo, L., Colombo, R. P., Silvani, V. A. et al. (2014). Evaluation of arbuscular mycorrhizal fungi capacity to alleviate abiotic stress of olive (Olea europaea L.) plants at different transplant conditions. The Scientific World Journal, 2014, 378950. DOI 10.1155/2014/378950. [Google Scholar] [CrossRef]
21. Porras-Soriano, A., Soriano-Martín, M. L., Porras-Piedra, A., Azcón, R. (2009). Arbuscular mycorrhizal fungi increased growth, nutrient uptake and tolerance to salinity in olive trees under nursery conditions. Journal of Plant Physiology, 166(13), 1350–1359. DOI 10.1016/j.jplph.2009.02.010. [Google Scholar] [CrossRef]
22. Calvo-Polanco, M., Sánchez-Castro, I., Cantos, M., García, J. L., Azcón, R. et al. (2016). Effects of different arbuscular mycorrhizal fungal backgrounds and soils on olive plants growth and water relation properties under well-watered and drought conditions. Plant, Cell and Environment, 39(11), 2498–2514. DOI 10.1111/pce.12807. [Google Scholar] [CrossRef]
23. Ouledali, S., Ennajeh, M., Zrig, A., Gianinazzi, S., Khemira, H. (2018). Estimating the contribution of arbuscular mycorrhizal fungi to drought tolerance of potted olive trees (Olea europaea). Acta Physiologiae Plantarum, 40(5), 81. DOI 10.1007/s11738-018-2656-1. [Google Scholar] [CrossRef]
24. Ben Hassena, A., Zouari, M., Trabelsi, L., Decou, R., Ben Amar, F. et al. (2021). Potential effects of arbuscular mycorrhizal fungi in mitigating the salinity of treated wastewater in young olive plants (Olea europaea L. cv. Chetoui). Agricultural Water Management, 245, 106635. DOI 10.1016/j.agwat.2020.106635. [Google Scholar] [CrossRef]
25. Boutaj, H., Chakhchar, A., Meddich, A., Wahbi, S., El Alaoui-Talibi, Z. et al. (2021). Mycorrhizal autochthonous consortium induced defense-related mechanisms of olive trees against Verticillium dahliae. Journal of Plant Diseases and Protection, 128(1), 225–237. DOI 10.1007/s41348-020-00365-3. [Google Scholar] [CrossRef]
26. Arici, E., Demirtas, A. (2019). The effectiveness of rhizosphere microorganisms to control Verticillium wilt disease caused by Verticillium dahliae Kleb. in olives. Arabian Journal of Geosciences, 12, 781. DOI 10.1007/s12517-019-4962-3. [Google Scholar] [CrossRef]
27. Theocharis, C., Michail, O., Dimitrios, A., Ioannis, T. (2013). Colonization of Greek olive cultivars’ root system by arbuscular mycorrhiza fungus: Root morphology, growth, and mineral nutrition of olive plants. Scientia Agricola, 70(3), 185–194. DOI 10.1590/S0103-90162013000300007. [Google Scholar] [CrossRef]
28. Ma, T., Ning, D., Li, Y. (2020). Analysis on the climatic factors of the main growing areas of Olea europaea L. in Yunnan Province. Forest Science and Technology, (6), 26–28. DOI 10.13456/j.cnki.lykt.2019.04.29.0003. [Google Scholar] [CrossRef]
29. Du, S., Gao, X., Ma, C., Shen, T., Bai, Y. et al. (2006). Technical specification for soil analysis. Beijing: China Agriculture Press. [Google Scholar]
30. Singer, M. J., Janitzky, P. (1986). Field and laboratory procedures used in a soil chronosequence study. Washington DC: US Government Printing Office. [Google Scholar]
31. McGonigle, T. P., Miller, M. H., Evans, D. G., Fairchild, G. L., Swan, J. A. (1990). A new method which gives an objective measure of colonization of roots by vesicular-arbuscular mycorrhizal fungi. New Phytologist, 115(3), 495–501. DOI 10.1111/j.1469-8137.1990.tb00476.x. [Google Scholar] [CrossRef]
32. Gerdemann, J. W., Nicolson, T. H. (1963). Spores of mycorrhizal Endogone species extracted from soil by wet sieving and decanting. Transactions of the British Mycological Society, 46(2), 235–244. DOI 10.1016/S0007-1536(63)80079-0. [Google Scholar] [CrossRef]
33. Schenck, N. C., Pérez, Y. (1990). Manual for the identification of VA mycorrhizal fungi. Gainesville, USA: Synergistic Publications. [Google Scholar]
34. Turrini, A., Caruso, G., Avio, L., Gennai, C., Palla, M. et al. (2017). Protective green cover enhances soil respiration and native mycorrhizal potential compared with soil tillage in a high-density olive orchard in a long term study. Applied Soil Ecology, 116(7), 70–78. DOI 10.1016/j.apsoil.2017.04.001. [Google Scholar] [CrossRef]
35. Meddad-Hamza, A., Hamza, N., Neffar, S., Beddiar, A., Gianinazzi, S. et al. (2017). Spatiotemporal variation of arbuscular mycorrhizal fungal colonization in olive (Olea europaea L.) roots across a broad mesic-xeric climatic gradient in North Africa. Science of the Total Environment, 583, 176–189. DOI 10.1016/j.scitotenv.2017.01.049. [Google Scholar] [CrossRef]
36. Estaún, V., Camprubí, A., Calvet, C., Pinochet, J. (2003). Nursery and field response of olive trees inoculated with two arbuscular mycorrhizal fungi, Glomus intraradices and Glomus mosseae. Journal of the American Society for Horticultural Science, 128(5), 767–775. DOI 10.21273/JASHS.128.5.0767. [Google Scholar] [CrossRef]
37. Ferrol, N., Barea, J. M., Azcón-Aguilar, C. (2002). Mechanisms of nutrient transport across interfaces in arbuscular mycorrhizas. Plant and Soil, 244(1), 231–237. DOI 10.1023/A:1020266518377. [Google Scholar] [CrossRef]
38. Chliyeh, M., Kachkouch, W., Zouheir, T., Touhami, A., Abdelkarim, F. M. et al. (2016). Evolution of a composite endomycorrhizal inoculums in function of time in the level of the olive plants rhizosphere. International Journal of Advances in Pharmacy, Biology and Chemistry, 5(1), 1–14. [Google Scholar]
39. Msairi, S., Chliyeh, M., Artib, M., El Gabardi, S., Selmaoui, K. et al. (2020). Effect of endomycorrhizal inoculum on the growth and protection of olive plants against Phytophthora palmivora. Tree Planters’ Notes, 63, 19–28. [Google Scholar]
40. Citernesi, A. S., Vitagliano, C., Giovannetti, M. (1998). Plant growth and root system morphology of Olea europaea L. rooted cuttings as influenced by arbuscular mycorrhizas. The Journal of Horticultural Science and Biotechnology, 73(5), 647–654. DOI 10.1080/14620316.1998.11511028. [Google Scholar] [CrossRef]
41. Mohammad, M. J., Hamad, S. R., Malkawi, H. I. (2003). Population of arbuscular mycorrhizal fungi in semi-arid environment of Jordan as influenced by biotic and abiotic factors. Journal of Arid Environments, 53(3), 409–417. DOI 10.1006/jare.2002.1046. [Google Scholar] [CrossRef]
42. Querejeta, J. I., Allen, M. F., Caravaca, F., Roldán, A. (2006). Differential modulation of host plant δ13C and δ18O by native and nonnative arbuscular mycorrhizal fungi in a semiarid environment. New Phytologist, 169(2), 379–387. DOI 10.1111/j.1469-8137.2005.01599.x. [Google Scholar] [CrossRef]
43. Jansa, J., Mozafar, A., Anken, T., Ruh, R., Sanders, I. et al. (2002). Diversity and structure of AMF communities as affected by tillage in a temperate soil. Mycorrhiza, 12(5), 225–234. DOI 10.1007/s00572-002-0163-z. [Google Scholar] [CrossRef]
44. Oehl, F., Sieverding, E., Ineichen, K., Ris, E. A., Boller, T. et al. (2005). Community structure of arbuscular mycorrhizal fungi at different soil depths in extensively and intensively managed agroecosystems. New Phytologist, 165(1), 273–283. DOI 10.1111/j.1469-8137.2004.01235.x. [Google Scholar] [CrossRef]
45. Turrini, A., Agnolucci, M., Palla, M., Tomé, E., Tagliavini, M. et al. (2017). Species diversity and community composition of native arbuscular mycorrhizal fungi in apple roots are affected by site and orchard management. Applied Soil Ecology, 116, 42–54. DOI 10.1016/j.apsoil.2017.03.016. [Google Scholar] [CrossRef]
46. Abbott, L. K., Robson, A. D. (1991). Factors influencing the occurrence of vesicular-arbuscular mycorrhizas. Agriculture, Ecosystems & Environment, 35(2), 121–150. DOI 10.1016/0167-8809(91)90048-3. [Google Scholar] [CrossRef]
47. Ji, B., Bentivenga, S. P., Casper, B. B. (2012). Comparisons of AM fungal spore communities with the same hosts but different soil chemistries over local and geographic scales. Oecologia, 168(1), 187–197. DOI 10.1007/s00442-011-2067-0. [Google Scholar] [CrossRef]
48. Silva, I. R. D., de Mello, C. M. A., Ferreira Neto, R. A., da Silva, D. K. A., de Melo, A. L. et al. (2014). Diversity of arbuscular mycorrhizal fungi along an environmental gradient in the Brazilian semiarid. Applied Soil Ecology, 84, 166–175. DOI 10.1016/j.apsoil.2014.07.008. [Google Scholar] [CrossRef]
49. Wang, Q., Bao, Y., Liu, X., Du, G. (2014). Spatio-temporal dynamics of arbuscular mycorrhizal fungi associated with glomalin-related soil protein and soil enzymes in different managed semiarid steppes. Mycorrhiza, 24(7), 525–538. DOI 10.1007/s00572-014-0572-9. [Google Scholar] [CrossRef]
50. Yu, J., Xue, Z., He, X., Liu, C., Steinberger, Y. (2017). Shifts in composition and diversity of arbuscular mycorrhizal fungi and glomalin contents during revegetation of desertified semiarid grassland. Applied Soil Ecology, 115, 60–67. DOI 10.1016/j.apsoil.2017.03.015. [Google Scholar] [CrossRef]
51. Montes-Borrego, M., Metsis, M., Landa, B. (2014). Arbuscular mycorhizal fungi associated with the olive crop across the Andalusian landscape: Factors driving community differentiation. PLoS One, 9(5), e96397. DOI 10.1371/journal.pone.0096397. [Google Scholar] [CrossRef]
52. Schreiner, R. P., Mihara, K. L. (2009). The diversity of arbuscular mycorrhizal fungi amplified from grapevine roots (Vitis vinifera L.) in Oregon vineyards is seasonally stable and influenced by soil and vine age. Mycologia, 101(5), 599–611. DOI 10.3852/08-169. [Google Scholar] [CrossRef]
53. Gao, X., Lv, J., Guo, C., Hu, A., Wu, X. et al. (2021). Species diversity of arbuscular mycorrhizal fungi in the rhizosphere of Hevea brasiliensis in Hainan Island, China. Phyton-International Journal of Experimental Botany, 90(1), 179–192. DOI 10.32604/phyton.2021.012968. [Google Scholar] [CrossRef]
Cite This Article
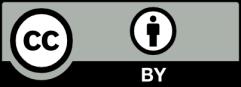
This work is licensed under a Creative Commons Attribution 4.0 International License , which permits unrestricted use, distribution, and reproduction in any medium, provided the original work is properly cited.